- 1Nantes Université, Inserm, The Enteric Nervous System in Gut and Brain Disorders, Nantes, France
- 2CHU Nantes, Inserm, Nantes Université, The Enteric Nervous System in Gut and Brain Disorders, Nantes, France
The enteric nervous system (ENS) continues to dazzle scientists with its ability to integrate signals, from the outside as well as from the host, to accurately regulate digestive functions. Composed of neurons and enteric glial cells, the ENS interplays with numerous neighboring cells through the reception and/or the production of several types of mediators. In particular, ENS can produce and release n-6 oxylipins. These lipid mediators, derived from arachidonic acid, play a major role in inflammatory and allergic processes, but can also regulate immune and nervous system functions. As such, the study of these n-6 oxylipins on the digestive functions, their cross talk with the ENS and their implication in pathophysiological processes is in full expansion and will be discussed in this review.
Introduction
The gastrointestinal tract (GI) fulfils complex tasks that are essential for survival including food digestion, absorption of nutrients and transit of luminal content. These processes are coordinated by a unique peripheral autonomic nervous system embedded in the gut wall and called the enteric nervous system [ENS; (1)]. The ENS consists of ganglionated networks organized in two main plexi, myenteric and submucosal, which regulate muscle and mucosal functions, respectively. Each ganglion is composed of neurons surrounded by enteric glial cells (EGCs) and these two cell types regulate GI functions via specific neuro- and glio-mediators. Regarding the enteric neurons, the most common neurotransmitters are acetylcholine, vasoactive intestinal peptide and nitric oxide while, among the numerous soluble mediators produced and released by EGCs, neuroptrophic factors such as glial derived neurotrophic factor (GDNF), brain-derived neurotrophic factor (BDNF) and epidermal growth factor (EGF) have been by far the most widely studied. More recent observations showed that enteric neurons and glial cells are also capable of producing bioactive lipids such as polyunsaturated fatty acids (PUFAs) and in particular oxylipins (2, 3). Oxylipins are associated with a diverse set of inflammatory processes linked to various diseases, including inflammatory bowel diseases (IBD). The production of some n-6 pro-inflammatory oxylipins is increased in the lower GI tract of IBD patients compared to control subjects (4–7) or in inflamed mucosa relative to non-inflamed mucosa (8–11) and correlate with inflammation severity (12). These findings support the idea of an imbalance between the n-6 pro-inflammatory and n-3 anti-inflammatory derivatives as pathological contributing factors, but this concept was challenged when it has been found for several derivatives that they could have pro- as well as anti-inflammatory properties (13–18). More importantly, recent findings define the importance of pro-resolutive properties of some n-6 derivatives (19) and their homeostatic functions (20, 21). In addition, we recently reported that EGCs produce mostly n-6 and little n-3 oxylipins and that some n-6 oxylipins that have a pro-resolutive and pro-homeostatic function are lacking in Crohn’s disease patients. The general effects of n-6 oxylipins on the GI tract have been reviewed elsewhere (22, 23) but as far as we know there is no existing review on the links between n-6 oxylipins and the ENS. The current article fills that void and covers the research in this subject area over the past two decades. Here, we first provide a brief overview of n-6 oxylipins, before discussing the interplay between the compound and the ENS and their potential roles in GI pathophysiology.
n-6 oxylipins
The time is long gone when lipids represented only inert fats. In addition to their role in the formation of biological structures (e.g., cell membranes or organelles), lipids have metabolic energy storage and cell signaling function. Signaling lipids, from lysophosphatidic acid to endocannabinoids, regulate essential functions such as synaptic transmission, vasoconstriction or inflammation. Among the most important are the Omega-3 (n-3) and Omega-6 (n-6) oxylipins, the family of oxygenated products formed from n-3 and n-6 PUFAs. In both Omega families, many forms of PUFAs exist: α-linolenic acid (ALA), eicosapentaenoic acid (EPA), and docosahexaenoic acid (DHA) from the n-3 family and linoleic acid (LA), dihomo-γ-linolenic acid (DGLA), and arachidonic acid (ARA) from the n-6 family are the important PUFAs for human health.
n-6 Oxylipins are synthesized from ARA by cyclooxygenase, lipoxygenase, and cytochrome P450 (CYP) monooxygenase activities (24; Figure 1). Cyclooxygenases (COX), also known as prostaglandin-endoperoxide synthase (PTGS) are oxidoreductases that allow the synthesis of prostaglandins (PG) and thromboxanes (TX). In humans there are two isoforms of COX: COX-1 and COX-2. COX-1 participates in the basal synthesis of eicosanoids and is constitutively expressed in most tissues. In contrast, the expression of COX-2 is induced by stimuli such as inflammation (25). Their actions are carried out in two stages: first they subtract a hydrogen from the carbon 13 of the ARA and they add two molecules of oxygen, which allows the formation of prostaglandin G2 (PGG2). Second, this PGG2 is reduced to PGH2 by active peroxidase. The synthesis of PG and TX is then carried out thanks to specific isomerases. Thus, the PGD synthetases (L-PGDS and H-PGDS), the PGE synthetases (mPGES-1, mPGES-2, and cPGES3), the PGF synthetase (PGFS), the PGI synthetase (PTGIS and the TX synthetase (TXAS) that allow the synthesis of PGD2 (which can give PGJ2 following dehydration), PGE2, PGF2α, PGI2 (or prostacyclin), and TXA2, respectively. The receptors for these compounds are G-protein coupled receptors, and a same eicosanoid can modulate its action depending on the targeted receptor subtype (26). The lipoxygenase (LOX) pathway allows the synthesis of hydroxyeicosatetraenoic acids (HETE) and leukotrienes (LT). These are non-heme iron enzymes that contain dioxygenases and are constitutively expressed in plants and animals. In the intestine we find the lipoxygenases 15-LOX (15-LOX-1 and 15-LOX-2), 12-LOX and 5-LOX which allow the synthesis of 15-HETE, 12-HETE, 5-HETE and LT, respectively. They will catalyze the reaction PUFA (ARA for example) + O2– > Hydroperoxyeicosatetraenoic acid (HpETE). These derivatives are then transformed into more stable one: HETE and LT (27). The LT receptors are well described, but little is known about those for HETE. However, it is recognized that they may be peroxisome proliferator-activated receptor (PPAR) agonists (28, 29). On the other hand, epoxy-PUFA are the oxylipins generated by CYP enzymes, later converted to their corresponding 1,2-diols by soluble epoxy hydrolase.
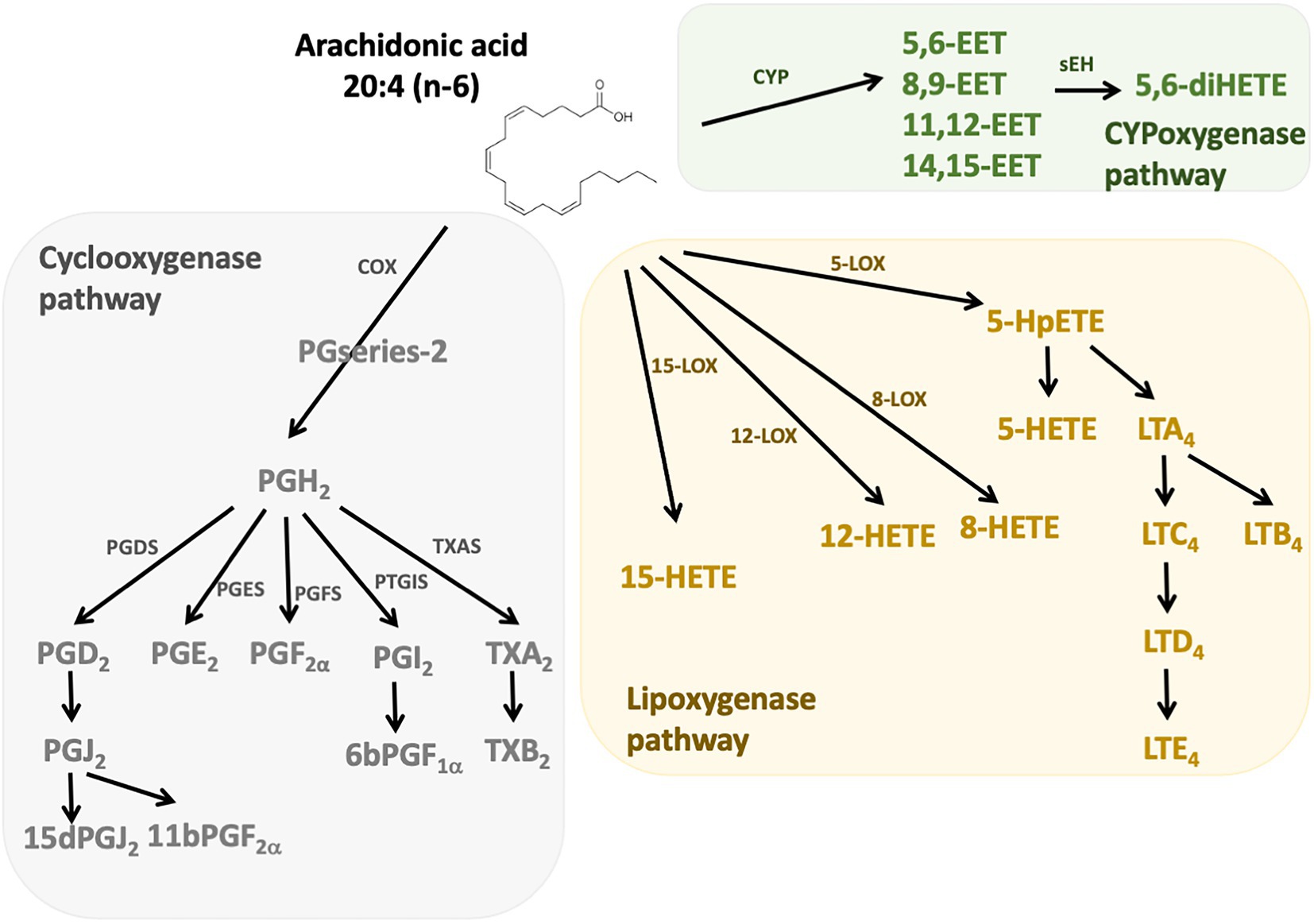
Figure 1. n-6 Oxylipins derived from arachidonic acid. The metabolism of PUFAs is a complex process involving several enzymes of desaturation, elongation, and β-oxidation. n-6 oxylipins are synthesized from arachidonic acid (ARA) through three major pathways, the first depending on cyclooxygenase (grey), the second on lipoxygenase (yellow), and the third on cytochrome P450 (CYP) monooxygenase activities (green). COX, cyclooxygenase; LOX, lipoxygenase; CYP, cytochrome P450; PGFS, prostaglandin F synthase; PGDS, prostaglandin D synthase, PGES, prostaglandin E synthase; PTGIS, prostaglandin I synthase; TXAS, thromboxane A2 synthase.
n-6 Oxylipins regulate ENS
Oxylipins play a major role in the regulation of inflammation not only in the central nervous system (CNS) but also in the GI tract. This prompted research on their potential effects on the ENS and numerous studies showed that n-6 oxylipins are indeed able to modulate neuronal activity and to exert neuroprotective effects. Regarding EGCs, although the data are still scarce, their phenotype and activity could be modified by oxylipins.
n-6 Oxylipins, enteric neuronal activity and neuroprotection
The first studies suggesting that n-6 oxylipins could modulate enteric neurotransmission and thus neuronal activity were performed in the myenteric plexus of guinea pig ileum and focused on the release of acethylcholine (Ach). Two independent groups showed that the release of Ach by either protein kinase C, DMPP (a nicotinic agonist) or neuronal activity was decreased in the presence of mepacrine, a PLA2 inhibitor, and that the opposite was observed when samples of myenteric plexus were treated with either a PLA2 activator (melittin) or ARA (30, 31). Additional pharmacological experiments allowed to identify the prostaglandins PGE2, PGI2 and PGF2 as critically involved in the chemically or electrically-induced release of Ach by enteric neurons (32–35) (Figure 2). Regarding the LOX pathway, AA861, a selective inhibitor of 5-lipoxygenase, inhibited acetylcholine release from the myenteric plexus induced by electrical field stimulation, an effect that was reversed by metabolites from the 5-lipoxygenase pathway, including 5-HETE, LT C4, D4 and E4 (36) (Figure 2). Besides Ach, it has also been shown that prostanoid signaling, including PGE2, regulated the cytokine-induced suppression of norepinephrine release in rat myenteric plexus (37). Although scarcer than for the myenteric plexus, some findings suggest that the n-6 oxylipins produced by the submucosal plexus might be also involved in the release of Ach. Interleukin-1β (IL-1β) potentiated the release of Ach induced electrical transmural stimulation in whole guinea pig ileum but not in longitudinal muscle/myenteric plexus (LMMP) only or in mucosal-free preparations and these effects were prevented with of PLA2, COX or LOX inhibitors (38) (Figure 2). Taken as a whole, these findings suggest that IL-1β stimulates cholinergic enteric neurons via a cascade of ARA metabolites produced in tissues at the mucosal and submucosal level, but not in the myenteric plexus or longitudinal muscles.
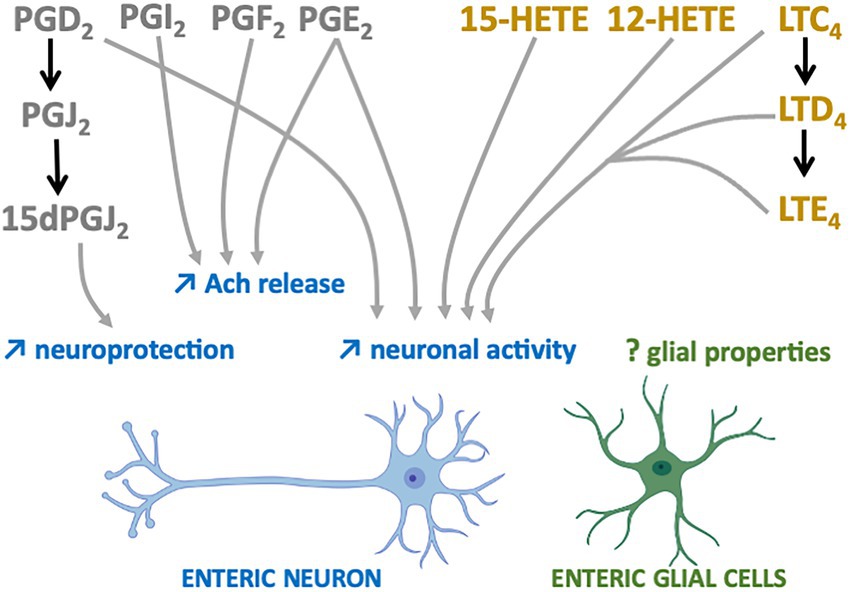
Figure 2. n-6 Oxylipins regulate the Enteric nervous system. COX-(grey) or LOX-(yellow) derived n-6 oxylipins increase (↑) different enteric neuron properties (blue) but their impacts on EGCs remain to be determined (?).
Prostaglandins are also capable of directly modulating the electrophysiological properties of enteric neurons. Treatment of myenteric ganglia from the guinea pig colon or ileum with PGE2 led to prolonged depolarization of enteric neurons with enhanced spike discharge together with an increased neuronal excitability (39–41) (Figure 2). More recently, myenteric neuron activity and contraction have been shown to depend on prostaglandin release (COX-2 pathway) from mucosal mast cells in a model of TNBS-induced colitis (42). Similar findings were observed when submucosal neurons of guinea pig colon were treated with PGD2 (43). The application of leukotrienes C4, D4 and E4 to myenteric and submucosal neurons of guinea pig resulted in slow depolarization of small intestine neurons, thereby suggesting that derivatives of the LOX pathway are also modulators of enteric neuronal excitability (Figure 2).
Oxylipins are well-known neuroprotectors in the CNS and this might be also the case in the ENS. 15-Deoxy-Delta-12,14-prostaglandin J2 (15d-PGJ2), a PGD2 metabolite produced by EGCs, has been identified as having neuroprotective effects (44) (Figure 2). Pretreatment of primary cultures of ENS with this n-6 oxylipin prevented the neurotoxicity induced by hydrogen peroxide. The involvement of EGCs in this beneficial effect was validated after glial genetic invalidation of the key enzyme involved in the synthesis of 15dPGJ2, the L-PGDS. The neuroprotective effect of this glial mediator appeared to be due to increased expression in enteric neurons of both glutamate cysteine ligase and intracellular glutathione.
n-6 Oxylipins and EGCs
In sharp contrast with the regulation of enterics neuron properties described below, data on the effects of n-6 oxylipins on the EGCs are lacking. The observation showing that a high fat diet, which controls in a major way the GI oxylipin content, increased the number of EGCs in myenteric plexus in a juvenile mouse model (45), but alter submucosal glial density where the expression of the glial marker calcium-binding protein S100β (S100β) was also decreased (46), might suggest that EGC morphology and/or reactivity could be modified via oxylipins. But this is the few we know, and while many studies described the regulation of central glia (astrocytes or microglia) through oxylipin (mostly n-3 oxylipin), the impact of a specific oxylipin on EGC markers and functions remain to be addressed.
The ENS is a source of n-6 oxylipins
While n-6 oxylipins can regulate the ENS, we could wonder whether ENS itself could be a source of n-6 oxylipins. Unlike other signaling, lipid messengers are produced on demand and degraded by metabolic enzymes to control their lifespan and signaling actions. The ENS contains the enzymatic machinery required for the production of n-6 oxylipins, and production of several have been measured in ENS or EGC in culture.
Secreted PLA2 (sPLA2-X) has been detected in the myenteric ganglia of small intestine and in neuronal fibers of the stomach, thereby suggesting that ARA-derived eicosanoids are produced by enteric neurons (47, 48). Regarding the cyclooxygenase pathway, both COX1 and 2 enzymes are found in the myenteric ganglia in both humans and mice (49–51). We further showed that both neurons and EGC from primary culture of rat ENS express the L-PGDS (52), and human EGC also express the aldo-keto reductase family 1 member C3 (AKR1C3) (53). The expression of these key enzymes is associated with the production of PGD2, 15dPGJ2, 11βPGF2α, PGE2, PGI2 (estimated by the measurement of its metabolite 6kPGF1α) and TXB2 in primary culture of rat ENS, rat or human EGC (52–54). The LOX pathway is also active in ENS as we have shown that human submucosal plexus and human EGC express the 15-LOX-2 (54). The downstream mediator, 15-HETE, or oxylipins produced from other LOX pathways such as LTB4, 5-HETE, 8-HETE and 12-HETE are also produced by rat and human EGC as demonstrated by the enlarged lipidomic analyzes (53, 54). No enzyme or specific metabolite from the CYP pathway has been specifically attributed to the ENS so far.
In an interesting way and comparable to what has been done in the CNS, the production of n-6 oxylipins by the ENS is regulated by inflammation. Seminal papers on the topic showed that COX2 and its downstream metabolites TXA2 and TXB2 were upregulated by TNF-α in isolated rat myenteric ganglia (55), while LPS induced COX-2 expression in enteric glial cells via autocrinal secretion of IL-1β (56). More recently, we showed that both neurons and EGC responded to LPS by increasing L-PGDS expression and its downstream metabolite PGD2 (10).
Therefore, it appears that ENS, in the same way as immune cells, muscle cells or epithelial cells, could be a source of n-6 oxylipins, and thereby regulate its neighboring cells to participate in the control of digestive functions.
n-6 Oxylipins: ENS crosstalk and intestinal functions
The n-6 oxylipins can be produced by enteric nervous cells, both at the neuronal and glial level, but these lipid messengers regulate ENS properties, suggesting that they could also play a crucial role in the regulation of intestinal functions controlled by ENS (Figure 3).
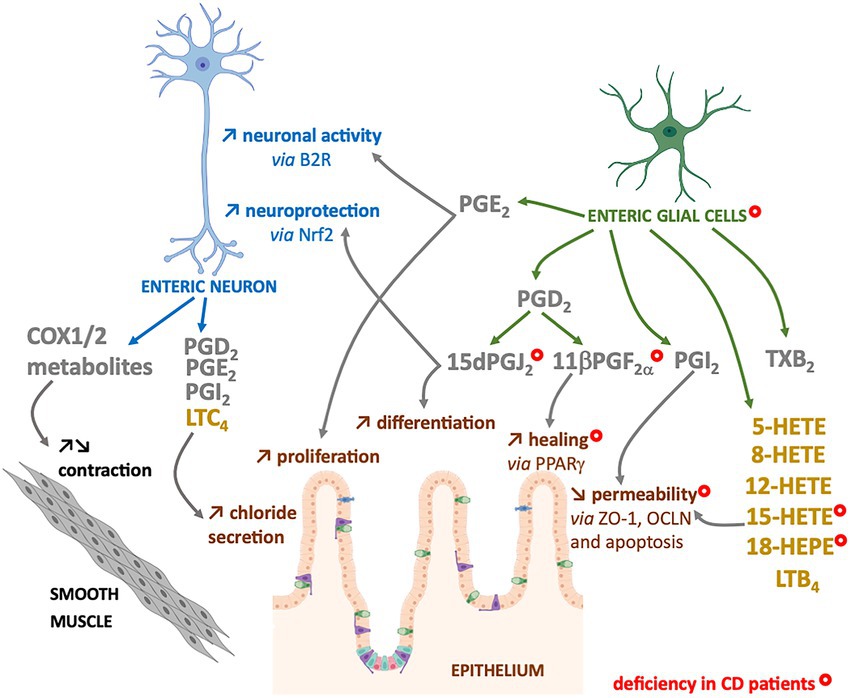
Figure 3. Enteric neurons and glial cells produce n-6 oxylipins to regulate the neighboring cells. Some n-6 oxylipin are to regulate glia or neurons, but also to control functions of smooth muscle or intestinal epithelial cells. COX-(grey) or LOX-(yellow) derived n-6 oxylipins produced by enteric neurons and/or glial cells can regulate different enteric neuron properties (blue), but also intestinal epithelial barrier properties (brown) or smooth muscle contraction (black). Marked with a () are cells, oxylipins or cellular functions deficient in chronic inflammatory disease that is Crohn’s disease (CD).
Intestinal epithelial regulation
The intestinal epithelial barrier (IEB) represents a central actor of intestinal homeostasis. Intestinal epithelial barrier integrity is ensured by a constant renewal thanks to a balance between proliferation, differentiation, migration and elimination of cells, but also by a tight regulation of permeability and repair. The role of EGCs in controlling the properties of the IEB is quite well studied, and our laboratory has highlighted the crucial role of n-6 oxylipins in this regulation. Thus, the 15dPGJ2 induced differentiation and inhibited the proliferation of epithelial cells (52) (Figure 3). We also demonstrated the involvement of glial 11βPGF2α in the control of IEB repair via an increase in intestinal epithelial cell spreading (53) (Figure 3). Finally, we have demonstrated that another glial mediator, the 15-HETE, controls IEB paracellular permeability, both in vitro and in vivo (54) (Figure 3). Thus, EGCs represent a source of n-6 oxylipins responsible for IEB strengthening.
Motility regulation
Inhibitors of both COX isoforms increased the contractions of human longitudinal muscles induced by electrical stimulation and are atropine-sensitive. It has been suggested that cholinergic neurons are thought to be primarily modulated by COX-1 activity, whereas COX-2 acts primarily at the muscular level to decrease muscarinic responses (50). Another study on rat duodenum also showed that inhibition of COX isoforms with indomethacin increased motility, probably via enteric nerve excitation (57). While the expression of COX1 and COX2 enzymes in neurons of myenteric ganglia of mouse or human colon, their role in the neuromuscular function is unclear. The inhibition of COX does not have the same effects on neuromuscular functions (peristalsis, spontaneous or Ach-induced activity, electrically-induced contractions, etc.…) depending on the species, but also according to the intestinal region (stomach, jejunum, distal colon, etc.) or the studied muscle layer (circular/longitudinal) (58). In addition, as enteric neurons can be the target but also the source of COX-pathway metabolites, and as many studies used COX inhibition or deletion without neuronal specification, it is difficult to conclude about the specific neuronal contribution (58) (Figure 3).
The LOX pathway has also been involved in motility regulation as a recent work demonstrate how the 12-HETE, can remodel the ENS and inhibit duodenal contraction (59).
Secretory regulation
The prostaglandins PGD2, E2, I2 and F2a as well as LTC4 increased short-circuit current, which represents chloride secretion and activated submucosal enteric neurons. The secretory response was reduced by treatment with tetrodotoxin or atropine, suggesting an involvement of cholinergic submucosal neurons in this regulation (60–62). Nevertheless, the prostaglandin regulation of secretion through ENS could be more complex, being accelerated with high concentrations of PGE2 (63) or sometimes presenting anti-secretory effect for rat colonic preparations treated with PGD2 (64, 65).
Immune system regulation
Prostanoids and leukotrienes are mediator of communication between macrophages or mast cells and ENS, but the ENS to immune cell communication through n-6 oxylipins has not been described yet.
One study demonstrated that in a co-culture of mast cells with rat submucosal neurons, inhibition of 5-LOX, resulted in a reduction of the number of responding neurons (66). In the same way, in small intestine of guinea pig sensitized to cow’s milk and exposed to beta-lactoglobulin, was observed an increase in neuronal excitability, both suppressed by treatment with a COX or 5-LOX inhibitors (67). Finally, one study suggests the involvement of macrophage-like cells found in rat myenteric plexuses in the effect of IL1β and IL-6 on the suppression of prostanoid-mediated norepinephrine release (37).
n-6 Oxylipins and ENS dysfunctions in gut disorders
In parallel to the involvement of the crosstalk between n-6 oxylipin and the ENS in the maintenance of intestinal homeostasis, increasing evidence suggest their dysregulation and role in different pathological conditions of the gut. Although numerous pre-clinical and clinical studies showed controversial results concerning the use of oxylipins or PUFAs, there is still much more to discover about the beneficial effects of these molecules, particularly in the field of IBD (68).
Inflammatory bowel diseases
The role of oxylipins in inflammatory bowel processes is multifaceted and unclear. Many studies associate n-6 with proinflammatory effects, and thereby an increased risk of developing IBD (8, 69–71). Conversely, many derivatives of ARA could also play an anti-inflammatory and pro-resolutive role (72), and treatment with COX inhibitors led to an exacerbation of the activity of the disease or induce relapse in IBD patients (73, 74). More recently, two eicosanoid synthesis pathway enzymes (PTGIS and PGD2 synthase) were identified among genes dysregulated in fibrotic Crohn’s disease (75). In the same manner, the concentration of a cluster of 10 eicosanoids (PGF2a, 15-HETE, TxB2, 11β PGF2α, PGE3, 15dPGJ2, 6kPGF1α, PGE2, PGD2 and 8 isoPGA2) were decreased in biospie supernatant form UC or CD patients, when biopsies came from a non-inflamed areas (76). These studies suggest that, while upregulated by acute inflammation, the defects in expression of synthesis enzyme and/or the low production of n-6 oxylipins are associated with chronic inflammation.
These changes of n-6 oxylipins production could be related to alterations of EGCs and enteric neurons observed in IBD patients. Indeed, in addition to neuronal structural alterations (77), increase in neuronal degeneration (78–81), and changes in neurochemical coding (82–85), neurons of the myenteric plexuses from active IBD patients expressed high level of COX2 that could contribute to the dysmotility observed in patients (86). Indeed, a decrease in the number of myenteric neurons per ganglion was observed after the induction colitis with TNBS in guinea pigs and especially an increase in COX2 expression and release of PGE2, TXA2 and LTB4 in the neuromuscular layer, was associated with a decrease in colonic propulsion and an increase in the excitability of myenteric neurons (87). In addition to neurons, EGCs from IBD patients present structural alterations (80), changes in glial marker expression (77, 88, 89) and a decrease in the production of four glial lipid mediators: the 15-HETE, 18-HEPE, 15dPGJ2 and 11βPGF2α (53) (Figure 3). The defect in 15dPGJ2 and 11βPGF2α production was explained by a decrease in L-PGDS and AKR1C3 synthesis enzyme expression by EGCs from CD patients (53). The functional consequences of the decrease of 15-HETE or 11βPGF2α productions by CD EGC have been identified as a loss of control of the IEB permeability or healing, respectively (53, 54) (Figure 3). Similarly, the decrease of 15dPGJ2 could be linked to a loss of neuroprotection from oxidative stress (44) and a defect in the proliferation and differentiation of intestinal epithelial cells (52) (Figure 3).
Thus, while most of the studies are focused on an active role of n-6 oxylipins in the deleterious effect of intestinal inflammation, we have to reconsider their necessity in the resolution of inflammation (90, 91) and the possible involvement of their defects in the ENS as a contributing factor to the pathological mechanisms of IBD.
Infectious diseases
Other studies suggest a detrimental role of n-6 oxylipins that target the ENS in several infectious diseases, especially during parasitic infections.
The Trypanosoma cruzi infection leads to myenteric neuron cell death mainly in the esophagus and colon and results in megaesophagus and megacolon, respectively (92). A treatment with low-dose aspirin during the acute phase of infection, known to inhibit COX, reduced parasitemia and protects myenteric neurons from cell death and plastic changes, notably their hypertrophy. This protection of cholinergic neurons was also associated with a recovered transit time by the aspirin treatment. In addition, treatment with aspirin decreases substance P and increases vasoactive intestinal peptide (VIP) productions, and could therefore help to regulate inflammation in these animals.
The infection with the parasite Cryptosporidium in piglet, induces intestinal villous damages associated with malabsorption and reduced sodium chloride (NaCl) absorption due to prostaglandin released from the inflamed tissue. The prostaglandins alter NaCl transport in this infection primarily by stimulating cholinergic interneurons that innervate VIPergic and cholinergic motor nerves (93). The ENS may be a potential target for pharmacological control of the acute diarrhea in this infection.
Other intestinal disorders
The involvement of n-6 oxylipins has also been suggested in many other pathologies associated with intestinal disorders, particularly via their role on neuronal excitation or their inhibitory role on intestinal contractility.
Patients with irritable bowel syndrome (IBS) are characterized by abdominal cramps, diarrhea or constipation. Treatments with the PGD2 antagonist BW A868C inhibited the longitudinal muscle contractions potentiated by supernatant of biopsies from IBS patient (94). In addition to this effect on motility, lipidomic studies have demonstrated that n-6 oxylipins can participate in visceral sensitivity. Quantification of the PUFA metabolites produced in biopsies from control and IBS patients have shown that 5,6-EET and PGE2 production are increased in diarrhea-predominant IBS patients and 5-oxoeicosatetraenoic acid (5-oxoETE) in IBS with predominant constipation (95, 96). These productions are correlated with pain and bloating and these oxylipins can activate afferent sensory neurons but the contribution of ENS in these processes is not described.
In the same way, about half of the patients with slow-transit constipation exhibits an elongated colon and outlet obstruction. One study showed increased levels of COX2 in muscularis and mucosa of partially obstructed mice, associated with colon elongation, slowing of transit, and loss of mucosal reflexes. The expression of COX2 was also found in subpopulations of nNos myenteric neurons, and PGE2 application completely abolished spontaneous inhibitory junction potentials and depolarize the circular muscle, suggesting that it was removing tonic inhibition. Removal of the obstruction or treatment with a selective COX2 inhibitor restored control parameters as spontaneous inhibitory junction potentials and mucosal reflexes (97). This study suggested that an increase in COX2 expression led to a hyperexcitable colon, largely due to suppression of inhibitory nerve pathways by prostaglandins.
In the case of food allergies, products of activated mast cells, including prostanoids and leukotrienes, are also known to stimulate nerve endings, to modulate neuronal excitability and to increase synaptic transmission, resulting in phenotypically and functionally altered submucosal secretomotor neurons (98).
In the context of postoperative ileus, a study showed, in the rat, that a bowel manipulation increased the expression of COX2, associated with an increased PGE2 release in the immune cells but also in subpopulation of myenteric neurons. This associated with a decrease in contractile smooth muscle activity (99). COX2 metabolites such as PGE2 produced by the ENS appear to be involved in enteric dysmotility, and administration of specific inhibitors could help to prevent the transit delay induced by bowel manipulation (58). The PGE2 produced by the ENS is not only involved in smooth muscle control, but also in epithelial proliferation, and a study nicely shown how tumor cells can induce PGE2 production by EGCs to activate colon cancer stem cells and stimulate tumorigenesis (100).
Finally, some ARA derivatives could also have an emetogenic role, and one study investigated the role of leukotrienes in this effect. Injections of different leukotrienes, such as LTC4 and D4 induced an increase in the frequency and percentage of least shrews receiving vomiting. Following LTC4-induced vomiting, they showed increased labeling of nuclei for Fos in ENS, suggesting that leukotrienes modulate emetic activity in part via enteric nervous components activation (101).
Conclusion
Nowadays, the crosstalk between n-6 oxylipins and the ENS is mainly represented by n-6 oxylipins regulation of neuronal activity and the subsequent modulation of muscle contraction Nevertheless, first evidence shows that n-6 oxylipins could also have a broader impact through their production by enteric glia to the control of the intestinal epithelial properties. The EGC-derived n-6 oxylipins that strengthen the intestinal epithelial barrier and participate in gut homeostasis, are lacking in patients with Crohn’s disease. We could speculate that they could also regulate other EGC neighboring cells such as ILC3, macrophages or T cells, and therefore could more broadly participate in pathological processes. New studies must go beyond the conventional wisdom of inflammatory prostaglandins, bring a better understanding of the kinetics, receptor type and cellular mechanisms regulated by specific n-6 oxylipins in order to elaborate new anti-inflammatory strategies, for example via supplementation with pro-resolutive mediators. Pharmacological approaches coupled with tissue-specific gene invalidation might facilitate the translation of fundamental discoveries into clinical solutions for gut diseases with aberrant lipid signaling.
Author contributions
MR-D and PD directed, constructed and produced the final version of the review. MM wrote the first draft. MR-D produced the data. KB-N conducted a critical reading. MN participated in the reflexion and work funding. All authors contributed to the article and approved the submitted version.
Funding
This work was supported by the “Institut national de la santé et de la recherche médicale (Inserm),” the Nantes Université, the SantéDige Foundation and the cluster IBD NExt from Nantes Excellence Trajectory (NExT Health and Engineering). MM benefits a doctoral fellowship managed by Bba Milk Valley, dairy industrial association. We are grateful to Regional Councils of BRetagne (grant no. 19008213) and Pays de la Loire (grant no. 2019-013227) for their financial support through the interregional project PROLIFIC. MR-D is supported by the Centre National pour la Recherche Scientifique (CNRS).
Acknowledgments
The authors thank Camille Pochard for the first draft of the manuscript.
Conflict of interest
The authors declare that the research was conducted in the absence of any commercial or financial relationships that could be construed as a potential conflict of interest.
Publisher’s note
All claims expressed in this article are solely those of the authors and do not necessarily represent those of their affiliated organizations, or those of the publisher, the editors and the reviewers. Any product that may be evaluated in this article, or claim that may be made by its manufacturer, is not guaranteed or endorsed by the publisher.
Abbreviations
15d-PGJ2, 15-Deoxy-Delta-12,14-prostaglandin J2; Ach, acethylcholine; AKR1C3, aldo-keto reductase family 1 member C3; ARA, arachidonic acid; BDNF, brain-derived neurotrophic factor; S100b, calcium-binding protein S100b; CNS, central nervous system; CD, Crohn’s disease; COX, cyclooxygenases; CYP, cytochrome P450; DGLA, dihomo-γ-linolenic acid; DHA, docosahexaenoic acid; EPA, eicosapentaenoic acid; EGCs, enteric glial cells; ENS, enteric nervous system; EETs, epoxyeicosatrienoic acids; EGF, epidermal growth factor; GI, gastrointestinal tract; GDNF, glial derived neurotrophic factor; GFAP, glial fibrillary acidic protein; HpETE, hydroperoxyeicosatetraenoic acid; HETE, hydroxyeicosatetraenoic acids; IBD, inflammatory bowel diseases; IL-1b, Interleukin-1β; IBS, irritable bowel syndrome; LT, leukotrienes; LA, linoleic acid; LOX, lipoxygenase; LMMP, longitudinal muscle/myenteric plexus; oxoETE, oxoeicosatetraenoic acid; PPAR, peroxisome proliferator-activated receptor; PG, prostaglandin; PGDS, PGD synthetase; PGES, PGE synthetase; PGFS, PGF synthetase; PTGIS, PGI synthetase; PLA2, phospholipase A2; PUFAs, polyunsaturated fatty acids; PTGS, prostaglandin-endoperoxide synthase; PG, prostaglandins; NaCl, sodium chloride; TX, thromboxanes; TXAS, TX synthetase; UC, ulcerative colitis; VIP, vasoactive intestinal peptide; ALA, α-linolenic acid.
References
2.Vergnolle, N, and Cirillo, C. Neurons and glia in the enteric nervous system and epithelial barrier function. Physiology (Bethesda). (2018) 33:269–80. doi: 10.1152/physiol.00009.2018
3.Pochard, C, Coquenlorge, S, Freyssinet, M, Naveilhan, P, Bourreille, A, Neunlist, M, et al. The multiple faces of inflammatory enteric glial cells: is Crohn’s disease a gliopathy? Am J Physiol Gastrointest Liver Physiol. (2018) 315:G1–G11. doi: 10.1152/ajpgi.00016.2018
4.Ikehata, A, Hiwatashi, N, Kinouchi, Y, Yamazaki, H, Ito, K, and Toyota, T. Altered leukotriene B4 metabolism in colonic mucosa with inflammatory bowel disease. Scand J Gastroenterol Janv. (1995) 30:44–9. doi: 10.3109/00365529509093234
5.Ligumsky, M, Karmeli, F, Sharon, P, Zor, U, Cohen, F, and Rachmilewitz, D. Enhanced thromboxane A2 and prostacyclin production by cultured rectal mucosa in ulcerative colitis and its inhibition by steroids and sulfasalazine. Gastroenterology. (1981) 81:444–9. doi: 10.1016/0016-5085(81)90594-1
6.Shannon, VR, Stenson, WF, and Holtzman, MJ. Induction of epithelial arachidonate 12-lipoxygenase at active sites of inflammatory bowel disease. Am J Phys. (1993) 264:G104–11. doi: 10.1152/ajpgi.1993.264.1.G104
7.Sharon, P, Ligumsky, M, Rachmilewitz, D, and Zor, U. Role of prostaglandins in ulcerative colitis. Enhanced production during active disease and inhibition by sulfasalazine. Gastroenterology. (1978) 75:638–40. doi: 10.1016/S0016-5085(19)31672-5
8.Hawkey, CJ, Karmeli, F, and Rachmilewitz, D. Imbalance of prostacyclin and thromboxane synthesis in Crohn’s disease. Gut. (1983) 24:881–5. doi: 10.1136/gut.24.10.881
9.Lauritsen, K, Staerk Laursen, L, Bukhave, K, and Rask-Madsen, J. Longterm olsalazine treatment: pharmacokinetics, tolerance and effects on local eicosanoid formation in ulcerative colitis and Crohn’s colitis. Gut. (1988) 29:974–82. doi: 10.1136/gut.29.7.974
10.Le Loupp, AG, Bach-Ngohou, K, Bourreille, A, Boudin, H, Rolli-Derkinderen, M, Denis, MG, et al. Activation of the prostaglandin D2 metabolic pathway in Crohn’s disease: involvement of the enteric nervous system. BMC Gastroenterol. (2015) 15:112. doi: 10.1186/s12876-015-0338-7
11.Zifroni, A, Treves, AJ, Sachar, DB, and Rachmilewitz, D. Prostanoid synthesis by cultured intestinal epithelial and mononuclear cells in inflammatory bowel disease. Gut. (1983) 24:659–64. doi: 10.1136/gut.24.7.659
12.Masoodi, M, Pearl, DS, Eiden, M, Shute, JK, Brown, JF, Calder, PC, et al. Altered colonic mucosal polyunsaturated fatty acid (PUFA) derived lipid mediators in ulcerative colitis: new insight into relationship with disease activity and pathophysiology. PLoS One. (2013) 8:e76532. doi: 10.1371/journal.pone.0076532
13.Ajuebor, MN, Singh, A, and Wallace, JL. Cyclooxygenase-2-derived prostaglandin D(2) is an early anti-inflammatory signal in experimental colitis. Am J Physiol Gastrointest Liver Physiol. (2000) 279:G238–44. doi: 10.1152/ajpgi.2000.279.1.G238
14.Gilroy, DW, Colville-Nash, PR, McMaster, S, Sawatzky, DA, Willoughby, DA, and Lawrence, T. Inducible cyclooxygenase-derived 15-deoxy(Delta)12-14PGJ2 brings about acute inflammatory resolution in rat pleurisy by inducing neutrophil and macrophage apoptosis. FASEB J. (2003) 17:2269–71. doi: 10.1096/fj.02-1162fje
15.Hokari, R, Kurihara, C, Nagata, N, Aritake, K, Okada, Y, Watanabe, C, et al. Increased expression of lipocalin-type-prostaglandin D synthase in ulcerative colitis and exacerbating role in murine colitis. Am J Physiol Gastrointest Liver Physiol. (2011) 300:G401–8. doi: 10.1152/ajpgi.00351.2010
16.Li, J, Kong, D, Wang, Q, Wu, W, Tang, Y, Bai, T, et al. Niacin ameliorates ulcerative colitis via prostaglandin D2-mediated D prostanoid receptor 1 activation. EMBO Mol Med. (2017) 9:571–88. doi: 10.15252/emmm.201606987
17.Rajakariar, R, Hilliard, M, Lawrence, T, Trivedi, S, Colville-Nash, P, Bellingan, G, et al. Hematopoietic prostaglandin D2 synthase controls the onset and resolution of acute inflammation through PGD2 and 15-deoxyDelta12 14 PGJ2. Proc Natl Acad Sci U S A. (2007) 104:20979–84. doi: 10.1073/pnas.0707394104
18.Vong, L, Ferraz, JGP, Panaccione, R, Beck, PL, and Wallace, JL. A pro-resolution mediator, prostaglandin D(2), is specifically up-regulated in individuals in long-term remission from ulcerative colitis. Proc Natl Acad Sci U S A. (2010) 107:12023–7. doi: 10.1073/pnas.1004982107
19.Gobbetti, T, Ducheix, S, le Faouder, P, Perez, T, Riols, F, Boue, J, et al. Protective effects of n-6 fatty acids-enriched diet on intestinal ischaemia/reperfusion injury involve lipoxin A4 and its receptor. Br J Pharmacol. (2015) 172:910–23. doi: 10.1111/bph.12957
20.Das, UN. Inflammatory bowel disease as a disorder of an imbalance between pro- and anti-inflammatory molecules and deficiency of resolution bioactive lipids. Lipids Health Dis. (2016) 15:11. doi: 10.1186/s12944-015-0165-4
21.Dennis, EA, and Norris, PC. Eicosanoid storm in infection and inflammation. Nat Rev Immunol. (2015) 15:511–23. doi: 10.1038/nri3859
22.Mazaleuskaya, LL, and Ricciotti, E. Druggable Prostanoid pathway. Adv Exp Med Biol. (2020) 1274:29–54. doi: 10.1007/978-3-030-50621-6_3
23.Mustonen, AM, and Nieminen, P. Dihomo-γ-Linolenic acid (20:3n-6)-metabolism, derivatives, and potential significance in chronic inflammation. Int J Mol Sci. (2023) 24:2116. doi: 10.3390/ijms24032116
24.Gabbs, M, Leng, S, Devassy, JG, Monirujjaman, M, and Aukema, HM. Advances in Our Understanding of Oxylipins Derived from Dietary PUFAs. Adv Nutr. (2015) 6:513–40.
25.Tanabe, T, and Tohnai, N. Cyclooxygenase isozymes and their gene structures and expression. Prostaglandins Other Lipid Mediat. (2002) 68-69:95–114. doi: 10.1016/S0090-6980(02)00024-2
26.Narumiya, S. Prostanoids in immunity: roles revealed by mice deficient in their receptors. Life Sci. (2003) 74:391–5. doi: 10.1016/j.lfs.2003.09.025
27.Rossi, P, Lindgren, JA, Kullman, C, and Jondal, M. Products of the lipoxygenase pathway in human natural killer cell cytotoxicity. Cell Immunol. (1985) 93:1–8. doi: 10.1016/0008-8749(85)90383-1
28.Naruhn, S, Meissner, W, Adhikary, T, Kaddatz, K, Klein, T, Watzer, B, et al. 15-hydroxyeicosatetraenoic acid is a preferential peroxisome proliferator-activated receptor beta/delta agonist. Mol Pharmacol. (2010) 77:171–84. doi: 10.1124/mol.109.060541
29.Shappell, SB, Gupta, RA, Manning, S, Whitehead, R, Boeglin, WE, Schneider, C, et al. 15S-Hydroxyeicosatetraenoic acid activates peroxisome proliferator-activated receptor gamma and inhibits proliferation in PC3 prostate carcinoma cells. Cancer Res. (2001) 61:497–503.
30.Saitoh, N, Fujimoto, R, Ishii, T, Nishio, H, Takeuchi, T, and Hata, F. Muscarinic autoinhibition and modulatory role of protein kinase C in acetylcholine release from the myenteric plexus of Guinea pig ileum. Jpn J Pharmacol. (1997) 74:155–63. doi: 10.1016/S0021-5198(19)31404-0
31.Yau, WM, Bowen, DJ, and Youther, ML. Evidence for an involvement of eicosanoids in neurokinin3-receptor mediated acetylcholine release from myenteric neurons. Neurosci Lett. (1991) 129:259–61. doi: 10.1016/0304-3940(91)90475-9
32.Das, M, and Ganguly, DK. Effect of prostaglandin E2 on acetylcholine release from some peripheral cholinergic nerve terminals. Eur J Pharmacol. (1984) 100:41–6. doi: 10.1016/0014-2999(84)90313-3
33.Fukunaga, Y, Mine, Y, Yoshikawa, S, Takeuchi, T, Hata, F, and Yagasaki, O. Role of prostacyclin in acetylcholine release from myenteric plexus of guinea-pig ileum. Eur J Pharmacol. (1993) 233:237–42. doi: 10.1016/0014-2999(93)90055-M
34.Takeuchi, T, Hata, F, and Yagasaki, O. Role of cyclic AMP in prostaglandin-induced modulation of acetylcholine release from the myenteric plexus of Guinea pig ileum. Jpn J Pharmacol. (1992) 60:327–33. doi: 10.1254/jjp.60.327
35.Takeuchi, T, Okuda, M, and Yagasaki, O. The differential contribution of endogenous prostaglandins to the release of acetylcholine from the myenteric plexus of the Guinea-pig ileum. Br J Pharmacol. (1991) 102:381–5. doi: 10.1111/j.1476-5381.1991.tb12182.x
36.Yoshikawa, S, Fukunaga, Y, Takeuchi, T, Nishio, H, and Hata, F. Modulation of acetylcholine release from the myenteric plexus of Guinea-pig ileum by 5-lipoxygenase metabolites of arachidonic acid. Eur. J. Pharmacol. [Internet]. (1993) 239:261–4. doi: 10.1016/0014-2999(93)91008-B
37.Rühl, A, Berezin, I, and Collins, SM. Involvement of eicosanoids and macrophage-like cells in cytokine-mediated changes in rat myenteric nerves. Gastroenterology. (1995) 109:1852–62. doi: 10.1016/0016-5085(95)90752-1
38.Mameya, S, Sawa, T, and Taniyama, K. Arachidonic acid cascade and stimulation of acetylcholine release by human recombinant interleukin-1 beta in Guinea pig ileum. J Pharmacol Exp Ther. (1995) 275:319–24.
39.Dekkers, JA, Akkermans, LM, and Kroese, AB. Effects of the inflammatory mediator prostaglandin E2 on myenteric neurons in Guinea pig ileum. Am J Phys. (1997) 272:G1451–6. doi: 10.1152/ajpgi.1997.272.6.G1451
40.Kelles, A, Janssens, J, and Tack, J. Electrical behaviour of interleukin-1 beta (IL-1 beta) and prostaglandin-E2 (PGE2) on colonic myenteric neurones. Neurogastroenterol Motil. (2002) 14:321–30. doi: 10.1046/j.1365-2982.2002.00336.x
41.Manning, BP, Sharkey, KA, and Mawe, GM. Effects of PGE2 in Guinea pig colonic myenteric ganglia. Am J Physiol Gastrointest Liver Physiol. (2002) 283:G1388–97. doi: 10.1152/ajpgi.00141.2002
42.Dou, D, Chen, L, Di, H, Song, Z, Li, S, Bu, X, et al. Vasopressin augments TNBS-induced colitis through enteric neuronal V1a receptor-mediated COX-2-dependent prostaglandin release from mast cells in mice. Neurogastroenterol. Motility. (2019) 31:e13493. doi: 10.1111/nmo.13493
43.Frieling, T, Rupprecht, C, Kroese, AB, and Schemann, M. Effects of the inflammatory mediator prostaglandin D2 on submucosal neurons and secretion in Guinea pig colon. Am J Phys. (1994) 266:G132–9. doi: 10.1152/ajpgi.1994.266.1.G132
44.Abdo, H, Mahé, MM, Derkinderen, P, Bach-Ngohou, K, Neunlist, M, and Lardeux, B. The omega-6 fatty acid derivative 15-deoxy-Δ12,14-prostaglandin J2 is involved in neuroprotection by enteric glial cells against oxidative stress. J Physiol. (2012) 590:2739–50. doi: 10.1113/jphysiol.2011.222935
45.Baudry, C, Reichardt, F, Marchix, J, Bado, A, Schemann, M, Des Varannes, SB, et al. Diet-induced obesity has neuroprotective effects in murine gastric enteric nervous system: involvement of leptin and glial cell line-derived neurotrophic factor. J Physiol. (2012) 590:533–44. doi: 10.1113/jphysiol.2011.219717
46.Stenkamp-Strahm, C, Patterson, S, Boren, J, Gericke, M, and Balemba, O. High-fat diet and age-dependent effects on enteric glial cell populations of mouse small intestine. Auton Neurosci. (2013) 177:199–210. doi: 10.1016/j.autneu.2013.04.014
47.Surrel, F, Jemel, I, Boilard, E, Bollinger, JG, Payré, C, Mounier, CM, et al. Group X phospholipase A2 stimulates the proliferation of colon cancer cells by producing various lipid mediators. Mol Pharmacol. (2009) 76:778–90. doi: 10.1124/mol.108.053371
48.Masuda, S, Murakami, M, Takanezawa, Y, Aoki, J, Arai, H, Ishikawa, Y, et al. Neuronal expression and neuritogenic action of group X secreted phospholipase A2. J Biol Chem. (2005) 280:23203–14. doi: 10.1074/jbc.M500985200
49.Bernardini, N, Colucci, R, Mattii, L, Segnani, C, Fornai, M, de Giorgio, R, et al. Constitutive expression of cyclooxygenase-2 in the neuromuscular compartment of normal human colon. Neurogastroenterol Motil août. (2006) 18:654–62. doi: 10.1111/j.1365-2982.2006.00795.x
50.Fornai, M, Blandizzi, C, Colucci, R, Antonioli, L, Bernardini, N, Segnani, C, et al. Role of cyclooxygenases 1 and 2 in the modulation of neuromuscular functions in the distal colon of humans and mice. Gut. (2005) 54:608–16. doi: 10.1136/gut.2004.053322
51.Porcher, C, Horowitz, B, Bayguinov, O, Ward, SM, and Sanders, KM. Constitutive expression and function of cyclooxygenase-2 in murine gastric muscles. Gastroenterology. (2002) 122:1442–54. doi: 10.1053/gast.2002.33065
52.Bach-Ngohou, K, Mahé, MM, Aubert, P, Abdo, H, Boni, S, Bourreille, A, et al. Enteric glia modulate epithelial cell proliferation and differentiation through 15-deoxy-12,14-prostaglandin J2. J Physiol. (2010) 588:2533–44. doi: 10.1113/jphysiol.2010.188409
53.Coquenlorge, S, Van Landeghem, L, Jaulin, J, Cenac, N, Vergnolle, N, Duchalais, E, et al. The arachidonic acid metabolite 11β-ProstaglandinF2α controls intestinal epithelial healing: deficiency in patients with Crohn’s disease. Sci Rep. (2016) 6:25203. doi: 10.1038/srep25203
54.Pochard, C, Coquenlorge, S, Jaulin, J, Cenac, N, Vergnolle, N, Meurette, G, et al. Defects in 15-HETE production and control of epithelial permeability by human enteric glial cells from patients with Crohn’s disease. Gastroenterology. (2016) 150:168–80. doi: 10.1053/j.gastro.2015.09.038
55.Rehn, M, Hild, D, and Diener, M. Upregulation of cyclooxygenase-2 and thromboxane A2 production mediate the action of tumor necrosis factor-alpha in isolated rat myenteric ganglia. Am J Physiol Gastrointest Liver Physiol. (2005) 289:G586–91. doi: 10.1152/ajpgi.00020.2005
56.Murakami, M, Ohta, T, and Ito, S. Lipopolysaccharides enhance the action of bradykinin in enteric neurons via secretion of interleukin-1beta from enteric glial cells. J Neurosci Res. (2009) 87:2095–104. doi: 10.1002/jnr.22036
57.Nylander, O, Hällgren, A, and Sababi, M. COX inhibition excites enteric nerves that affect motility, alkaline secretion, and permeability in rat duodenum. Am J Physiol Gastrointest Liver Physiol. (2001) 281:G1169–78. doi: 10.1152/ajpgi.2001.281.5.G1169
58.Fornai, M, Antonioli, L, Colucci, R, Bernardini, N, Ghisu, N, Tuccori, M, et al. Emerging role of cyclooxygenase isoforms in the control of gastrointestinal neuromuscular functions. Pharmacol Ther. (2010) 125:62–78. doi: 10.1016/j.pharmthera.2009.09.007
59.Abot, A, Wemelle, E, Laurens, C, Paquot, A, Pomie, N, Carper, D, et al. Identification of new enterosynes using prebiotics: roles of bioactive lipids and mu-opioid receptor signalling in humans and mice. Gut. (2021) 70:1078–87. doi: 10.1136/gutjnl-2019-320230
60.Frieling, T, Rupprecht, C, Dobreva, G, and Schemann, M. Differential effects of inflammatory mediators on ion secretion in the Guinea-pig colon. Comp Biochem Physiol A Physiol. (1997) 118:341–3. doi: 10.1016/S0300-9629(96)00315-5
61.Frieling, T, Becker, K, Rupprecht, C, Dobreva, G, Häussinger, D, and Schemann, M. Leukotriene-evoked cyclic chloride secretion is mediated by enteric neuronal modulation in Guinea-pig colon. Naunyn Schmiedeberg's Arch Pharmacol. (1997) 355:625–30. doi: 10.1007/PL00004993
62.Schulzke, JD, Pfaffenbach, S, Fromm, A, Epple, HJ, Troeger, H, and Fromm, M. Prostaglandin I(2) sensory input into the enteric nervous system during distension-induced colonic chloride secretion in rat colon. Acta Physiol (Oxf). (2010) 199:305–16. doi: 10.1111/j.1748-1716.2010.02096.x
63.Karaki, SI, and Kuwahara, A. Regulation of intestinal secretion involved in the interaction between neurotransmitters and prostaglandin E2. Neurogastroenterol Motil. (2004) 16:96–9. doi: 10.1111/j.1743-3150.2004.00482.x
64.Goerg, KJ, Diener, C, Diener, M, and Rummel, W. Antisecretory effect of prostaglandin D2 in rat colon in vitro: action sites. Am J Phys. (1991) 260:G904–10. doi: 10.1152/ajpgi.1991.260.6.G904
65.Diener, M, Nobles, M, Schmitt, C, and Rummel, W. Characterization of the antisecretory action of prostaglandin D2 in the rat colon. Acta Physiol Scand. (1992) 145:19–24. doi: 10.1111/j.1748-1716.1992.tb09331.x
66.Bell, A, Althaus, M, and Diener, M. Communication between mast cells and rat submucosal neurons. Pflugers Arch. (2015) 467:1809–23. doi: 10.1007/s00424-014-1609-9
67.Liu, S, Hu, HZ, Gao, N, Gao, C, Wang, G, Wang, X, et al. Neuroimmune interactions in Guinea pig stomach and small intestine. Am J Physiol Gastrointest Liver Physiol. (2003) 284:G154–64. doi: 10.1152/ajpgi.00241.2002
68.Ungaro, F, Rubbino, F, Danese, S, and D’Alessio, S. Actors and factors in the resolution of intestinal inflammation: lipid mediators as a new approach to therapy in inflammatory bowel diseases. Front Immunol. (2017) 8:1331. doi: 10.3389/fimmu.2017.01331
69.Hou, JK, Abraham, B, and El-Serag, H. Dietary intake and risk of developing inflammatory bowel disease: a systematic review of the literature. Am J Gastroenterol. (2011) 106:563–73. doi: 10.1038/ajg.2011.44
70.Shoda, R, Matsueda, K, Yamato, S, and Umeda, N. Epidemiologic analysis of Crohn disease in Japan: increased dietary intake of n-6 polyunsaturated fatty acids and animal protein relates to the increased incidence of Crohn disease in Japan. Am J Clin Nutr. (1996) 63:741–5. doi: 10.1093/ajcn/63.5.741
71.Sharon, P, and Stenson, WF. Metabolism of arachidonic acid in acetic acid colitis in rats. Similarity to human inflammatory bowel disease. Gastroenterology. (1985) 88:55–63. doi: 10.1016/S0016-5085(85)80132-3
72.Gobbetti, T, Le Faouder, P, Bertrand, J, Dubourdeau, M, Barocelli, E, Cenac, N, et al. Polyunsaturated fatty acid metabolism signature in ischemia differs from reperfusion in mouse intestine. PLoS One. (2013) 8:e75581. doi: 10.1371/journal.pone.0075581
73.Felder, JB, Korelitz, BI, Rajapakse, R, Schwarz, S, Horatagis, AP, and Gleim, G. Effects of nonsteroidal antiinflammatory drugs on inflammatory bowel disease: a case-control study. Am J Gastroenterol. (2000) 95:1949–54. doi: 10.1111/j.1572-0241.2000.02262.x
74.Meyer, AM, Ramzan, NN, Heigh, RI, and Leighton, JA. Relapse of inflammatory bowel disease associated with use of nonsteroidal anti-inflammatory drugs. Dig Dis Sci. (2006) 51:168–72. doi: 10.1007/s10620-006-3103-5
75.Sadler, T, Bhasin, JM, Xu, Y, Barnholz-Sloan, J, Chen, Y, Ting, AH, et al. Genome-wide analysis of DNA methylation and gene expression defines molecular characteristics of Crohn’s disease-associated fibrosis. Clin Epigenetics. (2016) 8:30. doi: 10.1186/s13148-016-0193-6
76.Pochard, C, Gonzales, J, Bessard, A, Mahe, MM, Bourreille, A, Cenac, N, et al. PGI2 inhibits intestinal epithelial permeability and apoptosis to alleviate colitis. Cell Mol Gastroenterol Hepatol. (2021) 12:1037–60. doi: 10.1016/j.jcmgh.2021.05.001
77.Villanacci, V, Bassotti, G, Nascimbeni, R, Antonelli, E, Cadei, M, Fisogni, S, et al. Enteric nervous system abnormalities in inflammatory bowel diseases. Neurogastroenterol Motil. (2008) 20:1009–16. doi: 10.1111/j.1365-2982.2008.01146.x
78.Ochoa-Cortes, F, Turco, F, Linan-Rico, A, Soghomonyan, S, Whitaker, E, Wehner, S, et al. Enteric glial cells: a new frontier in neurogastroenterology and clinical target for inflammatory bowel diseases. Inflamm Bowel Dis. (2016) 22:433–49. doi: 10.1097/MIB.0000000000000667
79.De Giorgio, R, Guerrini, S, Barbara, G, Stanghellini, V, De Ponti, F, Corinaldesi, R, et al. Inflammatory neuropathies of the enteric nervous system. Gastroenterology. (2004) 126:1872–83. doi: 10.1053/j.gastro.2004.02.024
80.Geboes, K, and Collins, S. Structural abnormalities of the nervous system in Crohn’s disease and ulcerative colitis. Neurogastroenterol Motil. (1998) 10:189–202. doi: 10.1046/j.1365-2982.1998.00102.x
81.Vasina, V, Barbara, G, Talamonti, L, Stanghellini, V, Corinaldesi, R, Tonini, M, et al. Enteric neuroplasticity evoked by inflammation. Auton Neurosci. (2006) 126-127:264–72. doi: 10.1016/j.autneu.2006.02.025
82.Bernstein, CN, Robert, ME, and Eysselein, VE. Rectal substance P concentrations are increased in ulcerative colitis but not in Crohn’s disease. Am J Gastroenterol. (1993) 88:908–13.
83.Neunlist, M, Aubert, P, Toquet, C, Oreshkova, T, Barouk, J, Lehur, PA, et al. Changes in chemical coding of myenteric neurones in ulcerative colitis. Gut. (2003) 52:84–90. doi: 10.1136/gut.52.1.84
84.Prigent, A, Chapelet, G, Guilhem, D, de Lataillade, A, Oullier, T, Durieu, E, et al. Tau accumulates in Crohn’s disease gut. FASEB J. (2020) 34:9285–96. doi: 10.1096/fj.202000414R
85.Prigent, A, Lionnet, A, Durieu, E, Chapelet, G, Bourreille, A, Neunlist, M, et al. Enteric alpha-synuclein expression is increased in Crohn’s disease. Acta Neuropathol. (2019) 137:359–61. doi: 10.1007/s00401-018-1943-7
86.Roberts, PJ, Morgan, K, Miller, R, Hunter, JO, and Middleton, SJ. Neuronal COX-2 expression in human myenteric plexus in active inflammatory bowel disease. Gut. (2001) 48:468–72. doi: 10.1136/gut.48.4.468
87.Linden, DR, Sharkey, KA, Ho, W, and Mawe, GM. Cyclooxygenase-2 contributes to dysmotility and enhanced excitability of myenteric AH neurones in the inflamed Guinea pig distal colon. J Physiol Lond. (2004) 557:191–205. doi: 10.1113/jphysiol.2004.062174
88.Cornet, A, Savidge, TC, Cabarrocas, J, Deng, WL, Colombel, JF, Lassmann, H, et al. Enterocolitis induced by autoimmune targeting of enteric glial cells: a possible mechanism in Crohn’s disease? Proc Natl Acad Sci U S A. (2001) 98:13306–11. doi: 10.1073/pnas.231474098
89.von Boyen, GBT, Schulte, N, Pflüger, C, Spaniol, U, Hartmann, C, and Steinkamp, M. Distribution of enteric glia and GDNF during gut inflammation. BMC Gastroenterol. (2011) 11:3. doi: 10.1186/1471-230X-11-3
90.Ho, GT, Cartwright, JA, Thompson, EJ, Bain, CC, and Rossi, AG. Resolution of inflammation and gut repair in IBD: translational steps towards complete mucosal healing. Inflamm Bowel Dis. (2020) 26:1131–43. doi: 10.1093/ibd/izaa045
91.Na, YR, Stakenborg, M, Seok, SH, and Matteoli, G. Macrophages in intestinal inflammation and resolution: a potential therapeutic target in IBD. Nat Rev Gastroenterol Hepatol. (2019) 16:531–43. doi: 10.1038/s41575-019-0172-4
92.Oda, JY, Belém, MO, Carlos, TM, Gouveia, R, Luchetti, BFC, Moreira, NM, et al. Myenteric neuroprotective role of aspirin in acute and chronic experimental infections with Trypanosoma cruzi. Neurogastroenterol Motil. (2017) 29:1–13. doi: 10.1111/nmo.13102
93.Argenzio, RA, Armstrong, M, and Rhoads, JM. Role of the enteric nervous system in piglet cryptosporidiosis. J Pharmacol Exp Ther. (1996) 279:1109–15.
94.Balestra, B, Vicini, R, Cremon, C, Zecchi, L, Dothel, G, Vasina, V, et al. Colonic mucosal mediators from patients with irritable bowel syndrome excite enteric cholinergic motor neurons. Neurogastroenterol Motil. (2012) 24:1118–e570. doi: 10.1111/nmo.12000
95.Bautzova, T, Hockley, JRF, Perez-Berezo, T, Pujo, J, Tranter, MM, Desormeaux, C, et al. 5-oxoETE triggers nociception in constipation-predominant irritable bowel syndrome through MAS-related G protein-coupled receptor D. Sci Signal. (2018) 11:1–13. doi: 10.1126/scisignal.aal2171
96.Cenac, N, Bautzova, T, Le Faouder, P, Veldhuis, NA, Poole, DP, Rolland, C, et al. Quantification and potential functions of endogenous agonists of transient receptor potential channels in patients with irritable bowel syndrome. Gastroenterology. (2015) 149:433–444.e7. doi: 10.1053/j.gastro.2015.04.011
97.Heredia, DJ, Grainger, N, McCann, CJ, and Smith, TK. Insights from a novel model of slow-transit constipation generated by partial outlet obstruction in the murine large intestine. Am J Physiol Gastrointest Liver Physiol. (2012) 303:G1004–16. doi: 10.1152/ajpgi.00238.2012
98.Undem, BJ, and Taylor-Clark, T. Mechanisms underlying the neuronal-based symptoms of allergy. J Allergy Clin Immunol. (2014) 133:1521–34. doi: 10.1016/j.jaci.2013.11.027
99.Schwarz, NT, Kalff, JC, Türler, A, Engel, BM, Watkins, SC, Billiar, TR, et al. Prostanoid production via COX-2 as a causative mechanism of rodent postoperative ileus. Gastroenterology. (2001) 121:1354–71. doi: 10.1053/gast.2001.29605
100.Valès, S, Bacola, G, Biraud, M, Touvron, M, Bessard, A, Geraldo, F, et al. Tumor cells hijack enteric glia to activate colon cancer stem cells and stimulate tumorigenesis. EBioMedicine. (2019) 49:172–88. doi: 10.1016/j.ebiom.2019.09.045
Keywords: omega-3, omega-6, enteric nervous system, diet, inflammation
Citation: Mantel M, Derkinderen P, Bach-Ngohou K, Neunlist M and Rolli-Derkinderen M (2023) Crosstalk between omega-6 oxylipins and the enteric nervous system: Implications for gut disorders? Front. Med. 10:1083351. doi: 10.3389/fmed.2023.1083351
Edited by:
Guillaume Sarrabayrouse, Université de Paris, FranceReviewed by:
Ahmed Ayedur Rahman, Harvard Medical School, United StatesValentina Caputi, University College Cork, Ireland
Vincent Rioux, Institut Agro Rennes-Angers, France
Copyright © 2023 Mantel, Derkinderen, Bach-Ngohou, Neunlist and Rolli-Derkinderen. This is an open-access article distributed under the terms of the Creative Commons Attribution License (CC BY). The use, distribution or reproduction in other forums is permitted, provided the original author(s) and the copyright owner(s) are credited and that the original publication in this journal is cited, in accordance with accepted academic practice. No use, distribution or reproduction is permitted which does not comply with these terms.
*Correspondence: Malvyne Rolli-Derkinderen, bWFsdnluZS5kZXJraW5kZXJlbkB1bml2LW5hbnRlcy5mcg==