- 1Department of Biosciences, School of Liberal Arts and Sciences, Mody University of Science and Technology, Lakshmangarh, Rajasthan, India
- 2Department of Life Sciences, Hemchandracharya North Gujarat University, Patan, Gujarat, India
- 3Department of Environment Sciences, School of Sciences, P P Savani University, Surat, Gujarat, India
- 4Department of Chemistry, Medicaps University, Indore, Madhya Pradesh, India
- 5School of Environment and Sustainable Development, Central University of Gujarat, Gandhinagar, Gujarat, India
- 6School of Chemical Sciences, Central University of Gujarat, Gandhinagar, Gujarat, India
- 7Department of Biochemistry, M B Patel Science College, Anand, Gujarat, India
- 8Redox Regulation Laboratory, Department of Zoology, College of Basic Science and Humanities, Odisha University of Agriculture and Technology, Bhubaneswar, India
- 9Department of Veterinary Clinical Sciences, College of Veterinary Medicine, Iowa State University, Ames, IA, United States
Medical-grade oxygen is the basic need for all medical complications, especially in respiratory-based discomforts. There was a drastic increase in the demand for medical-grade oxygen during the current pandemic. The non-availability of medical-grade oxygen led to several complications, including death. The oxygen concentrator was only the last hope for the patient during COVID-19 pandemic around the globe. The demands also are everlasting during other microbial respiratory infections. The yield of oxygen using conventional molecular zeolites in the traditional oxygen concentrator process is less than the yield noticed when its nano-form is used. Nanotechnology has enlightened hope for the efficient production of oxygen by such oxygen concentrators. Here in the current review work, the authors have highlighted the basic structural features of oxygen concentrators along with the current working principle. Besides, it has been tried to bridge the gap between conventional oxygen concentrators and advanced ones by using nanotechnology. Nanoparticles being usually within 100 nm in size have a high surface area to volume ratio, which makes them suitable adsorbents for oxygen. Here authors have suggested the use of nano zeolite in place of molecular zeolites in the oxygen concentrator for efficient delivery of oxygen by the oxygen concentrators.
1. Introduction
Oxygen is the basic requirement for both a healthy and a diseased individual. There are certain medical diseases, especially cardiovascular respiratory disorders, where there is a requirement for pure or concentrated oxygen by the patient (1, 2). This pure or concentrated oxygen is indeed known as medical oxygen, and in the absence of it, a patient may experience hypoxemia [low levels of oxygenated blood (3)]. Hypoxemia is caused by a range of common conditions—including childhood pneumonia, newborn conditions (4, 5), and obstetric emergencies (6, 7). The body of the sufferer will experience severe detrimental consequences from hypoxemia on the cells that carry out crucial biological functions (8). The atmospheric air with a 21% O2 concentration and when its percentage is below 19.5% by volume and/or highly elevated CO2 level in air is called an oxygen-deficient condition (9). The O2 deficient in body a comparatively O2 less environment can lead to a condition called asphyxiation (10). When severe hypoxemia is not quickly diagnosed and addressed, it can lead to death.
For a healthy individual, 21% of oxygen is sufficient, but for a patient suffering from a respiratory disease or cardiovascular then, there is a requirement for concentrated or pure oxygen or medical grade oxygen. Oxygen is listed as an essential drug in India. If pure oxygen is not provided to the patient on time, during hypoxemia, then the lung will be devoid of oxygenated blood, from where the oxygen will not be supplied to the vital organs of the body (11). As a result, it will lead to damage of organs and, ultimately, the death of the patients. Based on the application, oxygen can be classified into two classes: medical and industrial grade. Medical grade oxygen is highly pure (12) in order to supply concentrated oxygen to the patient, while impurities could be permitted in the industrial grade, which is needed in steel making, etc. Moreover, medical-grade oxygen should be supplied in sterilized cylinders in order to prevent other diseases as per the gas cylinder rule 1981 (13, 14).
For the patient’s recovery from several illnesses, including respiratory infections such as asthma, emphysema, and perhaps other severe acute respiratory syndrome (SARS)-coronavirus-2 (CoV2) infestations, supplemental oxygen is recommended (15). Oxygen therapy is provided by means of an oxygen cylinder, oxygen generation plant, and oxygen concentrators. The demand for oxygen concentrators has grown drastically due to the SARS-CoV2. In order to help patients with lower blood oxygen levels, oxygen concentrators are biomedical equipment that typically concentrates oxygen from the atmosphere (16). Oxygen concentrators have been used for the last several decades, especially in low-income countries, where the medical facility is poorly developed, or in remote or hilly areas where hospitals are very far. Oxygen concentrators could be either fixable or static, or they could be mobile or portable (16).
As the word “concentrator” indicates the concentration or enrichment of anything, and here oxygen is being concentrated from the air mixture. So, in layman’s language, we can say that this device concentrates oxygen from the air by eliminating nitrogen from them. Our atmospheric air contains a mixture of gases, mainly nitrogen (78%), oxygen (21%), and a small number of other gases. So, the main purpose of this device is to eliminate the nitrogen (which is present in a larger amount) from the air and supply the residual component, i.e., oxygen up to 90% purity, to the patient. When the air is sent through an oxygen concentrator, it is converted to 90–95 percent pure oxygen and 5–10 percent nitrogen. Since it is challenging to obtain that amount of oxygen alone without the aid of medical equipment, the nitrogen is segregated to ensure that patients receive the maximum dose of oxygen available. Home-based oxygen concentrators have numerous advantages compared to oxygen cylinders, which may leak or burst (17). Compared to conventional oxygen cylinders, oxygen concentrators are significantly less hazardous (18). Old-fashioned oxygen cylinders have been replaced with household and transportable oxygen concentrators that can “generate” their own oxygen (19). From time to time, there were several modifications also in the oxygen concentrator; for instance, few investigators used it alone, few used it with other oxygen devices like ventilators (20), and few used nanomaterials in place of micron-sized zeolites to increase the efficiency of the concentrator (21). Molecular zeolites are artificially produced molecular sieves. They are prepared from alkali metal aluminosilicates. They have common applications in the gas chromatography also to sieve the molecules. The calcium aluminosilicate has pore diameter of 0.5 nm, while sodium aluminosilicate has pore diameter of 1 nm. They are produced using nanotechnology tools and techniques. According to some investigators, especially from Africa, Nigeria, where continuous electricity supply is a big challenge, the oxygen concentrators were fitted with solar panels for a continuous, uninterrupted supply of oxygen to the patient (18, 22).
We searched oxygen concentrators and portable oxygen concentrators on Science Direct for the time frame of 2017–2022, and the authors found a sharp increase in the number of articles in these 6 years. The key word used for this search was “oxygen concentrators.” The number of articles drastically increased after the COVID-19 pandemic. Figure 1 shows the articles available in Science Direct within this time frame. So, from this research, we tried to bridge this gap with the latest information on oxygen concentrators which may help medical professionals in the future. It may also help in increasing the efficiency of president oxygen concentrators by using advancements in technology.
In the current review work, emphasis is given to the basic working principle of oxygen concentrators. Besides this, recent trends in the utilization of portable oxygen concentrators have been discussed. Nanosized zeolites are produced from the molecular zeolites using nanotechnology tools. These are crystalline microporous solids and have physicochemical properties similar to those of micron-sized crystals. The method for increasing the efficiency of current oxygen concentrators by using nanosized molecular zeolites is elegantly reviewed with an objective that the use of nanosized molecular zeolites may solve the demands of oxygen supply in a future pandemic or any disease outbreak.
2. Types of oxygen based on their applications
There are essentially two sorts of oxygen depending upon grade: medical and industrial. Many industries run their production facilities using commercial oxygen (23). Manufacturing oxygen for industrial purposes is also required to meet industrial standards. Oxygen plants used in the generation of oxygen for industrial applications must meet the specifications of the industry. The procedure for generating medical and industrial oxygen is the same. Furthermore, medical oxygen is produced with a significant purity level, and the equipment used to produce it is made to the best of its ability (24). Medical oxygen is ultra-pure oxygen that has been created for use in the human body and is utilized in medical procedures. Medical oxygen is purified in the air separation process until it fulfills regulatory requirements (25).
In operating rooms, emergency departments, and ambulances, medical-grade oxygen is being used. Medical oxygen production requires adequate drug licenses and adherence to standard operating procedures, and it is recognized as a drug on the World Health Organization (WHO)’s list of essential medicines. To generate medical oxygen, it is also required to adhere to Indian Pharmacopeia (IP) guidelines (25). Figure 2 shows the types of oxygen as per the requirement and applications.
2.1. Liquid medical oxygen
Highly pure oxygen in the form of liquid medical oxygen (LMO) is being used for medical care and was invented for use in the human body (26). Liquification promotes smoother conveyance plus greater storage. These kinds of LMO can indeed be kept in a mobile cylinder that is attached to the main conduit. Due to the high concentration of liquid oxygen, a compact reservoir may hold much of the gas. Sarkar et al. in 2014 described the importance of liquid ventilation in treating several diseases and considered it effective over oxygen in the form of gas (4, 27).
3. Oxygen therapy
Oxygen therapy is the most commonly reliable method to treat hypoxemia in cardiovascular and respiratory system-related disorders (28, 29) and during pandemic disease outbreaks. All these patients require a continuous supply of medical-grade oxygen for the proper functioning of their vital organs and body. The method of distribution and equipment used for oxygen therapy influence the selection of variables, such as the patient’s individual requirements and the discretion of the treating hospital personnel (30). Oxygen cylinders, oxygen production facilities, and oxygen concentrators are used to administer oxygen therapy.
3.1. Areas where oxygen therapy is required
Multiple chronic and acute medical disorders, including chronic asthma, cystic fibrosis, pulmonary hypertension, obstructive sleep apnea, heart failure, cases of anaphylaxis, severe trauma, seizure, or hypothermia, are managed by oxygen therapy (31–33). Whenever hypoxemia is found in any of the following situations, oxygen therapy is necessary for operating rooms as well. In the case of children and neonates, oxygen therapy is required during prematurity, birth asphyxia, acute sepsis, shock, severe pneumonia, meningitis, brain injury, coma, anemia, severe malaria, heart failure, acute asthma, etc. Among adults, oxygen therapy is required during, pneumonia, interstitial lung disease, pulmonary sarcoidosis, lower and upper respiratory infections, pneumoconiosis, severe malaria, meningitis, ischemia, etc. Figure 3 shows various conditions requiring oxygen in different age groups.
3.1.1. In adults
i. The main conditions requiring oxygen are hypoxemia, breathlessness, and dyspnea (34).
ii. Every patient’s regular vitals must include pulse oximetry.
iii. Any patient with a SpO2 below 90% on a pulse oximeter or clinical hypoxemia symptoms should be given oxygen, according to the recommendation (35).
iv. Under some serious circumstances, such as brain injury/infection/coma, shock, sepsis, severe anemia, heart failure, or severe heart failure.
v. Whenever the Oxygen saturation, i.e., SpO2 is <94%, oxygen should also be administered (26).
vi. While seeking medical examination as well as background, every seriously sick person should be given oxygen by paramedics, nurses, and other medical practitioners.
According to the clinical recommendations for oxygen therapy, oxygen should be administered to all patients who are in need.
3.1.2. Oxygen care in neonates
Newborn infants receiving oxygen therapy have lower normal oxygen saturation levels in their body as compared to the older neonates. And the condition of severity in low oxygen concentration in their body of neonates is high if they are delivered prematurely. Walsh et al. in 2009, reported to use oxygen for the treatment of several diseases like respiratory disorders and hypoxia, and the treatment process has positive and detrimental effects on the patients. The investigators reported that the extent of use of oxygen in neonates is not well known but they have shown that such treatment system has a positive role in the arsenal for neonates (36).
Dennery in 2010 reported that using medical oxygen in preterm infants is not recommended as it may have a detrimental effect in the long term in neonates. So, the liberal use of oxygen (blood oxygen saturation of >94%) and restrictive use (resulting in a blood oxygen saturation of <80–85%) are detrimental and may have a long-term negative effect. So, appropriate concentration and duration of oxygen may be effective in neonates and infants (37). Ramji et al. in 2015 reported that the use of oxygen had been challenged earlier in both clinical and biochemical results in neonates due to their adverse outcomes. But now, its use is recommended to treat ill neonates in the delivery room and neonatal intensive care unit (NICU). The use of oxygen has shown improvement in neonate survival (38).
3.1.3. Oxygen care in children’s care
Among the most common respiratory ailments in children under the age of five is pneumonia, which accounts for over 15% of all annual fatalities worldwide. Out of this 15%, almost 13% of children with pneumonia develop hypoxemia (39–41). Pneumonia can be caused by bacteria, fungi and viruses (42). In such cases, the oxygen-holding capacity of alveoli and, ultimately, the lungs get reduced, and child needs urgent pure or medical oxygen. If pulse oximetry and oxygen therapy are consistently accessible, the annual child mortality rate could be reduced. Children living at or above 2,500 m above sea level with <90% blood oxygen levels as measured by pulse oximetry are required to receive oxygen therapy (43, 44). If normal oxygen saturation in children exposed to higher altitudes (>2,500 m above sea level) is lesser than that in children living at sea level, a SpO2 level of 87 percent can be considered as a criterion for administering oxygen. Any children with a SpO2 below 90% should indeed be administered oxygen while being evaluated by pulse oximetry (45).
4. Common oxygen supply system
There are several medical oxygen supply methods to patients, but the most common methods are the form of oxygen cylinders, oxygen concentrators, and liquid tanks & oxygen generators (46). The following methods are mainly used to administer medical oxygen (shown in Figure 4).
5. Requirements of medical gas (oxygen) and air points
All critical care areas of the hospitals, such as emergency wards, operation theatres (OT), intensive care unit (ICU), NICU, and labor room, should have an uninterrupted 24 × 7 h oxygen supply. Besides this high dependency unit (HDU) should also have a proper oxygen supply. As per industry norms, the requirement of oxygen supply in hospitals should be as shown in Table 1.
6. Side effects of breathing pure oxygen
Even though a low level of oxygen in the blood is not ideal, this does not entail that breathing extremely pure oxygen at high pressure and for long periods is beneficial to a patient’s health. Breathing oxygen for a longer time and breathing oxygen at high pressure negatively impact an individual’s health. Breathing pure oxygen for a longer time could lead to the following symptoms and diseases in the patient, shown in Figure 5. While, Figure 6 shows the side effects of breathing pure oxygen at high pressure.
7. Components of oxygen concentrators
The oxygen concentrators have several components like control, filters, air inlet, waste outlet, and flow splitter (47). The control comprises the power switch, oxygen cock, and valve for adjustment below (48, 49). A series of filters are arranged in order, external coarse (gross particle filter), pre-filter, and inlet filter (compressor inlet filter (0.1 μm) in the oxygen concentrators. Besides this, it has a compressor (20 PSI), heat exchanger, 4-way solenoid-controlled valve, sieve canisters with zeolites, pressure equalization valve, check valve, pressure regulator, product canister, outlet filter (0.3 μm), and flow meter (50, 51). The zeolite crystals present in canisters have an average lifespan of 60,000 h (49). Figure 7 shows various components of an oxygen concentrator.
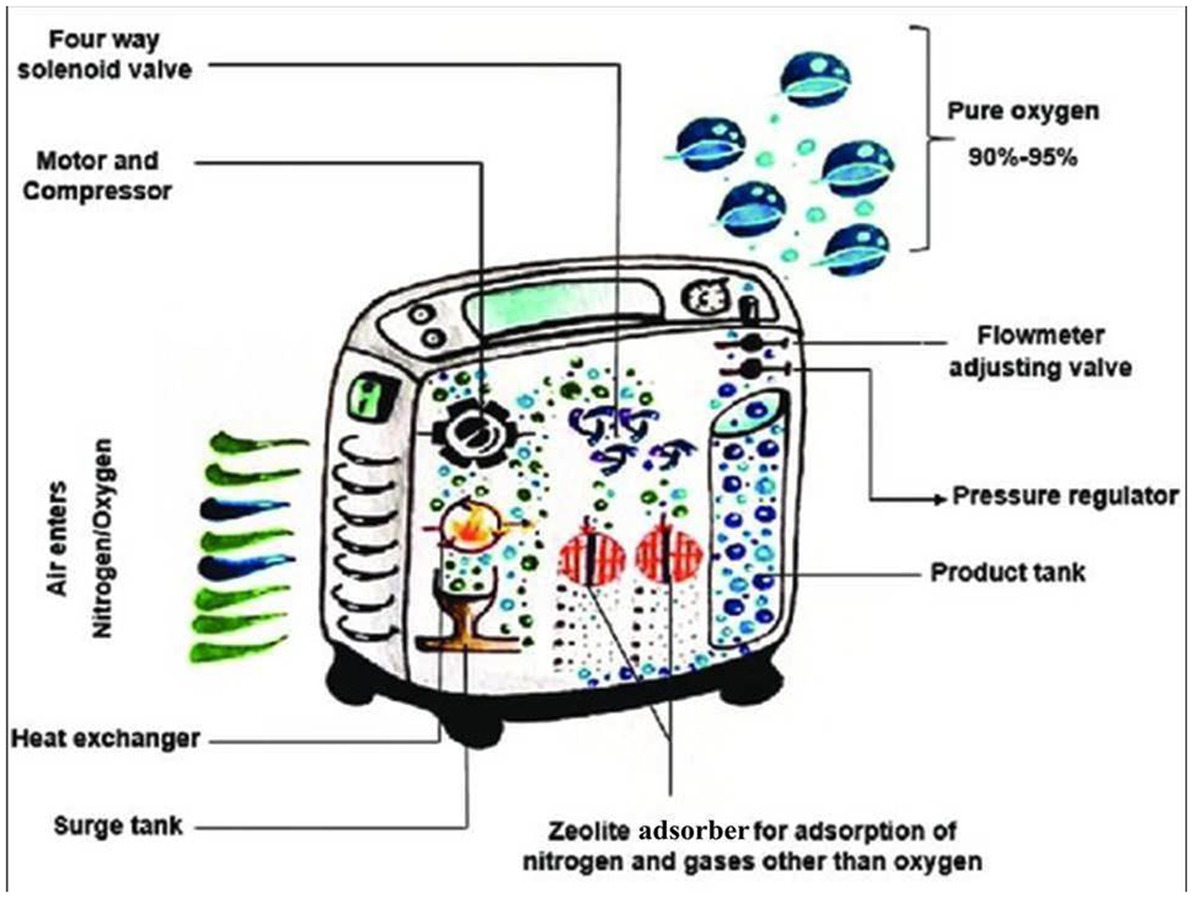
Figure 7. Various components of an oxygen concentrator [adopted from Todur et al. (47)].
8. Principle of oxygen concentrators
Oxygen concentrators work on the principle of “pressure swing adsorption” (PSA) technology (52, 53). The word “pressure swing adsorption” implies that the applied pressure keeps on swinging, and consequently, there is continuous adsorption and desorption of gases, i.e., two stages from the molecular sieves. Two stages work in alternation, 1. Adsorption/Production 2. Blowdown/Purge (52). While Figure 8 shows detailed four phases of PSA technology of an oxygen concentrator. The compressed air (21% O2+ 78% N2) is supplied to one of the columns present in the canister. These columns are filled with adsorbent zeolite materials made of aluminum-silicate hydrates (54).
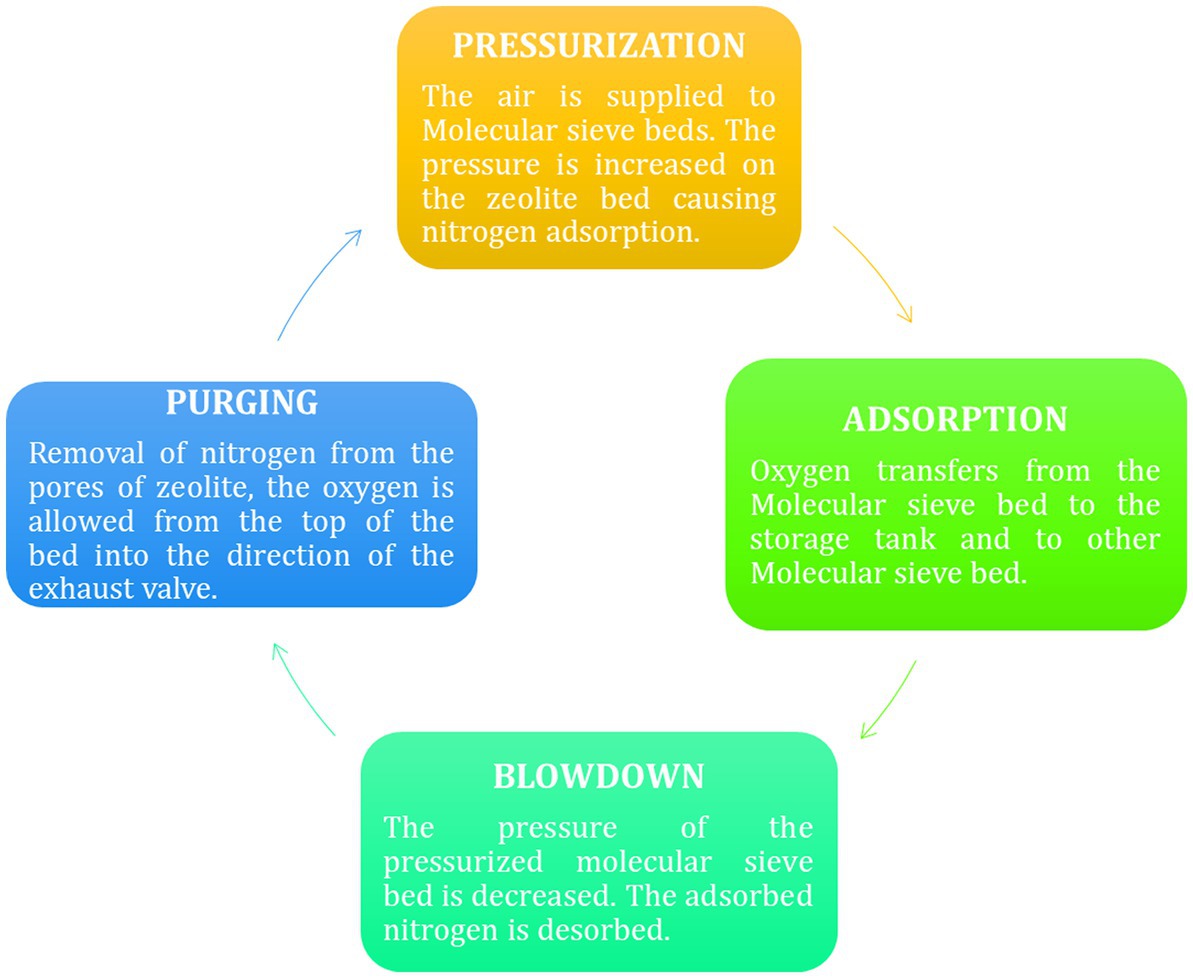
Figure 8. Four stages of PSA cycle (51).
Firstly, column one gets compressed air, during which N2 gets adsorbed on the zeolites’ surface while the O2 (present in lower content in air) is passed through these zeolite columns and is collected in an oxygen tank. Due to the continuous adsorption first column gets saturated, so the valve closes, and pressurized air is moved into the second canister containing molecular sieves (55). During this period, the pressure on the earlier column gets lowered, due to which the adsorbed nitrogen gas is released into the surrounding. This particular step, where the column releases the N2 from the column and gets prepared for the next cycle, is called regeneration (56). Again, in the next cycle, another column will be regenerated by releasing the adsorbed N2, this adsorption and desorption continue due to a swing in pressure; hence it is called “pressure swing technology.”
9. Working of oxygen concentrators
A stable supply of oxygen-enriched airflow is delivered by oxygen concentrators. Devices called oxygen concentrators (also known as oxygen generators) drive indoor air through several filters to eliminate particles, germs, and other impurities (57). The first stage in the concentration procedure includes pumping air into one of the two cylinders, which comprise a semi-permeable membrane or a “molecular sieve” element. Next, nitrogen is removed, generating pure oxygen (90 percent or more) and a minor amount of certain other gases present in indoor air (58–61). An accessible source of oxygen-enriched air is an oxygen concentrator. Nitrogen is desorbed and pulled outside into the environment simultaneously in another tank. The second step includes the cylinders’ functionality being reverted in a scheduled loop to ensure that patients receive a steady oxygen supply. Certain kinds of high-flow oxygen concentrators can produce up to 10 L/min of oxygen, whereas low-flow oxygen concentrators typically give an oxygen flow of 0.5–5 L/min (62, 63).
In essence, continuous flow dose administration and pulse mode distribution are indeed the two different ways oxygen is administered in oxygen concentrators. While pulse mode delivery pulses an oxygen “bolus” (57) whenever the user starts to breathe, continuous flow dose treatment provides a consistent, smooth, and efficient oxygen flow depending on the configuration value of Lmin−1.
Since it provides oxygen through the cannula whenever you breathe, the pulse dose setting is typically utilized during the day. Additionally, pulse dosage concentrators have a much more compact design as well as a better battery life (63). The working principle of an oxygen concentrator is shown below in Figure 9.
10. Types of oxygen concentrators
Both stationary and portable oxygen concentrators are available. A continuous oxygen supply is provided by stationary (home) concentrators, which have an average weight of around 10 kg and a flow rate of 0.5 to 10–15 L/min. For long-term oxygen therapy (LTOT) users who want a smaller, portable oxygen supply in a movable apparatus, portable oxygen concentrators are indeed the latest innovation available (64, 65). Weight, size, oxygen flow settings, range of L/min, battery life, and other characteristics of portable concentrators differ (63). Table 2 shows the major differences between a portable oxygen concentrator (POC) and a stationary oxygen concentrator (SOC).
A more recent choice is a super-compact home concentrator, which might weigh as little as 4.5 kg. These portable devices operate on both alternating current (AC; for example, from a wall outlet) and direct current (DC; for example, from a cigarette lighter socket; e.g., they can be smoothly shifted from one place to the other or they can be transported by car during travel). Presently, these can handle oxygen flow rates of up to 2 L/min (63). Zeolites are used in various processes to improve product quality, processing rates, energy efficiency, and the environmental consequences of the oxygen purification process (66). Figure 10A is a stationary oxygen concentrator, while 10B is a POC.
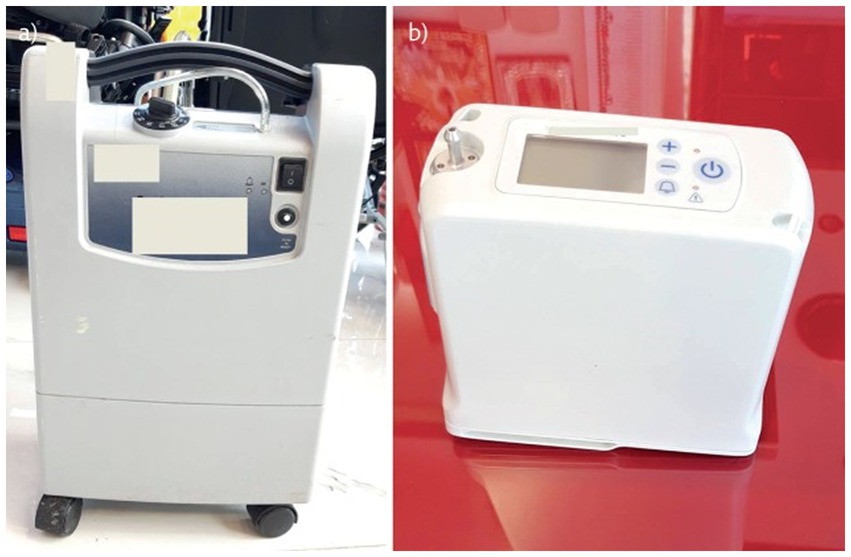
Figure 10. Oxygen concentrators. (A) Stationary oxygen concentrator. (B) Portable oxygen concentrator adapted from Hardvella (63).
11. Clinical applications of oxygen concentrators
In the medical profession, there have been four main instances under which oxygen concentrators are being used: oxygen therapy in small hospitals; oxygen therapy for pre-term neonates with chronic lung disease who need oxygen after discharge; oxygen therapy for older children with chronic obstructive pulmonary disease, pneumonia, pulmonary embolism, or emphysema; and oxygen therapy for children with acute respiratory distress syndrome (ARDS) with extensive fibrosis who need O2 therapy for a prolonged period. Besides this, oxygen concentrators could be used in hospitals during surgery to maintain tissue oxygenation during anesthesia and the resuscitation of patients (67). Figure 11 shows the clinical applications of oxygen concentrators.
12. Supportive data regarding utilization of oxygen concentrators
Ress and Dudley (1998) (68) reported the role of oxygen therapy, especially oxygen or oxygen concentrators for patients being treated at home. Employing a recently designed polymer of poly [1-(trimethylsilyl)-1-propyne] with an oxygen permeability of 61 × 10 cm3 (STP) cm/cm2 s cmHg, which itself is 17 times greater than those of the membrane material of traditional concentrators, Akutsu et al. in 1990 built a small, light-weight oxygen concentrator. The oxygen and nitrogen selectivity was 1.80. The dimension and weight of the O2 concentrator were about 325 × 180 × 150 mm 4.0 kg, respectively. It generated an oxygen concentration of about 30%, and the maximum flow rate was 41/min (68). Ritz et al. (69) suggested using oxygen concentrators during mass causality situations. A range of oxygen concentrator layouts is accessible, including smaller, more portable units that may serve a single patient and bigger units that could also serve a group of patients or an entire facility (69).
Pollock and Natoli (70) reported the use of chemical oxygen generation for oxygen therapy. To assess the effectiveness of the emOx mobile non-pressurized emergency powdered oxygenation delivery system. This product, which chemically creates oxygen, is promoted to be used as urgent first aid until trained medical support is accessible (70). According to McCoy et al. (71), there are now more possibilities for home oxygen therapy equipment than there were a few years ago due to technological advancements, financial aid from third-party providers, and patient demands. With the development of intermittent-flow delivery, oxygen concentrators that yield 99 percent oxygen authenticity have replaced packaged gas systems (oxygen concentrators) that delivered oxygen that was 99 percent pure, according to the United States Pharmacopeia (71, 72).
In patients with chronic obstructive pulmonary disease (COPD), Yanez et al. (73) documented oxygenation using a single portable pulse-dose oxygen-conserving device and integrated stationery and portable oxygen delivery systems. They said that portable oxygen devices, i.e., oxygen concentrators, simplify and facilitate patient therapy. The subjects chose to use a single portable oxygenation system when in an ambulance or at home. Moreover, fixed and portable systems functioning together generated higher amounts of oxygen than portable systems working alone (73). Katz et al. (74) analyzed the oxygen uptake during rest and exercise in mild COPD patients using portable oxygen concentrators in order to improve the effectiveness of oxygen generation and nitrogen adsorption in oxygen concentrators (74).
Pan et al. (75) suggested the usage of nanosized zeolites. They suggested the device as a basic requirement for lung infection-associated patients. To effectively adsorb nitrogen from the air, a 13X nanosize zeolite with Li+ exchanged is utilized as the adsorbent. To better understand the pressurization and depressurization processes taking place inside the microporous region of nanosized zeolites, a dynamic model of the pressure and vacuum swing adsorption units was created (21). The effectiveness of continuous versus pulsed oxygen administration was compared by Chen et al. in 2017 utilizing an accurate adult nasal airway replica. The main objectives of this work were to examine steady flow (SF) from a compressed oxygen tank to PF for a commercial POC and to construct a prospective in vitro analysis for inhaling oxygen supply employing a set of plausible airways replicas (75).
In a pediatric ward in Malawi, Meyers et al. in 2018 documented employing bubble continuous positive airway pressure (bCPAP) to treat seriously unwell children. Humidified bCPAP air/oxygen flow was given by customized oxygen concentrators or oxygen cylinders. Further research is required to determine the function of bCPAP and other non-invasive ventilator support methods in an effective healthcare program for seriously sick children with MOF at the tertiary and district hospital levels in low-resource economies (76). Branson in 2018 stated that patients with COPD and severe resting hypoxemia were shown to have a greater chance of survival when receiving long-term oxygen treatment (LTOT) at residence. It has already been demonstrated that oxygen supplementation administered by an oxygen concentrator during physical activity and exercise reduces symptoms and maintains arterial oxygen saturation, but it does not enhance long-term effectiveness (77). Melani et al. (78) reported the importance of oxygen-producing devices (O2 concentrators) in home oxygen therapy. Besides this, they also explained the role of a pulse oximeter, etc., in-home oxygen therapy (78).
Pulsed oxygen delivery through portable oxygen concentrators against continuous flow oxygen delivery was compared in an in-vitro-in silico research by Chen et al. in 2019. The effectiveness of four POCs with continuous flow oxygen was compared using in vitro bench measurements and in silico numerical simulations by anticipating the FIO2 at the trachea and entering the acini. In respect of volume-averaged FIO2, continuous flow oxygen provided adequate (>2% absolute) oxygen for all nominally equivalent pulse flow levels of >2 (79). Bench-wise comparative research between modern portable oxygen concentrators and various breathing techniques was conducted by Martin et al. in 2019. Considering three realistic breathing patterns, resting, and oronasal inhalation while sleeping, three modern devices were bench-tested (80). In Madrid, Spain, patients undergoing portable oxygen therapy were the subject of a telephone-based study conducted by Alises et al. in 2019. The survey looked at patients’ present situations, behaviors, and perceptions.
Patients selected from a registry undergoing treatment with portable oxygen were interviewed over the phone. Most patients used mobile oxygen concentrators (99.59 percent) (81). According to Litch and Bishop (82), using oxygen concentrators to give medical oxygen in isolated, high-altitude environments significantly impacts supply and related costs. In a rural hospital located at 3,900 m in the Nepal Himalayas, researchers highlighted the significance of oxygen concentrators. The investigators also stated that oxygen concentrations of more than 80% might be sustained at delivery flow rates of 2–5 L per minute (82). According to the findings of the research conducted by Sardesai et al. (83), portable oxygen concentrators are recommended to be utilized for home-isolated COVID patients. This is particularly the case for patients who do not exhibit severe symptoms and for whom there is no requirement for BIPAP. Through the follow-up with COVID-19 patients undergoing home oxygen therapy, feedback was obtained (83).
According to Shen in 2020, oxygen therapy has the potential to improve the antiviral response of the immune system. Treatment with nighttime oxygen may stop the progression of COVID-19. SARS-CoV2 is known to use ACE2, which is the same cell invasion receptor as SARS-CoV2. It is secure and simple to apply in therapeutic settings (a home O2 concentrator is enough for a patient) (84). According to Duke et al. in 2020, oxygen therapy is a crucial medical treatment and a critical part of functional hospital setups. They emphasized the application of oxygen concentrators in especially low-income countries, where medical facilities are not so well developed. So, it is best suited for African and Asia Pacific countries, which lack better medical infrastructure (85).
Cuerpo et al. (86) suggested the use of oxygen concentrators along with the noninvasive ventilation device in interstitial lung disease patients, which can improve home oxygen therapy. They employed both oxygen concentrators alone and in combination with non-invasive ventilation, and they discovered that the average SaO2 increased to 91% (88–93) versus 88% (86–90%); p = 0.0005 as well as a decline in the measure of time with oxygen saturation below 90%: 36% (6–56%) versus 58% (36–77%); p 0.0001 (86). In Sabah, Malaysia, during the COVID-19 pandemic, Cheah et al. (87), recommended using a dual oxygen concentrator system for manual breathing. According to the investigators, oxygen concentrators are a life-saving alternative for patients, and they could even save a severely ill patient during COVID (87). For use in medical applications, Vemula and Matthew in 2021 designed an experimental “Snap-on” and an independent single-bed oxygen concentrator. For continuous oxygen supply, a unique single-bed, “Snap-on,” and independent medical oxygen concentrator design based on a rapid pressure swing adsorption technique was explored. When connected to a preexisting compressed gas stream, the Snap-on concentrator’s configuration makes it simple to use the devices and efficiently produce oxygen for medical uses. Because of its separate compressor and ease of portability, the Snap-on concentrator is extremely significant for oxygen therapy, meeting the demands of a higher number of patients in the circumstances like COVID-19 (56).
In 38 remote health institutions in nine regions of Papua New Guinea, Pulsan et al. (88) examined a scheme for enhancing credible oxygen therapy using oxygen concentrators, pulse oximeters, and renewable solar power. In rural and remote hospitals and healthcare institutions, solar-powered oxygen systems can be installed on a broad scale and have been linked to a decrease in child fatalities and a reduction in referrals (88). According to Madaan et al. (89), rural India’s oxygen needs should focus on employing a supply of substantially pure oxygen that is secure, affordable, simple to access, and mass-producible. The arrangement of a self-sustaining oxygen concentrator (pressure swing adsorption with multiple molecular sieve technology) that provide oxygen at high flow rates through a centrally controlled distribution network to 100 or even more bedded community hospitals, with backup from an oxygen bank of 10 × 10 cylinders, may be able to achieve this. It could also act as a facility for replenishing local oxygen cylinders for medical emergencies inside the hospital premises and for delivery to ambulances, primary health centers, and other remote locations (89).
13. Types of zeolites used in oxygen concentrator
Zeolites are micron-sized, crystalline, porous compounds made up of alumina and silica hydrates along with an alkali metal (90). Its structure has numerous pores, making it suitable as an adsorbent. It is widely used in the oxygen concentrator due to its ability to withstand higher temperatures and its tendency to regenerate completely aster adsorption (51). Zeolite molecular sieves for oxygen concentrators come in two primary varieties: sodium type and lithium type (91). The oxygen concentrator is compact and easy to transport because lithium-type zeolite molecular sieves are much more effective than sodium-type zeolite molecular sieves that decreases the amounts of oxygen generation. Since sodium oxygen molecular sieve is considerably less expensive than lithium molecular sieve, sodium oxygen molecular sieve is also readily available in markets.
All the above factors make oxygen concentrators more economical and environmentally friendly (51). Recently Sami et al. (51) studied various types of zeolites used in oxygen concentrators and concluded that there are mainly four types of zeolites used as adsorbents. These four types of zeolites are 5A zeolite, Oxysiv 5, 7 & Sylobead MS S 624, LiX zeolite, and LiLSX zeolite. As per their investigation, it was concluded that various absorbents in oxygen concentrators have different cycles and purity of oxygen produced by them (51). The properties of these four commonly used zeolites are summarized in Table 3.
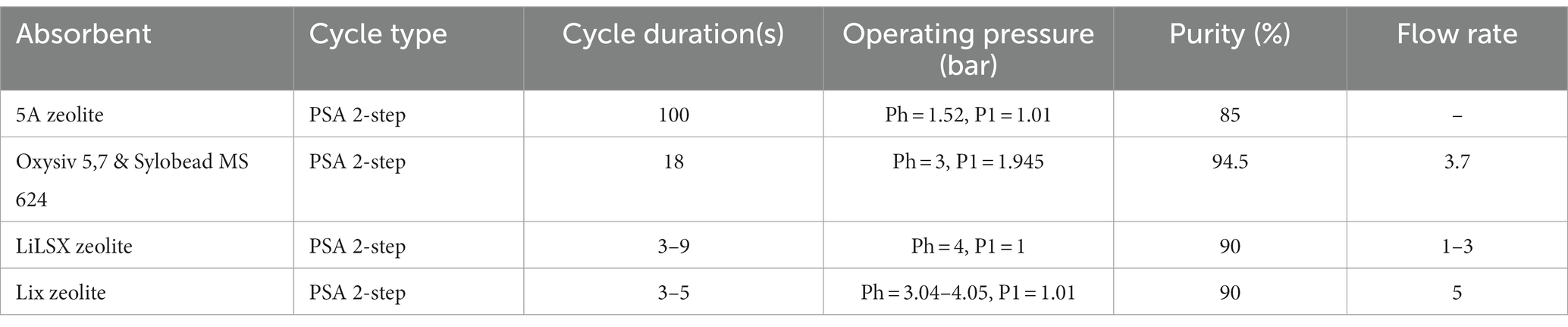
Table 3. Literature survey on zeolites used for oxygen concentrators adopted from Pan et al. in 2017 and Semi et al. in 2022 (92).
14. Drawbacks of micron-sized molecular sieves
Since the size of the currently used molecular sieves is in microns, less nitrogen could be adsorbed on their surface due to size. Consequently, the efficiency of oxygen generation, as well as the purity of oxygen, are both less. So, to overcome this, ultrafine zeolites have to be used to enhance the efficiency and purity of the oxygen. Zeolites are widely used in oxygen concentrators to adsorb the nitrogen on their surface. This process takes place under pressure swing adsorption technology, which concentrates oxygen from the air. The zeolites have more affinity for nitrogen and less affinity for oxygen. Since most oxygen concentrators use micron-sized molecular sieves to adsorb the nitrogen, their efficiency is not 100%; rather, it falls to 87–93%, depending on several other factors (92).
15. Role of nanotechnology in oxygen concentrators
There are several procedures by which the efficiency of such oxygen concentrators and the purity of oxygen can be achieved. One such process is the application of nanozeolites, which are used for this purpose since they are smaller in size and contain more reactive sites on their surface (93). Nanozeolites are a type of zeolites which are produced from the molecular zeolites and these have particle distribution and sizes of <200 nm. Due to their small size, these nanozeolites exhibit significant surface–to–volume ratios (SVR) and large surface values. More nitrogen will be adsorbed on the surface of nanozeolites compared to micron-sized zeolites because of the high surface area-to-volume ratio (94–96).
Some investigators have used nanozeolites in oxygen concentrators for nitrogen adsorption and obtained satisfactory results. Some of the examples are provided below in chronological order. In a continuous adsorption and desorption cycle with a cycle period of 90 s, Pan et al. (92), employed nanozeolites as molecular sieves in which the outcome of the oxygen concentrator is a flow of enriched oxygen at about 90 vol percent. The adsorption column measures 20 cm in length and 3 cm in diameter. To effectively adsorb nitrogen from the air, a 13X nanosize zeolite with Li+ exchanged is utilized as the adsorbent. To better understand the pressurization and depressurization processes taking place inside the microporous region of nanosized zeolites, a simulation of the pressure and vacuum swing adsorption units was devised (92). Besides this, several investigators have also reported the surface modification of such nanozeolites by other metallic particles to make the process more efficient (1, 97–99).
In this regard, chabazite (CHA) zeolites were manufactured at different range diameters of 120–300 nm, whereas the smaller sample (120 nm) also consisted of tiny particles (around 20 nm) (100). These small units of the CHA zeolites are basically used along with zeolites for gas separation technologies. The design and development of such nano-sized zeolite-based specimens are highly appreciated for constructing vacuum swing adsorption devices due to their thermal stability and compressive stress. Moreover, the hybrid CHA zeolites are prepared by doping a few desired ions, such as Sr2+, K+, and Na+ cations, by avoiding organic substances (100). The as-prepared membranes are useful to form a chemically stable system as the cation species interact well with the negatively occurring aluminum oxygen sites (AlO2)− in a tetrahedral geometry (101). Nowadays, various models have been explored to design and develop zeolite-based oxygen concentrators. For example, a molecular sieve with particular model numbers and activated alumina in PSA oxygen generators.
The higher production of O2 includes a larger amount of nitrogen adsorption. Additionally, the selectivity in N2 and O2 (nitrogen/oxygen) allows it to be called with different model names, such as the JLOX-500 series molecular sieve. Using nanotechnologies, these systems show better wear resistance and good service rate as the pore sizes, surface, and structural modifications are carried out under preparation procedures (102). The use of nano-based techniques felicitates a cost-effective oxygen production with lower energy consumption by using the nano-sized zeolites and their derivatives using metal cations and organic units. Thus, the chemical structure of the zeolite is extensively recommended for the efficient oxygen concentrator due to its chemical and physical strength, including its (SiO4)4+ and (AlO4)5+ tetrahedral geometry sharing the partially negatively charged oxygen atoms. Extensively, the isotherm concept has been derived from understanding the isotherm adsorption of the zeolites using Langmuir, Freundlich, and Tempkin model’s theorem, and the characteristics of adsorption application are being studied (103).
Pseudo-first order, pseudo-second order, Bangham, intra-particle diffusion, and Elovich models were used to assess the kinetics of the adsorption objects, demonstrating the feasibility of the applicability of gaseous adsorption (103). As oxygen concentrators basically include gas compressors and adsorption sciences, nano-sized zeolites have been encouraged in this sector. Overall, stronger structural aspects with the change in cationic oxidation states help to modify the framework of the zeolite, which affects the adsorption nature and separates the gases, mainly O2 and N2/O2. These physicochemical properties of the synthetic zeolite with their morphological changes could be widely used in modern nanotechnology and sciences toward newly designed oxygen concentrators/systems and their accessories (104–106). Overal evolution in use of molecular zeolites to nano-zeolites has gained importance to tackle the O2 supply chain issue that had been severely felt in medical care during the pandemics in developing countries such as India and similar countries (107–110).
16. Conclusion
Oxygen is one of the basic and essential medical drugs required by patients in less to highly severe cases. The demand and supply of mobile oxygen generators have increased drastically in the last couple of years. So, portable oxygen concentrators, initially proven life-supportive in hilly areas in African countries, have now gained importance. Its economical, mobile natures, and ease of handling, have made basic needs in every house. The utilization of four basic types of zeolites as an adsorbent has limitations in the sense of generation, purity of oxygen, etc., and efficiency being micron in size. So, the nanosized zeolites can potentially make the process of adsorption and desorption in oxygen concentrators more effective due to their small size and high surface area-to-volume ratio. Nanosized zeolites have the potential to be a game-changer in the delivery of pure oxygen to the patient in the face of current and future pandemics caused by a wide range of viruses and bacteria.
Author contributions
VY, BP, and DKS: conceptualization, data curation, formal analysis, funding acquisition, investigation, methodology, project administration, resources, software, supervision, validation, visualization, roles and writing – original draft, and writing – review and editing. NC, GI, AR, BiS, and BhS: concept, writing – original draft, writing – review and editing, and revision. All authors contributed to the article and approved the submitted version.
Acknowledgments
The authors are thankful for proving basic infrastructure facilities by Mody University of Science and Technology, Sikar, Rajasthan, India.
Conflict of interest
The authors declare that the research was conducted in the absence of any commercial or financial relationships that could be construed as a potential conflict of interest.
Publisher’s note
All claims expressed in this article are solely those of the authors and do not necessarily represent those of their affiliated organizations, or those of the publisher, the editors and the reviewers. Any product that may be evaluated in this article, or claim that may be made by its manufacturer, is not guaranteed or endorsed by the publisher.
References
1. Williams, E, Piaggio, D, Andellini, M, and Pecchia, L. 3D-printed activated charcoal inlet filters for oxygen concentrators: a circular economy approach. Dev Eng. (2022) 7:100094. doi: 10.1016/j.deveng.2022.100094
2. Urich, MD, Vemula, RR, and Kothare, MV. Implementation of an embedded model predictive controller for a novel medical oxygen concentrator. Comput Chem Eng. (2022) 160:107706. doi: 10.1016/j.compchemeng.2022.107706
3. Dhont, J, di Tella, M, Dubois, L, Aznar, M, Petit, S, Spałek, M, et al. Conducting research in radiation oncology remotely during the COVID-19 pandemic: coping with isolation. Clin Transl Radiat Oncol. (2020) 24:53–9. doi: 10.1016/j.ctro.2020.06.006
4. Sarkar, M, Niranjan, N, and Banyal, PK. Mechanisms of hypoxemia. Lung India. (2017) 34:47. doi: 10.4103/0970-2113.197116
5. Basnet, S, Adhikari, RK, and Gurung, CK. Hypoxemia in children with pneumonia and its clinical predictors. Indian J Pediatr. (2006) 73:777–81. doi: 10.1007/BF02790384
6. Chen, YL, Chen, YC, Wang, HT, Chang, YT, Fang, YN, Hsueh, S, et al. The impact of intermittent hypoxemia on left atrial remodeling in patients with obstructive sleep apnea syndrome. Life. (2022) 12:12. doi: 10.3390/life12020148
7. Hirota, K, Mayahara, T, Fujii, Y, and Nishi, K. Asymptomatic hypoxemia as a characteristic symptom of coronavirus disease: a narrative review of its pathophysiology. COVID. (2022) 2:47–61. doi: 10.3390/covid2010004
8. Chiumello, D, and Brioni, M. Severe hypoxemia: which strategy to choose. Crit Care. (2016) 20:132. doi: 10.1186/s13054-016-1304-7
9. Cheng, S, Luo, Y, Zhang, J, Shi, R, Wei, S, Dong, K, et al. The highly effective therapy of ovarian cancer by bismuth-doped oxygen-deficient BaTiO3 with enhanced sono-piezocatalytic effects. Chem Eng J. (2022) 442:136380. doi: 10.1016/j.cej.2022.136380
10. Nakamura, M, and Noguchi, K. Tolerant mechanisms to O2 deficiency under submergence conditions in plants. J Plant Res. (2020) 133:343–71. doi: 10.1007/s10265-020-01176-1
11. Tiep, B, Carter, R, Zachariah, F, Williams, AC, Horak, D, Barnett, M, et al. Oxygen for end-of-life lung cancer care: managing dyspnea and hypoxemia. Expert Rev Respir Med. (2013) 7:479–90. doi: 10.1586/17476348.2013.816565
12. Gardner, B. The manufacture, storage and supply of medical gases. S Afr Fam Pract. (2013) 55:S40–3. doi: 10.1080/20786204.2013.10874363
13. Chen, Y, Wang, Q, Lei, J, Liu, Q, He, W, Liu, S, et al. Design and engineering application of medical oxygen supply system in novel coronavirus pneumonia treatment hospital. J Build Eng. (2021) 44:103170. doi: 10.1016/j.jobe.2021.103170
14. Wood, MH, Hailwood, M, and Koutelos, K. Reducing the risk of oxygen-related fires and explosions in hospitals treating Covid-19 patients. Process Saf Environ Prot. (2021) 153:278–88. doi: 10.1016/j.psep.2021.06.023
15. Singh, S, and Sharma, BB. Severe acute respiratory syndrome-coronavirus 2 and novel coronavirus disease 2019: an extraordinary pandemic. Lung India. (2020) 37:268–71. doi: 10.4103/lungindia.lungindia_170_20
16. Hardavella, G, Karampinis, I, Frille, A, Sreter, K, and Rousalova, I. Oxygen devices and delivery systems, Oxygen devices and delivery systems. Breathe. (2019) 15:e108–16. doi: 10.1183/20734735.0204-2019
18. Bakare, AA, Graham, H, Ayede, AI, Peel, D, Olatinwo, O, Oyewole, OB, et al. Providing oxygen to children and newborns: a multi-faceted technical and clinical assessment of oxygen access and oxygen use in secondary-level hospitals in Southwest Nigeria. Int Health. (2019) 12:60–8. doi: 10.1093/inthealth/ihz009
19. Shiao, SY, and Ou, CN. Validation of oxygen saturation monitoring in neonates. Am J Crit Care. (2007) 16:168–78.
20. Myers, S, Dinga, P, Anderson, M, Schubert, C, Mlotha, R, Phiri, A, et al. Use of bubble continuous positive airway pressure (bCPAP) in the management of critically ill children in a Malawian paediatric unit: an observational study. BMJ Open Respir Res. (2019) 6:e000280. doi: 10.1136/bmjresp-2018-000280
21. Pan, M, Omar, HM, and Rohani, S. Application of nanosize zeolite molecular sieves for medical oxygen concentration. Nano. (2017) 7:7. doi: 10.3390/nano7080195
22. Duke, T, Pulsan, F, Panauwe, D, Hwaihwanje, I, Sa'avu, M, Kaupa, M, et al. Solar-powered oxygen, quality improvement and child pneumonia deaths: a large-scale effectiveness study. Arch Dis Child. (2021) 106:224–30. doi: 10.1136/archdischild-2020-320107
23. Singh, A, and Shivapuji, A. S3 medical oxygen generator design document for 3 Nm3/h medical oxygen generation system medical grade oxygen generation view project DSTRHEES view project. (India: Indian Institute of Science) (2021). doi: 10.13140/RG.2.2.10172.56969
24. Priority medical devices list for the COVID-19 response and associated technical specifications. (2020).
25. Ackley, MW. Medical oxygen concentrators: a review of progress in air separation technology. Adsorption. (2019) 25:1437–74. doi: 10.1007/s10450-019-00155-w
26. PIB Mumbai. Liquid Medical Oxygen: Explainer. Ministry of Health and Family Welfare (2021). Available at: https://pib.gov.in/PressReleasePage.aspx?PRID=1716197 [Accessed on April 14, 2023]
27. Sarkar, S, Paswan, A, and Prakas, S. Liquid ventilation. Anesth Essays Res. (2014) 8:277–82. doi: 10.4103/0259-1162.143109
28. Martin, DS, and Grocott, MPW. Oxygen therapy in critical illness: precise control of arterial oxygenation and permissive hypoxemia*. Crit Care Med. (2013) 41:423–32. doi: 10.1097/CCM.0b013e31826a44f6
29. Okba, NMA, Müller, M, Li, W, Wang, C, GeurtsvanKessel, C, Corman, V, et al. Severe acute respiratory syndrome coronavirus 2−specific antibody responses in coronavirus disease patients. Emerg Infect Dis J. (2020) 26:1478–88. doi: 10.3201/eid2607.200841
30. Fairley, HB. Oxygen therapy for surgical patients. Am Rev Respir Dis. (1980) 122:37–44. doi: 10.1164/arrd.1980.122.5P2.37
31. Reiter, J, Gileles-Hillel, A, Cohen-Cymberknoh, M, Rosen, D, Kerem, E, Gozal, D, et al. Sleep disorders in cystic fibrosis: a systematic review and meta-analysis. Sleep Med Rev. (2020) 51:101279. doi: 10.1016/j.smrv.2020.101279
32. Jiang, B, and Wei, H. Oxygen therapy strategies and techniques to treat hypoxia in COVID-19 patients. Eur Rev Med Pharmacol Sci. (2020) 24:10239–10246. doi: 10.26355/eurrev_202010_23248
33. Allardet-Servent, J, Sicard, G, Metz, V, and Chiche, L. Benefits and risks of oxygen therapy during acute medical illness: just a matter of dose! Rev Med Interne. (2019) 40:670–6. doi: 10.1016/j.revmed.2019.04.003
34. Baldwin, J, and Cox, J. Treating dyspnea: is oxygen therapy the best option for all patients? Med Clin N Am. (2016) 100:1123–30. doi: 10.1016/j.mcna.2016.04.018
35. Rebecca, M. Pulse oximetry: what the nurse needs to know. Nurs Stand. (2017) 31:42–5. doi: 10.7748/ns.2017.e9940
36. Walsh, BK, Brooks, TM, and Grenier, BM. Oxygen therapy in the neonatal care environment. Respir Care. (2009) 54:1193–202.
37. Dennery, PA. Oxygen administration in the care of neonates: a double-edged sword. Chin Med J. (2010) 123:2938–42. doi: 10.3760/cma.j.issn.0366-6999.2010.20.034
38. Ramji, S, Saugstad, OD, and Jain, A. Current concepts of oxygen therapy in neonates. Indian J Pediatr. (2015) 82:46–52. doi: 10.1007/s12098-014-1571-8
39. Karki, S, Fitzpatrick, AL, and Shrestha, S. Risk factors for pneumonia in children under 5 years in a teaching Hospital in Nepal. Kathmandu Univ Med J. (2015) 12:247–52. doi: 10.3126/kumj.v12i4.13729
40. Das, CS. Acute respiratory ailments in pediatric age group and role of CRP in diagnosis and management In: W Ansar and S Ghosh, editors. Clinical significance of C-reactive protein. Singapore: Springer Singapore (2020). 213–48.
41. Keith Grimwood, ABC. Long term effects of pneumonia in young children. Pneumonia. (2015) 6:101–14. doi: 10.15172/pneu.2015.6/621
42. Shan, C, Yao, YF, Yang, X, Zhou, YW, Gao, G, Peng, Y, et al. Infection with novel coronavirus (SARS-CoV-2) causes pneumonia in rhesus macaques. Cell Res. (2020) 30:670–7. doi: 10.1038/s41422-020-0364-z
43. Dimich, I, Singh, PP, Adell, A, Hendler, M, Sonnenklar, N, and Jhaveri, M Evaluation of oxygen saturation monitoring by pulse oximetry in neonates in the delivery system. Can J Anaesth. (1991) 38:985–8. doi: 10.1007/BF03008616
44. Rauniyar, NK, Pujari, S, and Shrestha, P. Study of oxygen saturation by pulse oximetry and arterial blood gas in ICU patients: a descriptive cross-sectional study. J Nepal Med Assoc. (2020) 58:789–93. doi: 10.31729/jnma.5536
45. Ross, PA, Newth, CJL, and Khemani, RG. Accuracy of pulse Oximetry in children. Pediatrics. (2014) 133:22–9. doi: 10.1542/peds.2013-1760
46. Little, CM, Merritt, M, and Wentworth, A. An improvised oxygen supply system for pandemic and disaster use. Acad Emerg Med. (2009) 16:558–63. doi: 10.1111/j.1553-2712.2009.00400.x
47. Todur, P, Chaudhuri, S, Eeshwar, M, Teckchandani, D, and Venkateswaran, R. Oxygen sources and delivery devices: essentials during COVID-19. Indian J Respir Care. (2021) 10:171. doi: 10.4103/ijrc.ijrc_63_21
48. Celli, BR, and Zuwallack, RL. 105 – pulmonary rehabilitation In: VC Broaddus, RJ Mason, JD Ernst, TE King, SC Lazarus, and JF Murray, et al., editors. Murray and Nadel’s textbook of respiratory medicine. 6th ed. Philadelphia: W.B. Saunders (2016). 1821–1831.e3.
49. Flecknell, P. Chapter 1 – basic principles of Anaesthesia In: P Flecknell, editor. Laboratory animal Anaesthesia. 4th ed. Boston: Academic Press (2016). 1–75.
51. Sami, A, Irfan, M, Uddin, R, Ali, AH, Khan, H, Khan, E, et al. Oxygen concentrator design: zeolite based pressure swing adsorption In: IEEC 2022. Basel Switzerland: MDPI (2022). 26.
52. Zhu, X, Liu, Y, Yang, X, and Liu, W. Study of a novel rapid vacuum pressure swing adsorption process with intermediate gas pressurization for producing oxygen. Adsorption. (2017) 23:175–84. doi: 10.1007/s10450-016-9843-4
53. Ackley, MW. Medical oxygen concentrators: a review of progress in air separation technology. Adsorption. (2019) 25:1437–74. doi: 10.1007/s10450-019-00155-w
54. Stoppa, F, Scordari, F, Mesto, E, Sharygin, V, and Bortolozzi, G. Calcium-aluminum-silicate-hydrate “cement” phases and rare Ca-zeolite association at Colle Fabbri, Central Italy. Central Eur J Geosci. (2010) 2:175–87. doi: 10.2478/v10085-010-0007-6
55. Hamed, HH. Oxygen separation from air using zeolite type 5A. Al-Qadisiyah Journal for Engineering Sciences. (2015) 8:147–158.
56. Vemula, RR, Urich, MD, and Kothare, MV. Experimental design of a “snap-on” and standalone single-bed oxygen concentrator for medical applications. Adsorption. (2021) 27:619–28. doi: 10.1007/s10450-021-00299-8
57. Blakeman, TC, Rodriquez, D, Britton, TJ, Johannigman, JA, Petro, MC, and Branson, RD. Evaluation of oxygen concentrators and chemical oxygen generators at altitude and temperature extremes. Mil Med. (2016) 181:160–8. doi: 10.7205/MILMED-D-15-00130
58. Arora, A, and Hasan, MMF. Flexible oxygen concentrators for medical applications. Sci Rep. (2021) 11:14317 doi: 10.1038/s41598-021-93796-3
59. Gurkin, VN, Kagramanov, GG, Loiko, A, Farnosova, EN, Blanko-Pedrekhon, AM, and Milyaev, AV. Development of a portable membrane oxygen concentrator. Membr Membr Technol. (2021) 3:186–91. doi: 10.1134/S2517751621030045
60. Gould, GA, Scott, W, Hayhurst, MD, and Flenley, DC. Technical and clinical assessment of oxygen concentrators. Thorax. (1985) 40:811–6. doi: 10.1136/thx.40.11.811
61. Ackley, MW. Medical oxygen concentrators: a review of progress in air separation technology. Adsorption. (2019) 25:1437–74. doi: 10.1007/s10450-019-00155-w
62. Evans, TW, Waterhouse, J, and Howard, P. Clinical experience with the oxygen concentrator. Br Med J (Clin Res Ed). (1983) 287:459–61. doi: 10.1136/bmj.287.6390.459
63. Hardavella, G, Karampinis, I, Frille, A, Sreter, K, and Rousalova, I. Oxygen devices and delivery systems. Breathe. (2019) 15:e108:–e116. doi: 10.1183/20734735.0204-2019
64. Dakkak, J, Tang, W, Smith, JT, Balasubramanian, A, Mattson, M, Ainechi, A, et al. Burden and unmet needs with portable oxygen in patients on long-term oxygen therapy. Ann Am Thorac Soc. (2021) 18:1498–505. doi: 10.1513/AnnalsATS.202005-487OC
65. Möller, S, Ivarsson, B, Nordström, LÅ, and Johansson, A. Ltot patients’ experience of a portable oxygen unit and health-related quality of life—a cross-sectional study. Healthcare (Switzerland). (2020) 8:8. doi: 10.3390/healthcare8020182
66. Matito-Martos, I, García-Reyes, J, Martin-Calvo, A, Dubbeldam, D, and Calero, S. Improving ammonia production using zeolites. J Phys Chem C. (2019) 123:18475–81. doi: 10.1021/acs.jpcc.9b05366
67. Rodriquez, DJ, Blakeman, TC, Dorlac, W, Johannigman, JA, and Branson, RD. Maximizing oxygen delivery during mechanical ventilation with a portable oxygen concentrator. J Trauma Acute Care Surg. (2010) 69:S87–93. doi: 10.1097/TA.0b013e3181e44b27
68. Rees, PJ, and Dudley, F. Provision of oxygen at home. BMJ. (1998) 317:935–8. doi: 10.1136/bmj.317.7163.935
69. Ritz, RH, and Previtera, JE. Oxygen Supplies During a Mass Casualty Situation. Respir Care. (2008) 53:215–24.
70. Pollock, NW, and Natoli, MJ. Chemical oxygen generation: evaluation of the green dot systems, inc portable, nonpressurized emOx device. Wilderness Environ Med. (2010) 21:244–9. doi: 10.1016/j.wem.2010.04.008
71. McCoy, RW. Options for home oxygen therapy equipment: storage and metering of oxygen in the home. Respir Care. (2013) 58:65–85. doi: 10.4187/respcare.01932
72. Duke, T, Peel, D, Graham, S, Howie, S, Enarson, PM, and Jacobson, R. Oxygen concentrators: a practical guide for clinicians and technicians in developing countries. Ann Trop Paediatr. (2010) 30:87–101. doi: 10.1179/146532810X12637745452356
73. Yáñez, AM, Prat, JP, Álvarez-Sala, JL, Calle, M, Díaz Lobato, S, Gonzalez, JLG, et al. Oxygenation with a single portable pulse-dose oxygen-conserving device and combined stationary and portable oxygen delivery devices in subjects with COPD. Respir Care. (2015) 60:382–7. doi: 10.4187/respcare.03470
74. Katz, I, Pichelin, M, Montesantos, S, Kang, MY, Sapoval, B, Zhu, K, et al. An in silico analysis of oxygen uptake of a mild COPD patient during rest and exercise using a portable oxygen concentrator. Int J COPD. (2016) 11:2427–34. doi: 10.2147/COPD.S112473
75. Chen, JZ, Katz, IM, Pichelin, M, Zhu, K, Caillibotte, G, Noga, ML, et al. Comparison of pulsed versus continuous oxygen delivery using realistic adult nasal airway replicas. Int J COPD. (2017) 12:2559–71. doi: 10.2147/COPD.S141976
76. Myers, S, Dinga, P, Anderson, M, Schubert, C, Mlotha, R, Phiri, A, et al. Use of bubble continuous positive airway pressure (bCPAP) in the management of critically ill children in a Malawian paediatric unit: an observational study. BMJ Open Respir Res. (2019) 6:e000280. doi: 10.1136/bmjresp-2018-000280
78. Melani, AS, Sestini, P, and Rottoli, P. Home oxygen therapy: re-thinking the role of devices. Expert Rev Clin Pharmacol. (2018) 11:279–89. doi: 10.1080/17512433.2018.1421457
79. Chen, JZ, Katz, IM, Pichelin, M, Zhu, K, Caillibotte, G, Finlay, WH, et al. In vitro–in silico comparison of pulsed oxygen delivery from portable oxygen concentrators versus continuous flow oxygen delivery. Respir Care. (2019) 64:117–29. doi: 10.4187/respcare.06359
80. Martin, DC. Contemporary portable oxygen concentrators and diverse breathing behaviours-a bench comparison. BMC Pulm Med. (2019) 19:19. doi: 10.1186/s12890-019-0980-x
81. Alises, SM, Caneiras, C, and Díaz-Lobato, S. A telephone-based survey of current trends, habits and beliefs in patients receiving portable oxygen therapy in Madrid, Spain. ERJ Open Res. (2019) 5:5. doi: 10.1183/23120541.00059-2018
82. Litch, JA, and Bishop, RA. Oxygen concentrators for the delivery of supplemental oxygen in remote high-altitude areas. Wilderness Environ Med. (2000) 11:189–91. doi: 10.1580/1080-6032(2000)011[0189:OCFTDO].2.3.CO;2
83. Sardesai, I, Grover, J, Garg, M, Nanayakkara, PB, di Somma, S, Paladino, L, et al. Short term home oxygen therapy COVID-19 patients: the COVID-HOT algorithm. J Fam Med Primary Care. (2020) 9:3209–19. doi: 10.4103/jfmpc.jfmpc_1044_20
84. Shen, C, Yue, X, Wang, J, Shi, C, and Li, W. Nocturnal oxygen therapy as an option for early COVID-19. Int J Infect Dis. (2020) 98:176–9. doi: 10.1016/j.ijid.2020.06.080
85. Leonard-Duke, J, Evans, S, Hannan, RT, Barker, TH, Bates, JHT, Bonham, CA, et al. Multi-scale models of lung fibrosis. Matrix Biol. (2020) 91-92:35–50. doi: 10.1016/j.matbio.2020.04.003
86. Cuerpo, S, Palomo, M, Hernández-González, F, Francesqui, J, Albacar, N, Hernández, C, et al. Improving home oxygen therapy in patients with interstitial lung diseases: application of a noninvasive ventilation device. Ther Adv Respir Dis. (2020) 14:175346662096302. doi: 10.1177/1753466620963027
87. Cheah, PK, Steven, EM, Ng, KK, Hashim, MI, Abdul Kadir, MH, and Roder, NP. The use of dual oxygen concentrator system for mechanical ventilation during COVID-19 pandemic in Sabah, Malaysia. Int J Emerg Med. (2021) 14:30. doi: 10.1186/s12245-021-00354-9
88. Pulsan, F, and Duke, T. Response to oxygen therapy using oxygen concentrators run off solar power in children with respiratory distress in remote primary health facilities in Papua New Guinea. Trop Dr. (2021) 51:15–9. doi: 10.1177/0049475520947886
89. Madaan, N, Paul, BC, and Guleria, R. Meeting oxygen requirements of rural India: a self-contained solution. Indian J Public Health. (2021) 65:82–4. doi: 10.4103/ijph.IJPH_1405_20
90. Yadav, VK, Suriyaprabha, R, Inwati, GK, Gupta, N, Singh, B, Lal, C, et al. A noble and economical method for the synthesis of low cost zeolites from coal fly ash waste. Adv Mater Process Technol. (2021) 8:301–319. doi: 10.1080/2374068X.2021.1927640
91. Kianfar, E. Zeolites: properties, applications, modification and selectivity. Advances in Research and Applications. ed. A. Mahler (NY, USA: Nova Science Publishers, Inc.) (2020) 1–29.
92. Pan, M, Omar, HM, and Rohani, S. Application of nanosize zeolite molecular sieves for medical oxygen concentration. Nano. (2017) 7:7. doi: 10.3390/nano7080195
93. Modi, S, Inwati, GK, Gacem, A, Saquib Abullais, S, Prajapati, R, Yadav, VK, et al. Nanostructured antibiotics and their emerging medicinal applications: an overview of nanoantibiotics. Antibiotics. (2022) 11:708. doi: 10.3390/antibiotics11060708
94. Yadav, VK, Choudhary, N, Khan, SH, Malik, P, Inwati, GK, Suriyaprabha, R, et al. “Synthesis and characterisation of nano-biosorbents and their applications for waste water treatment,” in Handbook of Research on Emerging Developments and Environmental Impacts of Ecological Chemistry. (Gujurat, India: IGI Global Publisher) (2020), 252–290. doi: 10.4018/978-1-7998-1241-8
95. Gnanamoorthy, G, Yadav, VK, Yadav, KK, Ramar, K, Alam, J, Shukla, AK, et al. Fabrication of different SnO2 nanorods for enhanced photocatalytic degradation and antibacterial activity. Environ Sci Pollut Res. (2021):1–11. doi: 10.1007/s11356-021-13627-w
96. Gnanamoorthy, G, Ramar, K, Ali, D, Yadav, VK, and Kumar, G. Synthesis and effective performance of Photocatalytic and antimicrobial activities of Bauhinia tomentosa Linn plants using of gold nanoparticles. Opt Mater (Amst). (2022) 123:111945. doi: 10.1016/j.optmat.2021.111945
97. Malik, P, Inwati, GK, Mukherjee, TK, Singh, S, and Singh, M. Green silver nanoparticle and Tween-20 modulated pro-oxidant to antioxidant curcumin transformation in aqueous CTAB stabilized peanut oil emulsions. J Mol Liq. (2019) 291:111252. doi: 10.1016/j.molliq.2019.111252
98. Kumar Inwati, G, Kumar, P, Roos, WD, and Swart, HC. Thermally induced structural metamorphosis of ZnO:Rb nanostructures for antibacterial impacts. Colloids Surf B: Biointerfaces. (2020) 188:110821. doi: 10.1016/j.colsurfb.2020.110821
99. Inwati, GK, Kumar, P, Singh, M, Yadav, VK, Kumar, A, Soma, VR, et al. Study of photoluminescence and nonlinear optical behaviour of AgCu nanoparticles for nanophotonics. Nano-Struct Nano-Objects. (2021) 28:100807. doi: 10.1016/j.nanoso.2021.100807
100. Kim, MZ, Alam, SF, Arepalli, D, Rehman, AU, Choi, WY, and Cho, CH. Prevention in thermal crack formation in chabazite (CHA) zeolite membrane by developing thin top zeolite and thick intermediate layers. Nano. (2021) 12:11. doi: 10.3390/nano11082113
101. Yadav, VK, Singh, B, and Choudhary, N. Characterization of Indian incense stick powders for their physical, chemical and mineralogical properties. World J Environ Biosci. (2020) 9:39–43.
102. Inwati, GK, Yadav, VK, Ali, IH, Vuggili, SB, Kakodiya, SD, Solanki, MK, et al. 2D personality of multifunctional carbon nitrides towards enhanced catalytic performance in energy storage and remediation. Appl Sci (Switzerland). (2022) 12, 3753–3569. doi: 10.3390/app12083753
103. Ho, Y-S. Review of second-order models for adsorption systems. J Hazard Mater. (2006) 136:681–9. doi: 10.1016/j.jhazmat.2005.12.043
104. Inwati, GK, Kumar, P, Singh, M, Yadav, VK, Kumar, A, Soma, VR, et al. Study of photoluminescence and nonlinear optical behaviour of AgCu nanoparticles for nanophotonics. Nano-Structures Nano-Objects. (2021) 28:100807. doi: 10.1016/j.nanoso.2021.100807
105. Yadav, VK, Choudhary, N, Ali, D, Gnanamoorthy, G, Inwati, GK, Almarzoug, MHA, et al. Experimental and computational approaches for the structural study of novel Ca-rich zeolites from incense stick ash and their application for wastewater treatment. Adsorpt Sci Technol. (2021) 2021:1–12. doi: 10.1155/2021/6066906
106. Yadav, VK, Inwati, GK, Ali, D, Gnanamoorthy, G, Bera, SP, Khan, SH, et al. Remediation of azure a dye from aqueous solution by using surface-modified coal Fly ash extracted Ferrospheres by mineral acids and toxicity assessment. Adsorpt Sci Technol. (2022) 2022:1–14. doi: 10.1155/2022/7012889
107. Paital, B, Das, K, and Parida, SK. Inter nation social lockdown versus medical care against COVID-19, a mild environmental insight with special reference to India. Sci Total Environ. 728:138914. doi: 10.1016/j.scitotenv.2020.138914
108. Paital, B. Nurture to nature via COVID-19, a self-regenerating environmental strategy of environment in global context. Sci Total Environ. (2020) 729:139088. doi: 10.1016/j.scitotenv.2020.139088
109. Das, K, and Paital, B. First week of social lockdown versus medical care against COVID-19 – with special reference to India. Curr Trend Biotechnol Pharmacol. (2020) 14:196–216. doi: 10.5530/ctbp.2020.2.20
Keywords: chabazite, microbial infection, molecular sieves, nano-zeolite, oxygen therapy, pneumonia, respiratory disorders
Citation: Yadav VK, Choudhary N, Inwati GK, Rai A, Singh B, Solanki B, Paital B and Sahoo DK (2023) Recent trends in the nanozeolites-based oxygen concentrators and their application in respiratory disorders. Front. Med. 10:1147373. doi: 10.3389/fmed.2023.1147373
Edited by:
Jun Duan, First Affiliated Hospital of Chongqing Medical University, ChinaReviewed by:
Oksana B. Stoliar, Ternopil Volodymyr Hnatyuk National Pedagogical University, UkraineEdmund Pool, University of the Western Cape, South Africa
Copyright © 2023 Yadav, Choudhary, Inwati, Rai, Singh, Solanki, Paital and Sahoo. This is an open-access article distributed under the terms of the Creative Commons Attribution License (CC BY). The use, distribution or reproduction in other forums is permitted, provided the original author(s) and the copyright owner(s) are credited and that the original publication in this journal is cited, in accordance with accepted academic practice. No use, distribution or reproduction is permitted which does not comply with these terms.
*Correspondence: Virendra Kumar Yadav, yadava94@gmail.com; Biswaranjan Paital, biswaranjanpaital@gmail.com; Dipak Kumar Sahoo, dsahoo@iastate.edu; dipaksahoo11@gmail.com