- 1Artialis S.A., Liège, Belgium
- 2musculoSKeletal Innovative Research Lab (mSKIL), Center for Interdisciplinary Research on Medicines, University of Liège, Liège, Belgium
- 3GeneQuine Biotherapeutics GmbH, Hamburg, Germany
- 4Department of Physical Therapy and Rehabilitation, Princess Paola Hospital, Vivalia, Marche-en-Famenne, Belgium
Objective: Osteoarthritis (OA) is the most common degenerative joint disease, characterized by a progressive loss of cartilage associated with synovitis and subchondral bone remodeling. There is however no treatment to cure or delay the progression of OA. The objective of this manuscript was to provide a scoping review of the preclinical and clinical studies reporting the effect of gene therapies for OA.
Method: This review followed the JBI methodology and was reported in accordance with the PRISMA-ScR checklist. All research studies that explore in vitro, in vivo, or ex vivo gene therapies that follow a viral or non-viral gene therapy approach were considered. Only studies published in English were included in this review. There were no limitations to their date of publication, country of origin, or setting. Relevant publications were searched in Medline ALL (Ovid), Embase (Elsevier), and Scopus (Elsevier) in March 2023. Study selection and data charting were performed by two independent reviewers.
Results: We found a total of 29 different targets for OA gene therapy, including studies examining interleukins, growth factors and receptors, transcription factors and other key targets. Most articles were on preclinical in vitro studies (32 articles) or in vivo animal models (39 articles), while four articles were on clinical trials related to the development of TissueGene-C (TG-C).
Conclusion: In the absence of any DMOAD, gene therapy could be a highly promising treatment for OA, even though further development is required to bring more targets to the clinical stage.
1. Introduction
Osteoarthritis (OA) is the most common degenerative joint disease (1). Traditionally considered as a disease of “wear and tear”, OA is now considered as a complex disorder affecting the whole joint and involving pro-inflammatory immune pathways (2, 3). For the patients, it is associated with a significant handicap and alteration of their quality of life. Currently, this pathology affects 500 million people worldwide and therefore contributes highly to the costs of the health and social care (4, 5).
Despite the high prevalence of OA, there is no treatment to cure or delay OA. Currently, medical treatment focuses on symptoms relief with painkillers and anti-inflammatory drugs (6, 7). Recommendations for OA non-surgical treatments are divided into non-pharmacological and pharmacological interventions with the purpose to reduce pain and joint stiffness and maintaining and improving mobility. The pharmacological treatments are dependent on patients' preferences, its phenotype, the severity of the diseases, and the presence of co-morbidities (8). In the absence of a disease-modifying OA drug (DMOAD), clinical guidelines suggest physical therapy, education, and weight management as a core treatment with pharmacological intervention if needed (9, 10).
The development of an effective treatment for OA is highly challenging. Recent advances have been made in the development of a range of biological drugs that position gene therapy as a promising option to overcome the limitations of traditional therapeutics in OA (11, 12). Gene therapy has the advantage of local delivery and, therefore, local production of therapeutic proteins for targeted, local treatment of the joint, along with a potentially reduced risk of systemic adverse events and drug-drug interactions (13). In addition, relatively long-term expression of the target gene can be achieved, thus avoiding repeated intra-articular (IA) injections.
While viral gene transfer is more efficient, non-viral gene delivery is considered safer (14, 15). It can involve lipid-based systems, polymers, nanoparticles, natural components, or simple plasmids (14, 15). Viral therapy, on the other hand, involves the administration of viral vectors, such as adenovirus (Ad), helper-dependent adenovirus (HDAd or HDV), adeno-associated virus (AAV), and retroviruses (RV) including lentivirus (LV) (13). Both non-viral and viral gene delivery systems can be used for direct in vivo administration or for cell-based approaches in which cells are genetically modified ex vivo and then infused into the patient. In cell-based approaches, different cell types can be used such as synovial fibroblasts, primary chondrocytes, mesenchymal stem cells (MSCs) or pluripotent stem cells. The investigated transgenes include both secreted proteins such as growth factors and anti-inflammatory proteins, as well as transcription factors, components of signaling pathways and small regulatory nucleic acids (miRNAs).
Gene therapy for OA treatment is a booming research topic, as evidenced by an explosion in the volume and in the quality of genomic studies, a growing number of preclinical and clinical-stage gene therapy drug candidates, and the first gene therapies being tested in clinical trials (11, 12). While a significant number of reviews of the literature exist about gene therapy in OA, no scoping reviews have thus far been published on this subject. We, therefore, propose this scoping review to map the relevant literature related to both non-viral and viral gene therapy in the management of OA.
The objective of this scoping review was to provide a comprehensive view on the current knowledge about gene therapies developed in the context of preclinical and clinical studies targeting OA. Four sub-questions were developed and will reflect the objectives of the scoping review:
(1) What are the delivery methods for gene therapies in OA?
(2) Which models have been developed to assess gene therapies in OA?
(3) What are the target genes in gene therapy approaches for OA?
(4) What are the effects observed for gene therapies in OA?
2. Methods
2.1. Eligibility criteria
Population: All research studies that address OA disease were included.
Concept: All research studies that explore in vitro, in vivo, or ex vivo gene therapies as well as clinical trials on gene therapies that follow a viral or non-viral gene therapy approach were considered. Gene therapies using siRNA approaches, or targeting miRNA, circRNA, as well as germline gene therapies including CRISPR/Cas9, were not taken into consideration.
Context: All research studies that include models of experimental OA, in vitro and in vivo models of OA, as well as clinical trials and studies on humans were included.
Types of sources: This scoping review considered all peer-reviewed published research studies that address the use of gene therapy in OA. Only studies published in English were considered for inclusion in this review. There were no limitations to their date of publication, country of origin, or setting.
The protocol for this review has not been registered.
2.2. Information sources
The following electronic databases were searched: Medline ALL Ovid, Embase, and Scopus. The most recent search was executed on March 7, 2023.
2.3. Search
The research team (MU and CL) undertook a preliminary search to identify controlled terms and keywords in titles and abstracts from relevant literature. Then, an extensive literature search was conducted.
The search strategies (see below for an example of Ovid MEDLINE and Appendix) were performed with the help of an information specialist experienced in evidence synthesis and adapted for each database. The search strategies focused on two concepts—gene therapy and osteoarthritis—and used a set of keywords and controlled terms.
Database: Ovid MEDLINE (R) ALL <1946 to March 02, 2023>
Search Strategy:
1 Genetic Therapy/ (52544)
2 Targeted gene repair/ (201)
3 ((gene or genes or genetic) adj3 (therap* or repair* or correction*)).ti,ab,kf. (82728)
4 DNA therap*.ti,ab,kf. (100)
5 1 or 2 or 3 or 4 (104404)
6 exp Osteoarthritis/ (75797)
7 osteoarthr*.ti,ab,kf. (92344)
8 osteo-arthr*.ti,ab,kf. (635)
9 arthros*.ti,ab,kf. (46096)
10 (degenerative adj3 arthriti*).ti,ab,kf. (1748)
11 (degenerative adj3 joint* adj3 disease*).ti,ab,kf. (3757)
12 coxarthros*.ti,ab,kf. (1705)
13 gonarthros*.ti,ab,kf. (1221)
14 6 or 7 or 8 or 9 or 10 or 11 or 12 or 13 (155956).
15 5 and 14 (450).
2.4. Selection of sources of evidence
All identified relevant records were uploaded into Covidence (https://www.covidence.org) and duplicates were removed. Following a pilot test, titles and abstracts were screened by two independent reviewers (MU & CL) for assessment according to the inclusion criteria for the scoping review. Then, the full text of selected papers was assessed according to the inclusion criteria by the same two independent reviewers. For both stages, discrepancies between the reviewers were resolved by discussion or by consulting a third reviewer (YH).
2.5. Data charting process
A data-charting form was jointly developed by two reviewers (MU & CL) to determine which variables to extract (experimental models, delivery methods, targets, and effects). This data charting form was used to extract data from selected studies. The same two reviewers independently charted the data, discussed the results, and updated, if necessary, the data-charting form to enable the capture of all relevant data to answer the review question.
2.6. Data items
Data on the population, concept, context, and key findings relevant to the review question was extracted. The data-charting form included the following items:
• Author and year of publication
• Objectives
• Participants (for clinical trials, only)
• Study design
• Disease models
• Delivery methods
• Targets
• Effects.
2.7. Synthesis of results
Data were analyzed and summarized quantitatively through numerical counts as well as descriptively. Data were presented graphically or in tabular form. A narrative summary accompanied the charted and/or tabulated results and describes how the results relate to the objectives and questions of the review.
3. Results
3.1. Selection of sources of evidence
The PRISMA flowchart outlining study selection is shown in Figure 1. An initial search identified 2,182 studies, 1,335 of which remained after the removal of duplicates. All but 274 of these studies were excluded at the stage of abstract review. Furthermore, 208 additional studies were excluded after reviewing the full manuscript. The reasons for exclusion at each stage are detailed in Figure 1. Overall, 66 studies responded to the inclusion criteria and constituted the study data.
3.2. Characteristics of sources of evidence
Most articles were on preclinical in vitro studies (32 articles) or in vivo animal models (39 articles), while four articles were on clinical trials.
3.3. Results of individual sources of evidence
We found a total of 29 different targets for gene therapy in the context of OA. These included studies examining interleukins (IL-1Ra alone or in combination with another target, TNF-RI, IL-1RII, IL-10, IL-4, TSG6, CrmA), growth factors, and receptors (IGF-1, relaxin, TGF-β1, BMP2 and 4, follistatin, GDF-5, FGF-2/bFGF), transcription factors (SOX9 alone or in combination with another target, KLF2 and 4, and ATF-4) and other key targets such as PRG4 (alone or in combination with another target), LOXL2, GlcAT-1, GGCX, kallistatin, RHEB, HSP70, PUM1, sCCR2 E3 and LRP3 (Figure 2).
4. Discussion
4.1. Summary of evidence
4.1.1. Interleukins
The activation of the immune system is closely linked to the initiation and perpetuation of low-grade systemic inflammation in OA (3). Interleukin-1 (IL-1) is considered among the most powerful molecules of the innate immune system and is linked to the pathogenesis of OA. It therefore appears to be a natural target for gene therapy. The IL-1 family is constituted of seven agonists including IL-1α and β, and four antagonists including the IL-1 receptor antagonist (IL-1Ra). The effect of IL-1 on joint tissues can be controlled by inhibiting its receptors using gene therapy-mediated overexpression of IL-1Ra (Table 1).
4.1.1.1. IL-1Ra
The therapeutic effect of IL-1Ra on the progression of OA lesions was evaluated by Pelletier et al. in a surgical dog OA model (ACLT model) (16). Interestingly, they observed a reduction in macroscopic lesion severity in animals that were injected with synovial cells retrovirally transduced with the IL-1Ra gene compared with the lac Z (control) group. Similarly, Baragi et al. observed a positive effect on human OA articular chondrocytes and OA cartilage explants transduced with Ad.RSV hIL-1ra cDNA (17). Nixon et al. investigated the disease-modifying properties of IL-1Ra gene therapy with an adenoviral-mediated overexpression of IL-1Ra under the control of the inflammation-inducible NF-kB promoter (HDAd-IL-1Ra) (18). In a anterior cruciate ligament transection (ACLT) mouse model of OA, they highlighted an improvement in histological scores, a decrease in osteophytes, and an increase in the volume and surface area of the cartilage. HDAd-IL-1Ra treatment also protected osteoarthritic mice against increased thermal hyperalgesia. Moreover, they confirmed these results in an osteochondral fragment horse OA model, observing an improvement in pain, claudication, and amplitude of movement, together with improved macroscopic and histological cartilage and synovium status. Deng et al. evaluated the potential of loaded nanomicelles to treat articular inflammation in a rat temporomandibular joint OA model (MIA model) (19). In this one, IL-1Ra mRNA reduced pain behavior and attenuated cartilage degradation as evidenced by the decrease of Mankin score. In addition, authors observed a downregulation of pro-inflammatory cytokines (IL-6 and TNF-α) blocking the MIA-induced inflammatory cascade. Finally, Senter et al. evaluated efficacy, biodistribution and safety of FX201 (HDAd expressing human IL-1Ra) and its ratIL-1Ra expressing ortholog vector in the rat ACLT OA model (20). IL-1Ra gene therapy significantly reduced joint damage in arthritic animals, and the gene therapy vectors were shown to be well-tolerated and to remain predominantly at the injection site after IA injection. Following these investigational new drug (IND)-enabling studies, a Phase 1 clinical trial with FX201 was conducted in 72 knee OA patients (NCT04119687).
Fernandes et al. developed a direct method using a non-viral vector, named “lipoplex” (21). It is a plasmid complexed to a lipid injected IA into the knees of rabbits. In a rabbit menisectomy model, they observed a dose-dependent reduction of lesion size associated with a reduction of the width of osteophytes. Another group, Zhang et al., evaluated a different non-viral strategy, in a rabbit surgery OA model (medial collateral ligament excision and medial menisectomy) (22). They demonstrated that the transfection efficiency of chitosan–DNA nanoparticles containing IL-1Ra or IL-10, was closely related to the gene product, and observed less severe cartilaginous lesions after treatment with IL-1Ra. Deng et al., employed a non-viral strategy using nanoparticles consisting of chitosan (CS)/hyaluronic acid (HA)/plasmidDNA (pDNA) encoding IL-1Ra to transfect primary synoviocytes (23). This strategy reduced MMP-3,−13, COX-2, and iNOS expression in IL-1β-stimulated synoviocytes. These results constitute a promising therapeutic approach for synovitis. Frisbie et al. investigated the effect of an Ad vector expressing IL-1Ra (Ad-EqIL-1Ra) in an equine osteochondral fragment OA model (24). They demonstrated that in addition to the conservation of the articular cartilage and the synovial membrane, the IA injection of the Ad-EqIL-1Ra vector significantly improved pain- and inflammation-related parameters. Goodrich et al. worked with an equine model, with the aim of developing the self-complementary AAV (scAAV) to produce higher levels of protein more quickly than with single stranded AAV (25). They proposed a dosing/alternative serotype redosing protocol, and examined the neutralizing antibody (Nab) response to the capsid. They did not observe any IA toxicity but the development of Nab against AAV capsid, which did however not decrease protein expression. Watson Levings et al. investigated the therapeutic capacity of an scAAV (sc-AAV.eqIL-1Ra) in two equine OA models (26, 27). On the first spontaneous model, they confirmed the safety. On the second, a surgically induced osteochondral fragmentation model of early OA, the results showed both a reduction in forelimb lameness and a reduction in inflammation. The authors also observed an improvement in the repair of osteochondral lesions, a reduction in joint effusion, and synovial proliferation. These data confirmed the results of previous studies.
Besides the “traditional” methods, Glass et al. investigated the possibility to combine gene therapy and tissue engineering by inducing IL-1Ra overexpression in human MSCs via scaffold-mediated lentiviral gene delivery (28). The results were quite promising since they demonstrated that the constructs could produce IL-1Ra in an inducible manner and that they protected against the effects of IL-1α. Gabner et al. also investigated the combination of tissue engineering methods employing bone marrow-derived equine MSCs transduced with a lentiviral vector expressing IL-1Ra gene under the control of the inducible NF-kB promoter (pSEWNFKBIL-1Ra) (29). They observed an increase of aggrecan, collagen IIA1 expression and a decrease of IL-6,−8, MMP-1, and−13 expression in equine chondrocytes stimulated with IL-1β or TNF-α.
Based on the assumption that the therapeutic effects of a single gene administration are limited, several teams have also evaluated the combinatorial effect of different targets. For example, Chen et al. investigated the combination of basic fibroblast growth factor (bFGF)/IL-1Ra and/or insulin-like growth factor 1 (IGF-1) (ADbFGF/ADIL-1Ra and/or ADIGF-1) (30). Transfection of human OA chondrocytes with two or three genes in different combinations resulted in increased proliferation of chondrocytes along with an increased synthesis of glycosaminoglycans, type II collagen, and TIMP-1, and a decreased expression of MMP-3,−13 and ADAMTS-5. In a rabbit ACLT model, the same team observed similar effects for type II collagen as well as a lower cartilage Mankin score. For his part, Zhang et al. evaluated the effect of IL-1Ra/IL-10 using retroviruses PLXRN-IL-1Ra and PLXRN-IL-10 in a rabbit OA model (medial collateral ligament excision and medial menisectomy) (31). While they observed no effect on synovitis, results on cartilage damage and glycosaminoglycan (GAG) content were promising. Haupt et al. tested the combinatorial effect of adenovirus-mediated overexpression of IL-1Ra with IGF-1 (equine AdIL-1Ra and equine AdIGF-1) to control cartilage degradation (32). In vitro, they demonstrated that the gene transfer promoted GAG and type II collagen synthesis and reduced IL-1β, IL-1α, and matrix metalloproteinases expression. Zang et al. evaluated the combined injection of IL-1Ra and TGFβ1 with liposomes in vivo (33). In a rabbit OA model (medial collateral excision and medial menisectomy), they observed a significant inhibition of cartilage matrix degradation as well as a prevention of osteophyte formation. The Mankin score was decreased in the transfected groups, and the expression of IL-1Ra and TGF-β1 was correlated to an increase of type II collagen expression and an extracellular matrix deposition. Finally, Wang et al. considered IL-1Ra with the soluble tumor necrosis factor-α receptor type I (sTNF-RI) (34). In a rabbit surgery OA model (Medial collateral ligament excision and medial menisectomy), IA injection of the combination Ad-IL-1Ra and Ad-sTNF-RI resulted in a decrease in cartilage lesions and synovitis whereas injection of sTNF-RI alone had no significant effect on these parameters. Multiple target combinations therefore seem to have beneficial effects in the context of gene therapy in OA.
Taken together, IL-1Ra gene therapy has been reported to improve clinical parameters such as pain and disease activity. In addition, beneficial effects on histological parameters of the synovial membrane and articular cartilage have been observed by different study groups.
4.1.1.2. IL-1RII
Type II IL-1β receptor (IL-1RII) serves as a “decoy” target for IL-1β. Previous data showed that it significantly inhibited IL-1-induced production of NO and/or PGE2 in synovial cells, chondrocytes, and epithelial cells. Under OA conditions, these same cells lack detectable amounts of IL-1Ra and IL-1RII, two molecules that normally antagonize IL-1 (44). In this context, and in order to reconstitute the functional expression of IL-1RII, Attur et al. transduced human articular OA chondrocytes and synoviocytes (IL-1RII− cells) with an Ad vector expressing IL-1RII, AdRSVRII (35). They observed a dose-dependent decrease of NO, PGE2, IL-6, IL-8, IL-1, and proteoglycan production in response to IL-1β. Another consequence was the release of sIL-1RII from transduced cells, thus protecting the other cells in co-culture and in transplants from the effect of IL-1β via sIL1-RII.
4.1.1.3. IL-10
Another interesting target for gene therapy is the cytokine IL-10. Produced by cells of innate and adaptive immunity, it is known for its anti-inflammatory and immunosuppressive properties. Thus, several gene therapies targeting this pleiotropic cytokine have been reported. In a rabbit OA model (medial collateral ligament excision and medial menisectomy), Zhang et al. were the first to evaluate the effect of retroviral IL-1Ra and IL-10 gene delivery on rabbit knee joints during the early inflammatory phase of OA (31). Thus, IA retroviral administration of IL-1Ra and IL-10 (PLXRN-IL-1Ra and PLXRN-IL-10) was able to impede an acute inflammatory reaction. IL-1Ra was more effective than IL-10 and most importantly, the co-injection of IL-1Ra and IL-10 was found to have significantly greater chondroprotective effects. Cartilage degradation and loss of proteoglycans was significantly more reduced by the combination than either one alone. In a 3D micromass synovial membrane model that mimics early-stage OA, Broeren et al. showed that adequate amounts of IL-10 transgene (lentivirus named CXCL10p-IL-10) reduced the synovial production of IL-1β and IL-6 and consequently, the inflammatory response (36). Farrell et al. assessed whether hMSCs overexpressing vIL-10 (via AdIL-10) were able to modify inflammation and adjust OA progression in a collagenase induced osteoarthritis model (CIOA) mouse model (37). Interestingly, the amount of activated CD4 and CD8 T-cells was significantly reduced in the vIL-10-expressing hMSCs group. A subsequent study conducted by Cameron et al. supports their findings (38). Indeed, in a stimulated, co-culture OA model, T-cell proliferation was significantly reduced by BM-MSCs overexpressing IL-10 (AAV-IL-10). This was accompanied by a reduced expression of inflammatory markers (IL-1β, IL-6, and TNF-α). Finally, considering the short half-life of IL-10 as well as its poor joint permeability, Watkins et al. used a plasmid DNA-based therapy for the production of a long-acting human IL-10 variant called hIL-10var (39). The first results of the 6-month GLP toxicology study looked promising. Bilateral IA injections of up to 1.5 mg of hIL-10var pDNA into canine stifle joints were well-tolerated and without pathologic findings. In addition, they have also conducted a small double-blinded, placebo-controlled study to assess the effect of IA hIL-10var pDNA on pain in companion dogs with naturally occurring OA, and observed a decrease of pain parameters without any adverse findings.
In summary, IL-10 gene therapy mainly reduces the expression of inflammatory markers. Protection from ECM degradation has only been reported in combination with other target genes.
4.1.1.4. IL-4
Among the anti-inflammatory cytokines, IL-4 is considered to have a strong therapeutic potential due to its inhibitory effect on IL-1β, the main mediator of inflammation leading to cartilage degradation. In this context, Lang et al. developed a non-viral transfection model to assess Cox-2 regulated IL-4 expression (pN3.Cox2.IL-4) (40). In an equine chondrocyte model, transfection with pN3.Cox-2.IL-4 of IL-1β or LPS-stimulated cells resulted in increased IL-4 expression and decreased expression of the inflammatory cytokines IL-1β,−6,−8 and the matrix degrading-enzymes MMP-1 and−3. Recently, Song et al. investigated the therapeutic potential of IL-4 overexpressing mesenchymal stem cells in spheroids (IL-4 MSC spheroid) (41). MSCs in spheroids are less prone to cell death after IA injection than naïve MSCs. In IL-1βstimulated primary rat chondrocytes in vitro, IL-4 MSC spheroids led to a reduction of IL-1β-induced apoptosis and a decrease in the production of OA factors (i.e., NO, iNOS, MMP-13), as well as an increase in the synthesis of cartilage extracellular matrix (ECM) (i.e., Col2). In vivo, in a rat anterior cruciate ligament transection (ACLT) with partial medial meniscectomy (MMx) (ACLT-MMx) model, an increased attenuation of tissue regeneration was observed after IA implantation of IL-4 MSC spheroids, along with improved chondroprotective and anti-inflammatory effects and better pain relief. Interestingly, these effects were higher in IL-4 MSC spheroids than in either IL-4 naïve MSCs or MSC spheroids without IL-4 suggesting that IL-4 MSC spheroids may increase the therapeutic efficacy of MSCs.
4.1.1.5. TSG6
Tumor necrosis factor-inducible gene 6 (TSG6) is an HA-binding protein associated with inflammatory processes. However, several studies report its protective effects in experimental arthritis. Inflammation and cartilage damage being two components of OA, Broeren et al. first demonstrated the functionality of TSG6 gene therapy in vitro by evaluating its effects on osteoclasts (42). They observed that overexpression of TSG6 using pShuttle-CMV-TSG-6, inhibited the resorption activity of osteoclasts. They then evaluated the effect of TSG6 therapy in vivo, in a CIOA mouse model. While no difference in protease activity or cartilage damage was observed, there was an increase in ectopic bone formation. Taken together, these data suggest that IA gene therapy with TSG6 does not seem to be a promising treatment for OA.
4.1.1.6. CrmA
The cytokine response modifier A (CrmA) is an inhibitor of caspases and IL-1β converting enzyme proteases and plays a role in attenuating IL-1β-induced inflammation and apoptosis in OA chondrocytes. Previously, due to their good biocompatibility, biodegradability and high stability, HA/CS microspheres have been shown to be a secure vehicle for the release of drugs. In this context, Qiu et al. investigated the use of these microspheres as vectors to deliver CrmA pDNA into OA synoviocytes (43). They demonstrated a decrease in IL-1β-mediated inflammation via a significant reduction of MMPs (MMP-3 and−13) in the HA/CS/pCrmA group compared to the control group.
4.1.2. Growth factors and receptors
In OA pathophysiology, the balance between catabolic and anabolic processes is crucial for the phenotype of chondrocytes. Thus, a promising approach in the context of gene therapy would be to either inhibit degradation or stimulate the synthesis of the ECM. Previously, it has been shown that insulin-like growth factor 1 IGF-1 stimulates matrix synthesis by stimulating type II collagen and aggrecan synthesis and promotes chondrocyte proliferation (Table 2).
4.1.2.1. IGF-1
Manning et al. evaluated the co-expression of IGF-1 and IL-4 using a dual promoter plasmid pVitro2, in an in vitro inflammatory model (45). In canine chondrocytes stimulated with IL-1β or TNF-α, the authors observed a decrease of pro-inflammatory mediators (i.e., IL-1β, TNFα, and IL-6) in the presence of IGF-1/IL-4 (pVitro2-IGF-1/IL-4), contrary to cells transfected with IGF-1 alone (pVitro2-IGF-1). They also observed a decrease in IGF-binding proteins and an increase in the key cartilage matrix proteins type II collagen and proteoglycans. These data suggested that the combination of genes could provide a better perspective in the context of gene therapy. Weimer et al. studied the overexpression of IGF-1 by rAAV transfection (rAAV-hIGF-1) and its effect on OA cartilage restoration in primary human OA chondrocytes in vitro and in explant cultures in situ (46). Prolonged IGF-1 secretion increased proliferation levels of chondrocytes and decreased apoptosis. Proteoglycan and type II collagen levels were also increased. Aguilar et al. compared the efficacy of AAV-mediated upregulation of IGF-1 (pAAV/IGF-1) (47). In articular bovine chondrocyte cultures and after transfection with pAAV/IGF-1, they observed a dose-dependent increase of IGF-1 and GAG production (58). However, IGF-1 added to media was less effective than endogenously produced IGF-1. In 2017, Aguilar et al. continued their studies on IGF-1 and developed a new material based on peptides (IGFBP-5) with a high affinity for IGF-1 and grafted a binding peptide sequence from IGFBP-5 onto alginate (48). Results demonstrated an increased binding affinity and availability of IGF-I as well as an increased synthesis of GAGs and hydroxyproline. According to the authors, “these data demonstrate the coordinated engineering of cell behavior and material chemistry to greatly enhance extracellular matrix synthesis and tissue assembly and can serve as a template for the enhanced performance of other therapeutic proteins” (48). Taken together, all these studies suggest that IGF-1 is a promising candidate for OA gene therapy.
Besides IGF-1, another target was studied, namely relaxin (RLN) (Table 2). This hormone belonging to the insulin superfamily, down-regulates TGF-β1-mediated collagen production and is important for matrix turnover by regulating MMP expression in the cartilage of synovial joints. In this context, Ko et al., investigated in vitro the effects of Ad-mediated RLN (Ad-RLN) expression on fibrosis inhibition in human OA synovial fibroblasts (49). Compared to control cultures, authors observed in Ad-RLN transfected synoviocytes, an increase of MMP-1 and conversely, a decrease of collagen IV, TIMP-1, and−2 protein expression suggesting that RLN exerts anti-fibrogenic effects on OA synovial fibroblasts via inhibition of collagen synthesis and collagenolytic pathways.
Taken together, IGF-1 gene therapy increased the production of major matrix components, such as proteoglycans and type II collagen, while also reducing pro-inflammatory mediators.
4.1.2.2. TGF-β1
The transforming growth factor-β (TGF-β) family has more than 35 members, including TGF-β, activins, and bone morphogenetic proteins (BMPs). Three isoforms of TGF-β are known (TGF-β1, -β2, and -β3), TGF-β1 being amongst the most prevalent growth factors involved in cartilage repair. Indeed, it directly stimulates the synthesis of proteoglycans and collagen, and antagonizes the effects of IL-1 on metalloproteinases in both normal and OA chondrocytes. In this context, Ulrich-Vinther et al. were among the first to evaluate the effect of overexpression of TGF-β1 on cartilage anabolism in human OA chondrocytes (Table 2) (50). They demonstrated that after AAV-TGF-β1 transduction, chondrocytes expressed higher levels of type II collagen and aggrecan, while the expression of MMP-3 was decreased. Venkatesan et al. also investigated the overexpression of TGF-β1 (rAAV-hTGF-beta) in human primary OA chondrocytes and OA cartilage explants (51). They observed an increase in proliferation and cell survival compared to the control vector. They highlighted a decrease of key markers of chondrocyte hypertrophy such as type-X collagen, MMP-13, the parathyroid hormone-related protein (PTHrP) and β-catenin and, on the contrary, an increased expression of protective TIMP-1 and−3. The development of TissueGene-C (TG-C), a mix of human allogeneic chondrocytes and irradiated cells overexpressing TGF-β1, represented a key step for the advancement of OA gene therapy. Thus, Noh et al. evaluated the potential of TG-C in vivo, in a rabbit surgically induced cartilage defect OA model. In the presence of TG-C, they observed a dose-dependent effect on cartilage regeneration (52). It was also studied in goats in a surgically induced single full-thickness cartilage defect model. They observed an increase in the proliferation of new chondrocytes and a positive effect on articular cartilage at 6 months. After a demonstration of safety and efficacy, the authors proceeded with a phase I clinical study of TG-C (Table 3). Ha et al. evaluated the dose-response of this cell therapy in OA patients (59). This was a single center, open-label, dose escalation study on 12 adults, to assess the dose-response of three different doses of TG-C. No serious treatment-related adverse events were observed. Swelling, effusion, and minor local reactions were dose dependent. Knee evaluation scores (KSCRS, WOMAC, and VAS) seemed to point toward a dose-dependent trend of efficacy. Cherian et al. led the development of a clinical phase II (60). The objective was to evaluate the efficacy of TG-C in patients with grade III OA (n = 102). The characteristics of the study were as follows: multi-center, double-blinded, placebo-controlled, and randomized. The authors observed improved knee function and pain as assessed by the International Knee Documentation Committee (IKDC) and the Visual Analog Scale (VAS) and in parallel, reduced analgesics use by patients. A phase IIa study evaluated the efficacy and safety of TG-C in patients who had late-stage knee OA (grade IV) (n = 27) (61). This was a multi-center and single-blinded study. There was an improvement in both symptoms and activity level and function of the knee; stiffness, motor function and pain improved significantly, as evidenced by improved IKDC, WOMAC, and VAS scores. Of note, approval of TG-C as gene therapy for OA under the name of Invossa was revoked in Korea when it became apparent that the transgenic cells were mainly HEK293 cells instead of chondrocytes, and its status is currently being investigated. In the US, on the other hand, trials are ongoing as HEK293 cells had been used from the beginning [reviewed in (64)].
Thus, Kim et al. conducted a phase III (NCT03291470), multi-center and double-blinded clinical trial including a total of 163 Kellgren–Lawrence grade III patients (62). Results highlighted a decrease in serum CTX-I and urine CTX-II levels, as well as significant improvements in function and pain in OA patients. Recently, Lee et al. investigated the effects of TG-C on pain and cartilage structure via the polarization of M2 macrophages (53). In an OA rat monosodium iodoacetate (MIA) model, pain relief and improvement of cartilage structure were demonstrated; an increase in IL-10 and TGF-β1 in synovial fluid was observed followed by induction of arginase 1 expression (M2 macrophage marker) and decrease of CD86 (M1 macrophage marker). The effect was therefore favorable for the induction of M2 macrophages implicated in tissue repair.
In summary, TGF-β1 gene therapy mainly restored synthesis of proteoglycan and type II collagen, while also reducing markers of hypertrophic differentiation.
4.1.2.3. BMPs
Bone morphogenic proteins (BMPs) are important players in the formation of functional joints and in the maintenance of cartilage homeostasis. Both BMP2 and BMP4 are involved in chondrogenesis, cartilage growth, and chondrocyte proliferation (23) and are therefore very interesting targets for OA gene therapy (Table 2). Muscle-derived stem cells (MDSCs) are a promising cell type for tissue engineering to allowre musculoskeletal regeneration. Gao et al. showed that hMDSCs lentivirally transduced to overexpress BMP2 have an increased chondrogenic differentiation capacity (54). In addition, these lentivirally-transduced hMDSCs improved cartilage repair and erosion in a rat MIA model in vivo. Interestingly, BMP2 delivery by coacervation resulted in similar articular cartilage repair as lenti-BMP2/GFP-mediated delivery. Matsumoto et al. showed that hMDSCs retrovirally transduced to overexpress BMP4 and transplanted into a rat MIA model of OA, are able to repair articular cartilage; osteophyte formation was however observed in some areas of the joint (55). A combination of soluble Fms-like Tyrosine Kinase 1 (sFLT-1) and BMP4-transduced hMDSCs exacerbated this effect without osteocyte formation. Moreover, increased differentiation and proliferation and decreased apoptosis was observed in chondrocytes in the animals transplanted with sFLT-1 and BMP4-transduced hMDSCs.
4.1.2.4. FST
Recently, Tang et al. were interested in the effect of follistatin (FST) delivery on metabolic inflammation (Table 2) (56). In the OA pathology, the authors assume that “FST delivery using a gene therapy approach has multifactorial therapeutic potential through its influence on muscle growth via inhibition of myostatin activity as well as other members of the TGFβ family” (56). In a mouse destabilization of the medial meniscus (DMM) OA model, FST gene therapy (AAV9-FST) reduced cartilage degeneration and decreased joint synovitis. The overexpression decreased the inflammatory cytokine, IL-1β and restored muscle performance by enhancing muscle growth and muscle performance. Finally, this therapy protected injury-mediated trabecular and cortical bone structure changes. This systemically delivered therapy is very promising especially for OA, but also for associated metabolic conditions, such as diseases of muscle wasting.
4.1.2.5. GDF-5
Like Deng et al. for IL-Ra, Chen et al. investigated a non-viral gene therapy vector to evaluate the therapeutic potential of the growth and differentiation factor-5 (GDF-5) (Table 2) (57). This factor belongs to the TGF-β and BMP superfamilies that regulate cell growth and differentiation. They generated nano-microspheres (NMPs) consisting of chitosan, HA, and chondroitin sulfate, and containing a GDF-5 plasmid. In rabbit chondrocytes, these GDF-5 containing NMPs increased the expression of collagen II and aggrecan, two key ECM proteins. In a rabbit ACLT and meniscectomy OA model, the authors demonstrated that these NMPs improved cartilage morphology and joint structure compared to the saline control group.
4.1.3. Transcription factors
Targeting transcription factors for OA gene therapy, such as sex-determining region Y-type high mobility group box 9 (SOX9), krüppel-like factor 2 or 4 (KLF2/KLF4), or activating transcription factor 4 (ATF-4), has the benefit of correcting gene expression profiles altered in OA toward the expression of genes implicated in the production of ECM compounds.
4.1.3.1. SOX9
SOX 9 is of particular interest because of its role in cartilage formation and chondrocyte differentiation. Furthermore, its expression is notably downregulated in OA (Table 4). The combinatorial effect of SOX9 and FGF2 overexpression after rAAV-mediated gene delivery to human OA chondrocytes or OA cartilage explants was evaluated by Cucchiarini et al. (66). They observed a higher production of proteoglycans and type II collagen, which was attributed to SOX9 rather than FGF2. The same effects were observed by Daniels et al. when transforming human articular OA chondrocytes in a 3D aggregate culture model after rAAV-mediated SOX9 overexpression (67). The combinatorial overexpression of SOX9 and TGF-β1 after rAAV-mediated gene delivery to human OA chondrocytes or OA cartilage explants as evaluated by Tao et al. showed the same effects, along with increased proliferation and cell density and enhanced levels of chondrogenic aggrecan (ACAN) and collagen type II alpha 1 chain (COL2A1) expression (68). The downside of rAAV-mediated gene delivery is however the widespread presence of neutralizing antibodies against the viral proteins in the host. Therefore, Urich et al. explored the potential of delivering rAAV-FLAG-hsox9 vectors via polymeric micelles into human primary OA chondrocytes. These micellar systems increased proliferation, type II collagen, and proteoglycan deposition in human primary OA chondrocytes stimulated with IL-1β and TNF-α (69).
4.1.3.2. KLF2 and 4
Interestingly, OA is associated with a decreased expression of KLF family members of transcription factors (72). Kawata et al. evaluated the therapeutic potential of KLF2 and 4 for OA. In human OA chondrocytes, meniscal cells or BMSCs, Ad-mediated overexpression of KLF2 or 4 induced an upregulation of cartilage genes, including COL2A1, COL11A2, COMP, PRG4, and SOX9 (70). Furthermore, AAV-mediated overexpression of KLF4 in a mouse DMM OA model not only upregulated above mentioned cartilage genes, but also resulted in reduced pain and an improved OARSI score, meniscus histopathological score, synovitis score, and bone score. The authors concluded “that KLF4 had therapeutic and protective effects against OA-associated tissue damage and pain” (70).
4.1.3.3. ATF4
Exosomes are small extracellular vesicles, which can contain proteins, RNA, and DNA, making them very interesting vehicles for gene delivery because of their high penetration and low immunogenicity. Wang et al. were the first to explore the therapeutic effects of serum-derived exosomes from meniscus-injury-induced OA mice overexpressing ATF4 (ATF4-OA-Exo) in a meniscus injury-induced mouse model of OA (Table 4) (71). These ATF-OA exosomes alleviated the cartilage damage and proteoglycan loss observed in these OA mice and decreased the number and size of osteophytes, as well as the cartilage Mankin score. In line with these observations, type II collagen was increased, while MMP-13 and inflammatory cytokines were decreased. In addition, ATF4-OA-exosome injection led to a partial recovery of the weakened autophagy in OA cartilage and inhibited apoptosis in tunicamycin (TM)- or TNF-α-treated chondrocytes, indicating that the effect ATF4-OA-Exo might be exhibited through the induction of autophagy.
4.1.4. Other key targets
4.1.4.1. PRG4
Proteoglycan 4 (PRG4), also known as lubricin, is a glycoprotein expressed by chondrocytes and synoviocytes and naturally secreted in synovial fluid. It is of particular interest for gene therapy, as it acts as a lubricant to reduce friction within articular cartilage, diminishes inflammation, and as an anabolic factor slows down OA progression (Table 5). In humans, Camptodactyly-Arthropathy-Coxa Vara-Pericarditis Syndrome, characterized by early onset OA, is caused by loss-of-function mutations in PRG4. Similarly, PRG4 knock-out mice also develop early OA. Ruan et al. reported that transgenic, joint-specific PRG4 overexpression protects mice against OA development and motor impairments in a mouse ACLT model and protects aging mice from natural OA development (73). Treatment with an HDV vector expressing PRG4 (HDV-PRG4) protected mice against OA development and improved structural joint status in a mouse ACLT model, as evidenced by improved histology scores, cartilage volume and bone area covered by cartilage. Later, Ruan et al. reported that in the mouse ACLT model, IA injection of HDV-PRG4 or a capsid-modified HDV (a10mabHDV-PRG4, which specifically targets chondrocytes), prior to surgical OA induction, protected animals from OA development (74). Cartilage volume and bone area covered by cartilage were preserved in both cases. The efficacy was, however, greater for the a10mabHDV-PRG4. When injecting viral vector 2 weeks after ACLT surgery, a 10-times lower effective dosage of a10mabHDV-PRG4 was needed to prevent post-ACTL OA compared with the untargeted HDV-PRG4. Using the low dose of a10mabHDV-PRG4, the preservation of cartilage volume was greater, and it covered a larger area of bone compared to the same dose of HDV-PRG4 vector. The effect of a10mabHDV-PRG4 was similar to the 10-fold higher dose of HDV-PRG4. Stone et al. explored the added benefits of a combinatorial gene therapy approach compared to targeting PRG4 alone (75). The IA co-injection of HDVs expressing IL-1Ra and PRG4 in a mouse ACLT model, resulted in better preservation of cartilage volume and bone area covered by cartilage than injection of either HDV alone. This was accompanied by the increase of anabolic and the decrease of catabolic and inflammatory gene expression. In the less severe DMM model, both PRG4 and IL-1Ra + PRG4 combinatorial gene therapy were able to maintain cartilage volume and covered surface area. While all treatment groups prevented OA-induced thermal hyperalgesia at the early timepoint, only the combined gene therapy showed significant protective effect at the late timepoint. The chondroprotective effect of PRG4 gene therapy was confirmed by Seol et al. in a rabbit ACLT model (76). IA injection of AAV-PRG4-GFP immediately after ACLT surgery inhibited cartilage damage and reduced the severity of post-traumatic OA (PTOA). In addition, more cartilage surface and superficial chondrocytes were covered by lubricin.
4.1.4.2. LOXL2
The copper-dependent amine oxidase lysis oxidase-like 2 (LOXL2) catalyzes the first step in the formation of crosslinks in collagens and elastin and has been shown to mediate endochondral ossification. Tashkandi et al. wanted to assess the potential of Ad-mediated overexpression of LOXL2 for OA treatment (Table 5) (77). In an IL-1β stimulated ATDC5 cartilage cell line, LOXL2 overexpression blunted the decrease of aggrecan and SOX9, and attenuated both the IL-1β mediated expression of ADAMTS4/5, and MMP-13, as well as the IL-1β induced activity of NF-κB. In chondrodysplasia (Cho/+) mice, LOXL2 overexpression protected these mice against progressive OA, as evidenced by increased proteoglycan deposition, increased aggrecan, type II collagen, and anabolic gene expression, and decreased MMP-13 and ADAMTS5 expression. Finally, in MIA-induced LOXL2 transgenic mice, LOXL2 overexpression protected against proteoglycan and aggrecan degradation and reduced MMP-13 levels. It protected these mice from MIA-induced OA-related decline in knee function. The authors, therefore, conclude that LOXL2 is a promising target for gene therapy for knee OA.
4.1.4.3. Proteoglycans
Proteoglycans (PG) are made of “core proteins” with covalently attached glycosaminoglycan (GAG) chains. Their depletion is a major hallmark in joint destruction, and factors that might accelerate PG synthesis and deposition are therefore of great interest for gene therapy. Venkatesan et al. investigated how GAG-synthesizing enzyme β1,3-glucuronosyltransferase-I (GlcAT-I) gene therapy might influence cartilage repair in IL-1β stimulated primary rat chondrocytes in vitro (Table 5) (78). Lipid-mediated overexpression of GlcAT-I inhibited IL-1β induced loss of PGs, and increased the GAG content and number of chains, rather than chain size. In addition, it restored PG synthesis in IL-1β treated cartilage explants.
4.1.4.4. γ-Glutamyl Carboxylase
γ-glutamyl carboxylase (GGCX) regulates the carboxylation of cartilage matrix Gla protein (MPG), a calcification inhibitor. Since the level of uncarboxylated, non-functional MPG (ucMPG) seems to be elevated, while GGCX seems to be reduced in OA patients, Fu et al. set out to investigate the effect of lentiviral-mediated GGCX overexpression in a rabbit ACLT model of OA (Table 5) (79). GGCX overexpression resulted in less ACLT-induced morphological changes and inflammation, which was accompanied by normalized levels of carboxylated MPG. MMP-13 and type X collagen were decreased, while type II collagen was increased to normal levels. GGCX, therefore, constitutes an interesting target for OA gene therapy.
4.1.4.5. Kallistatin
Kallistatin, a serine proteinase inhibitor and known inhibitor of angiogenesis, has been shown to protect cardiomyocytes from apoptosis and to prevent an inflammatory response after myocardial ischemia-reperfusion injury. Hsieh et al. investigated the effect of its Ad-mediated overexpression in a rat ACLT OA model after IA delivery (Table 5) (80). They observed a reduced inflammatory response as evidenced by reduced levels of IL-1β and TNF-α in the joints, as well as an overall reduced OA severity and a reduced number of apoptotic cells. In addition, there was less macrophage infiltration, hyperplasia, and synovitis in Adenoviral vector encoding human kallistatin (AdHKBP) animals, which was exacerbated when treated in combination with HA. Kallistatin gene therapy might therefore be an interesting alternative for OA treatment, especially when administered in combination with HA.
4.1.4.6. RHEB
Ras homolog enriched in the brain (RHEB) is part of the Ras family and is involved in cell growth, proliferation, and differentiation. Ashraf et al. were interested in its effect on OA chondrocytes in vitro and OA progression in a mouse DMM model (Table 5) (81). Human articular OA chondrocytes displayed normalized morphology, reduced senescence, and decreased oxidative stress when transfected with a vector containing RHEB cDNA. Ad-mediated overexpression of RHEB in a DMM mouse model resulted in attenuated cartilage destruction, suppressed expression of ADAMTS5, MMP-13, type 10 collagen, and COL2A1, and inhibited apoptosis. The authors, therefore, concluded that RHEB is important for maintaining the chondrogenic phenotype of chondrocytes and is likely involved in preventing the progression of OA in vivo.
4.1.4.7. HSP70
Heat shock protein 70 (HSP70) is expressed as a protective agent upon various types of stresses. Grossin et al. set out to investigate its cyto- and/or chondroprotective role in a rat MIA OA model (Table 5) (82). HSP70 overexpression was successfully achieved by electroporation and resulted in the inhibition of endochondral ossification in the deep layer and reduced severity of OA-induced lesions. The authors conclude that HSP70 might therefore be a novel chondroprotective target for gene therapy in OA.
4.1.4.8. PUM1
RNA-binding proteins (RBPs) have been shown to play a role in age-related degenerative diseases (86). Yoon et al. investigated the effect of lentiviral-mediated overexpression of the RBP Pumilio1 (PUM1) in a mouse DMM OA model (83). They observed that it prevented cartilage destruction and chondrocyte loss, and significantly improved the OARSI score. They therefore conclude that PUM1 might be an interesting target for OA therapy.
4.1.4.9. sCCR2 E3
The monocyte chemoattractant protein-1 (MCP-1/CCL2) - C-C chemokine receptor type 2 (CCR2) pathway is involved in OA progression, and ways to block this pathway are being explored (87). Na et al. investigated the effect of a fusion protein constituted of 20 amino acids of the third extracellular domain (E3) of the CCL2 receptor and a soluble CCL2 receptor (sCCR2) in a rat MIA OA model (84). Intra-articular injection of a sCCR2 E3 vector via electroporation resulted in reduced pain and less bone loss and cartilage degradation, as well as a lower OARSI and Mankin score. In addition, the expression of inflammatory cytokines IL-1β and IL-6 and catabolic factor MMP-13 was reduced. sCCR2-E3 therefore protects against cartilage damage and inhibits catabolic factors.
4.1.4.10. LRP3
Low-density lipoprotein (LDL) receptor-related proteins (LRPs) play an important part in the regulation of cholesterol metabolism and have recently been shown to be involved in the onset and progression of OA (88). Cao et al. investigated the potential role of LRP3 in OA pathogenesis and therapy (85). In TNF-α stimulated rat OA chondrocytes, lentiviral-mediated overexpression of Lrp3 induced the expression of the anabolic genes COL2A1, ACAN, and SOX9, and restored expression of proteoglycan and GAG. Furthermore, in a rat ACLT OA model, it inhibited cartilage degradation and restored proteoglycan and type II collagen levels. The expression of anabolic genes COL2A1, ACAN, and SOX9 was increased, while the expression of catabolic genes ADAMTS5 and MMP13 was decreased. The authors conclude that LRP3 overexpression may be a new therapeutic target for OA therapy by delaying the degeneration of cartilage.
5. Limitations
This scoping review took a rigorous approach based on the JBI framework to the conduct of scoping reviews and was reported following the PRISMA-ScR checklist. To our knowledge, it is the first scoping review of preclinical and clinical studies reporting the effect of gene therapies used to treat OA. It does however suffer from one minor limitation: unpublished work, and ongoing clinical trials were not included. We searched for ongoing clinical trials on gene therapy in OA on clinicaltrials.gov and discussed the outcome of our search in the conclusion and perspective section.
6. Conclusions
In the last decade, research on gene therapy has been in full expansion, as evidenced by an increasing number of scientific articles on the subject. In the absence of any DMOAD, gene therapy is of special interest in the context of OA. Both viral and non-viral gene therapy approaches are highly promising treatment alternatives of OA in the future, and several targets have been explored and studied. Both technologies have, however, their own advantages and inconveniences. While viral gene therapy is highly efficient and allows for targeted and sustained delivery of genes, it might activate the innate immune system and cause local inflammation in the host. This is why more recently, several groups have started to explore the option of non-viral gene therapy approaches, such as NMPs or exosomes. While non-viral therapies have the handicap of relatively low transfection efficiency and transient gene expression, they are considered a safer option particularly for OA because of their reduced risk to cause an inflammatory response. CRISPR/Cas9-mediated gene editing, while not the subject of this review, is another alternative for the development of new gene therapies for OA. The technology has revolutionized the field of gene editing and is widely considered an accurate and easy-to-use tool for genome editing. As an example, intra-articular injection of AAV expressing CRISPR/Cas9 components that target MMP-13, IL-1β, and/or nerve growth factor (NGF) suggest that combined deletion of these genes has beneficial effects on both pain management and joint integrity (89). The disadvantage of CRISPR/Cas9 is however the difficulty to deliver enough material to mature cells for efficient genome editing activity. Moreover, it requires the presence of a PAM sequence near the target site, thus limiting its effective targeting range. Most importantly, the relatively high frequency of off-target effects is a significant challenge for employing CRISPR/Cas9 for gene therapy, particularly in clinical applications.
Alternatively, several groups have explored the possibility of targeting multiple genes, so as to heighten and potentiate their effect. In OA with its complex physiopathology, targeting only one actor might not be sufficient to efficiently treat the disease long-term. This strategy seems very promising, and good pre-clinical results have been obtained for various combinations, for example, IL-1Ra and PRG4, FGF2 and SOX9, or IL1-Ra and IL-10.
The main targets identified for gene therapy of OA belong to the interleukin family (IL-1Ra, IL-10, IL-4, TSG6, and CrmA), growth factors and their receptors (IGF-1, relaxin, TGF-β1, BMP2 and 4, follistatin, GDF-5, and FGF2/bFGF), and transcription factors (SOX9, KLF2 and 4, and ATF-4). In addition, lubricin, LOXL2 and other enzymes and proteins are being explored for their therapeutic potential (Figure 3).
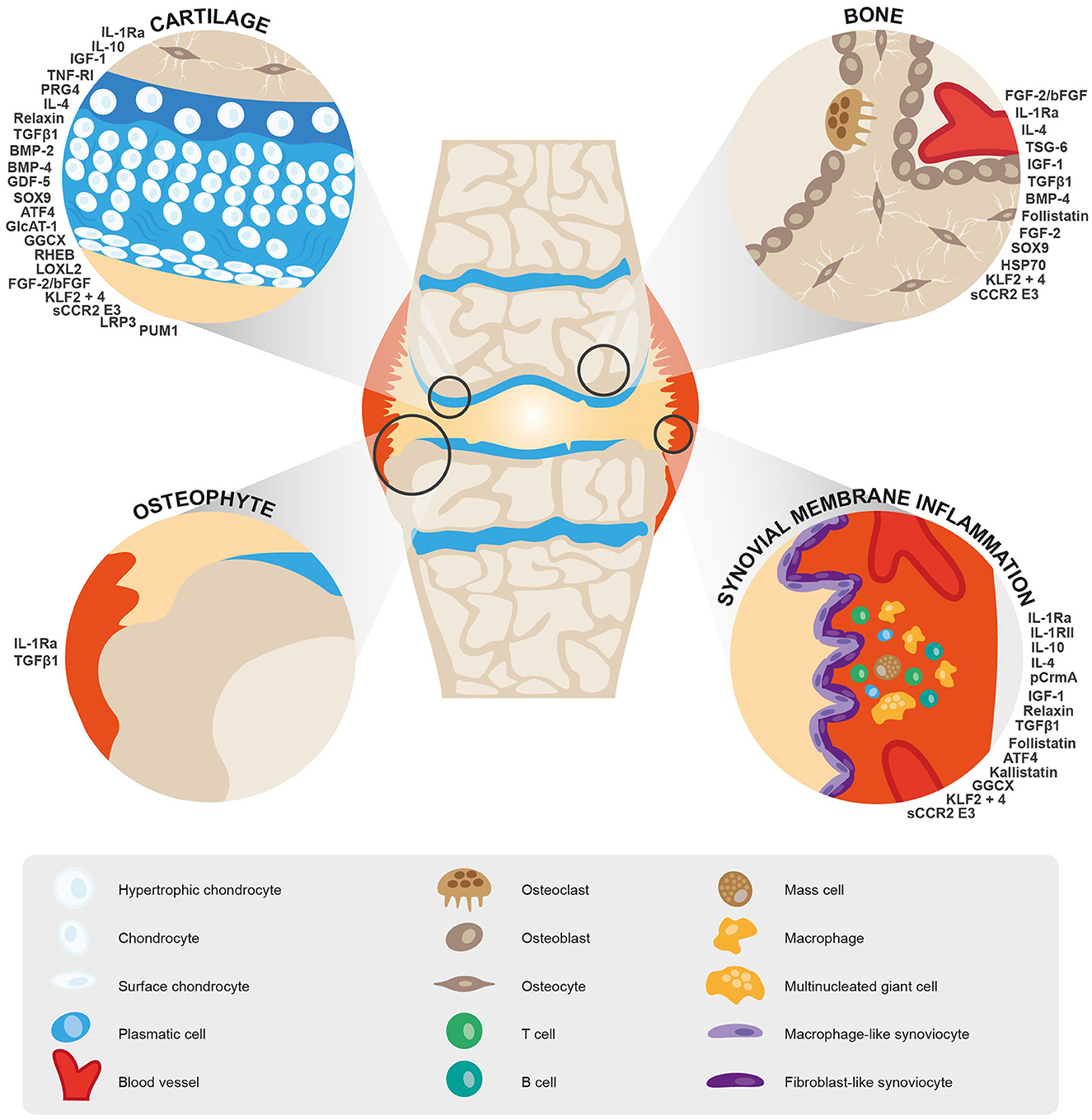
Figure 3. Schematic representation of targets identified for gene therapy of OA and their therapeutic potential.
Three Phase I studies (NCT03282149, NCT03477487, and NCT03769662) have been successfully completed for XT-150, the locally injectable non-viral therapy expressing interleukin (IL)-10v described by Watkins et al. and discussed above (39). A multinational, double-blind, placebo-controlled Phase II study (NCT04124042) is currently evaluating the safety and efficacy of XT-150 in adults with moderate to severe pain due to knee OA. A Phase I, open-label study (NCT02790723) evaluating the safety of intra-articular injection of scAAV2.5IL-1Ra in nine subjects with moderate knee OA has recently been completed with encouraging results. A Phase I open-label, single ascending dose study (NCT04119687) assessing the safety and tolerability of FX201, the HDAd-IL-1Ra intra-articular gene therapy described by Senter et al., in 72 subjects with moderate to severe knee OA has been recently completed, and the sponsor reported “very compelling” results (no further details were given) (20). A post marketing surveillance study (NCT03412864) including 3,000 OA patients (Kellgren–Lawrence grade III) assessing adverse events as primary endpoints is currently underway for TG-C.
In conclusion, gene therapy is a highly promising treatment for OA, even though it still is in its early stages and further development is required to bring more targets to the clinical stage, as has been done with TG-C targeting TGF-β1, XT-150 targeting IL-10, FX201 and scAAV2.5-IL-1Ra. Overall, the optimal system (s) for safe and effective disease-specific therapy still need to be identified, including the vector type or non-viral delivery system, the therapeutic single gene or gene combination, and the levels of therapeutic gene expression. As more and more clinical trials for gene therapy in OA are being undertaken, we need guidelines for their design and conduct.
Data availability statement
The original contributions presented in the study are included in the article/Supplementary material, further inquiries can be directed to the corresponding author.
Author contributions
MU, CL, and YH: drafting manuscript. MU, CL, JG, KG, SP, and YH: revising manuscript content and approving final version of manuscript. All authors contributed to the article and approved the submitted version.
Acknowledgments
The authors would like to thank Dr. Nancy Durieux from the University of Liège—Research Unit for a life-Course perspective on Health and Education, Faculty of Psychology, Speech and Language Therapy and Educational Sciences for her help in setting up research strategies and their implementation into Covidence.
Conflict of interest
YH is the founder and president of Artialis S.A. and received consulting fees from Laboratoires Expanscience, Tilman, Biose, Immubio, Naturex, and GeneQuine Biotherapeutics GmbH.
The remaining authors declare that the research was conducted in the absence of any commercial or financial relationships that could be construed as a potential conflict of interest.
Publisher's note
All claims expressed in this article are solely those of the authors and do not necessarily represent those of their affiliated organizations, or those of the publisher, the editors and the reviewers. Any product that may be evaluated in this article, or claim that may be made by its manufacturer, is not guaranteed or endorsed by the publisher.
Supplementary material
The Supplementary Material for this article can be found online at: https://www.frontiersin.org/articles/10.3389/fmed.2023.1148623/full#supplementary-material
References
1. Lambert C, Borderie D, Dubuc J-E, Rannou F, Henrotin Y. Type II collagen peptide Coll2-1 is an actor of synovitis. Osteoarthr Cartil. (2019) 27:1680–91. doi: 10.1016/j.joca.2019.07.009
2. Loeser RF, Goldring SR, Scanzello CR, Goldring MB. Osteoarthritis: a disease of the joint as an organ. Arthritis Rheum. (2012) 64:1697–707. doi: 10.1002/art.34453
3. Lambert C, Zappia J, Sanchez C, Florin A, Dubuc J-E, Henrotin Y. The damage-associated molecular patterns (DAMPs) as potential targets to treat osteoarthritis: perspectives from a review of the literature. Front Med. (2020) 7:607186. doi: 10.3389/fmed.2020.607186
4. Abramoff B, Caldera FE. Osteoarthritis: pathology, diagnosis, and treatment options. Med Clin N Am. (2020) 104:293–311. doi: 10.1016/j.mcna.2019.10.007
5. Lewis R, Gómez Álvarez CB, Rayman M, Lanham-New S, Woolf A, Mobasheri A. Strategies for optimising musculoskeletal health in the 21 st century. BMC Musculoskel Disord. (2019) 20:164. doi: 10.1186/s12891-019-2510-7
6. Bannuru RR, Osani MC, Vaysbrot EE, Arden NK, Bennell K, Bierma-Zeinstra SMA, et al. OARSI guidelines for the non-surgical management of knee, hip, and polyarticular osteoarthritis. Osteoarthr Cartil. (2019) 27:1578–89. doi: 10.1016/j.joca.2019.06.011
7. Kolasinski SL, Neogi T, Hochberg MC, Oatis C, Guyatt G, Block J, et al. 2019 American college of rheumatology/arthritis foundation guideline for the management of osteoarthritis of the hand, hip, and knee. Arthrit Rheumatol. (2020) 72:220–33. doi: 10.1002/art.41142
8. Austine J, Nair S, Mirza K. Perspective of orthopedists on pain management in osteoarthritis: a qualitative study. Indian J Palliat Care. (2016) 22:410–5. doi: 10.4103/0973-1075.191764
9. Skou ST, Roos EM. Physical therapy for patients with knee and hip osteoarthritis: supervised, active treatment is current best practice. Clin Exp Rheumatol. (2019) 37(Suppl. 120):112–7.
10. Nelson AE, Allen KD, Golightly YM, Goode AP, Jordan JM. A systematic review of recommendations and guidelines for the management of osteoarthritis: The Chronic Osteoarthritis Management Initiative of the US Bone and Joint Initiative. Semin Arthritis Rheum. (2014) 43:701–12. doi: 10.1016/j.semarthrit.2013.11.012
11. Salem HS, Ehiorobo JO, Parvizi J, Mont MA. The safety and efficacy of a novel cell-based gene therapy for knee osteoarthritis. Surg Technol Int. (2019) 35:370–6.
12. Grässel S, Muschter D. Recent advances in the treatment of osteoarthritis. F1000Research. (2020) 9:F1000. doi: 10.12688/f1000research.22115.1
13. Grol MW, Lee BH. Gene therapy for repair and regeneration of bone and cartilage. Curr Opin Pharmacol. (2018) 40:59–66. doi: 10.1016/j.coph.2018.03.005
14. Gantenbein B, Tang S, Guerrero J, Higuita-Castro N, Salazar-Puerta AI, Croft AS, et al. Non-viral gene delivery methods for bone and joints. Front Bioengin Biotechnol. (2020) 8:598466. doi: 10.3389/fbioe.2020.598466
15. Uzieliene I, Kalvaityte U, Bernotiene E, Mobasheri A. Non-viral gene therapy for osteoarthritis. Front Bioeng Biotechnol. (2021) 8:618399. doi: 10.3389/fbioe.2020.618399
16. Pelletier JP, Caron JP, Evans C, Robbins PD, Georgescu HI, Jovanovic D, et al. In vivo suppression of early experimental osteoarthritis by interleukin- 1 receptor antagonist using gene therapy. Arthritis Rheum. (1997) 40:1012–9. doi: 10.1002/art.1780400604
17. Baragi VM, Renkiewicz RR, Jordan H, Bonadio J, Hartman JW, Roessler BJ. Transplantation of transduced chondrocytes protects articular cartilage from interleukin 1-induced extracellular matrix degradation. J Clin Invest. (1995) 96:2454–60. doi: 10.1172/JCI118303
18. Nixon AJ, Grol MW, Lang HM, Ruan MZC, Stone A, Begum L, et al. Disease-modifying osteoarthritis treatment with interleukin-1 receptor antagonist gene therapy in small and large animal models. Arthritis Rheumatol. (2018) 70:1757–68. doi: 10.1002/art.40668
19. Deng J, Fukushima Y, Nozaki K, Nakanishi H, Yada E, Terai Y, et al. Anti-inflammatory therapy for temporomandibular joint osteoarthritis using mRNA medicine encoding interleukin-1 receptor antagonist. Pharmaceutics. (2022) 14:1785. doi: 10.3390/pharmaceutics14091785
20. Senter R, Boyce R, Repic M, Martin EW, Chabicovsky M, Langevin-Carpentier G, et al. Efficacy and safety of FX201, a novel intra-articular IL-1Ra gene therapy for osteoarthritis treatment, in a rat model. Hum Gene Ther. (2022) 33:541–9. doi: 10.1089/hum.2021.131
21. Fernandes J, Tardif G, Martel-Pelletier J, Lascau-Coman V, Dupuis M, Moldovan F, et al. In vivo transfer of interleukin-1 receptor antagonist gene in osteoarthritic rabbit knee joints. Prevention of osteoarthritis progression. Am J Pathol. (1999) 154:1159–69. doi: 10.1016/S0002-9440(10)65368-0
22. Zhang X, Yu C, Xu S, Zhang C, Tang T, Dai K. Direct chitosan-mediated gene delivery to the rabbit knee joints in vitro and in vivo. Biochem Biophys Res Commun. (2006) 341:202–8. doi: 10.1016/j.bbrc.2005.12.171
23. Deng RH, Qiu B, Zhou PH. Chitosan/hyaluronic acid/plasmid-DNA nanoparticles encoding interleukin-1 receptor antagonist attenuate inflammation in synoviocytes induced by interleukin-1 beta. J Mater Sci Mater Med. (2018) 29:155. doi: 10.1007/s10856-018-6160-3
24. Frisbie DD, Ghivizzani SC, Robbins PD, Evans CH, McIlwraith CW. Treatment of experimental equine osteoarthritis by in vivo delivery of the equine interleukin-1 receptor antagonist gene. Gene Ther. (2002) 9:12–20. doi: 10.1038/sj.gt.3301608
25. Goodrich LR, Grieger JC, Phillips JN, Khan N, Gray SJ, McIlwraith CW, et al. ScAAVIL-1ra dosing trial in a large animal model and validation of long-term expression with repeat administration for osteoarthritis therapy. Gene Ther. (2015) 22:536–45. doi: 10.1038/gt.2015.21
26. Watson Levings RS, Smith AD, Broome TA, Rice BL, Gibbs EP, Myara DA, et al. Self-complementary adeno-associated virus-mediated interleukin-1 receptor antagonist gene delivery for the treatment of osteoarthritis: Test of efficacy in an equine model. Hum Gene Ther Clin Dev. (2018) 29:101–12. doi: 10.1089/humc.2017.143
27. Watson Levings RS, Broome TA, Smith AD, Rice BL, Gibbs EP, Myara DA, et al. Gene therapy for osteoarthritis: pharmacokinetics of intra-articular self-complementary adeno-associated virus interleukin-1 receptor antagonist delivery in an equine model. Hum Gene Ther Clin Dev. (2018) 29:90–100. doi: 10.1089/humc.2017.142
28. Glass KA, Link JM, Brunger JM, Moutos FT, Gersbach CA, Guilak F. Tissue-engineered cartilage with inducible and tunable immunomodulatory properties. Biomaterials. (2014) 35:5921–31. doi: 10.1016/j.biomaterials.2014.03.073
29. Gabner S, Ertl R, Velde K, Renner M, Jenner F, Egerbacher M, et al. Cytokine-induced interleukin-1 receptor antagonist protein expression in genetically engineered equine mesenchymal stem cells for osteoarthritis treatment. J Gene Med. (2018) 20:e3021. doi: 10.1002/jgm.3021
30. Chen B, Qin J, Wang H, Magdalou J, Chen L. Effects of adenovirus-mediated bFGF, IL-1Ra and IGF-1 gene transfer on human osteoarthritic chondrocytes and osteoarthritis in rabbits. Exp Mol Med. (2010) 42:684–95. doi: 10.3858/emm.2010.42.10.067
31. Zhang X, Mao Z, Yu C. Suppression of early experimental osteoarthritis by gene transfer of interleukin-1 receptor antagonist and interleukin-10. J Orthop Res. (2004) 22:742–50. doi: 10.1016/j.orthres.2003.12.007
32. Haupt JL, Frisbie DD, McIlwraith CW, Robbins PD, Ghivizzani S, Evans CH, et al. Dual transduction of insulin-like growth factor-I and interleukin-1 receptor antagonist protein controls cartilage degradation in an osteoarthritic culture model. J Orthop Res. (2005) 23:118–26. doi: 10.1016/j.orthres.2004.06.020
33. Zhang P, Zhong ZH Yu HT, Liu B. Exogenous expression of IL-1Ra and TGF-β1 promotes in vivo repair in experimental rabbit osteoarthritis. Scand J Rheumatol. (2015) 44:404–11. doi: 10.3109/03009742.2015.1009942
34. Wang HJ, Yu CL, Kishi H, Motoki K, Mao Z, Bin, et al. Suppression of experimental osteoarthritis by adenovirus-mediated double gene transfer. Chin Med J. (2006) 119:1365–73. doi: 10.1097/00029330-200608020-00009
35. Attur MG, Dave MN, Leung MY, Cipolletta C, Meseck M, Woo SLC, et al. Functional genomic analysis of type II IL-1β decoy receptor: potential for gene therapy in human arthritis and inflammation. J Immunol. (2002) 168:2001–10. doi: 10.4049/jimmunol.168.4.2001
36. Broeren MGA, de Vries M, Bennink MB, Arntz OJ, van Lent PLEM, van der Kraan PM, et al. Suppression of the inflammatory response by disease-inducible interleukin-10 gene therapy in a three-dimensional micromass model of the human synovial membrane. Arthritis Res Ther. (2016) 18:186. doi: 10.1186/s13075-016-1083-1
37. Farrell E, Fahy N, Ryan AE, Flatharta CO, O'Flynn L, Ritter T, et al. VIL-10-overexpressing human MSCs modulate naïve and activated T lymphocytes following induction of collagenase-induced osteoarthritis. Stem Cell Res Ther. (2016) 7:74. doi: 10.1186/s13287-016-0331-2
38. Cameron AD, Even KM, Linardi RL, Berglund AK, Schnabel LV, Engiles JB, et al. Adeno-associated virus-mediated overexpression of interleukin-10 affects the immunomodulatory properties of equine bone marrow-derived mesenchymal stem cells. Hum Gene Ther. (2021) 32:907–18. doi: 10.1089/hum.2020.319
39. Watkins LR, Chavez RA, Landry R, Fry M, Green-Fulgham SM, Coulson JD, et al. Targeted interleukin-10 plasmid DNA therapy in the treatment of osteoarthritis: Toxicology and pain efficacy assessments. Brain Behav Immun. (2020) 90:155–66. doi: 10.1016/j.bbi.2020.08.005
40. Lang A, Neuhaus J, Pfeiffenberger M, Schröder E, Ponomarev I, Weber Y, et al. Optimization of a nonviral transfection system to evaluate Cox-2 controlled interleukin-4 expression for osteoarthritis gene therapy in vitro. J Gene Med. (2014) 16:352–63. doi: 10.1002/jgm.2812
41. Song SY, Hong J, Go S, Lim S, Sohn HS, Kang M, et al. Interleukin-4 gene transfection and spheroid formation potentiate therapeutic efficacy of mesenchymal stem cells for osteoarthritis. Adv Healthc Mater. (2020) 9:1901612. doi: 10.1002/adhm.201901612
42. Broeren MGA, Di Ceglie I, Bennink MB, van Lent PLEM, van den Berg WB, Koenders MI, et al. Treatment of collagenase-induced osteoarthritis with a viral vector encoding TSG-6 results in ectopic bone formation. PeerJ. (2018) 6:e4771. doi: 10.7717/peerj.4771
43. Qiu B, Xu XF, Deng RH, Xia GQ, Shang XF, Zhou PH. Hyaluronic acid-chitosan nanoparticles encoding CrmA attenuate interleukin-1β induced inflammation in synoviocytes in vitro. Int J Mol Med. (2019) 43:1076–84. doi: 10.3892/ijmm.2018.3997
44. Attur MG, Dave M, Cipolletta C, Kang P, Goldring MB, Patel IR, et al. Reversal of autocrine and paracrine effects of interleukin 1 (IL-1) in human arthritis by type II IL-1 decoy receptor. Potential for pharmacological intervention. J Biol Chem. (2000) 275:40307–15. doi: 10.1074/jbc.M002721200
45. Manning K, Rachakonda PS, Rai MF, Schmidt MFG. Co-expression of insulin-like growth factor-1 and interleukin-4 in an in vitro inflammatory model. Cytokine. (2010) 50:297–305. doi: 10.1016/j.cyto.2010.01.010
46. Weimer A, Madry H, Venkatesan JK, Schmitt G, Frisch J, Wezel A, et al. Benefits of recombinant adeno-associated virus (rAAV)-mediated insulinlike growth factor I (IGF-I) overexpression for the long-term reconstruction of human osteoarthritic cartilage by modulation of the IGF-I axis. Mol Med. (2012) 18:346–58. doi: 10.2119/molmed.2011.00371
47. Aguilar IN, Trippel SB, Shi S, Bonassar LJ. Comparison of efficacy of endogenous and exogenous IGF-I in stimulating matrix production in neonatal and mature chondrocytes. Cartilage. (2015) 6:264–72. doi: 10.1177/1947603515578691
48. Aguilar IN, Trippel S, Shi S, Bonassar LJ. Customized biomaterials to augment chondrocyte gene therapy. Acta Biomater. (2017) 53:260–7. doi: 10.1016/j.actbio.2017.02.008
49. Ko JH, Kang YM, Yang JH, Kim JS, Lee WJ, Kim SH, et al. Regulation of MMP and TIMP expression in synovial fibroblasts from knee osteoarthritis with flexion contracture using adenovirus-mediated relaxin gene therapy. Knee. (2019) 26:317–29. doi: 10.1016/j.knee.2019.01.010
50. Ulrich-Vinther M, Stengaard C, Schwarz EM, Goldring MB, Soballe K. Adeno-associated vector mediated gene transfer of transforming growth factor - β1 to normal and osteoarthritic human chondrocytes stimulates cartilage anabolism. Eur Cells Mater. (2005) 10:40–50. doi: 10.22203/eCM.v010a05
51. Venkatesan JK, Rey-Rico A, Schmitt G, Wezel A, Madry H, Cucchiarini M. rAAV-mediated overexpression of TGF-β stably restructures human osteoarthritic articular cartilage in situ. J Transl Med. (2013) 11:211. doi: 10.1186/1479-5876-11-211
52. Noh MJ, Copeland RO Yi Y, Choi KB, Meschter C, Hwang S, et al. Pre-clinical studies of retrovirally transduced human chondrocytes expressing transforming growth factor-beta-1 (TG-C). Cytotherapy. (2010) 12:384–93. doi: 10.3109/14653240903470639
53. Lee H, Kim H, Seo J, Choi K, Lee Y, Park K, et al. TissueGene-C promotes an anti-inflammatory micro-environment in a rat monoiodoacetate model of osteoarthritis via polarization of M2 macrophages leading to pain relief and structural improvement. Inflammopharmacology. (2020) 28:1237–52. doi: 10.1007/s10787-020-00738-y
54. Gao X, Cheng H, Awada H, Tang Y, Amra S, Lu A, et al. A comparison of BMP2 delivery by coacervate and gene therapy for promoting human muscle-derived stem cell-mediated articular cartilage repair. Stem Cell Res Ther. (2019) 10:346. doi: 10.1186/s13287-019-1434-3
55. Matsumoto T, Cooper GM, Gharaibeh B, Meszaros LB Li G, Usas A, et al. Cartilage repair in a rat model of osteoarthritis through intraarticular transplantation of muscle-derived stem cells expressing bone morphogenetic protein 4 and soluble Flt-1. Arthritis Rheum. (2009) 60:1390–405. doi: 10.1002/art.24443
56. Tang R, Harasymowicz NS, Wu CL, Collins KH, Choi YR, Oswald SJ, et al. Gene therapy for follistatin mitigates systemic metabolic inflammation and post-traumatic arthritis in high-fat diet–induced obesity. Sci Adv. (2020) 6:eaaz7492 doi: 10.1126/sciadv.aaz7492
57. Chen Z, Deng S, Yuan D, Liu K, Xiang X, Cheng L, et al. Novel nano-microspheres containing chitosan, hyaluronic acid, and chondroitin sulfate deliver growth and differentiation factor-5 plasmid for osteoarthritis gene therapy. J Zhejiang Univ Sci B. (2018) 19:910–23. doi: 10.1631/jzus.B1800095
58. Antoniades HN, Owen AJ. Growth factors and regulation of cell growth. Annu Rev Med. (1982) 33:445–63. doi: 10.1146/annurev.me.33.020182.002305
59. Ha CW, Noh MJ, Choi KB, Lee KH. Initial phase i safety of retrovirally transduced human chondrocytes expressing transforming growth factor-beta-1 in degenerative arthritis patients. Cytotherapy. (2012) 14:247–56. doi: 10.3109/14653249.2011.629645
60. Cherian JJ, Parvizi J, Bramlet D, Lee KH, Romness DW, Mont MA. Preliminary results of a phase II randomized study to determine the efficacy and safety of genetically engineered allogeneic human chondrocytes expressing TGF-β1 in patients with grade 3 chronic degenerative joint disease of the knee. Osteoarthr Cartil. (2015) 23:2109–18. doi: 10.1016/j.joca.2015.06.019
61. Ha CW, Cho JJ, Elmallah RK, Cherian JJ, Kim TW, Lee MC, et al. A multicenter, single-blind, phase IIa clinical trial to evaluate the efficacy and safety of a cell-mediated gene therapy in degenerative knee arthritis patients. Hum Gene Ther Clin Dev. (2015) 26:125–30. doi: 10.1089/humc.2014.145
62. Kim MK, Ha CW, In Y, Cho S, Do, Choi ES, et al. A multicenter, double-blind, phase III clinical trial to evaluate the efficacy and safety of a cell and gene therapy in knee osteoarthritis patients. Hum Gene Ther Clin Dev. (2018) 29:48–59. doi: 10.1089/humc.2017.249
63. Sellon S, Smith J, De la Vega R, Wisniewski S, Jurisson M, Scrabeck T, et al. A phase I clinical trial of osteoarthritis gene therapy (NCT02790723). Mol Ther. (2022) 30:376.
64. Evans CH, Ghivizzani SC, Robbins PD. Osteoarthritis gene therapy in 2022. Curr Opin Rheumatol. (2023) 35:37–43. doi: 10.1097/BOR.0000000000000918
65. Kelley S, Kivitz A, Senter B, Golod D, Cinar A, Martin E, et al. Interim data from the first-in-human Phase 1 trial of FX201, an intra-articular, helper-dependent adenoviral gene therapy for osteoarthritis - safety, tolerability, biodistribution, and preliminary evaluationof clinical activity in 5 patients. Mol Ther. (2021) 29:288.
66. Cucchiarini M, Terwilliger EF, Kohn D, Madry H. Remodelling of human osteoarthritic cartilage by FGF-2, alone or combined with Sox9 via rAAV gene transfer. J Cell Mol Med. (2009) 13:2476–88. doi: 10.1111/j.1582-4934.2008.00474.x
67. Daniels O, Frisch J, Venkatesan JK, Rey-Rico A, Schmitt G, Cucchiarini M. Effects of raav-mediated sox9 overexpression on the biological activities of human osteoarthritic articular chondrocytes in their intrinsic three-dimensional environment. J Clin Med. (2019) 8:1637. doi: 10.3390/jcm8101637
68. Tao K, Rey-Rico A, Frisch J, Venkatesan JK, Schmitt G, Madry H, et al. rAAV-mediated combined gene transfer and overexpression of TGF-β and SOX9 remodels human osteoarthritic articular cartilage. J Orthop Res. (2016) 34:2181–90. doi: 10.1002/jor.23228
69. Urich J, Cucchiarini M, Rey-Rico A. Therapeutic delivery of raav sox9 via polymeric micelles counteracts the effects of osteoarthritis-associated inflammatory cytokines in human articular chondrocytes. Nanomaterials. (2020) 10:1238. doi: 10.3390/nano10061238
70. Kawata M, Teramura T, Ordoukhanian P, Head SR, Natarajan P, Sundaresan A, et al. Krüppel-like factor-4 and Krüppel-like factor-2 are important regulators of joint tissue cells and protect against tissue destruction and inflammation in osteoarthritis. Ann Rheum Dis. (2022). doi: 10.1136/annrheumdis-2021-221867. [Epub ahead of print].
71. Wang Y, He SH, Liang X, Zhang XX Li SS, Li TF. ATF4-modified serum exosomes derived from osteoarthritic mice inhibit osteoarthritis by inducing autophagy. IUBMB Life. (2021) 73:146–58. doi: 10.1002/iub.2414
72. Fisch KM, Gamini R, Alvarez-Garcia O, Akagi R, Saito M, Muramatsu Y, et al. Identification of transcription factors responsible for dysregulated networks in human osteoarthritis cartilage by global gene expression analysis. Osteoarthr Cartil. (2018) 26:1531–8. doi: 10.1016/j.joca.2018.07.012
73. Ruan MZC, Dawson B, Jiang M-M, Gannon F, Heggeness M, Lee BHL. Quantitative imaging of murine osteoarthritic cartilage by phase-contrast micro-computed tomography. Arthritis Rheum. (2013) 65:388–96. doi: 10.1002/art.37766
74. Ruan MZC, Cerullo V, Cela R, Clarke C, Lundgren-Akerlund E, Barry MA, et al. Treatment of osteoarthritis using a helper-dependent adenoviral vector retargeted to chondrocytes. Mol Ther Methods Clin Dev. (2016) 3:16008. doi: 10.1038/mtm.2016.8
75. Stone A, Grol MW, Ruan MZC, Dawson B, Chen Y, Jiang M-M, et al. Combinatorial Prg4 and Il-1ra gene therapy protects against hyperalgesia and cartilage degeneration in post-traumatic osteoarthritis. Hum Gene Ther. (2019) 30:225–35. doi: 10.1089/hum.2018.106
76. Seol D, Choe HH, Zheng H, Brouillette MJ, Fredericks DC, Petersen EB, et al. Intra-articular adeno-associated virus-mediated proteoglycan 4 gene therapy for preventing posttraumatic osteoarthritis. Hum Gene Ther. (2022) 33:529–40. doi: 10.1089/hum.2021.177
77. Tashkandi M, Ali F, Alsaqer S, Alhousami T, Cano A, Martin A, et al. Lysyl oxidase-like 2 protects against progressive and aging related knee joint osteoarthritis in mice. Int J Mol Sci. (2019) 20:4798. doi: 10.3390/ijms20194798
78. Venkatesan N, Barré L, Benani A, Netter P, Magdalou J, Fournel-Gigleux S, et al. Stimulation of proteoglycan synthesis by glucuronosyltransferase-I gene delivery: a strategy to promote cartilage repair. Proc Natl Acad Sci USA. (2004) 101:18087–92. doi: 10.1073/pnas.0404504102
79. Fu X, Qiu R, Tang C, Wang X, Cheng X, Yin M. Effects of GGCX overexpression on anterior cruciate ligament transection-induced osteoarthritis in rabbits. Mol Med Rep. (2018) 17:3821–8. doi: 10.3892/mmr.2017.8304
80. Hsieh JL, Shen PC, Shiau AL, Jou IM, Lee CH, Teo ML, et al. Adenovirus-mediated kallistatin gene transfer ameliorates disease progression in a rat model of osteoarthritis induced by anterior cruciate ligament transection. Hum Gene Ther. (2009) 20:147–58. doi: 10.1089/hum.2008.096
81. Ashraf S, Kim BJ, Park S, Park H, Lee SH. RHEB gene therapy maintains the chondrogenic characteristics and protects cartilage tissue from degenerative damage during experimental murine osteoarthritis. Osteoarthr Cartil. (2019) 27:1508–17. doi: 10.1016/j.joca.2019.05.024
82. Grossin L, Cournil-Henrionnet C, Pinzano A, Gaborit N, Dumas D, Etienne S, et al. Gene transfer with HSP 70 in rat chondrocytes confers cytoprotection in vitro and during experimental osteoarthritis. FASEB J. (2006) 20:65–75. doi: 10.1096/fj.04-2889com
83. Yoon DS, Lee K-M, Choi Y, Ko EA, Lee N-H, Cho S, et al. TLR4 downregulation by the RNA-binding protein PUM1 alleviates cellular aging and osteoarthritis. Cell Death Differ. (2022) 29:1364–78. doi: 10.1038/s41418-021-00925-6
84. Na HS, Lee S-Y, Lee DH, Woo JS, Choi S-Y, Cho K-H, et al. Soluble CCR2 gene therapy controls joint inflammation, cartilage damage, and the progression of osteoarthritis by targeting MCP-1 in a monosodium iodoacetate (MIA)-induced OA rat model. J Transl Med. (2022) 20:428. doi: 10.1186/s12967-022-03515-3
85. Cao C, Shi Y, Zhang X, Li Q, Zhang J, Zhao F, et al. Cholesterol-induced LRP3 downregulation promotes cartilage degeneration in osteoarthritis by targeting Syndecan-4. Nat Commun. (2022) 13:7139. doi: 10.1038/s41467-022-34830-4
86. Dong Q, Wei L, Zhang MQ, Wang X. Regulatory RNA binding proteins contribute to the transcriptome-wide splicing alterations in human cellular senescence. Aging. (2018) 10:1489–505. doi: 10.18632/aging.101485
87. Wojdasiewicz P, Poniatowski ŁA, Szukiewicz D. The role of inflammatory and anti-inflammatory cytokines in the pathogenesis of osteoarthritis. Mediat Inflamm. (2014) 2014:561459. doi: 10.1155/2014/561459
88. Lara-Castillo N, Johnson ML, LRP. receptor family member associated bone disease. Rev Endocr Metab Disord. (2015) 16:141–8. doi: 10.1007/s11154-015-9315-2
89. Zhao L, Huang J, Fan Y, Li J, You T, He S, et al. Exploration of CRISPR/Cas9-based gene editing as therapy for osteoarthritis. Ann Rheum Dis. (2019) 78:676–82. doi: 10.1136/annrheumdis-2018-214724
Glossary
OA, osteoarthritis; DMOAD, disease-modifying osteoarthritis drug; IA, intra-articular; Ad, Adenovirus; HDAd or HDV, helper-dependent adenovirus; AAV, adeno-associated virus; RV, retroviruses; LV, lentivirus; MSCs, mesenchymal stem cells; miRNAs, microRNAs; siRNA, small interfering RNA; circRNA, circular RNA; IL, interleukin; IL-1Ra, interleukin 1 receptor antagonist; ACLT, anterior cruciate ligament transection; IND, investigational new drug; CS, chitosan; HA, hyaluronic acid; pDNA, plasmidDNA; Sc AAV, self-complementary AAV; Nab, neutralizing antibodies; TNF-α, Tumor Necrosis Factor alpha; MMPs, matrix metalloproteinases; bFGF, basic fibroblast growth factor; IGF-1, insulin-like growth factor-1; TIMP-1, Tissue inhibitor matrix metalloproteinase 1; ADAMTS5, disintegrin and metalloproteinase with thrombospondin motifs 5; GAG, glycosaminoglycan; TGFβ1, transforming growth factor-β1; sTNF-RI, soluble tumor necrosis factor-α receptor type I; IL-1RII, type II IL-1β receptor; NO, nitric oxide; iNOS, inducible nitric oxide synthase; PGE2, prostaglandin E2; CIOA, collagenase induced osteoarthritis; BM-MSCs, Bone marrow-derived mesenchymal stromal cells; Cox-2, cyclooxygenase-2; LPS, lipopolysaccharide; ECM, extracellular matrix; TSG6, tumor necrosis factor α-stimulated gene 6; CrmA, cytokine response modifier A; IKDC, International Knee Documentation Committee; RLN, relaxin; TIMP-1, tissue inhibitor of metalloprotease-1; BMPs, bone morphogenetic proteins; LAP, pro-peptide latency-associated peptide; LTBPs, latent TGF-β binding proteins;; PTHrP, parathyroid hormone-related peptide; TG-C, TissueGene-C; FDA, Food and Drug Administration; KSCRS, Knee Society Clinical Rating System; VAS, Visual Analog Scale; IKDC, International Knee Documentation Committee; MIA, monosodium iodoacetate; MDSCs, muscle-derived stem cells; sFLT-1, soluble Fms-like Tyrosine Kinase 1; FST, follistatin; DMM, destabilization of the medial meniscus; GDF-5, growth and differentiation factor-5; NMPs, nano-microspheres; SOX9, sex-determining region Y-type high mobility group box 9; KLF, krüppel-like factor; ATF-4, activating transcription factor 4; ACAN, aggrecan; COL2A1, collagen type II alpha 1 chain; RNA, ribonucleic acid; DNA, deoxyribonucleic acid; PRG4, Proteoglycan 4; PTOA, post-traumatic OA; LOXL2, the copper-dependent amine oxidase lysis oxidase-like 2; PG, Proteoglycans; GlcAT-I, GAG-synthesizing enzyme β1,3-glucuronosyltransferase-I; GGCX, γ-glutamyl carboxylase; MPG, matrix Gla protein; ucMPG, non-functional MPG; RHEB, Ras homolog enriched in the brain; HSP70, Heat shock protein 70; RBP, RNA-binding protein; PUM1, Pumilio 1; MCP-1, monocyte chemoattractant protein-1; CCR2, C-C chemokine receptor type 2; LDL, Low-density lipoprotein; LRO, LDL receptor-related proteins; PRISMA-ScR, PRISMA Extension for Scoping Reviews; NGF, nerve growth factor.
Keywords: genetic therapy, osteoarthritis, gene transfer techniques, interleukins, growth factors, transcription factors
Citation: Uebelhoer M, Lambert C, Grisart J, Guse K, Plutizki S and Henrotin Y (2023) Interleukins, growth factors, and transcription factors are key targets for gene therapy in osteoarthritis: A scoping review. Front. Med. 10:1148623. doi: 10.3389/fmed.2023.1148623
Received: 20 January 2023; Accepted: 15 March 2023;
Published: 03 April 2023.
Edited by:
Elisa Belluzzi, University of Padua, ItalyReviewed by:
Magali Cucchiarini, Saarland University Medical Center, GermanyChristopher Evans, Mayo Clinic, United States
Laurie R. Goodrich, Colorado State University, United States
Susanne Grässel, University Medical Center Regensburg, Germany
Dominik R. Haudenschild, University of California, Davis, United States
Copyright © 2023 Uebelhoer, Lambert, Grisart, Guse, Plutizki and Henrotin. This is an open-access article distributed under the terms of the Creative Commons Attribution License (CC BY). The use, distribution or reproduction in other forums is permitted, provided the original author(s) and the copyright owner(s) are credited and that the original publication in this journal is cited, in accordance with accepted academic practice. No use, distribution or reproduction is permitted which does not comply with these terms.
*Correspondence: Melanie Uebelhoer, bWVsYW5pZS51ZWJlbGhvZXImI3gwMDA0MDthcnRpYWxpcy5jb20=
†These authors have contributed equally to this work and share first authorship