- 1Department of Anesthesia, General Intensive Care and Pain Medicine, Medical University of Vienna, Vienna, Austria
- 2Department of Anesthesiology and Intensive Care Medicine, AUVA Trauma Center Vienna, Vienna, Austria
According to the Berlin Definition of acute respiratory distress syndrome (ARDS), a positive end-expiratory pressure (PEEP) of at least 5 cmH2O is required to diagnose and grade ARDS. While the Berlin consensus statement specifically acknowledges the role of non-invasive ventilation (NIV) in mild ARDS, this stratification has traditionally presumed a mechanically ventilated patient in the context of moderate to severe ARDS. This may not accurately reflect today’s reality of clinical respiratory care. NIV and high-flow nasal cannula oxygen therapy (HFNO) have been used for managing of severe forms of acute hypoxemic respiratory failure with growing frequency, including in patients showing pathophysiological signs of ARDS. This became especially relevant during the COVID-19 pandemic. The levels of PEEP achieved with HFNO have been particularly controversial, and the exact FiO2 it achieves is subject to variability. Pinpointing the presence of ARDS in patients receiving HNFO and the severity in those receiving NIV therefore remains methodically problematic. This narrative review highlights the evolution of the ARDS definition in the context of non-invasive ventilatory support and provides an overview of the parallel development of definitions and ventilatory management of ARDS. It summarizes the methodology applied in clinical trials to classify ARDS in non-intubated patients and the respective consequences on treatment. As ARDS severity has significant therapeutic and prognostic consequences, and earlier treatment in non-intubated patients may be beneficial, closing this knowledge gap may ultimately be a relevant step to improve comparability in clinical trial design and outcomes.
1. Introduction
In pathophysiological terms, acute respiratory distress syndrome (ARDS) is an inflammatory reaction of the lung to certain triggers (1). It results in loss of integrity of the alveolo-capillary barrier with consecutive protein-rich exudates causing non-hydrostatic pulmonary edema and inactivation of surfactant. The inflammation is sustained and propagated by a complex immunologic interplay and eventually progresses to either recovery, various degrees of chronic fibrotic lung damage, or even death.
The clinical features of ARDS are those of type 1 respiratory failure, namely profound, refractory hypoxia paired with bilateral opacities in chest x-ray (CXR) or computed tomography (CT). Of note, even in the original description by David Ashbaugh and colleagues, this acute hypoxemic respiratory failure (AHRF) is neither in proportion to nor a consequence of coexisting prior lung disease, congestive heart failure, or fluid overload (2). In fact, it may occur in the absence of any of the mentioned factors. Since Ashbaugh’s report, several definitions have been proposed to characterize the phenotype of ARDS and standardize its diagnosis, treatment, and severity stratification for research (Table 1). The currently used Berlin Definition of ARDS was published in 2012 and has replaced the American-European Consensus Conference (AECC) definition (3–5).
2. Ventilatory support in acute hypoxemic respiratory failure
2.1. Non-invasive ventilatory support
In clinical practice, any type of respiratory failure is commonly managed with an escalating sequence of respiratory support methods, including conventional supplemental oxygen therapy, various types of non-invasive ventilatory support, and, if the aforementioned strategies and pharmaceutical treatments, such as antibiotics or bronchodilators, fail to improve the patient’s condition, endotracheal intubation and mechanical ventilation (6, 7). Non-invasive ventilation (NIV) has been proposed as an early intervention in mild ARDS to reduce the rate of intubation, ventilator associated pneumonia, and length of intensive care unit (ICU) stay (8). NIV can be administered via a tight-fitting facemask as well as a helmet, each entailing their own advantages and disadvantages (9). Helmet NIV may be associated with reduced mortality and a lower rate of intubation according to one meta-analysis, although the included trials analyzed were small in size (10).
Not all guidelines deem the current – in some respects equivocal – evidence sufficient to recommend NIV in new-onset AHRF (11). Just as patients may benefit from avoidance of intubation by successful non-invasive respiratory care, a delay in intubation by prolonged attempts of NIV as well as NIV in patients with a PaO2/FiO2 ratio of less than 150 may be harmful (12, 13). However, a recent meta-analysis has challenged this assumption in the context of COVID-19, as it found no difference in mortality between early and late intubation (14). AHRF with a PaO2/FiO2 ratio of 146 or less, a high expiratory minute volume as well as ARDS have been reported to be independent risk factors for NIV failure, defined as the need for endotracheal intubation, although the exact clinical triggers for this decision varied among protocols (15, 16). Interestingly, in one trial during the earlier phase of the pandemic, COVID-19 patients had an increased risk of NIV failure compared to matched non-COVID-19 ARDS patients (17). Strenuous respiratory efforts in patients receiving NIV for a prolonged time prior to intubation have also been linked to a phenomenon termed patient self-inflicted lung injury (P-SILI), which may further contribute to worse outcomes (18, 19).
In recent years, high flow nasal oxygen (HFNO) therapy has emerged as an effective and well-tolerated tool of post-extubation respiratory care (20, 21) and treatment of respiratory failure (22). Warmed, humidified oxygen is delivered to the patient at a flow rate that may match or considerably exceed peak inspiratory flow rates, reducing the work of breathing. In addition, HFNO leads to dead space washout, improving decarboxylation. It may create a small positive end-expiratory airway pressure (PEEP) in the order of 2–7 cmH2O, but the exact magnitude of this effect is still subject to debate and may depend on the gas flow as well as the caliber of cannulas, and may be significantly decreased if the subject opens their mouth (23–25).
High flow nasal oxygen has been suggested to reduce the rate of endotracheal intubation in both COVID-19-associated and non-COVID-19-associated AHRF in several studies and guidelines (26–29). One trial also suggested lower mortality rates with HFNO compared to conventional oxygen therapy and NIV, although this result was not assessed as the primary outcome (30), and was put into question by a recent Cochrane review (31). Nonetheless, current guidelines recommend the use of HFNO as a first-line therapy for acute type 1 respiratory failure, and guidelines of the European Respiratory Society have explicitly recommended HFNO over NIV and conventional oxygen therapy in these patients (11, 32, 33).
Immunocompromised patients represent a specific patient population, in whom treatment with NIV for AHRF plays an important role. In these patients, treatment with NIV is more frequently applied than in other patients with AHRF (34). This may partially be explained by evidence suggesting that immunocompromised patients requiring invasive mechanical ventilation (MV) have a higher mortality (35). In addition to recent evidence, findings suggest that especially the use of HFNO may significantly reduce the rate of intubation in immunocompromised patients with AHRF (36). However, there may be a downside to this trend, as delaying intubation in an immunocompromised patient who ultimately requires invasive MV is associated with a higher mortality (37). Therefore, correct assessment of disease severity seems to be of utmost importance in in this special patient population as to not delay necessary treatment decisions.
2.2. Invasive mechanical ventilation
Guidelines and strategies for invasive MV have evolved over the past decades (6). The current standard of care focuses on the avoidance of ventilator associated lung injury, using a bundle of care termed lung protective ventilation (38). This involves limiting tidal volumes to 6 ml/kg predicted body weight, avoiding plateau pressures (Pplat) beyond 30 cmH2O, low driving pressure, i.e., the difference between PEEP and Pplat (39), and applying a higher level of PEEP in moderate to severe ARDS (40). In recent years, the COVID-19 pandemic and the associated paucity of resources has shifted clinical and academic interest to non-invasive ventilatory support even in more severe cases of AHRF.
3. Aspects of the Berlin definition
An early diagnosis of ARDS in patients with respiratory failure may lead to timely initiation of evidence-based measures to improve outcomes and has been recommended in clinical guidelines (6). This led us to review the respective aspects of the definition.
3.1. Timing
“Acute” is commonly defined as onset within 1 week after exposure to a clinical risk factor or “new or worsening respiratory symptoms” (4). The main differences to the predecessor definition of the AECC are the specification of a period and the requirement of a risk factor. In certain conditions, the onset of respiratory symptoms may precede ARDS far more than 1 week. Early variant COVID-19 infections have shown a tendency to delayed clinical deterioration, with a time to ICU admission and ARDS diagnosis exceeding 1 week of symptoms in a significant proportion of patients (41). In addition, patients with COVID-19 or other underlying diseases with a duration of ICU stay and mechanical ventilation for more than 1 week may experience protracted episodes of respiratory failure. Causes may be bacterial or fungal superinfections and ventilator-associated pneumonia that only eventually fulfill all ARDS criteria at the same time.
3.2. Chest imaging
The diagnosis of ARDS requires the presence of bilateral opacities on CXR or, in contrast to the AECC criteria, in a CT scan (4). However, patients presenting with bilateral opacities of only two quadrants and thereby qualifying for ARDS showed similar outcomes as AHRF patients with opacities of two unilateral quadrants in a large observational study (42). Although a requirement for more extensive opacities was discussed for severe ARDS, these considerations have been dismissed.
Lung ultrasound (LUS) has gained increased availability and frequency of routine use. A growing body of literature supports its application, a development already observed in the 2009 influenza pandemic (43) and further accelerated by the COVID-19 pandemic. LUS-based protocols have been proposed as an adjunct measure for the diagnosis and monitoring of ARDS in emergency departments (44) and ICUs (45). A frequently quoted systematic approach is the LUS score, ranging from 0 (normal lung) to 36 (severe lung injury) (46, 47). It is a semi-quantitative measure of severity of lung injury based on the presence of B-lines, pleural line abnormalities, and consolidations assessed in 12 defined anatomical regions. In a retrospective study in COVID-19 patients comparing a modified LUS protocol and chest CT, scores <13 and >23 were shown to have high sensitivity and specificity for mild and severe disease, respectively, whereas scores in the mid-range provided less diagnostic information (48). The latter observation as well as limitations inherent to the technique (i.e., diagnostic value may be influenced by operator experience, available time, etc.) may pose obstacles to the widespread implementation of LUS in incipient ARDS. The operator learning curve has been described variably. While some have suggested a steep learning curve, requiring a practitioner to perform as few as a dozen of examinations to gain sufficient skill, other experts are of the opinion that true competence may not be attained in a short time (49). However, the fact that LUS has been shown to predict mortality may still warrant its consideration in future ARDS definitions (50). Because of increased routine use of LUS, ICU patients may not undergo routine CXR as often (51), and LUS may be more readily available in low resource settings, where access to high end radiological devices may be limited (52).
3.3. Origin of edema
Prior to Ashbaugh’s landmark description of what is today known as ARDS, a condition termed “congestive atelectasis” with features including tachypnea, hypoxemia, a “stiff” lung and alveolar collapse was described in patients who had received large quantities of intravenous fluids and transfusions (53). Some authors consider this phenomenon a predecessor of ARDS. Indeed, cardiogenic pulmonary edema and fluid overload are frequent differential diagnoses of ARDS. In contrast to these early descriptions, both the AECC and the Berlin Definition require that the etiology of respiratory failure should not be “fully explained” by those conditions (4, 54). The current definition also no longer calls for measuring pulmonary artery wedge pressure. However, even in the absence of a clinical risk factor, it is necessary to objectively rule out hydrostatic edema. This process may be assisted by bedside echocardiography or different methods of cardiac output measurement, but has not yet been strictly defined. This may be due to concerns about a diagnostic delay caused by awaiting the availability of expert echocardiographic measurements or cardiac output measurement devices. In addition, patients with transient fluid overload and oxygenation perturbations persisting only for a few hours with subsequent rapid clinical improvement may not be the target population for therapeutic clinical trials in ARDS, so that certain clinical study protocols require persistence of impaired oxygenation for a specified period prior to randomization (e.g., NCT03567577: clinical stability for 8 h and NCT04417036: persistence of a PaO2/FiO2 ratio < 300 for a minimum of 4 h).
3.4. Oxygenation
The Berlin Definition uses the ratio of PaO2/FiO2 to stratify ARDS severity into mild, moderate and severe, with ratios of 300–201, 200–101, and 100 or less, respectively (4). Apart from the partially refined criteria, as compared to the AECC definition, and eliminating the term “acute lung injury (ALI)” for mild ARDS, a key requirement for diagnosis is a minimum PEEP or, in mild ARDS, continuous positive airway pressure (CPAP), of 5 cmH2O. The rationale was that the PaO2/FiO2 ratio may vary according to ventilator settings, particularly PEEP (54).
As discussed previously, respiratory failure is initially managed with non-invasive interventions in clinical practice. This is a challenge to the effort to validly diagnose, categorize, and study ARDS for several reasons. In non-intubated patients, there is no definitive consensus on the PEEP achieved with HFNO, which has been recommended for managing mild and moderate ARDS (55). The median end expiratory nasopharyngeal pressure achieved with HFNO in healthy volunteers with closed mouths using flow rates of 40 and 60 l/min has been reported as 3.6 and 6.8 cmH2O, respectively (24). Opening the mouth virtually nullified this effect. Of note, using CPAP set to 4 cmH2O achieved a median nasopharyngeal pressure of 3.1 cmH2O.
Furthermore, Riviello and colleagues highlighted diagnostic limitations in low-resource countries (52). The possible scarcity of available blood gas analyzers and mechanical ventilators may be overcome by use of their Kigali modification of the Berlin criteria, which omits the PEEP criterion and assesses oxygenation using an SpO2/FiO2 index. Several SpO2-based indices have been proposed to that end. Such indices may facilitate and expedite ARDS diagnosis, correlate to PaO2/FiO2 ratio, especially if PEEP is applied, and have even been suggested by some authors to be predictive of outcomes (56–58). Nevertheless, FiO2 applied during non-invasive support may be inaccurate and overestimated due to leakage and gas mixture in the upper airways, bearing risks for bias when compared to intubated patients.
4. Overview of ARDS definitions used in clinical trials involving non-intubated patients
Various authors and study groups have employed different methods to address the issue of measuring the PaO2/FiO2 ratio to define ARDS in non-intubated patients. In several publications, the term ARDS has been omitted in favor of the more general description “acute (hypoxemic) respiratory failure” (30, 59). Data from such studies have been implemented in guidelines for the management of ARDS (55). One guideline on NIV resolved to cluster ARDS, pneumonia, and hypoxemic respiratory failure in one collective category (11).
A significant body of evidence that – partially – still informs guidelines, originates from the era of the AECC definition (8, 15). Other studies applied the definition of ALI or the AECC criteria in their patient selection after the publication of the Berlin criteria, thus circumventing the issue of a PEEP requirement (60).
Formally, the Berlin criteria only explicitly acknowledge a diagnosis of ARDS in patients receiving non-invasive respiratory support in the context of mild disease (4). A methodologic approach to fully comply with the Berlin criteria in all aspects except for this limitation was employed in a trial assessing early prone positioning in patients with moderate to severe ARDS on HFNO and NIV. To determine disease severity, patients received an initial treatment with PEEP of 5 cmH2O via NIV facemask for 30 min (FiO2 0.5) (12). A similar strategy for diagnosis and stratification was used in the LUNG-SAFE study (13, 61), a trial focused on a predefined sequence of conventional oxygen therapy, HFNO and NIV in patients with AHRF and ARDS (62), and a clinical study evaluating predictors of NIV failure (16).
Conversely, in a recent study assessing the effect of NIV on intubation rate, as compared to conventional oxygen therapy via Venturi mask in early mild pneumonia-associated ARDS, the PEEP criterion of the (otherwise employed) Berlin definition was consciously omitted (63). This strategy was chosen to avoid the dilemma of switching the control group back from a system delivering PEEP to a Venturi mask. The same method was employed in two recent studies evaluating intubation rates in patients with HFNO in acute respiratory failure and, specifically, the subsets of ARDS and COVID-19 ARDS (58).
Of note, Hultström and colleagues reported a poor predictive value of the PaO2/FiO2 ratio on HFNO for ARDS severity assessed on MV in a cohort of COVID-19 patients (64). While the ratios were similar when NIV and MV were compared, most patients showed improvements of the PaO2/FiO2 ratio when switched to MV. This may also explain the remarkably low mortality of seemingly moderate to severe ARDS found in a trial on HFNO in the early months of the pandemic (58).
5. Current research gaps and perspectives
The history of the ARDS definition is one of repeated reappraisal of evidence and reevaluation of contemporary methods. This section addresses current knowledge gaps that may be factored into a future revised definition of ARDS (Figure 1).
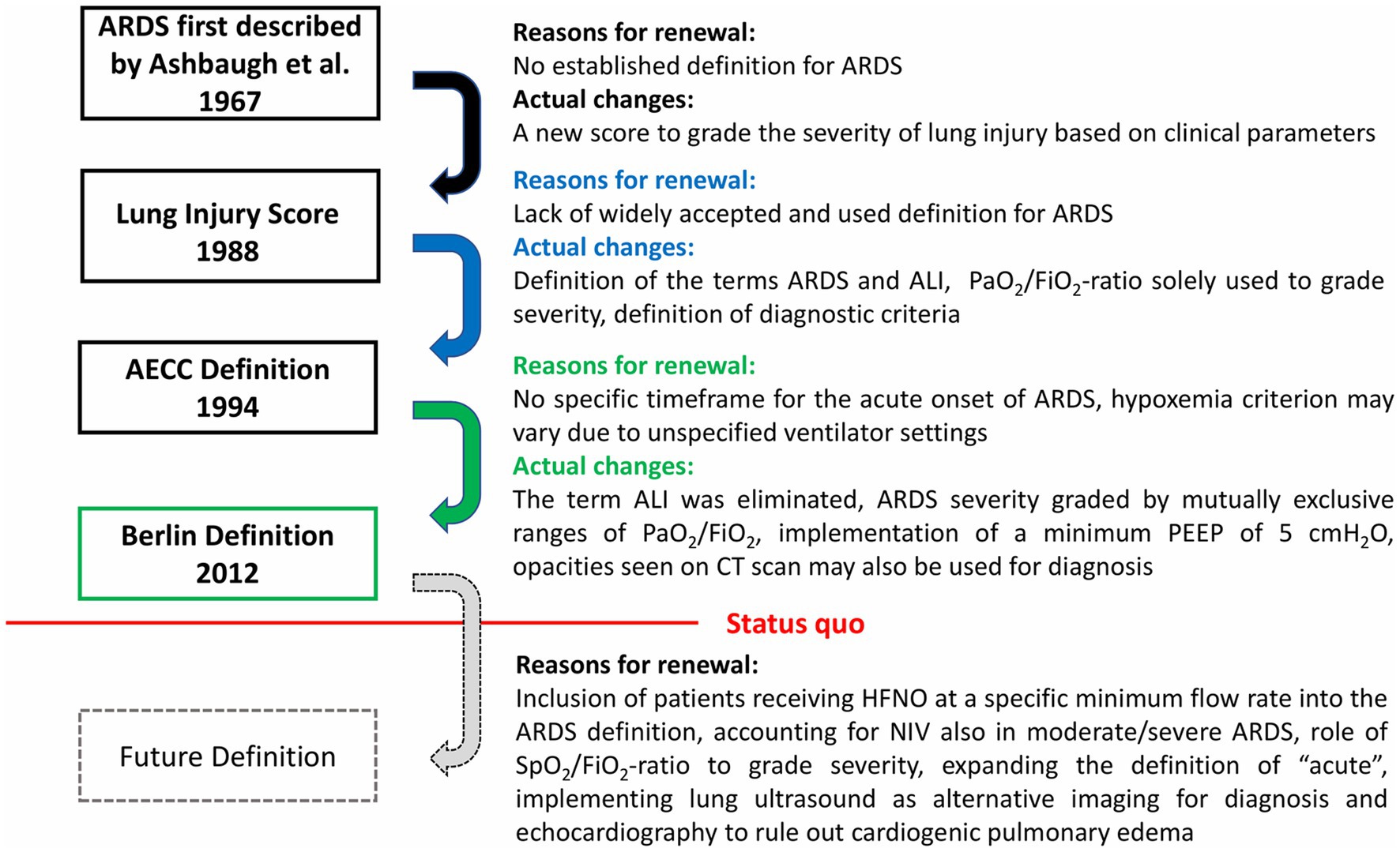
Figure 1. Questions arising in the development of ARDS definitions and future challenges. Multiple adaptations of the definition of acute respiratory distress syndrome (ARDS) have been implemented since its first description in 1967. Further changes leading to a new ARDS definition may arise from a growing body of evidence and constant evolution of clinical practice in acute respiratory failure. ALI, acute lung injury; PEEP, positive end-expiratory pressure; HFNO, high flow nasal oxygen therapy; NIV, non-invasive ventilation.
The dilemma of valid ARDS diagnosis in patients receiving HFNO has been addressed before, and has become more evident during the COVID-19 pandemic and the associated scarcity of resources (65). The latter has led to the widespread use of non-invasive ventilatory support in patients who would normally have been intubated and mechanically ventilated (14, 17, 18, 28, 58). Some authors have therefore suggested an expansion of the Berlin Definition to include patients receiving HFNO at a flow rate of at least 30 l/min (66). Considering experimental data showing that a median PEEP of ≥5 cmH2O was not achieved at a flow rate of 40 l/min, but could be achieved at a flow rate of 60 l/min in most patients, any such expansion would warrant discussion of this cutoff (24).
Evidence on the rationale for assessing ARDS severity on HFNO is equivocal. A switch from HFNO to MV may lead to significant improvements of the PaO2/FiO2 ratio in the range of 50–150 mmHg and thus, to a different classification of ARDS severity (64). Whether or not this observation is entirely linked to constant and direct application of PEEP with MV or lack thereof as well as inaccurate FiO2 under HFNO is uncertain. An additional finding of a trial assessing the pharyngeal end expiratory pressure under HFNO and CPAP was that a CPAP set to 4 cmH2O achieved a median end-expiratory pressure of 3.1 cmH2O (24). This observation may raise doubt as to whether a tight-fitting CPAP mask set to 5 cmH2O as described in the current ARDS definition could indeed achieve that pressure.
Other novel therapeutic approaches may also warrant reconsideration of our current definition of ARDS. While prone positioning of intubated patients with severe ARDS is now considered standard of care due to reduced mortality (67), awake prone positioning in non-intubated patients has more recently emerged as a promising therapeutic option during the COVID-19 pandemic (12, 68). Awake prone positioning has recently been shown to reduce mortality and improve oxygenation, although effects on other clinically relevant outcomes are less clear (69). In the light of these findings and the increasing use of this practice, future definitions of ARDS may be required to take such interventions into account in interpreting a PaO2/FiO2 ratio. For example, future research may address the question of whether severity is best defined according to baseline oxygenation indices or “best oxygenation achieved,” using a bundle of such maneuvers and non-invasive respiratory support methods.
The definition of ARDS severity based on the PaO2/FiO2 ratio alone provides a good predictive value for mortality, ranging from 27 to 32 and 45% in mild, moderate, and severe ARDS, respectively (4). This singular index may, however, only reflect one layer of a multi-facetted pathophysiology. For example, right ventricular (RV) dysfunction, a feature that frequently accompanies ARDS, is not reflected in the current classification, and has been linked to worse outcomes (70–72). Several mechanisms have been proposed how the RV is stressed in ARDS, even in patients receiving lung protective ventilation. Limiting the driving pressure appears to protect the RV (73), while permissive hypercapnia and conservative oxygenation targets may promote pulmonary vasoconstriction. High transpulmonary pressures caused by MV may lead to compression of the pulmonary vasculature, and vascular obstruction due to coagulopathy might further increase RV afterload (74). Consecutively, reduced preload and cardiac output in the systemic circulation as well as venous congestion, leading to edema and a reduced arterio-venous pressure gradient, may worsen systemic perfusion and contribute to multiorgan dysfunction in ARDS (75). Therefore, accounting for RV failure according to clearly validated criteria in future ARDS definitions with regards to disease severity may be prudent. Further clarification of the clinical relevance of the heart-lung-interplay in ARDS may broaden our understanding of potential benefits of early initiation of interventions, such as prone positioning, inhaled pulmonary vasodilators and extracorporeal life support and in this subgroup of patients (74, 76).
6. Conclusion
Further research in the respiratory care of ARDS outside invasive MV is required. The rapid developments in this field over the last years paired with, and accelerated by, the immense burden of disease of COVID-19-associated ARDS have exposed potential gaps in the applicability of our current ARDS definition. Addressing this issue may lead to faster identification and guideline-directed treatment of ARDS, ultimately offering the potential to improve outcomes. Thus, dedicated research informing a possible evidence-based expansion or update of the Berlin Definition is needed.
Author contributions
MZ and MW drafted the manuscript. FK and MW prepared the figure. MW prepared the table. SK, FK, RU, and KK assisted with literature search and reviewed and edited the manuscript. KK supervised the writing process. All authors contributed to the article and approved the submitted version.
Funding
KK reports a grant from Apeptico GmbH that was used in part for covering open access expenses. The funder was not involved in the design of the article, the collection of literature sources, the interpretation of data, the writing of this article, or the decision to submit it for publication.
Conflict of interest
KK reports travel expenses from Biotest GmbH and grants from Apeptico GmbH, Biotest GmbH, Alterras Therapeutics GmbH, and Bayer AG. RU reports travel expenses and honoraria from Biotest GmbH, grants from CCORE, a patent (EP 151897774, minimally invasive liquid lung), and holds equity in CCORE Technology, a medical device company that develops minimally invasive blood purification devices.
The remaining authors declare that the research was conducted in the absence of any commercial or financial relationships that could be construed as a potential conflict of interest.
Publisher’s note
All claims expressed in this article are solely those of the authors and do not necessarily represent those of their affiliated organizations, or those of the publisher, the editors and the reviewers. Any product that may be evaluated in this article, or claim that may be made by its manufacturer, is not guaranteed or endorsed by the publisher.
References
1. Thompson, BT, Chambers, RC, and Liu, KD. Acute respiratory distress syndrome. N Engl J Med. (2017) 377:562–72. doi: 10.1056/NEJMra1608077
2. Ashbaugh, DG, Bigelow, DB, and Levine, BE. Acute respiratory distress in adults. Lancet. (1967) 290:319–23. doi: 10.1016/S0140-6736(67)90168-7
3. Murray, JF, Matthay, MA, Luce, JM, and Flick, MR. An expanded definition of the adult respiratory distress syndrome. Am Rev Respir Dis. (1988) 138:720–3. doi: 10.1164/ajrccm/138.3.720
4. The ARDS Definition Task Force. Acute respiratory distress syndrome: the Berlin definition. JAMA. (2012) 307:2526–33. doi: 10.1001/jama.2012.5669
5. Bernard, GR, Artigas, A, Brigham, KL, Carlet, J, Falke, K, Hudson, L, et al. Definitions, mechanisms, relevant outcomes, and clinical trial coordination. Am J Respir Crit Care Med. (1994) 149:818–24. doi: 10.1164/ajrccm.149.3.7509706
6. Tasaka, S, Ohshimo, S, Takeuchi, M, Yasuda, H, Ichikado, K, Tsushima, K, et al. ARDS Clinical Practice Guideline 2021. J Intensive Care. (2022) 10:32. doi: 10.1186/s40560-022-00615-6
7. Gottlieb, J, Capetian, P, Hamsen, U, Janssens, U, Karagiannidis, C, Kluge, S, et al. S3-Leitlinie Sauerstoff in der Akuttherapie beim Erwachsenen [German S3 guideline - oxygen therapy in the acute Care of Adult Patients]. Pneumologie. (2022) 76:159–216. doi: 10.1055/a-1554-2625
8. Antonelli, M, Conti, G, Rocco, M, Bufi, M, De Blasi, RA, Vivino, G, et al. A comparison of noninvasive positive-pressure ventilation and conventional mechanical ventilation in patients with acute respiratory failure. N Engl J Med. (1998) 339:429–35. doi: 10.1056/NEJM199808133390703
9. Grieco, DL, Maggiore, SM, Roca, O, Spinelli, E, Patel, BK, Thille, AW, et al. Non-invasive ventilatory support and high-flow nasal oxygen as first-line treatment of acute hypoxemic respiratory failure and ARDS. Intensive Care Med. (2021) 47:851–66. doi: 10.1007/s00134-021-06459-2
10. Chaudhuri, D, Jinah, R, Burns, KEA, Angriman, F, Ferreyro, BL, Munshi, L, et al. Helmet noninvasive ventilation compared to facemask noninvasive ventilation and high-flow nasal cannula in acute respiratory failure: a systematic review and meta-analysis. Eur Respir J. (2022) 59:2101269. doi: 10.1183/13993003.01269-2021
11. Rochwerg, B, Brochard, L, Elliott, MW, Hess, D, Hill, NS, Nava, S, et al. Official ERS/ATS clinical practice guidelines: noninvasive ventilation for acute respiratory failure. Eur Respir J. (2017) 50:1602426. doi: 10.1183/13993003.02426-2016
12. Ding, L, Wang, L, Ma, W, and He, H. Efficacy and safety of early prone positioning combined with HFNC or NIV in moderate to severe ARDS: a multi-center prospective cohort study. Crit Care. (2020) 24:28. doi: 10.1186/s13054-020-2738-5
13. Bellani, G, Laffey, JG, Pham, T, Madotto, F, Fan, E, Brochard, L, et al. Noninvasive ventilation of patients with acute respiratory distress syndrome. Insights from the LUNG SAFE study. Am J Respir Crit Care Med. (2017) 195:67–77. doi: 10.1164/rccm.201606-1306OC
14. Papoutsi, E, Giannakoulis, VG, Xourgia, E, Routsi, C, Kotanidou, A, and Siempos, II. Effect of timing of intubation on clinical outcomes of critically ill patients with COVID-19: a systematic review and meta-analysis of non-randomized cohort studies. Crit Care. (2021) 25:121. doi: 10.1186/s13054-021-03540-6
15. Antonelli, M, Conti, G, Moro, M, Esquinas, A, Gonzalez-Diaz, G, Confalonieri, M, et al. Predictors of failure of noninvasive positive pressure ventilation in patients with acute hypoxemic respiratory failure: a multi-center study. Intensive Care Med. (2001) 27:1718–28. doi: 10.1007/s00134-001-1114-4
16. Carteaux, G, Millán-Guilarte, T, De Prost, N, Razazi, K, Abid, S, Thille, AW, et al. Failure of noninvasive ventilation for De novo acute hypoxemic respiratory failure: role of tidal volume. Crit Care Med. (2016) 44:282–90. doi: 10.1097/CCM.0000000000001379
17. Menga, LS, Cese, LD, Bongiovanni, F, Lombardi, G, Michi, T, Luciani, F, et al. High failure rate of noninvasive oxygenation strategies in critically ill subjects with acute hypoxemic respiratory failure due to COVID-19. Respir Care. (2021) 66:705–14. doi: 10.4187/respcare.08622
18. Battaglini, D, Robba, C, Ball, L, Silva, PL, Cruz, FF, Pelosi, P, et al. Noninvasive respiratory support and patient self-inflicted lung injury in COVID-19: a narrative review. Br J Anaesth. (2021) 127:353–64. doi: 10.1016/j.bja.2021.05.024
19. Weaver, L, Das, A, Saffaran, S, Yehya, N, Scott, TE, Chikhani, M, et al. High risk of patient self-inflicted lung injury in COVID-19 with frequently encountered spontaneous breathing patterns: a computational modelling study. Ann Intensive Care. (2021) 11:109. doi: 10.1186/s13613-021-00904-7
20. Hernández, G, Vaquero, C, Colinas, L, Cuena, R, González, P, Canabal, A, et al. Effect of postextubation high-flow nasal cannula vs noninvasive ventilation on reintubation and postextubation respiratory failure in high-risk patients: a randomized clinical trial. JAMA. (2016) 316:1565–74. doi: 10.1001/jama.2016.14194
21. Thille, AW, Muller, G, Gacouin, A, Coudroy, R, Decavèle, M, Sonneville, R, et al. Effect of postextubation high-flow nasal oxygen with noninvasive ventilation vs high-flow nasal oxygen alone on reintubation among patients at high risk of extubation failure: a randomized clinical trial. JAMA. (2019) 322:1465–75. doi: 10.1001/jama.2019.14901
22. Rittayamai, N, Grieco, DL, and Brochard, L. Noninvasive respiratory support in intensive care medicine. Intensive Care Med. (2022) 48:1211–4. doi: 10.1007/s00134-022-06762-6
23. Corley, A, Caruana, LR, Barnett, AG, Tronstad, O, and Fraser, JF. Oxygen delivery through high-flow nasal cannulae increase end-expiratory lung volume and reduce respiratory rate in post-cardiac surgical patients. Br J Anaesth. (2011) 107:998–1004. doi: 10.1093/bja/aer265
24. Vieira, F, Bezerra, FS, Coudroy, R, Schreiber, A, Telias, I, Dubo, S, et al. High-flow nasal cannula compared with continuous positive airway pressure: a bench and physiological study. J Appl Physiol. (2022) 132:1580–90. doi: 10.1152/japplphysiol.00416.2021
25. Dysart, K, Miller, TL, Wolfson, MR, and Shaffer, TH. Research in high flow therapy: mechanisms of action. Respir Med. (2009) 103:1400–5. doi: 10.1016/j.rmed.2009.04.007
26. Ni, Y-N, Luo, J, Yu, H, Liu, D, Ni, Z, Cheng, J, et al. Can high-flow nasal cannula reduce the rate of endotracheal intubation in adult patients with acute respiratory failure compared with conventional oxygen therapy and noninvasive positive pressure ventilation? Chest. (2017) 151:764–75. doi: 10.1016/j.chest.2017.01.004
27. Messika, J, Ben Ahmed, K, Gaudry, S, Miguel-Montanes, R, Rafat, C, Sztrymf, B, et al. Use of high-flow nasal cannula oxygen therapy in subjects with ARDS: a 1-year observational study. Respir Care. (2015) 60:162–9. doi: 10.4187/respcare.03423
28. Nasa, P, Azoulay, E, Khanna, AK, Jain, R, Gupta, S, Javeri, Y, et al. Expert consensus statements for the management of COVID-19-related acute respiratory failure using a Delphi method. Crit Care. (2021) 25:106. doi: 10.1186/s13054-021-03491-y
29. Rochwerg, B, Granton, D, Wang, DX, Helviz, Y, Einav, S, Frat, JP, et al. High flow nasal cannula compared with conventional oxygen therapy for acute hypoxemic respiratory failure: a systematic review and meta-analysis. Intensive Care Med. (2019) 45:563–72. doi: 10.1007/s00134-019-05590-5
30. Frat, J-P, Thille, AW, Mercat, A, Girault, C, Ragot, S, Perbet, S, et al. High-flow oxygen through nasal cannula in acute hypoxemic respiratory failure. N Engl J Med. (2015) 372:2185–96. doi: 10.1056/NEJMoa1503326
31. Lewis, SR, Baker, PE, Parker, R, and Smith, AF. High-flow nasal cannulae for respiratory support in adult intensive care patients. Cochrane Database Syst Rev. (2021) 2021:CD010172. doi: 10.1002/14651858.CD010172.pub3
32. Oczkowski, S, Ergan, B, Bos, L, Chatwin, M, Ferrer, M, Gregoretti, C, et al. ERS clinical practice guidelines: high-flow nasal cannula in acute respiratory failure. Eur Respir J. (2022) 59:2101574. doi: 10.1183/13993003.01574-2021
33. Rochwerg, B, Einav, S, Chaudhuri, D, Mancebo, J, Mauri, T, Helviz, Y, et al. The role for high flow nasal cannula as a respiratory support strategy in adults: a clinical practice guideline. Intensive Care Med. (2020) 46:2226–37. doi: 10.1007/s00134-020-06312-y
34. LUNG SAFE Investigators and the ESICM Trials GroupCortegiani, A, Madotto, F, Gregoretti, C, Bellani, G, Laffey, JG, et al. Immunocompromised patients with acute respiratory distress syndrome: secondary analysis of the LUNG SAFE database. Crit Care. (2018) 22:157. doi: 10.1186/s13054-018-2079-9
35. Azoulay, E, Mokart, D, Kouatchet, A, Demoule, A, and Lemiale, V. Acute respiratory failure in immunocompromised adults. Lancet Respir Med. (2019) 7:173–86. doi: 10.1016/S2213-2600(18)30345-X
36. Cortegiani, A, Crimi, C, Sanfilippo, F, Noto, A, Di Falco, D, Grasselli, G, et al. High flow nasal therapy in immunocompromised patients with acute respiratory failure: a systematic review and meta-analysis. J Crit Care. (2019) 50:250–6. doi: 10.1016/j.jcrc.2018.12.015
37. Dumas, G, Lemiale, V, Rathi, N, Cortegiani, A, Pène, F, Bonny, V, et al. Survival in immunocompromised patients ultimately requiring invasive mechanical ventilation: a pooled individual patient data analysis. Am J Respir Crit Care Med. (2021) 204:187–96. doi: 10.1164/rccm.202009-3575OC
38. Acute Respiratory Distress Syndrome NetworkBrower, RG, Matthay, MA, Morris, A, Schoenfeld, D, Thompson, BT, et al. Ventilation with lower tidal volumes as compared with traditional tidal volumes for acute lung injury and the acute respiratory distress syndrome. N Engl J Med. (2000) 342:1301–8. doi: 10.1056/NEJM200005043421801
39. Amato, MBP, Meade, MO, Slutsky, AS, Brochard, L, Costa, ELV, Schoenfeld, DA, et al. Driving pressure and survival in the acute respiratory distress syndrome. N Engl J Med. (2015) 372:747–55. doi: 10.1056/NEJMsa1410639
40. Papazian, L, Aubron, C, Brochard, L, Chiche, J-D, Combes, A, Dreyfuss, D, et al. Formal guidelines: management of acute respiratory distress syndrome. Ann Intensive Care. (2019) 9:69. doi: 10.1186/s13613-019-0540-9
41. Yang, X, Yu, Y, Xu, J, Shu, H, Xia, J, Liu, H, et al. Clinical course and outcomes of critically ill patients with SARS-CoV-2 pneumonia in Wuhan, China: a single-centered, retrospective, observational study. Lancet Respir Med. (2020) 8:475–81. doi: 10.1016/S2213-2600(20)30079-5
42. Pham, T, Pesenti, A, Bellani, G, Rubenfeld, G, Fan, E, Bugedo, G, et al. Outcome of acute hypoxaemic respiratory failure: insights from the LUNG SAFE study. Eur Respir J. (2021) 57:2003317. doi: 10.1183/13993003.03317-2020
43. Testa, A, Soldati, G, Copetti, R, Giannuzzi, R, Portale, G, and Gentiloni-Silveri, N. Early recognition of the 2009 pandemic influenza a (H1N1) pneumonia by chest ultrasound. Crit Care. (2012) 16:R30. doi: 10.1186/cc11201
44. Chen, W-T, Wang, H-Y, Ye, Q-H, Tang, M, and Wang, Y-W. The diagnostic values of lung ultrasound for ARDS. Eur Rev Med Pharmacol Sci. (2022) 26:6642–6. doi: 10.26355/eurrev_202209_29764
45. Lazzeri, C, Bonizzoli, M, Batacchi, S, Socci, F, Matucci-Cerinic, M, and Peris, A. Combined lung and cardiac ultrasound in COVID-related acute respiratory distress syndrome. Intern Emerg Med. (2021) 16:1779–85. doi: 10.1007/s11739-021-02646-7
46. Dell’Aquila, P, Raimondo, P, Racanelli, V, De Luca, P, De Matteis, S, Pistone, A, et al. Integrated lung ultrasound score for early clinical decision-making in patients with COVID-19: results and implications. Ultrasound J. (2022) 14:21. doi: 10.1186/s13089-022-00264-8
47. Bouhemad, B, Mongodi, S, Via, G, and Rouquette, I. Ultrasound for “lung monitoring” of ventilated patients. Anesthesiology. (2015) 122:437–47. doi: 10.1097/ALN.0000000000000558
48. Zieleskiewicz, L, Markarian, T, Lopez, A, Taguet, C, Mohammedi, N, Boucekine, M, et al. Comparative study of lung ultrasound and chest computed tomography scan in the assessment of severity of confirmed COVID-19 pneumonia. Intensive Care Med. (2020) 46:1707–13. doi: 10.1007/s00134-020-06186-0
49. Wolstenhulme, S, and McLaughlan, JR. Lung ultrasound education: simulation and hands-on. Br J Radiol. (2021) 94:20200755. doi: 10.1259/bjr.20200755
50. Sun, Z, Zhang, Z, Liu, J, Song, Y, Qiao, S, Duan, Y, et al. Lung ultrasound score as a predictor of mortality in patients with COVID-19. Front Cardiovasc Med. (2021) 8:633539. doi: 10.3389/fcvm.2021.633539
51. Salzmann, C, Schmidt, C, Sauvigny, D, Janneck, M, and Lock, G. Thoraxsonografie bei Aufnahme auf einer COVID-Verdachtsstation – differenzialdiagnostisch hilfreich oder Zeitverschwendung? Pneumologie. (2021) 75:856–63. doi: 10.1055/a-1502-8844
52. Riviello, ED, Kiviri, W, Twagirumugabe, T, Mueller, A, Banner-Goodspeed, VM, Officer, L, et al. Hospital incidence and outcomes of the acute respiratory distress syndrome using the Kigali modification of the Berlin definition. Am J Respir Crit Care Med. (2016) 193:52–9. doi: 10.1164/rccm.201503-0584OC
53. Hatch, HB, and Carrera, GM. Congestive atelectasis: a fatal postoperative complication. J Thorac Surg. (1959) 37:257–60. doi: 10.1016/S0096-5588(20)30090-8
54. Ferguson, ND, Fan, E, Camporota, L, Antonelli, M, Anzueto, A, Beale, R, et al. The Berlin definition of ARDS: an expanded rationale, justification, and supplementary material. Intensive Care Med. (2012) 38:1573–82. doi: 10.1007/s00134-012-2682-1
55. Vosteen, K-H. Arbeitsgemeinschaft der Wissenschaftlichen Medizinischen Fachgesellschaften (AWMF). Unfallchirurgie. (1992) 18:55–6. doi: 10.1007/BF02588242
56. Wick, KD, Matthay, MA, and Ware, LB. Pulse oximetry for the diagnosis and management of acute respiratory distress syndrome. Lancet Respir Med. (2022) 10:1086–98. doi: 10.1016/S2213-2600(22)00058-3
57. Rice, TW, Wheeler, AP, Bernard, GR, Hayden, DL, Schoenfeld, DA, and Ware, LB. Comparison of the SpO2/FIO 2 ratio and the PaO2/FIO 2 ratio in patients with acute lung injury or ARDS. Chest. (2007) 132:410–7. doi: 10.1378/chest.07-0617
58. Panadero, C, Abad-Fernández, A, Rio-Ramírez, MT, Acosta Gutiérrez, CM, Calderón-Alcalá, M, López-Riolobos, C, et al. High-flow nasal cannula for acute respiratory distress syndrome (ARDS) due to COVID-19. Multidiscip Respir Med. (2020) 15:693. doi: 10.4081/mrm.2020.693
59. Azevedo, J, Montenegro, W, Leitao, A, Silva, M, Prazeres, J, and Maranhao, J. High flow nasal cannula oxygen (hfnc) versus non-invasive positive pressure ventilation (nippv) in acute hypoxemic respiratory failure. A pilot randomized controlled trial. Intensive Care Med Exp. (2015) 3:A166. doi: 10.1186/2197-425X-3-S1-A166
60. Zhan, Q, Sun, B, Liang, L, Yan, X, Zhang, L, Yang, J, et al. Early use of noninvasive positive pressure ventilation for acute lung injury: a multicenter randomized controlled trial. Crit Care Med. (2012) 40:455–60. doi: 10.1097/CCM.0b013e318232d75e
61. Bellani, G, Laffey, JG, Pham, T, Fan, E, Brochard, L, Esteban, A, et al. Epidemiology, patterns of care, and mortality for patients with acute respiratory distress syndrome in intensive care units in 50 countries. JAMA. (2016) 315:788–800. doi: 10.1001/jama.2016.0291
62. Frat, J-P, Brugiere, B, Ragot, S, Chatellier, D, Veinstein, A, Goudet, V, et al. Sequential application of oxygen therapy via high-flow nasal cannula and noninvasive ventilation in acute respiratory failure: an observational pilot study. Respir Care. (2015) 60:170–8. doi: 10.4187/respcare.03075
63. He, H, Sun, B, Liang, L, Li, Y, Wang, H, et al. A multicenter RCT of noninvasive ventilation in pneumonia-induced early mild acute respiratory distress syndrome. Crit Care. (2019) 23:1–13. doi: 10.1186/s13054-019-2575-6
64. Hultström, M, Hellkvist, O, Covaciu, L, Fredén, F, Frithiof, R, Lipcsey, M, et al. Limitations of the ARDS criteria during high-flow oxygen or non-invasive ventilation: evidence from critically ill COVID-19 patients. Crit Care. (2022) 26:55. doi: 10.1186/s13054-022-03933-1
65. Sen-Crowe, B, Sutherland, M, McKenney, M, and Elkbuli, A. A closer look into global hospital beds capacity and resource shortages during the COVID-19 pandemic. J Surg Res. (2021) 260:56–63. doi: 10.1016/j.jss.2020.11.062
66. Matthay, MA, Thompson, BT, and Ware, LB. The Berlin definition of acute respiratory distress syndrome: should patients receiving high-flow nasal oxygen be included? Lancet Respir Med. (2021) 9:933–6. doi: 10.1016/S2213-2600(21)00105-3
67. Guérin, C, Reignier, J, Richard, J-C, Beuret, P, Gacouin, A, Boulain, T, et al. Prone positioning in severe acute respiratory distress syndrome. N Engl J Med. (2013) 368:2159–68. doi: 10.1056/NEJMoa1214103
68. Fazzini, B, Page, A, Pearse, R, and Puthucheary, Z. Prone positioning for non-intubated spontaneously breathing patients with acute hypoxaemic respiratory failure: a systematic review and meta-analysis. Br J Anaesth. (2022) 128:352–62. doi: 10.1016/j.bja.2021.09.031
69. Cardona, S, Downing, J, Alfalasi, R, Bzhilyanskaya, V, Milzman, D, Rehan, M, et al. Intubation rate of patients with hypoxia due to COVID-19 treated with awake proning: a meta-analysis. Am J Emerg Med. (2021) 43:88–96. doi: 10.1016/j.ajem.2021.01.058
70. Zochios, V, Parhar, K, Tunnicliffe, W, Roscoe, A, and Gao, F. The right ventricle in ARDS. Chest. (2017) 152:181–93. doi: 10.1016/j.chest.2017.02.019
71. Chotalia, M, Ali, M, Alderman, JE, Patel, JM, Parekh, D, and Bangash, MN. Cardiovascular subphenotypes in patients with COVID -19 pneumonitis whose lungs are mechanically ventilated: a single-centre retrospective observational study. Anaesthesia. (2022) 77:763–71. doi: 10.1111/anae.15700
72. Dugar, S, Sato, R, Zochios, V, Duggal, A, and Vallabhajosyula, S. Defining right ventricular dysfunction in acute respiratory distress syndrome. J Cardiothorac Vasc Anesth. (2022) 36:632–4. doi: 10.1053/j.jvca.2021.09.001
73. Mekontso Dessap, A, Boissier, F, Charron, C, Bégot, E, Repessé, X, Legras, A, et al. Acute cor pulmonale during protective ventilation for acute respiratory distress syndrome: prevalence, predictors, and clinical impact. Intensive Care Med. (2016) 42:862–70. doi: 10.1007/s00134-015-4141-2
74. Isgro, G, Yusuff, HO, and Zochios, V. The right ventricle in COVID-19 lung injury: proposed mechanisms, management, and research gaps. J Cardiothorac Vasc Anesth. (2021) 35:1568–72. doi: 10.1053/j.jvca.2021.01.014
75. Zochios, V, Yusuff, H, and Schmidt, M. On behalf of protecting the right ventricle network (PRORVnet). Acute right ventricular injury phenotyping in ARDS. Intensive Care Med. (2022) 49:99–102. doi: 10.1007/s00134-022-06904-w
Keywords: hypoxia, non-invasive ventilation, high-flow nasal cannula, high-flow nasal oxygen, acute respiratory failure, Berlin definition, acute respiratory distress syndrome
Citation: Zbiral M, Weber M, König S, Kraft F, Ullrich R and Krenn K (2023) Usefulness and limitations of the acute respiratory distress syndrome definitions in non-intubated patients. A narrative review. Front. Med. 10:1088709. doi: 10.3389/fmed.2023.1088709
Edited by:
Tobias Kammerer, University Hospital of Cologne, GermanyReviewed by:
Driss Laghlam, Clinique Ambroise Paré, FranceCopyright © 2023 Zbiral, Weber, König, Kraft, Ullrich and Krenn. This is an open-access article distributed under the terms of the Creative Commons Attribution License (CC BY). The use, distribution or reproduction in other forums is permitted, provided the original author(s) and the copyright owner(s) are credited and that the original publication in this journal is cited, in accordance with accepted academic practice. No use, distribution or reproduction is permitted which does not comply with these terms.
*Correspondence: Katharina Krenn, ✉ a2F0aGFyaW5hLmtyZW5uQG1lZHVuaXdpZW4uYWMuYXQ=