- 1School of Medical Sciences, University of Campinas (UNICAMP), Campinas, Brazil
- 2Hematology and Hemotherapy Center, University of Campinas (UNICAMP), Campinas, Brazil
- 3Department of Pathology, School of Medical Sciences, University of Campinas (UNICAMP), Campinas, Brazil
Thrombotic primary antiphospholipid syndrome (t-PAPS) is an acquired condition characterized by heterogeneous thrombotic manifestations, which is intriguing since venous and arterial thrombosis appear to have distinct pathogenesis. Gene expression analysis may constitute a new approach to evaluate potential similarities or differences between the clinical manifestations of t-PAPS. Recently, dysregulation of the ANXA3, TNFAIP6, TXK, BACH2, and SERPINB2 genes has been associated with both arterial and venous thrombosis in the general population. Therefore, the aim of this study was to examine whether ANXA3, TNFAIP6, TXK, BACH2, and SERPINB2 expression was associated with t-PAPS. Gene expression was quantified by qPCR of total leukocyte mRNA. In this case-control study, 102 t-PAPS patients, 17 asymptomatic antiphospholipid (aPL) carriers and 100 controls were evaluated. Increased expression of ANXA3 (P = 0.008) and TNFAIP6 (P = 0.001) and decreased expression of the TXK gene (P = 0.0001) were associated with an increased risk of t-PAPS compared to the control. ANXA3 upregulation was more evident in cases of arterial thrombosis and multiple thrombotic events. There was no difference in the expression of these genes between triple and non-triple aPL positivity. ANXA3, TNFAIP6, TXK, BACH2, and SERPINB2 expression levels were also similar between aPL carriers and controls (P = 0.77; P = 0.48; P = 0.08; P = 0.73, and P = 0.13, respectively). In conclusion, our results showed that genes related to hemostasis (ANXA3) and immunity (TNFAIP6, TXK) are dysregulated in t-PAPS compared to controls. Gene dysregulation was not detected in aPL carriers and was not related to the aPL profile, suggesting that this gene signature is related to thrombotic manifestations rather than to aPL burden. Our results suggest that innate immunity and hemostasis pathways are associated with t-PAPS at a molecular level and may play a role in disease severity.
Introduction
Antiphospholipid syndrome (APS) is an acquired prothrombotic condition characterized by thrombosis or pregnancy complications (1) due to the presence of antiphospholipid antibodies (aPLs). These antibodies are directed against phospholipid-binding proteins, particularly beta2-glycoprotein I, found in cell membranes, including monocytes, platelets, and endothelial cells (2, 3). In primary APS (PAPS), there is no underlying systemic autoimmune disease.
The activation of platelets, monocytes, and endothelial cell membranes by aPLs leads to a hypercoagulable state (4), which is further enhanced by a secondary stimulus (second trigger), such as oral contraceptive use, infectious or inflammatory diseases, hypertension, and dyslipidemia (4, 5), resulting in thrombotic events. PAPS-associated thrombosis (t-PAPS) is one of the few thrombotic disorders that occurs indistinctly in veins, arteries or capillaries, affecting different organs and tissues (6).
Although t-PAPS is described as a single disease, arterial and venous complications differ in pathology, clinical course, treatment and prognosis, suggesting that different mechanisms underlie these thrombotic complications. Recently, studies aimed at evaluating the vascular impairment of t-PAPS have shown that aPLs can induce changes in the expression of genes associated with procoagulant and proinflammatory markers, leading to a prothrombotic state (7). Therefore, gene expression analysis may be a new approach to study the etiology of thrombosis in APS.
Recently, the profile of genes associated with venous and arterial thrombosis in the general population (not associated with APS) was evaluated by meta-analysis (8). In this study, five databases were analyzed and gene expression levels in whole blood from patients with venous thrombosis or arterial cardiovascular disease were compared with gene expression levels in controls. A total of 124 genes showed differential expression levels between these diseases. A further 473 genes had altered expression in the same direction in venous and arterial disease (168 upregulated and 305 downregulated). Of these, six genes were most strongly associated with both venous and arterial thrombosis: G0S2, BCL2A1, TNFAIP6 (upregulated), CLIC3, BACH2, and TXK (downregulated). The results of the meta-analysis suggest that a specific gene profile may be associated with the occurrence of both arterial and venous thrombosis in patients without APS.
Comparing the results of the meta-analysis described above (8) with the results of previous APS studies (9, 10), we found that the genes ANXA3, TNFAIP6, TXK, BACH2, and SERPINB2 are differentially expressed in both venous and arterial thrombosis as well as in APS compared to healthy subjects. Considering that the heterogeneity of t-PAPS manifestations cannot be explained by a single prothrombotic mechanism, it is possible that a gene signature underlies the occurrence and severity of thrombosis in PAPS.
In this context, the primary aim of this study was to determine the expression in patients with t-PAPS of genes previously associated with both arterial and venous thrombosis in the general population. We also investigated whether these genes are dysregulated in asymptomatic aPL carriers.
Materials and methods
Study design and participant selection
In this case-control study, we initially included consecutive patients with t-PAPS treated at the Hematology and Hemotherapy Center of the University of Campinas–Hemocentro-UNICAMP and individuals without a history of thrombosis (controls). Subsequently, asymptomatic aPL carriers were also included in the study. The study was approved by the local Ethics Committee (CAAE: 14902019.1.0000.5404), and the procedures were only performed after a signed informed consent form was obtained from patients.
We included patients with confirmed APS, a history of at least one previous thrombotic event within the previous 10 years. Cancer, pregnancy, isolated obstetric APS, systemic autoimmune diseases and infectious diseases were reasons for exclusion. APS diagnosis was confirmed based on the Sydney criteria (11).
Individuals with no previous history of thrombotic events and negative aPL results (called controls) were selected. Controls were recruited among people from the same geographic region as the patients and matched to them according to sex and age group (± 5 years). Controls were selected among students, university staff and voluntary blood donors. Previous venous thrombosis, stroke, myocardial infarction, neoplasia, and pregnancy were reasons for exclusion. Individuals with persistently positive aPL results and no previous history of thrombosis or obstetric complications suggestive of APS were selected as asymptomatic aPL carriers. aPL carriers group was composed of asymptomatic patients under investigation of prolonged activated partial thromboplastin time (aPTT), immune thrombocytopenia (ITP) patients treated at the same center as t-PAPS or among healthy controls whose aPL tests were positive. Only individuals without signs of infection or acute inflammation were tested for aPL. As part of the diagnosis criteria (11), aPL positivity was confirmed after a 12-week interval. Exclusion criteria were the same as for healthy controls.
Patients, controls and aPL carriers answered a questionnaire about their demographic data, habits, health status and use of medication. Information regarding clinical data was also obtained by consulting the electronic medical record.
Laboratory procedures and mRNA expression of selected genes
Blood samples were collected upon inclusion into anticoagulant ethylenediaminetetraacetic acid (EDTA)-containing tubes. The samples were processed within 2 h of collection, the red blood cells were lysed with a buffer containing ammonium chloride and ammonium bicarbonate and centrifuged in a refrigerated centrifuge at 4°C, and the leukocyte layer was separated for RNA isolation. Samples were stored at −80°C until analysis.
As mentioned above, the relative expression of the five genes of interest was investigated in t-PAPS patients. These genes were selected among those described in a recent meta-analysis that evaluated genes with concordant regulation in arterial and venous thrombosis (8). The ones most associated with arterial and venous thrombosis, three upregulated (ANXA3, TNFAIP6, and SERPINB2) and two downregulated (TXK and BACH2), were selected to be validated in thrombotic APS.
TRIzol™ reagent (Invitrogen™) was used to isolate mRNA from total leukocytes, following the manufacturer’s instructions. Next, samples were transcribed into cDNA using the RevertAid First Strand cDNA Synthesis Kit® (Thermo Scientific™). The order and steps of incubation were performed according to manufacturer’s instructions.
The primers used in the real-time quantitative PCR (qPCR) reaction were designed in the Gene Runner program (Supplementary Table 1). We verified the specificity, dissociation temperature, and formation of secondary structures of the primer pair. The slope of a standard curve is commonly used to estimate the amplification efficiency of a real-time qPCR. The estimated efficiency (EFF) calculation for a real-time PCR assay is EFF = (10−1/slope−1)×100 (12). An ANXA3 calibration curve was obtained at a primer concentration of 150 nM [slope −3.4, correlation coefficient (CC) = 0.99 and EFF 93%], TNFAIP6 at 150 nM [slope −3.3, CC = 0.99, and EFF 99%], TXK at 150 nM [slope −3.3, CC = 0.99 and EFF 98%], BACH2 at 150 nM [slope −3.4, CC = 0.99 and EFF 95%], SERPINB2 at 300 nM [slope −3.3, CC = 0.98 and EFF 99%], RHOA at 150 nM [slope −3.2, CC = 0.99 and EFF 104%], and EEF2 at 150 nM [slope −3.3, CC = 0.99 and EFF 99%]. Relative expression of genes was normalized using EEF2 and RHOA as reference genes. Real-time amplification detection was performed using a real-time PCR thermocycler (QuantStudio 6 and 7–Thermo Fisher Scientific). Negative controls (NTC–No Template Control) were pipetted into all plates (wells containing only SYBR and primers) without the addition of cDNA to verify the absence of contamination. Reactions were performed in 96-well plates (Applied Biosystems) sealed with optical adhesives (Applied Biosystems). The relative expression was denoted as an “arbitrary unit” (AU).
Statistical analysis
Descriptive analyses were performed using frequency tables for categorical variables and position and dispersion measures for numerical variables, which were expressed as the mean and standard deviation (if the variable was normally distributed) or median and interquartile range [IQR] (if the variable was non-normally distributed).
To compare relative mRNA expression between two groups, we used either a parametric t-test or a non-parametric Mann-Whitney test, depending on the distribution of values (normal vs. non-normal). For the correlation between two variables, a non-parametric test of the Spearman rank correlation coefficient was used.
Logistic regression models were used to evaluate the association between relative ANXA3, TNFAIP6, TXK, BACH2, and SERPINB2 mRNA expression and t-PAPS. Next, patients with t-PAPS were divided into 3 subgroups: (i) venous thrombosis or arterial thrombosis; (ii) single thrombotic event or multiple thrombotic events; and (iii) non-triple aPL-positive or triple aPL-positive. Logistic regression models were used to identify the association between mRNA expression and the subgroups, using the control group as a reference. The results were expressed as odds ratios (ORs) and 95% confidence intervals (CIs).
SPSS version 25.0 for Windows (SPSS Inc., Chicago, IL, USA) was used for statistical analyses. GraphPad Prism version 8.0 (GraphPad Software Inc., La Jolla, CA, USA) was used for graph plotting.
Results
Patient’s selection and clinical characteristics
A total of 219 participants were included in the study: 102 t-PAPS, 100 healthy controls and 17 asymptomatic aPL carriers. Figure 1 illustrates the flowchart of the selection process of controls and patients, as well as the reasons for exclusion from the study.
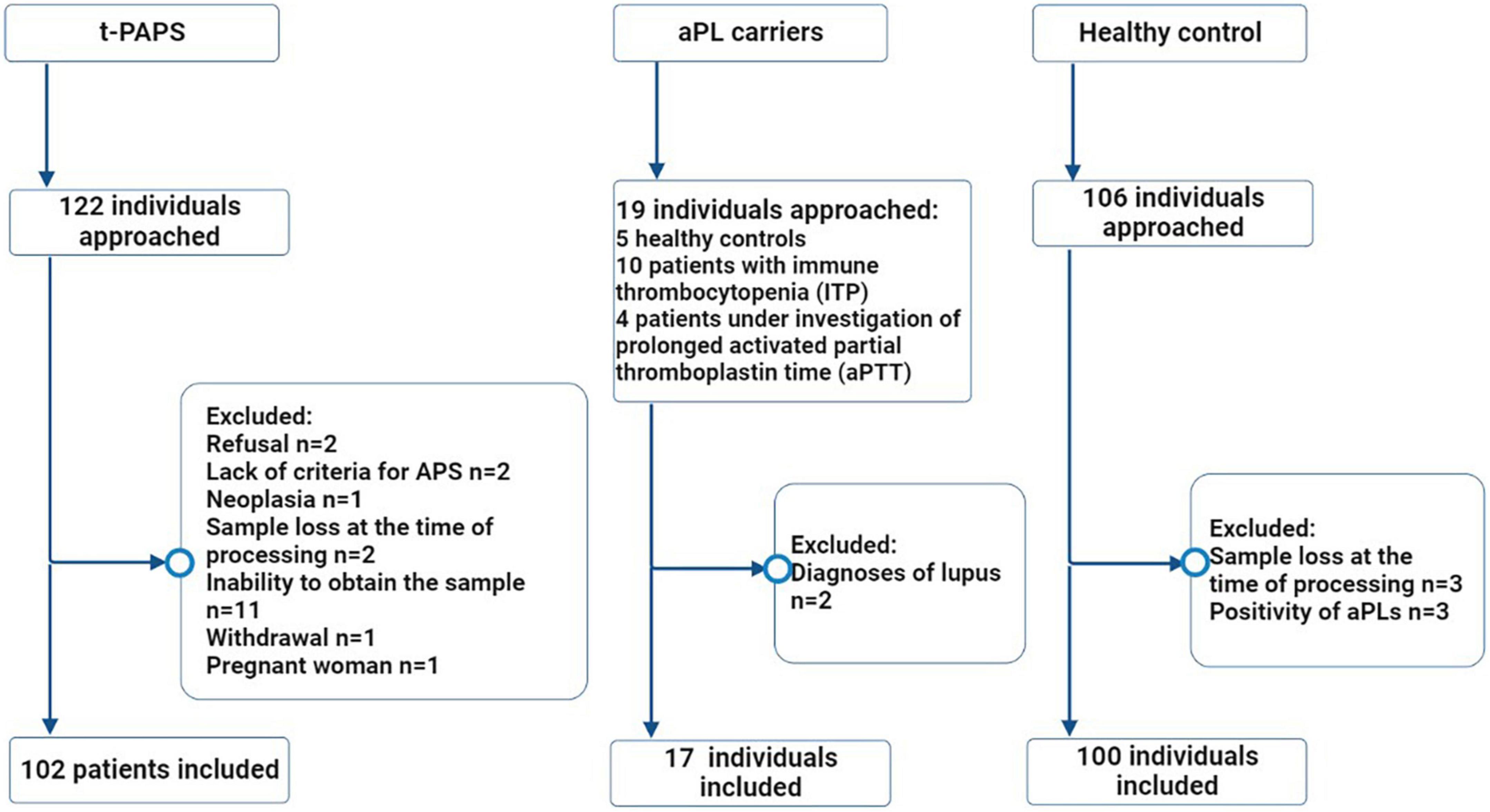
Figure 1. Flowchart of the selection process of study participants. Legend: The flowchart illustrates the number of individuals who were approached (n = 247), the excluded participants and the reasons for exclusions, the final number of individuals who were included in the study (n = 219) and the distribution between the groups. t-PAPS, primary thrombotic APS; aPL, antiphospholipid antibodies; *n, absolute number.
Participants’ demographic, clinical and laboratory features are shown in Table 1. Cardiovascular risk factors were more prevalent in t-PAPS than in asymptomatic aPL carriers or controls. Most t-PAPS and asymptomatic aPL carriers were positive for lupus anticoagulant, while 22% of t-PAPS and 29% of asymptomatic aPL carriers were triple positive for aPLs. Details on APS-associated thrombotic events are presented in Table 2. The median age at the time of the first thrombosis was 35 years old [IQR 25–48], 60% of thrombotic events were unprovoked, 72% were venous and 28% arterial thrombosis. The first thrombotic event was deep vein thrombosis in 42% of the patients, ischemic stroke in 25%, pulmonary thromboembolism (PE) in 15%, and cerebral venous thrombosis in 12%. Portal vein thrombosis, acute myocardial infarction, intestinal thrombosis and retinal thrombosis occurred in 6% of the patients. A total of 36% of the patients had multiple thrombotic events. The median time elapsed between thrombotic events was 47 months [IQR 12.0–91.5].
ANXA3, TNFAIP6, and TXK are differentially expressed in patients with t-PAPS as compared to the control group
When comparing relative mRNA expressions, t-PAPS patients had higher levels of ANXA3 and TNFAIP6 and lower levels of TXK than healthy controls [P = 0.005; P = 0.002, and P = < 0.0001, respectively]. There were no statistically relevant differences between groups in BACH2 and SERPINB2 levels (Figure 2, panel A). Consequently, increased ANXA3 and TNFAIP6 mRNA expression and decreased TXK mRNA expression were associated with a higher risk of t-PAPS (Figure 2, panel B).
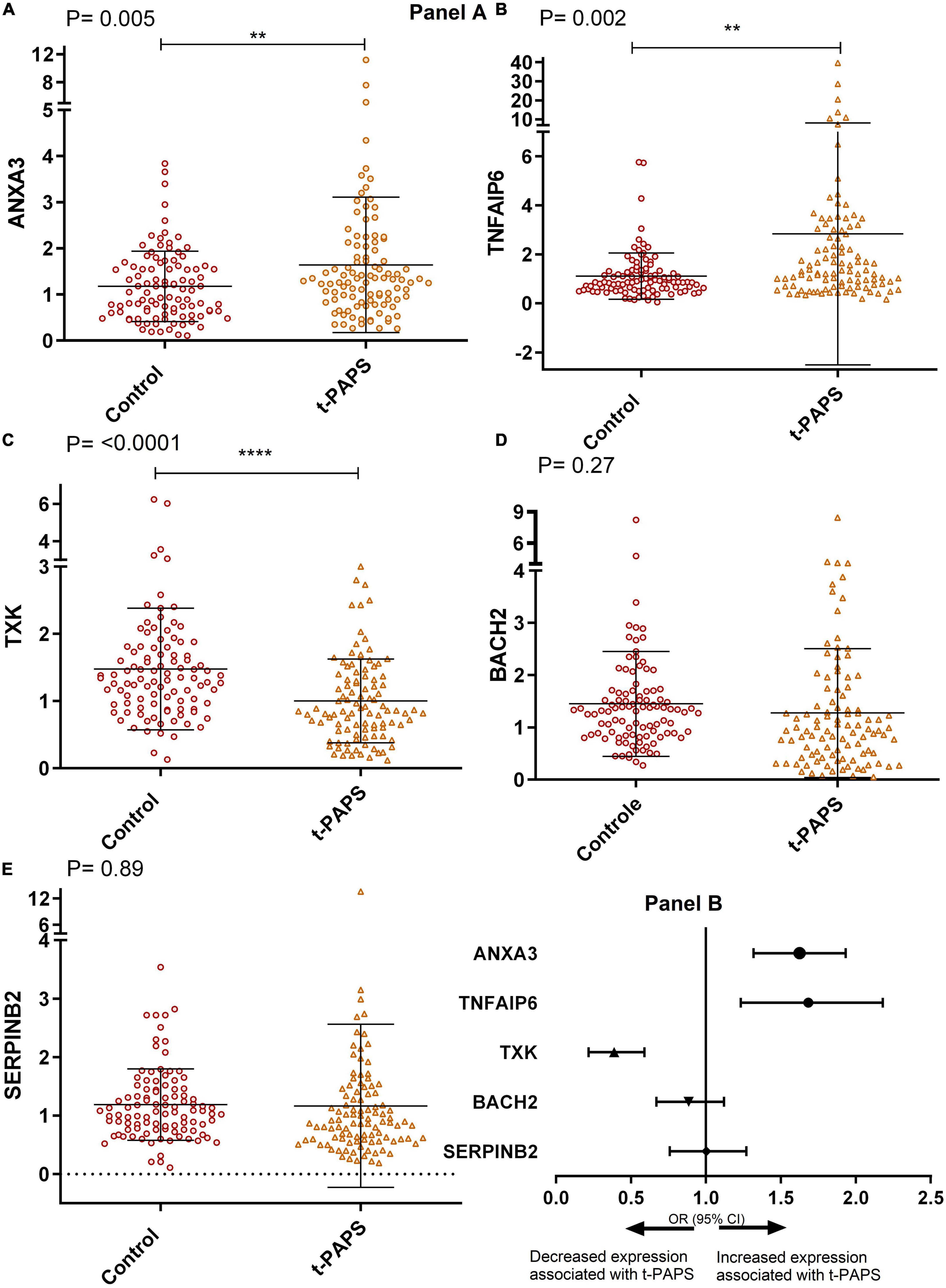
Figure 2. Panel (A) comparative graphs of the expression of genes related to thrombosis. Relative expression in total leukocytes of the genes: (A) ANXA3, (B) TNFAIP6, (C) TXK, (D) BACH2, and (E) SERPINB2. To illustrate the graphs above, mean and SD were used, and P-values were calculated using Student’s t-test. t-PAPS = primary thrombotic APS. The mean ANXA3 mRNA expression was 1.17 AU [95% CI 1.03–1.33] in controls and 1.64 AU [95% CI 1.36–1.93] in t-PAPS [P = 0.005]. The mean TNFAIP6 expression was 1.10 AU [95% CI 0.92–1.29] in controls and 2.84 [95% CI 1.89–3.89] in t-PAPS [P = 0.002]. The mean TXK expression was 1.47 AU [95% CI 1.30–1.66] in controls and 1.00 AU [95% CI 0.88–1.12] in t-PAPS [P = < 0.0001]. The mean BACH2 expression was 1.45 AU [95% CI 1.25–1.65] in controls and 1.27 AU [95% CI 1.03–1.52] in t-PAPS [P = 0.27]. Finally, the mean SERPINB2 expression was 1.19 AU [95% CI 1.07–1.31] in controls and 1.17 AU [95% CI 0.89–1.44] in t-PAPS [P = 0.89]. Panel (B) association of ANXA3, TNFAIP6, TXK, BACH2, and SERPINB2 relative expression with t-PAPS diagnosis. Increased ANXA3 mRNA expression [OR = 1.57; 95% CI 1.13–2.18; P = 0.008] and TNFAIP6 expression [OR = 1.64; 95% CI 1.23–2.18; P = 0.001] and decreased TXK mRNA expression [OR = 0.36; 95% CI 0.22–0.59; P = 0.0001] were associated with a higher risk of t-PAPS compared with controls. BACH2 and SERPINB2 mRNA expression was not associated with t-PAPS [OR = 0.87; 95% CI 0.67–1.12; P = 0.28 and OR = 0.98; 95% CI 0.75–1.3; P = 0.89, respectively]. OR, odds ratio; CI, confidence interval; t-PAPS, primary thrombotic APS. **P < 0.01 and ****P = < 0.0001.
In addition, as shown in Figure 3, the mRNA expression levels of ANXA3, TNFAIP6, TXK, BACH2, and SERPINB2 were similar between asymptomatic aPL carriers and controls. [P = 0.77; P = 0.48; P = 0.08; P = 0.73, and P = 0.13, respectively].
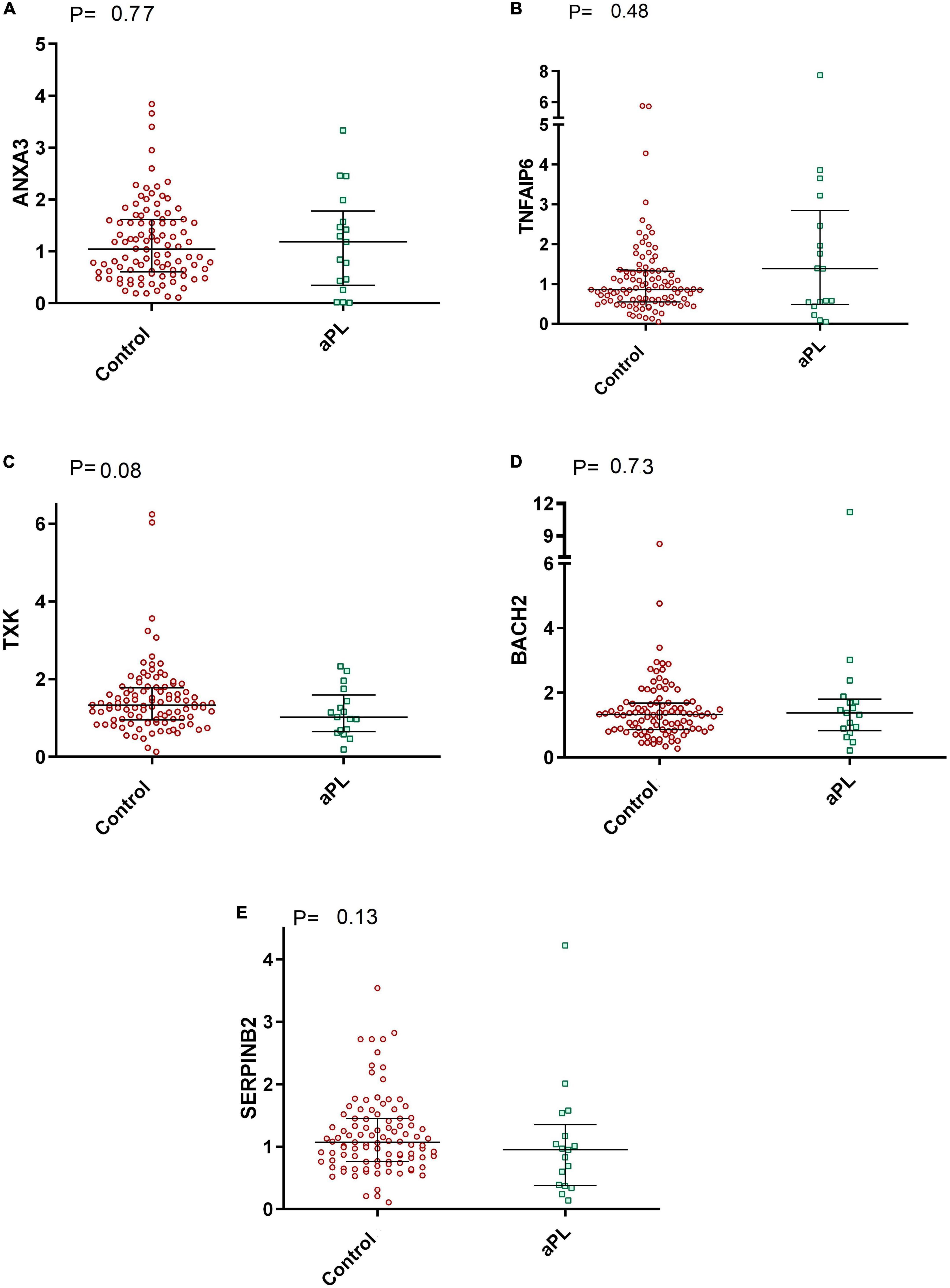
Figure 3. Comparative graphs of the expression of genes related to thrombosis. Legend: Relative expression of the genes (A) ANXA3, (B) TNFAIP6, (C) TXK, (D) BACH2, and (E) SERPINB2 in total leukocytes was evaluated in aPL carriers compared to controls. Median and IQR were used by the Mann-Whitney test. aPL, asymptomatic aPL carriers (aPL +). The median relative expression of the ANXA3 gene was 1.04 (IQR 0.60–1.61) in control subjects and 1.18 (IQR 0.34–1.78) in aPL + subjects. The relative expression of TNFAIP6 was 0.86 (IQR 0.55–1.32) in controls and 1.38 (IQR 0.49–2.84) in aPL +. The relative expression of TXK was 1.33 (IQR 0.95–1.77) in controls and 1.02 (IQR 0.65–1.59) in aPL + patients. BACH2 relative expression was 1.32 (IQR 0.86–1.68) in controls and 1.38 (IQR 0.82–1.80) in aPL +. Finally, the relative expression of SERPINB2 was 1.07 (IQR 0.76–1.45) in controls and 0.95 (IQR 0.38–1.35) in aPL +.
Elevated ANXA3 expression tends to be associated with arterial thrombosis and multiple thrombotic events
The association of ANXA3, TNFAIP6, TXK, BACH2, and SERPINB2 mRNA expression with the type of thrombosis (arterial or venous) in t-PAPS is shown in Figure 4, Panel A. ANXA3 mRNA upregulation was more evident in arterial thrombosis than in venous thrombosis when compared with controls. The relative expression of the other genes did not differ significantly between arterial and venous t-PAPS compared to controls.
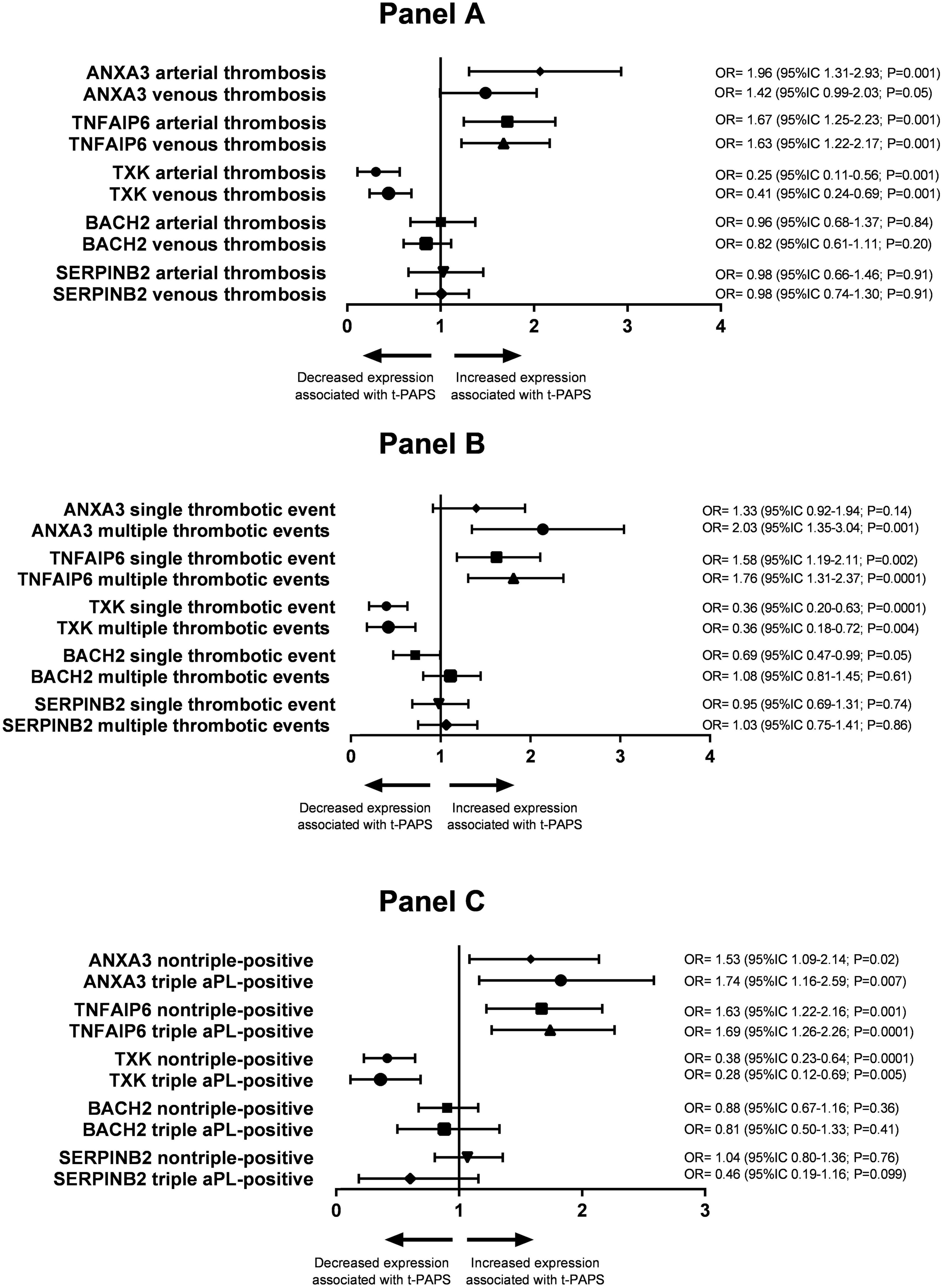
Figure 4. Panel (A) association between the relative expression of the study genes and arterial or venous thrombosis in t-PAPS. Panel (B) association between the relative expression of the study genes and a single thrombotic event or multiple events in t-PAPS. Panel (C) association between the relative expression of the study genes and non-triple-positive or triple aPL-positive subgroups. OR, odds ratio; CI, confidence interval; t-PAPS, primary thrombotic APS.
Next, the association of ANXA3, TNFAIP6, TXK, BACH2, and SERPINB2 mRNA expression with the number of previous thrombosis events (single or multiple thrombosis) is shown in Figure 4, Panel B. ANXA3 mRNA upregulation was, again, more pronounced in multiple thrombosis than in single thrombosis when compared to controls. BACH2 mRNA downregulation was more pronounced in single thrombosis than in multiple thrombosis compared to controls. The relative expression of the remaining genes did not differ between single and multiple thrombotic events compared to controls.
Finally, ANXA3, TNFAIP6, TXK, BACH2, and SERPINB2 mRNA expression levels were similar between non-triple-positive and triple aPL-positive t-PAPS (Figure 4, Panel C).
Discussion
Antiphospholipid syndrome is a good clinical model to evaluate similarities and differences between venous and arterial thrombosis, as the disease is characterized by thrombotic manifestations in multiple vascular sites. This heterogeneous presentation is intriguing because venous and arterial thrombosis appear to have different pathogenesis. While stasis-related mechanisms, such as immobilization and congestive heart failure, and hypercoagulability-related mechanisms, such as familial thrombophilia and oral contraceptive use, play a fundamental role in the occurrence of venous thrombosis (13), arterial thrombosis occurs in high-flow sites and is mainly dependent on vascular integrity and platelet function (14, 15). However, some clinical aspects may be common to both arterial and venous thrombosis. Age, obesity and hypertension are known risk factors for atheromatous disease and arterial thrombosis. These factors may also contribute to the development of venous thrombosis (16, 17).
Much remains to be understood about common pathologic mechanisms leading to heterogeneous thrombotic manifestations in APS. One way to investigate common or diverse causes of the heterogeneous clinical presentation of APS is to study the genetic profile of the disease. Translational studies have allowed the identification of gene sets (or signatures) associated with PAPS (18) and secondary APS (SAPS) (19), as well as the expression profile of genes that differentiate thrombotic APS from APS with obstetric complications (9).
For example, obstetric APS is characterized by differentially expressed genes involved in cell adhesion, extracellular matrix, embryogenesis, and skeletal development, whereas thrombotic APS is characterized by differentially expressed genes involved in pro-inflammatory cytokine production, cellular response to stress, oxidative stress, and cellular homeostasis (9). PAPS and SAPS share the expression of genes involved in the type 1 interferon signature, inflammation and atherosclerosis (10), whereas PAPS differs from systemic lupus erythematosus (SLE) by the expression of genes related to mitochondrial function, oxidative stress and antioxidant capacity (10, 18, 19). We recently observed that genes related to the type 1 interferon signature were overexpressed in t-SAPS, but not in t-PAPS, compared to controls (20), suggesting that the inflammatory profile is different between these two presentations of APS. A recent meta-analysis identified 16 genes differentially expressed in t-PAPS compared to controls that were expressed in 32 different tissues, which may explain the fact that thrombosis in APS occurs in any vascular site (18). Thus, measurement of gene expression has been used to develop new biological concepts, refine disease classification, improve diagnostic and prognostic accuracy, and identify new molecular targets for treatment.
We chose to quantify ANXA3, TNFAIP6, TXK, BACH2, and SERPINB2 mRNA expression in t-PAPS based on the results of a meta-analysis using a bioinformatics panel to explore the differences and similarities between venous thromboembolism (VTE) and cardiovascular disease. The results of the meta-analysis represented the first comparison of venous and arterial thrombosis at the transcriptomic level, analyzing gene expression datasets from microarray studies involving human patients with cardiovascular disease or VTE in the Gene Expression Omnibus (GEO) public repository (8); however, they needed to be validated in a real-world cohort.
In this context, this study analyzed the expression of genes previously associated with venous and arterial thrombosis in the general population in t-PAPS and asymptomatic aPL carriers. Our results showed that the expression of ANXA3 and TNFAIP6 is upregulated and TXK is downregulated in t-PAPS compared to controls. Furthermore, increased ANXA3 mRNA expression is more pronounced in patients with arterial thrombosis or multiple thromboses, suggesting that ANXA3 is not only associated with thrombosis in PAPS but also with the severity of the thrombotic event.
ANXA3, TNFAIP6, TXK, BACH2, and SERPINB2 are mainly associated with immunity and hemostasis. ANXA3 plays a role in regulation of cell growth, regulation of endothelial cell migration, maintenance of cellular phospholipids and signal transduction pathways and has been associated with stroke in animal studies (21). TNFAIP6 is involved in cell-cell and cell-matrix interactions in inflammation and cancer, and its increased expression in t-PAPS may be explained by the inflammatory process of the disease (8, 22). TXK acts on pathways related to the blood-brain barrier, transmigration of immune cells, and regulates the development, function, and differentiation of T-cells and NKT cells (23, 24). BACH2 is responsible for immune regulation, mainly related to the adaptive immune response, and has been implicated in B-cell function (25, 26). SERPINB2 regulates the production of plasminogen activator inhibitor-2 and is directly related to fibrinolysis pathways (8, 27). Our study showed that t-PAPS is associated with dysregulation of genes related to hemostasis (ANXA3) and inflammation/immunity (TNFAIP6 and TXK). ANXA3 has also been associated with the occurrence of arterial thrombosis and multiple thrombotic events. Interestingly, this gene has been shown to be upregulated in rodent models of ischemic stroke in animal studies (28). Therefore, our results suggest that innate immunity and hemostasis are associated with thrombotic manifestations of t-PAPS at the molecular level, with the potential to detect more severe forms of the disease.
In the meta-analysis that inspired this study, the association between BACH2 and SERPINB2 with arterial and venous thrombosis was weaker than that of the other genes. Moreover, from a biological point of view, SERPINB2 regulates the plasminogen activator-2 inhibitor pathway, which is responsible for a fibrinolytic mechanism that is less associated with APS-related hypercoagulability. BACH2 is a gene related to adaptive/humoral immunity, it has been described as a regulator of adaptive immunity through T-cell maintenance and B-cell maturation. In t-PAPS patients, we observed a dysregulation of genes associated with innate immunity.
The estimated incidence of thrombosis in asymptomatic aPL+ carriers is approximately 1% per year (29). Present in up to 5% of the general population, aPL antibodies may either be clinically irrelevant or confer an increased risk of thrombotic manifestations (30). In this context, we included the group of asymptomatic aPL carriers in this study to evaluate whether the molecular alterations observed in t-PAPS are also present in asymptomatic aPL carriers. Interestingly, the mRNA expression levels of ANXA3, TNFAIP6, TXK, BACH2, and SERPINB2 were similar between asymptomatic aPL carriers and controls. Gene expression was not affected by aPL profile, particularly aPL triple positivity. Taken together, these results suggest that dysregulation of ANXA3, TNFAIP6, and TXK, although associated with thrombotic manifestations of PAPS, is not due to the presence or burden of aPL antibodies.
Limitations of our study include the fact that we included a group of patients treated in a tertiary care center and, therefore, it is possible that our patients presented with a more severe clinical profile. The cohort is heterogeneous because of the variable duration of the disease; however, there was no correlation between the time elapsed since diagnosis of t-PAPS and the relative expression of the genes (data not shown). Data collection was performed retrospectively by consulting electronic medical records, which may contain imprecise information.
Conclusion
The results demonstrated that genes related to both innate immunity and hemostasis were associated with thrombotic manifestations in PAPS and with disease severity (arterial thrombosis and multiple thrombotic events), independent of the aPL profile. Therefore, our findings suggest a molecular link between hemostasis, immune response and thrombosis in PAPS, providing new insights into the mechanisms underlying the development of thrombosis in PAPS.
Data availability statement
The original contributions presented in this study are included in the article/Supplementary material, further inquiries can be directed to the corresponding author.
Ethics statement
The studies involving human participants were reviewed and approved by the CEP UNICAMP–Campus Campinas. The patients/participants provided their written informed consent to participate in this study.
Author contributions
BJ performed the laboratory and statistical analyses and drafted the manuscript. BM, BH, JO, AS, CV, and EP revised the manuscript. FO designed the study and the analyses and revised the manuscript. All authors contributed to the article and approved the submitted version.
Funding
This study was funded by the São Paulo Research Foundation–FAPESP (grant number: 2016/14172-6) and the National Council for Scientific and Technological Development-CNPq (grant number: 43833902018-5). BJ received financial support from FAPESP (grant numbers: 2019/20136-0 and 2021/07150-4) and the Coordination for the Improvement of Higher Education Personnel (CAPES)–88887.499972/2020-00.
Conflict of interest
The authors declare that the research was conducted in the absence of any commercial or financial relationships that could be construed as a potential conflict of interest.
Publisher’s note
All claims expressed in this article are solely those of the authors and do not necessarily represent those of their affiliated organizations, or those of the publisher, the editors and the reviewers. Any product that may be evaluated in this article, or claim that may be made by its manufacturer, is not guaranteed or endorsed by the publisher.
Supplementary material
The Supplementary Material for this article can be found online at: https://www.frontiersin.org/articles/10.3389/fmed.2023.1139906/full#supplementary-material
References
1. Gardiner C, Hills J, MacHin S, Cohen H. Diagnosis of antiphospholipid syndrome in routine clinical practice. Lupus. (2013) 22:18–25.
2. Espinosa G, Cervera R. Antiphospholipid syndrome: Frequency, main causes and risk factors of mortality. Nat Rev Rheumatol. (2010) 6:296–300. doi: 10.1038/nrrheum.2010.47
3. Meroni P, Borghi M, Raschi E, Tedesco F. Pathogenesis of antiphospholipid syndrome: understanding the antibodies. Nat Rev Rheumatol. (2011) 7:330. doi: 10.1038/nrrheum.2011.52
4. Ruiz-Irastorza G, Crowther M, Branch W, Khamashta M. Antiphospholipid syndrome. Lancet. (2010) 376:1498–509.
5. Lopes M, Danowski A, Funke A, Rêgo J, Levy R, de Andrade D. Update on antiphospholipid antibody syndrome. Rev Assoc Med Bras. (2017) 63:994–9.
6. Cervera R, Piette J, Font J, Khamashta M, Shoenfeld Y, Camps M, et al. Antiphospholipid syndrome: Clinical and immunologic manifestations and patterns of disease expression in a cohort of 1,000 patients. Arthritis Rheum. (2002) 46:1019–27. doi: 10.1002/art.10187
7. Lopez-pedrera C, Barbarroja N, Patiño-trives A. New Biomarkers for Atherothrombosis in Antiphospholipid Syndrome : Genomics and Epigenetics Approaches. Front Immunol. (2019) 10:764. doi: 10.3389/fimmu.2019.00764
8. Hounkpe B, de Oliveira Benatti R, de Sá Carvalho B, de Paula E. Identification of common and divergent gene expression signatures in patients with venous and arterial thrombosis using data from public repositories. PLoS One. (2020) 15:e0235501. doi: 10.1371/journal.pone.0235501
9. Ripoll V, Pregnolato F, Mazza S, Bodio C, Grossi C, Mcdonnell T, et al. Gene expression pro filing identi fies distinct molecular signatures in thrombotic and obstetric antiphospholipid syndrome. J Autoimmun. (2018) 93:114–23. doi: 10.1016/j.jaut.2018.07.002
10. Knight J, Meng H, Coit P, Yalavarthi S, Sule G, Gandhi A, et al. Activated signature of antiphospholipid syndrome neutrophils reveals potential therapeutic target. JCI Insight. (2017) 2:e93897. doi: 10.1172/jci.insight.93897
11. Miyakis S, Lockshin M, Atsumi T, Derksen R, Groot P, Koike T. International consensus statement on an update of the classification criteria for definite antiphospholipid syndrome. J Thromb Haem. (2006) 4:295–306. doi: 10.1111/j.1538-7836.2006.01753.x
12. Donia D, Divizia M, Pana’ A. Use of armored RNA as a standard to construct a calibration curve for real-time RT-PCR. J Virol Methods. (2005) 126:157–63. doi: 10.1016/j.jviromet.2005.02.004
16. Green D. Risk of future arterial cardiovascular events in patients with idiopathic venous thromboembolism. Hematology. (2009) 6:259–66. doi: 10.1182/asheducation-2009.1.259
17. Ageno W, Becattini C, Brighton T, Selby R, Kamphuisen P. Cardiovascular risk factors and venous thromboembolism: A meta-analysis. Circulation. (2008) 117:93–102.
18. Islam A, Saif S, Alam F. Genetic risk factors in thrombotic primary antiphospholipid syndrome : a systematic review with bioinformatic analyses. Autoimmun Rev. (2018) 17:226–43. doi: 10.1016/j.autrev.2017.10.014
19. Perez-Sanchez C, Barbarroja N, Messineo S, Ruiz-Limon P, Rodriguez-Ariza A, Jimenez-Gomez Y, et al. Gene profiling reveals specific molecular pathways in the pathogenesis of atherosclerosis and cardiovascular disease in antiphospholipid syndrome, systemic lupus erythematosus and antiphospholipid syndrome with lupus. Ann Rheum Dis. (2015) 74:1441–9. doi: 10.1136/annrheumdis-2013-204600
20. Rosa dos Santos A, de Oliveira Vaz C, Hounkpe B, Jacintho B, Oliveira J, Tripiquia Vechiatto Mesquita G, et al. Association between interferon-I producing plasmacytoid dendritic cells and thrombotic antiphospholipid syndrome. Lupus. (2022) 31:1067–77. doi: 10.1177/09612033221101731
21. Wang X, Li X, Xu L, Zhan Y, Yaish-Ohad S, Erhardt J, et al. Up-regulation of secretory leukocyte protease inhibitor (SLPI) in the brain after ischemic stroke: Adenoviral expression of SLPI protects brain from ischemic injury. Mol Pharmacol. (2003) 64:833–40. doi: 10.1124/mol.64.4.833
22. Danchuk S, Ylostalo J, Hossain F, Sorge R, Ramsey A, Bonvillain R, et al. Human multipotent stromal cells attenuate lipopolysaccharide-induced acute lung injury in mice via secretion of tumor necrosis factor-α-induced protein 6. Stem Cell Res Ther. (2011) 2:27. doi: 10.1186/scrt68
23. Kashiwakura J, Suzuki N, Nagafuchi H, Takeno M, Takeba Y, Shimoyama Y. Txk, a Nonreceptor Tyrosine Kinase of the Tec Family, Is Expressed in T Helper Type 1 Cells and Regulates Interferon γ Production in Human T Lymphocytes. J Exp Med. (2002) 190:1147–54. doi: 10.1084/jem.190.8.1147
24. Schneider H, Schwartzberg P, Rudd C. Resting lymphocyte kinase (RIk/Txk) phosphorylates the YVKM motif and regulates PI 3-kinase binding to T-Cell antigen CTLA-4. Biochem Biophys Res Commun. (1998) 252:14–9. doi: 10.1006/bbrc.1998.9559
25. Yoshida C, Yoshida F, Sears D, Hart S, Ikebe D, Muto A, et al. Bcr-Abl signaling through the PI-3/S6 kinase pathway inhibits nuclear translocation of the transcription factor Bach2, which represses the antiapoptotic factor heme oxygenase-1. Blood. (2007) 109:1211–9. doi: 10.1182/blood-2005-12-040972
26. Afzali B, Grönholm J, Vandrovcova J, O’Brien C, Sun H, Vanderleyden I, et al. BACH2 immunodeficiency illustrates an association between super-enhancers and haploinsufficiency. Nat Immunol. (2017) 18:813–23. doi: 10.1038/ni.3753
27. Corsetti J, Salzman P, Ryan D, Moss A, Zareba W, Sparks C. Influences on plasminogen activator inhibitor-2 polymorphism-associated recurrent cardiovascular disease risk in patients with high HDL cholesterol and inflammation. Atherosclerosis. (2016) 250:1–8. doi: 10.1016/j.atherosclerosis.2016.04.017
28. Junker H, Suofu Y, Venz S, Sascau M, Herndon J, Kessler C, et al. Proteomic identification of an upregulated isoform of annexin A3 in the rat brain following reversible cerebral ischemia. Glia. (2007) 55:1630–7. doi: 10.1002/glia.20581
29. Arnaud L, Mathian A, Ruffatti A, Erkan D, Tektonidou M, Cervera R, et al. Efficacy of aspirin for the primary prevention of thrombosis in patients with antiphospholipid antibodies: An international and collaborative meta-analysis. Autoimmun Rev. (2014) 13:281–91. doi: 10.1016/j.autrev.2013.10.014
30. Clark E, Silver R, Branch D. Do Antiphospholipid Antibodies Cause Preeclampsia and HELLP Syndrome? Curr Rheumatol Rep. (2007) 9:219–25.
Keywords: antiphospholipid syndrome, thrombosis, gene expression, antiphospholipid antibody, mRNA
Citation: Jacintho BC, Mazetto Fonseca BdM, Hounkpe BW, Oliveira JD, dos Santos APR, Vaz CdO, de Paula EV and Orsi FA (2023) Evaluation of a gene signature related to thrombotic manifestations in antiphospholipid syndrome. Front. Med. 10:1139906. doi: 10.3389/fmed.2023.1139906
Received: 07 January 2023; Accepted: 03 March 2023;
Published: 23 March 2023.
Edited by:
Antoni Riera-Mestre, Bellvitge University Hospital, SpainReviewed by:
Pau Cerdà Serra, Bellvitge University Hospital, SpainAriadna Padró-Miquel, Bellvitge University Hospital, Spain
Copyright © 2023 Jacintho, Mazetto Fonseca, Hounkpe, Oliveira, dos Santos, Vaz, de Paula and Orsi. This is an open-access article distributed under the terms of the Creative Commons Attribution License (CC BY). The use, distribution or reproduction in other forums is permitted, provided the original author(s) and the copyright owner(s) are credited and that the original publication in this journal is cited, in accordance with accepted academic practice. No use, distribution or reproduction is permitted which does not comply with these terms.
*Correspondence: Fernanda Andrade Orsi, ZmVyb3JzaUB1bmljYW1wLmJy