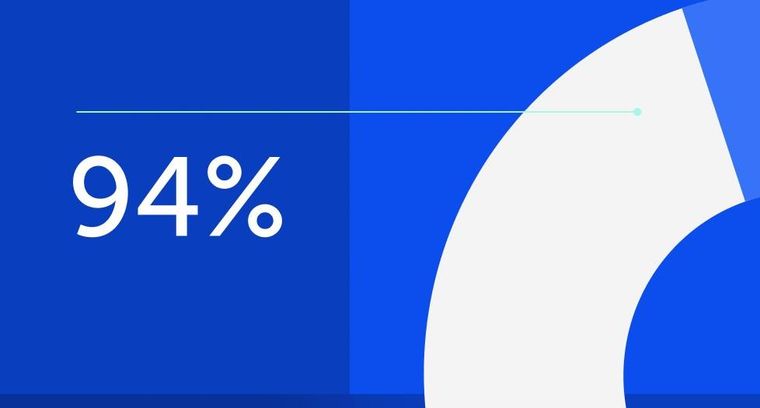
94% of researchers rate our articles as excellent or good
Learn more about the work of our research integrity team to safeguard the quality of each article we publish.
Find out more
ORIGINAL RESEARCH article
Front. Med., 04 August 2023
Sec. Gastroenterology
Volume 10 - 2023 | https://doi.org/10.3389/fmed.2023.1087715
This article is part of the Research TopicGastrointestinal Microbiota Crosstalk with the Host’s Metabolism: the Role of Microbiota-Modulating AgentsView all 7 articles
Introduction: Antibiotic effects on gut bacteria have been widely studied, but very little is known about the consequences of such treatments on the mycobiota, the fungal part of the microbiota and how the length of administration influences both microbiota. Here, we examined the effect of antibiotics (ATB) on the composition of bacterial and fungal microbiota and how the administration of Saccharomyces boulardii CNCM I-745 influences both microbiota.
Methods: In order to get closer to the human microbiota, the mice used in this study were subjected to fecal microbiota transfer (FMT) using human feces and subsequently called human microbiotaassociated (HMA) mice. These mice were then treated with amoxicillinclavulanate antibiotics and supplemented with S. boulardii during and after ATB treatment to understand the effect of the yeast probiotic on both bacterial and fungal microbiota. Bacterial and fungal microbiota analyses were done using 16S and ITS2 rRNA amplicon-based sequencing.
Results: We showed that the administration of S. boulardii during ATB treatment had very limited effect on the fungal populations on the long term, once the yeast probiotic has been cleared from the gut. Concerning bacterial microbiota, S. boulardii administration allowed a better recovery of bacterial populations after the end of the ATB treatment period. Additionally, 16S and ITS2 rRNA sequence analysis revealed that 7 additional days of S. boulardii administration (17 days in total) enhanced the return of the initial bacterial equilibrium.
Discussion: In this study, we provide a comprehensive analysis of how probiotic yeast administration can influence the fungal and bacterial microbiota in a model of broad-spectrum antibiotherapy.
The gut microbiota has been widely studied for the last two decades, and the development of next-generation sequencing (NGS) has allowed the identification of its pivotal role in various pathologies in recent years. Indeed, the gut microbiota has been identified as a cofactor in diseases of the digestive tract, such as inflammatory bowel disease (IBD) and colorectal cancer (CRC), as well as in diseases indirectly related to the gut, such as allergies, diabetes, obesity and liver diseases (1–8).
Probiotics are living, active organisms that have beneficial effects on the health of the host and can be found in fermented foods, dietary supplements, and even drugs (9). They can be used to improve gastrointestinal disorders, such as diarrhea (infectious and antibiotic-associated), necrotizing enterocolitis, Clostridioides difficile infection, IBD (ulcerative colitis and Crohn’s disease) and irritable bowel syndrome (IBS) (10, 11). There are several probiotic products, most of which belong to the group of lactic acid-producing bacteria, but very few are yeasts. The few that are available belong to the Saccharomyces genus (e.g., S. cerevisiae and S. boulardii). However, S. boulardii CNCM I-745 was the first one to be discovered and is widely used throughout the world. Discovered a century ago in 1920, S. boulardii has been shown to prevent and reduce the occurrence of diarrhea associated with antibiotic treatment (12–14). It also exerts protective properties in the treatment of Clostridioides difficile and Helicobacter infections (15–17). It has also been identified as protective in mouse models of IBD and IBS (18, 19).
Antibiotics are used in humans to treat a large number of infections with rather high efficacy. However, they also disrupt intestinal microorganisms, and dysbiosis can be detected long after the end of antibiotic treatment (20). The effects of antibiotics on the bacterial microbiota are particularly well described, but very little is known about their impact on the fungal microbiota (mycobiota).
Kabbani and colleagues studied the effect of the combination of Saccharomyces boulardii CNCM I-745 and amoxicillin-clavulanate on the gut microbiota in humans and reported a milder effect on microbiota shifts in the presence of S. boulardii, including less overgrowth of Escherichia and a reduction in antibiotic-associated diarrhea (13). However, this study, like the majority of research on the gut microbiota, focused solely on the bacterial component, while other members of the gut microbiota, such as fungi, viruses and archaea, were neglected.
The objectives of this study were to evaluate the effects of an antibiotic (amoxicillin-clavulanate – ATB) and the probiotic Saccharomyces boulardii CNCM I-745 on the gut fungal and bacterial microbiota in HMA mice. We chose the amoxicillin-clavulanate since it is one of the most commonly used antibiotics in the primary care setting (21). We also examined the impact of S. boulardii administration after the end of ATB treatment. S. boulardii was able to attenuate the dysbiosis associated with ATB when administered during treatment and maintain a higher diversity when administered continuously during and after ATB treatment.
Therefore, this study will help us to understand the effect of S. boulardii CNCM I-745 administration during ATB treatment on both bacterial and fungal microbiota to improve medical practices in the future.
Six-week-old female C57BL/6 J mice were purchased from Janvier Laboratory (Le Genest, France) and used 1 week after delivery. Animals were kept in humidity- and temperature-controlled rooms under a 12 h light–dark cycle and had access to a chow diet and water ad libitum. Mice were grouped by types of treatment in cages with a maximum of 4 mice per cages as follow (Figure 1):
Figure 1. Effect of Saccharomyces boulardii supplementation during antibiotic treatment on the mycobiota. (A) Setup of the entire experiment to decipher the effect of the yeast probiotic S. boulardii on the fungal and bacterial microbiota after antibiotic treatment (ATB). Group (Grp) 1: HMA mice treated with the Vehicle for 16 days, n = 8; Grp 2: HMA mice treated with the ATB for 10 days, then Vehicle for the following 6 days, n = 8; Grp 3: HMA mice treated with S. boulardii for 23 days, then Vehicle for the following 7 days, n = 8; Grp 4: HMA mice treated with the ATB and S. boulardii for 10 days, then Vehicle for the following 13 days, n = 8; and Grp 5: HMA mice treated with the ATB and S. boulardii for 10 days, then S. boulardii for the following 13 days, then Vehicle for the following 7 days, n = 8. (B–E) Human microbiota-associated (HMA) mice treated with the ATB or ATB plus S. boulardii for 10 days: Grp 2 “ATB” n = 8; Grp 4 and 5 “ATB + S. boulardii” n = 16 (Grp 4 n = 8, Grp 5 n = 8), the experiment was performed 2 times. (B) Experimental design for the administration of the ATB and probiotic over 10 days. (C) α–Diversity of the fungal microbiota (ITS2 rRNA) in the fecal microbiota, described by observed species and Shannon and Simpson indexes. (D,E) Taxa selected with the differential abundance analysis based on the negative binomial distribution (DESeq2) with padj <0.05.
Group (Grp) 1: HMA mice treated with the Vehicle for 16 days, n = 8; Grp 2: HMA mice treated with the ATB for 10 days, then Vehicle for the following 6 days, n = 8; Grp 3: HMA mice treated with S. boulardii for 23 days, then Vehicle for the following 7 days, n = 8; Grp 4: HMA mice treated with the ATB and S. boulardii for 10 days, then Vehicle for the following 13 days, n = 8; and Grp 5: HMA mice treated with the ATB and S. boulardii for 10 days, then S. boulardii for the following 13 days, then Vehicle for the following 7 days, n = 8. Depending on the question the feces from each group were collected at different time points identified in each figure. As Grp 4 and 5 received the exact same treatment till day 10 (ATB plus S. boulardii), the 4 cages of Grp 4 and 5 were identical for our analysis till day 10. Considering this, we decided to increase the size of the group treated with ATB and S. boulardii at day 10 from 8 to 16 by using all mice from Grp 4 and Grp 5 collected in all 4 cages.
Every experiment was repeated two times, so a total of 40 mice were used in the results presented in this study but 80 were used for the repetition of the experiments. All experiments were performed in accordance with the ethics committee “Comité d’Ethique en Experimentation Animale” (COMETHEA C2EA – 45, Jouy en Josas, France).
Mice received feces from a healthy donor as previously described (22). Briefly, feces from humans were collected and immediately stored at 4°C in an anaerobiosis generator (Genbox, Biomérieux, Capronne, France) to favor the preservation of anaerobic bacteria. Samples were processed within 24 h in a Coy chamber. The feces were rapidly diluted 100-fold in brain heart infusion (BHI, Becton Dickinson, Franklin Lakes, United States) supplemented with 0.5 mg/mL L-cysteine (Sigma–Aldrich, St. Louis, MO, United States) and 20% skim milk (Becton Dickinson, Franklin Lakes, United States) (vol/vol) and stored in aliquots at −80°C. This ready-to-use fecal suspension was used for FMT to the mice.
Mice were fasted for 1 h and then subjected to bowel cleansing by oral-gastric gavage with PEG (polyethylene glycol, Macrogol 4,000, Fortrans, Ipsen Pharma, France). Bowel cleansing with PEG was performed only once. 4 hours later, mice received human feces by oral gastric gavage (350 μL of resuspended feces prepared as described above). Mice were then allowed free access to food and water. FMT was repeated once a week for 3 weeks before the antibiotic was given by oral gavage without any PEG treatment.
Amoxicillin-clavulanate (5,1, 150 mg/kg, Sandoz, Bale, Switzerland) was resuspended in NaCl and administered to mice daily by intragastric gavage for 10 days. Concentration of the mice ATB treatment was determined using the quantity per kilogram prescribed to human.
Saccharomyces boulardii CNCM I-745 (Biocodex, Gentilly, France) was used in this study. Lyophilized yeast was resuspended in Vehicle (60 mg/day/mouse) and directly administered by 200 μL intragastric gavage during and after the ATB treatment. The control group was administered 200 μL of Vehicle daily.
Fecal samples were collected in the morning and frozen for microbiota analysis. Mice were individually placed in a sterile container to defecate and fecal pellets were collected using sterile tips and placed in a sterile 1.5 mL tube for immediate freezing in dry ice. All samples were stored at −80°C until use.
Fecal total DNA was extracted from weighed content samples as previously described, with modifications (23). After nucleic acid precipitation with isopropanol, DNA suspensions were incubated overnight at 4°C and centrifuged at 20,000 × g for 30 min. The supernatants were transferred to a new tube containing 2 μL of RNase (RNase A, 10 mg/mL; EN0531; Fermentas, Villebon sur Yvette, France) and incubated at 37°C for 30 min. Nucleic acids were precipitated by the addition of 1 mL of absolute ethanol and 50 μL of 3 M sodium acetate and centrifuged at 20,000 × g for 10 min. The DNA pellets were washed with 70% ethanol 3 times, dried and resuspended in 100 μL of Tris-EDTA (TE) buffer (10 mM Tris–HCl, 1 mM EDTA, adjusted pH 8).
The DNA suspensions were stored at −20°C for real-time qPCR analysis of the 16S or ITS2 rRNA sequences analyses.
Bacterial diversity was determined for each sample by targeting a portion of the ribosomal genes. PCR was performed to prepare amplicons using V3-V4 oligonucleotides (primers PCR1F_460: 5′-CTTTCCCTACACGACGCTCTTCCGATCTACGGRAGGCAGCAG-3′ and PCR1R_460: 5′-GGAGTTCAGACGTGTGCTCTTCCGATCTTACCAGGGTATCTAAT CCT-3′) (24–26). Amplicon quality was verified by gel electrophoresis, and samples were sent to the @BRIDGe platform for sequencing on an Illumina MiSeq (Illumina, San Diego, CA, United States).
A similar approach was used for fungal microbiota using the primers ITS2 (sense) 5′-GTGARTCATCGAATCTTT-3′ and (antisense) 5′-GATATGCTTAAGTTCAGCGGGT-3′ and the optimized and standardized ITS2 rRNA-amplicon-library preparation protocol (Metabiote, GenoScreen, Lille, France).
The 16S rRNA sequences were demultiplexed and quality filtered using the QIIME version 2.1.0 software package (27). The sequences were then assigned to ASVs (amplicon sequence variant) using the DADA2 algorithm (28) with a 97% pairwise identity threshold and classified taxonomically using the SILVA reference database (version 13.8) for bacteria (29). For the ITS2 rRNA sequences, data were processed using the FROGS pipeline (30) for sequence quality control, and filtering and affiliation of taxa was performed with the UNITE ITS database (version 8_2) (31) using the FROGS guidelines for ITS data.1 Rarefaction analysis was performed and used to compare the relative abundance of OTUs across samples. α-diversity was estimated using the Shannon diversity index or the number of observed species. β-diversity was measured using the Jaccard distance matrix and was used to build principal coordinates analysis (PCoA) plots. Using these tools, we did not observe any cage effect in our different sets of experiments. Differential abundance analysis based on the negative binomial distribution (DESeq2) algorithm was used to identify taxa that were specific to the treatment (32). The raw sequence data were deposited in the SRA database from the NCBI under the following accession numbers (PRJNA896895).
GraphPad Prism version 7 (San Diego, CA, United States) was used to perform all analyses and prepare graphs. For all data displayed in graphs, the results are expressed as the mean ± SEM (n = 7 to 12 per group). For comparisons between two groups, Student’s t test for unpaired data was used. For comparisons among more than two groups, one-way analysis of variance (ANOVA) and a post hoc Tukey or Dunnett test were used. For all statistical tests, differences with a p value less than 0.05 were considered statistically significant: *p < 0.05, **p < 0.01, ***p < 0.001. Statistical significance of sample grouping for β-diversity analysis was performed using Permanova method (999 permutations).
The evaluation of the impact of the yeast probiotic on the fungal and bacterial microbiota after ATB treatment was assessed using a complex setup of in vivo experiments. The complete setup is presented in Figure 1A with 5 different groups of 8 mice each. However, for analysis purposes we did not analyze all timepoints but we choose to focus on specific time points in order to decipher the impact of each treatment on both fungal and bacterial microbiota. At these specific time points feces from mice of the selected treated groups were collected and the microbiota composition analyzed. The fungal and bacterial microbiota analyses are separately presented as they were impacted in different manners.
As the first step of characterization of the yeast probiotic effects on the mycobiota on ATB treated mice, we evaluated the effect of Saccharomyces boulardii CNCM I-745 administration on the mycobiota of mice treated for 10 days with ATB by sequencing the ITS2 region of DNA from fecal samples (Figure 1B). We chose to study HMA mice to help further understand fungal and bacterial interactions within the human gut microbiota. Daily administration of yeast biased the study of α- or β-diversity because the constant and very high levels of S. boulardii skewed the ITS2 rRNA analyses and potentially masked the modifications of other taxa. As expected, administration of S. boulardii during ATB treatment increased the levels of the Saccharomycetaceae family and reduced the α-diversity of the fungal community (Figure 1C) because only one species was in high abundance (Figure 1D). Using the DESeq2 R package for differential analysis, we identified 6 families that were significantly impacted by S. boulardii: (p value adjusted <0.05): Atheliaceae, Debaryomycetaceae, Erysiphaceae, Hypocreales, Pleosporaceae and Tremellaceae. However, while the higher relative abundance cannot be contested for Tremellaceae, the reduced relative abundance could be due only to the over-representation of S. boulardii in the total sequences. Additional quantitative real time PCR or CFU would be necessary for the absolute abundance characterization in order to account for actual decrease of fungi families. Nevertheless, S. boulardii slightly increased the levels of the Tremellaceae family (Figure 1E) and seemed to contained the development of five specific families that would need further investigation using absolute quantification.
In an attempt to see the effect of S. boulardii administration without the cofounding effect of the gavage with S. boulardii we compared the fungal microbiota of mice under antibiotic treatment with or without supplementation with S. boulardii but 1 week after the discontinuation of the S. boulardii administration (Supplementary Figure S1A). In this context, α-and β-diversity analyses did not show any statistical differences as well as the specific analysis using DesEQ2 statistical tools (Supplementary Figure S1B) suggesting no long-term effect of the yeast probiotic supplementation on the fungal microbiota.
Finally, with the aim of describing the evolution of the mycobiota when the patient had stopped his medication, we examined the composition of the mycobiota 1 week after all treatments were stopped. We performed ITS rRNA sequencing of mouse feces without S. boulardii administration at D16, 1 week after the end of antibiotic therapy, and with S. boulardii administration at D30, 1 week after the end of yeast supplementation (Figure 2A). We were then able to analyze α- and β-diversities, as it is known that S. boulardii cannot become established in the human gut and is cleared within 24 to 48 h (33). Moreover, when administered daily to mice and then stopped, the yeast is undetectable after 1 week (34). The effect of S. boulardii administration 1 week after the end of supplementation showed a slight and nonsignificant increase in α-diversity (Shannon index) compared with mice that did not receive S. boulardii treatment (Figure 2B). An analysis of β-diversity showed no distinct clusters (Figure 2C), meaning that the mycobiota composition 1 week after the end of all treatments was similar between all groups.
Figure 2. One week after stopping all the treatments, the mycobiota returned to equilibrium. (A–C) HMA mice treated (ATB _ Vehicle) or not (Vehicle) with the ATB for 10 days and S. boulardii for 30 days with (ATB + S. boulardii _ Vehicle) or without the ATB (S. boulardii _ Vehicle). Grp 1 “Vehicle” n = 8; Grp 2 “ATB _Vehicle” n = 8; Grp 3 “S. boulardii _Vehicle” n = 8; Grp 5 “ATB + S. boulardii_Vehicle” n = 8, the experiment was performed 2 times. (A) Experimental design for the administration of the ATB and probiotic over 30 days. (B) α–Diversity of the fungal microbiota (ITS2 rRNA) in the fecal microbiota after ATB and probiotic treatments, described by the Shannon index. (C) β-Diversity. Principal coordinates analysis of Jaccard distances with each sample colored according to the treatment. Groups were compared using Permanova method.
While several studies have described the effects of ATB treatment and S. boulardii CNCM I-745 on gut bacterial microbiota composition (13), the longitudinal evaluation of S. boulardii administration during and after ATB treatment has rarely been reported and not in detail. Here, we followed different courses of treatment and analyzed how S. boulardii impacts the bacterial microbiota.
ATB treatment induced global changes in the bacterial microbiota, with a decrease in many commensal bacterial families, but these ecosystem modifications also favored the development of several other antibiotic-resistant bacteria favored by the release of many niches from sensitive bacteria. Hence, the families Clostridia vadin BB60 group, Enterobacteriaceae, Enterococcaceae, Monoglobaceae, Peptostreptococcaceae and Tannerellaceae were significantly induced after ATB treatment (Supplementary Figures S2A,B).
Interestingly, when S. boulardii was administered during ATB treatment (Figure 3A), it increased the number of observed species (Figure 3B) and the α-diversity (Shannon index) compared with mice that only received ATB treatment (Figure 3C). It also induced a global new composition of the bacterial microbiota, as illustrated by the clear clustering of the groups with or without S. boulardii (Jaccard index, p value = 0.001) (Figure 3D). Differential analysis using the DESeq2 tool showed that S. boulardii was able to reduce the levels of all the families increased by ATB treatment. Specifically, it reduced the levels of the 6 families significantly increased by ATB treatment: Clostridia vadin BB60 group, Enterobacteriaceae, Enterococcaceae, Monoglobaceae, Peptostreptococcaceae and Tannerellaceae (Figure 3E). S. boulardii was able to increase the abundances of the families Acidaminococcaceae, Akkermansiaceae, Atopobiaceae, Christensenellaceae, Lachnospiraceae, Marinifilaceae, Muribaculaceae, Oscillospiraceae, Rikenellaceae, Ruminococcaceae and Sutterellaceae (Figure 3F). Taken together, these data confirm a strong influence of the coadministration of S. boulardii CNCM I-745 during ATB treatment on the bacterial ecosystem, with the capacity to reduce the imbalance induced by ATB treatment and create an alternative bacterial ecosystem with the enhancement of other families.
Figure 3. Effect of Saccharomyces boulardii supplementation during antibiotic treatment on the bacterial microbiota. (A–F) HMA mice treated with the ATB or ATB and S. boulardii (ATB + S. boulardii) for 10 days. Grp 2 “ATB” n = 8; Grp 4 and 5 “ATB + S. boulardii” n = 16 (Grp 4 n = 8, Grp 5 n = 8), experiment was done 2 times. (A) Experimental design for the administration of ATB and probiotics over 10 days. (B,C) α–Diversity of the bacterial microbiota (16S rRNA) in the fecal microbiota, described by (B) observed species and (C) Shannon indices. (D) β-Diversity. Principal coordinates analysis of Jaccard distances with each sample colored according to the treatment. Groups were compared using Permanova method. (E,F) Taxa selected with the differential abundance analysis based on the negative binomial distribution (DESeq2) with padj <0.05 (E) decreased or (F) increased by the probiotic treatment unless otherwise indicated (n.s.: no significant differences).
As with the mycobiota, we evaluated the consequences for the bacterial community when S. boulardii administration was continued for one (Supplementary Figure S3A) or two (Figure 4A) weeks after the end of ATB treatment. With regards to the microbiota analysis, we did not identify any clear improvement when S. boulardii administration was continued for only 1 week (Supplementary Figures S3B–D), except for an additional decrease in the families Clostridia vadinBB60 and Tannerellaceae (Supplementary Figure S3E). Remarkably, when administered for 2 weeks after the end of ATB treatment (23 days in total), S. boulardii facilitated an increase in the number of observed species (Figure 4B) and the α-diversity (Shannon index) (Figure 4C) compared with mice that received S. boulardii only during ATB treatment. Similar to that after 10 and 16 days, the microbiota were globally different after 23 days and clustered separately in the β-diversity analysis (Jaccard index) (Figure 4D). Again, S. boulardii maintained a decrease in the levels of Clostridia vadinBB60 group and Tannerellaceae families and an increase in the levels of Lachnospiraceae and Oscillospiraceae (Figures 4E,F). With respect to the levels of the other families with antibiotic-resistant bacteria, Enterobacteriaceae, Enterococcaceae, Monoglobaceae, and Peptostreptococcaceae, S. boulardii administration only during ATB treatment seemed to be sufficient to maintain the low levels of these bacteria.
Figure 4. Effect of Saccharomyces boulardii supplementation on the bacterial microbiota for 2 weeks after antibiotic therapy. (A–F) HMA mice treated with the ATB and S. boulardii for 10 days and then no treatment for 2 weeks (ATB + S. boulardii _Vehicle) or two more weeks of S. boulardii (ATB + S. boulardii_ S. boulardii). Grp 4 “ATB + S. boulardii_Vehicle” n = 8; Grp 5 “ATB + S. boulardii S. boulardii” n = 8, the experiment was performed 2 times. (A) Experimental design for the administration of the ATB and probiotic over 23 days. (B,C) α–Diversity of the bacterial microbiota (16S rRNA) in the fecal microbiota, described by (B) observed species and (C) Shannon indices. (D) β-Diversity. Principal coordinates analysis of Jaccard distances with each sample colored according to the treatment. Groups were compared using Permanova method. (E,F) Taxa selected with the differential abundance analysis based on the negative binomial distribution (DESeq2) with padj <0.05 (E) decreased or (F) increased by the probiotic treatment.
Finally, we examined the composition of the bacterial microbiota 1 week after the cessation of all treatments (Figure 5A). As expected, α-diversity, evaluated by observed species or the Shannon index, was reduced by ATB treatment. This reduction was prevented by S. boulardii probiotic treatment (Figures 5B,C).
Figure 5. One week after stopping all treatments, S. boulardii facilitated the restoration of eubiosis. (A–D) HMA mice treated (ATB _ Vehicle) or not (Vehicle) with the ATB for 10 days and S. boulardii for 30 days with (ATB + S. boulardii _ Vehicle) or without the ATB (S. boulardii _ Vehicle). Grp 1 “Vehicle” n = 8; Grp 2 “ATB_Vehicle” n = 8; Grp 3 “S. boulardii_Vehicle” n = 8; Grp 5 “ATB + S. boulardii_Vehicle” n = 8, the experiment was performed 2 times. (A) Experimental design for the administration of the ATB and probiotic over 30 days. (B,C) α–Diversity of the bacterial microbiota (16S rRNA) in the fecal microbiota, described by (B) observed species and (C) Shannon indices. (D) β-Diversity. Principal coordinates analysis of Jaccard distances with each sample colored according to the treatment. Groups were compared using Permanova method.
Interestingly, β-diversity analysis showed that the microbiota of HMA mice untreated or treated only with S. boulardii 1 week after the end of treatment were very similar to each other (Figure 5D), suggesting that if S. boulardii had influenced the bacterial microbiota by itself, this effect did not last. However, these two clusters were very different from the cluster of microbiota exposed to ATB only. Consequently, even after 1 week without the ATB, the bacterial microbiota was still profoundly different from the initial microbiota. Remarkably, if the HMA mice were treated with S. boulardii during and after ATB treatment, the newly formed microbiota differed clearly not only from the microbiota only treated with ATB but also from the starting untreated microbiota, suggesting an intermediate state reached with S. boulardii administration.
Our objective was to study the effects of supplementation with the probiotic Saccharomyces boulardii CNCM I-745 during ATB treatment on both the bacterial and fungal gut microbiota. Indeed, no study has focused on the effect of this probiotic on the mycobiota, whereas it is widely used in human health. It was thus necessary to describe more precisely the impact of its administration on the fungal populations of the gut microbiota.
Our observations were made in vivo after FMT with feces from a healthy human donor. The FMT technique transfers a fecal suspension from a healthy donor after intestinal cleansing with polyethylene glycol, according to the published protocol (22). This technique allowed the transfer of part of the human microbiota without using germ-free mice that are immunologically immature or antibiotics, which for obvious reasons could not be used in our study on the effect of ATB (22). Therefore, this technique allowed us to work with a gut microbiota closer to humans than simple conventional mice.
S. boulardii CNCM I-745 is widely used in medicine to prevent and reduce the occurrence of diarrhea associated with ATB treatment (14). Its impact on the composition of the bacterial gut microbiota during ATB therapy has been studied in various contexts in humans (13). In the present study, we provide a comprehensive characterization of the change in both fungal and bacterial gut microbiota in human microbiota-associated mice triggered by supplementation with S. boulardii during and after ATB therapy with amoxicillin-clavulanate.
The first part of the study investigated the benefit of S. boulardii on mycobiota alterations during ATB therapy using fecal ITS rRNA sequencing. ITS rRNA data analysis was hampered by the daily oral gavage with yeast, which biased the prevalence analysis due to an overwhelming quantity of Saccharomyces in one group. However, using a differential abundance analysis tool (DESeq2) examining the levels of specific families that were modified, we were able to show that S. boulardii, when given during ATB treatment, increased the abundance of the Tremellaceae family. The genus Tremella, rich in polysaccharides, protein, dietary fiber and vitamin D, has been used for centuries in Chinese medicine to treat chest congestion, asthma and constipation; to balance blood sugar levels and cholesterol; and to reduce inflammation (35). Polysaccharides from this genus have been described as potent anti-inflammatory molecules (36). While the relative abundance decreases of Atheliaceae, Debaryomycetaceae, Erysiphaceae, Hypocreales and Pleosporaceae families was clear and probably due to the gavage with S. boulardii, the absolute quantification would be needed to conclude on the effect on these specific families. Overall, these data suggest that yeast supplementation during ATB treatment do modify the fungal population but there is a need for alternative technics to completely characterize these modifications. One week after the end of all treatments, the composition of the mycobiota was similar between all groups. These results advocate that the probiotic yeast S. boulardii CNCM I-745 administration might slightly affect the fungal population but that the effects do not last long after the end of probiotic treatment.
The second part of this study investigated the beneficial effect of S. boulardii on alterations in the bacterial microbiota during ATB therapy using fecal 16S rRNA sequencing. Administration of S. boulardii can have a slight impact on the bacterial composition of healthy subjects (37). When administered during ATB treatment, S. boulardii reduced the levels of the families Clostridia vadin BB60 group, Enterobacteriaceae, Enterococcaceae, Monoglobaceae, Peptostreptococcaceae and Tannerellaceae. These families were indeed favored in the gut after ATB treatment due to their antibiotic resistance to amoxicillin-clavulanate and the release of niches, particularly the Clostridia vadin BB60 group and Enterobacteriaceae families, given the high levels in mice on ATB without S. boulardii. Among the Enterobacteriaceae family, Escherichia coli, Klebsiella, Salmonella and Shigella species are responsible for diarrhea, fever and emerging antibiotic resistance (38). Enterococcus faecalis and E. faecium can cause systemic infections and result in urinary tract, intra-abdominal, pelvic and soft tissue infections (39, 40). Peptostreptococcus is associated with CRC risk, as its levels are significantly higher in CRC patients than in controls (41). On the other hand, the probiotic yeast was associated with an increase in the levels of Acidaminococcaceae, Akkermansiaceae, Atopobiaceae, Christensenellaceae, Lachnospiraceae, Marinifilaceae, Muribaculaceae, Oscillospiraceae, Rikenellaceae, Ruminococcaceae and Sutterellaceae families compared to mice that did not receive S. boulardii during ATB therapy. The probiotic Akkermansia muciniphila has been reported to reduce the risks of diabetes and obesity (42). The abundance of the Christensenellaceae family, inversely related to host body mass index and thus metabolic health, has been associated with human longevity and may also play a role in obesity and IBD (43). Additionally, with regard to obesity, Muribaculaceae appeared to be overrepresented in a group of mice fed a high-fat diet (44). The Lachnospiraceae family has been widely studied due to its ability to produce short-chain fatty acids and has therefore been associated with reduced severity of several diseases, such as IBD and CRC. Blautia hansenii in particular has been identified as protective in a mouse model of IBD (41, 45). The genus Alistipes, belonging to the family Rikenellaceae, is decreased in those with liver microbiota-related diseases and IBD and thus may play a protective role in the progression of cirrhosis and liver fibrosis, as well as colitis and CRC (46). Among the Ruminococcaceae family, Faecalibacterium prausnitzii has been particularly studied in regards to IBD and has shown anti-inflammatory properties in vitro and in vivo (47, 48).
Taken together, these data suggest that the administration of S. boulardii CNCM I-745 during ATB treatment helps preserve the microbiota from pathogenic bacteria and promotes certain bacteria identified as beneficial to host health. When supplementation was continued for one to 2 weeks after the end of ATB treatment, the yeast continued to maintain a low level of antibiotic-resistant bacteria, almost undetectable for the Enterobacteriaceae family, and promote higher levels of the family Lachnospiraceae, which is considered beneficial to host health. Finally, one week after stopping all treatments, mice that received S. boulardii during and after ATB therapy showed an intermediate state, promoting a return to eubiosis for the bacterial microbiota. These results suggest that S. boulardii should be given during ATB therapy instead of immediately after and should be continued after the end of therapy for at least 2 weeks. However, it is important to keep in mind that these results have been obtained with mice groups of limited size (8 to 12) for each time point and some other variations can have been missed or some over-evaluated.
In conclusion, it clearly appears that the administration of S. boulardii CNCM I-745 during amoxicillin-clavulanate treatment has a deep impact on microbial equilibrium. The identification of the microorganisms modulated by the yeast probiotic suggests that its use during ATB treatment is beneficial in many aspects regarding the bacterial microbiota, mostly by promoting beneficial microorganisms and keeping the development of deleterious ones at a minimum. In addition, prolonging the treatment at the end of ATB therapy extends these beneficial effects on the bacterial community.
The datasets presented in this study can be found in online repositories. The names of the repository/repositories and accession number(s) can be found at: https://www.ncbi.nlm.nih.gov/, PRJNA896895.
The animal study was reviewed and approved by COMETHEA C2EA – 45, Jouy en Josas, France.
MS and MR designed the study and analyzed and interpreted the data. MS, YW, AL, GC, CM, and CD helped to collect the data. MS and MR wrote the manuscript. M-LM, PL, and HS commented on and corrected the manuscript. All authors contributed to the article and approved the submitted version.
The authors declare that this study received funding from Biocodex. The funder was not involved in the collection, analysis and interpretation of data, nor the writing of this article. The original study design was initially discussed between the research group and the funder. The decision to submit the data for publication was approved by the funder.
The authors declare that the research was conducted in the absence of any commercial or financial relationships that could be construed as a potential conflict of interest. The original study design was initially discussed between the research group and Biocodex. The decision to submit the data for publication was approved by Biocodex.
All claims expressed in this article are solely those of the authors and do not necessarily represent those of their affiliated organizations, or those of the publisher, the editors and the reviewers. Any product that may be evaluated in this article, or claim that may be made by its manufacturer, is not guaranteed or endorsed by the publisher.
The Supplementary material for this article can be found online at: https://www.frontiersin.org/articles/10.3389/fmed.2023.1087715/full#supplementary-material
ANOVA, analysis of variance; ATB, antibiotic; CRC, colorectal cancer; FMT, fecal microbiota transfer; Grp, group; HMA, human microbiota-associated; IBD, inflammatory bowel disease; IBS, irritable bowel syndrome; LDA, linear discriminant analysis; NaCl, sodium chloride; NGS, next-generation sequencing; PCoA, principal coordinates analysis; PEG, polyethylene glycol; S. boulardii, Saccharomyces boulardii; SEM, standard error of the mean.
1. Fan, Y, and Pedersen, O. Gut microbiota in human metabolic health and disease. Nat Rev Microbiol. (2021) 19:55–71. doi: 10.1038/s41579-020-0433-9
2. West, CE, Renz, H, Jenmalm, MC, Kozyrskyj, AL, Allen, KJ, Vuillermin, P, et al. The gut microbiota and inflammatory noncommunicable diseases: associations and potentials for gut microbiota therapies. J Allergy Clin Immunol. (2015) 135:3–13; quiz 14. doi: 10.1016/j.jaci.2014.11.012
3. Liguori, G, Lamas, B, Richard, ML, Brandi, G, da Costa, G, Hoffmann, TW, et al. Fungal dysbiosis in mucosa-associated microbiota of Crohn’s disease patients. J Crohns Colitis. (2016) 10:296–305. doi: 10.1093/ecco-jcc/jjv209
4. Sokol, H, Leducq, V, Aschard, H, Pham, HP, Jegou, S, Landman, C, et al. Fungal microbiota dysbiosis in IBD. Gut. (2017) 66:1039–48. doi: 10.1136/gutjnl-2015-310746
5. Jain, U, Ver Heul, AM, Xiong, S, Gregory, MH, Demers, EG, Kern, JT, et al. Debaryomyces is enriched in Crohn’s disease intestinal tissue and impairs healing in mice. Science. (2021) 371:1154–9. doi: 10.1126/science.abd0919
6. Limon, JJ, Tang, J, Li, D, Wolf, AJ, Michelsen, KS, Funari, V, et al. Malassezia is associated with Crohn’s disease and exacerbates colitis in mouse models. Cell Host Microbe. (2019) 25:377–388.e6. doi: 10.1016/j.chom.2019.01.007
7. Aykut, B, Pushalkar, S, Chen, R, Li, Q, Abengozar, R, Kim, JI, et al. The fungal mycobiome promotes pancreatic oncogenesis via activation of MBL. Nature. (2019) 574:264–7. doi: 10.1038/s41586-019-1608-2
8. Yang, AM, Inamine, T, Hochrath, K, Chen, P, Wang, L, Llorente, C, et al. Intestinal fungi contribute to development of alcoholic liver disease. J Clin Investig. (2017) 127:2829–41. doi: 10.1172/JCI90562
9. Food and Agricultural Organization of the United Nations and World Health Organization. (2001). Health and nutritional properties of probiotics in food including powder Milk with live lactic acid Bacteria. Report of a joint FAO/WHO expert consultation on evaluation of health and nutritional properties of probiotics in food including powder Milk with live lactic acid Bacteria, 1–34.
10. Sarowska, J, Choroszy-Król, I, Regulska-Ilow, B, Frej-Mądrzak, M, and Jama-Kmiecik, A. The therapeutic effect of probiotic bacteria on gastrointestinal diseases. Adv Clin Exp Med. (2013) 22:759–66.
11. Gareau, MG, Sherman, PM, and Walker, WA. Probiotics and the gut microbiota in intestinal health and disease. Nat Rev Gastroenterol Hepatol. (2010) 7:503–14. doi: 10.1038/nrgastro.2010.117
12. Szajewska, H, and Kołodziej, M. Systematic review with meta-analysis: Saccharomyces boulardii in the prevention of antibiotic-associated diarrhoea. Aliment Pharmacol Ther. (2015) 42:793–801. doi: 10.1111/apt.13344
13. Kabbani, TA, Pallav, K, Dowd, SE, Villafuerte-Galvez, J, Vanga, RR, Castillo, NE, et al. Prospective randomized controlled study on the effects of Saccharomyces boulardii CNCM I-745 and amoxicillin-clavulanate or the combination on the gut microbiota of healthy volunteers. Gut Microbes. (2017) 8:17–32. doi: 10.1080/19490976.2016.1267890
14. Moré, MI, and Swidsinski, A. Saccharomyces boulardii CNCM I-745 supports regeneration of the intestinal microbiota after diarrheic dysbiosis – a review. Clin Exp Gastroenterol. (2015) 8:237–55. doi: 10.2147/CEG.S85574
15. McFarland, LV, Surawicz, CM, Greenberg, RN, Fekety, R, Elmer, GW, Moyer, KA, et al. A randomized placebo-controlled trial of Saccharomyces boulardii in combination with standard antibiotics for Clostridium difficile disease. JAMA. (2014) 271:1913–8. doi: 10.1001/jama.1994.03510480037031
16. Cárdenas, PA, Garcés, D, Prado-Vivar, B, Flores, N, Fornasini, M, Cohen, H, et al. Effect of Saccharomyces boulardii CNCM I-745 as complementary treatment of Helicobacter pylori infection on gut microbiome. Eur J Clin Microbiol Infect Dis. (2020) 39:1365–72. doi: 10.1007/s10096-020-03854-3
17. Cifuentes, SG, Prado, MB, Fornasini, M, Cohen, H, Baldeón, ME, and Cárdenas, PA. Saccharomyces boulardii CNCM I-745 supplementation modifies the fecal resistome during Helicobacter pylori eradication therapy. Helicobacter. (2022) 27:e12870. doi: 10.1111/hel.12870
18. Sovran, B, Planchais, J, Jegou, S, Straube, M, Lamas, B, Natividad, JM, et al. Enterobacteriaceae are essential for the modulation of colitis severity by fungi. Microbiome. (2018) 6:152–16. doi: 10.1186/s40168-018-0538-9
19. Constante, M, De Palma, G, Lu, J, Jury, J, Rondeau, L, Caminero, A, et al. Saccharomyces boulardii CNCM I-745 modulates the microbiota–gut–brain axis in a humanized mouse model of irritable bowel syndrome. Neurogastroenterol Motil. (2021) 33:1–9. doi: 10.1111/nmo.13985
20. Wipperman, MF, Fitzgerald, DW, Juste, MAJ, Taur, Y, Namasivayam, S, Sher, A, et al. Antibiotic treatment for tuberculosis induces a profound dysbiosis of the microbiome that persists long after therapy is completed. Sci Rep. (2017) 7:10767. doi: 10.1038/s41598-017-10346-6
21. Dolk, FCK, Pouwels, KB, Smith, DRM, Robotham, JV, and Smieszek, T. Antibiotics in primary care in England: which antibiotics are prescribed and for which conditions? J Antimicrob Chemother. (2018) 73:ii2–ii10. doi: 10.1093/jac/dkx504
22. Wrzosek, L, Ciocan, D, Borentain, P, Spatz, M, Puchois, V, Hugot, C, et al. Transplantation of human microbiota into conventional mice durably reshapes the gut microbiota. Sci Rep. (2018) 8:6854. doi: 10.1038/s41598-018-25300-3
23. Godon, JJ, Zumstein, E, Dabert, P, Habouzit, F, and Moletta, R. Molecular microbial diversity of an anaerobic digestor as determined by small-subunit rDNA sequence analysis. Appl Environ Microbiol. (1997) 63:2802–13. doi: 10.1128/aem.63.7.2802-2813.1997
24. Andersson, AF, Lindberg, M, Jakobsson, H, Bäckhed, F, Nyrén, P, and Engstrand, L. Comparative analysis of human gut microbiota by barcoded pyrosequencing. PLoS One. (2008) 3:e2836. doi: 10.1371/journal.pone.0002836
25. Liu, Z, Desantis, TZ, Andersen, GL, and Knight, R. Accurate taxonomy assignments from 16S rRNA sequences produced by highly parallel pyrosequencers. Nucleic Acids Res. (2008) 36:e120. doi: 10.1093/nar/gkn491
26. Lluch, J, Servant, F, Païssé, S, Valle, C, Valière, S, Kuchly, C, et al. The characterization of novel tissue microbiota using an optimized 16S metagenomic sequencing pipeline. PLoS One. (2015) 10:e0142334. doi: 10.1371/journal.pone.0142334
27. Caporaso, JG, Kuczynski, J, Stombaugh, J, Bittinger, K, Bushman, FD, Costello, EK, et al. QIIME allows analysis of high-throughput community sequencing data. Nat Methods. (2010) 7:335–6. doi: 10.1038/nmeth.f.303
28. Callahan, BJ, McMurdie, PJ, Rosen, MJ, Han, AW, Johnson, AJA, and Homes, SP. DADA2: high-resolution sample inference from Illumina amplicon data. Nat Methods. (2016) 13:581–3. doi: 10.1038/nmeth.3869
29. Quast, C, Pruesse, E, Yilmaz, P, Gerken, J, Schweer, T, Yarza, P, et al. The SILVA ribosomal RNA gene database project: improved data processing and web-based tools. Nucleic Acids Res. (2013) 41:D590–6. doi: 10.1093/nar/gks1219
30. Escudié, F, Auer, L, Bernard, M, Mariadassou, M, Cauquil, L, Vidal, K, et al. FROGS: find, rapidly, OTUs with galaxy solution. Bioinformatics. (2018) 34:1287–94. doi: 10.1093/bioinformatics/btx791
31. Nilsson, RH, Larsson, K-H, Taylor, AFS, Bengtsson-Palme, J, Jeppesen, TS, Schigel, D, et al. The UNITE database for molecular identification of fungi: handling dark taxa and parallel taxonomic classifications. Nucleic Acids Res. (2019) 47:D259–64. doi: 10.1093/nar/gky1022
32. Love, MI, Huber, W, and Anders, S. Moderated estimation of fold change and dispersion for RNA-seq data with DESeq2. Genome Biol. (2014) 15:550–21. doi: 10.1186/s13059-014-0550-8
33. Buts, JP, and De Keyser, N. Effects of Saccharomyces boulardii on intestinal mucosa. Dig Dis Sci. (2006) 51:1485–92. doi: 10.1007/s10620-005-9016-x
34. Barc, M-C, Charrin-Sarnel, C, Rochet, V, Bourlioux, F, Sandré, C, Boureau, H, et al. Molecular analysis of the digestive microbiota in a gnotobiotic mouse model during antibiotic treatment: influence of Saccharomyces boulardii. Anaerobe. (2008) 14:229–33. doi: 10.1016/j.anaerobe.2008.04.003
35. Shahrajabian, MH, Sun, W, Cheng, Q, and Khoshkharam, M. Exploring the quality of foods from ancient China based on traditional Chinese medicine In: Functional foods and nutraceuticals in metabolic and non-communicable diseases. eds. R.B. Singh, A.A. Isaza, and S. Watanabe Amsterdam, Netherland: Elsevier (2022). 87–105.
36. Wu, Y-J, Wei, Z-X, Zhang, F-M, Linhardt, RJ, Sun, P-L, and Zhang, A-Q. Structure, bioactivities and applications of the polysaccharides from Tremella fuciformis mushroom: a review. Int J Biol Macromol. (2019) 121:1005–10. doi: 10.1016/j.ijbiomac.2018.10.117
37. Akil, I, Yilmaz, O, Kurutepe, S, Degerli, K, and Kavukcu, S. Influence of oral intake of Saccharomyces boulardii on Escherichia coli in enteric flora. Pediatr Nephrol. (2006) 21:807–10. doi: 10.1007/s00467-006-0088-4
38. Kotloff, KL. Bacterial diarrhoea. Curr Opin Pediatr. (2022) 34:147–55. doi: 10.1097/MOP.0000000000001107
39. Vu, J, and Carvalho, J. Enterococcus: review of its physiology, pathogenesis, diseases and the challenges it poses for clinical microbiology. Front Biol (Beijing). (2011) 6:357–66. doi: 10.1007/s11515-011-1167-x
40. Agudelo Higuita, NI, and Huycke, MM. Enterococci: From Commensals to Leading Causes of Drug Resistant Infection. Boston: Massachusetts Eye and Ear Infirmary (2014):385–408. doi: 10.1128/9781555817923.ch10,
41. Ahn, J, Sinha, R, Pei, Z, Dominianni, C, Wu, J, Shi, J, et al. Human gut microbiome and risk for colorectal cancer. J Natl Cancer Inst. (2013) 105:1907–11. doi: 10.1093/jnci/djt300
42. Rodrigues, VF, Elias-Oliveira, J, Pereira, ÍS, Pereira, JA, Barbosa, SC, Machado, MSG, et al. Akkermansia muciniphila and gut immune system: a good friendship that attenuates inflammatory bowel disease, obesity, and diabetes. Front Immunol. (2022) 13:1–8. doi: 10.3389/fimmu.2022.934695
43. Waters, JL, and Ley, RE. The human gut bacteria Christensenellaceae are widespread, heritable, and associated with health. BMC Biol. (2019) 17:83. doi: 10.1186/s12915-019-0699-4
44. Cao, W, Chin, Y, Chen, X, Mi, Y, Xue, C, Wang, Y, et al. The role of gut microbiota in the resistance to obesity in mice fed a high fat diet. Int J Food Sci Nutr. (2020) 71:453–63. doi: 10.1080/09637486.2019.1686608
45. Wang, Y, Spatz, M, Da Costa, G, Michaudel, C, Lapiere, A, Danne, C, et al. Deletion of both Dectin-1 and Dectin-2 affects the bacterial but not fungal gut microbiota and susceptibility to colitis in mice. Microbiome. (2022) 10:91. doi: 10.1186/s40168-022-01273-4
46. Parker, BJ, Wearsch, PA, Veloo, ACM, and Rodriguez-Palacios, A. The genus Alistipes: gut Bacteria with emerging implications to inflammation, Cancer, and mental health. Front Immunol. (2020) 11:906. doi: 10.3389/fimmu.2020.00906
47. Sokol, H, Pigneur, B, Watterlot, L, Lakhdari, O, Bermúdez-Humarán, LG, Gratadoux, J-J, et al. Faecalibacterium prausnitzii is an anti-inflammatory commensal bacterium identified by gut microbiota analysis of Crohn disease patients. Proc Natl Acad Sci U S A. (2008) 105:16731–6. doi: 10.1073/pnas.0804812105
Keywords: microbiota, mycobiota, antibiotics, Saccharomyces boulardii CNCM I-745, treatment
Citation: Spatz M, Wang Y, Lapiere A, Da Costa G, Michaudel C, Danne C, Michel M-L, Langella P, Sokol H and Richard ML (2023) Saccharomyces boulardii CNCM I-745 supplementation during and after antibiotic treatment positively influences the bacterial gut microbiota. Front. Med. 10:1087715. doi: 10.3389/fmed.2023.1087715
Received: 02 November 2022; Accepted: 04 July 2023;
Published: 04 August 2023.
Edited by:
Angel Lanas, University of Zaragoza, SpainReviewed by:
Benedikt M. Mortzfeld, University of Massachusetts Medical School, United StatesCopyright © 2023 Spatz, Wang, Lapiere, Da Costa, Michaudel, Danne, Michel, Langella, Sokol and Richard. This is an open-access article distributed under the terms of the Creative Commons Attribution License (CC BY). The use, distribution or reproduction in other forums is permitted, provided the original author(s) and the copyright owner(s) are credited and that the original publication in this journal is cited, in accordance with accepted academic practice. No use, distribution or reproduction is permitted which does not comply with these terms.
*Correspondence: Madeleine Spatz, bWFkZWxlaW5lLnNwYXR6QGlucmFlLmZy; Mathias L. Richard, bWF0aGlhcy5yaWNoYXJkQGlucmFlLmZy
Disclaimer: All claims expressed in this article are solely those of the authors and do not necessarily represent those of their affiliated organizations, or those of the publisher, the editors and the reviewers. Any product that may be evaluated in this article or claim that may be made by its manufacturer is not guaranteed or endorsed by the publisher.
Research integrity at Frontiers
Learn more about the work of our research integrity team to safeguard the quality of each article we publish.