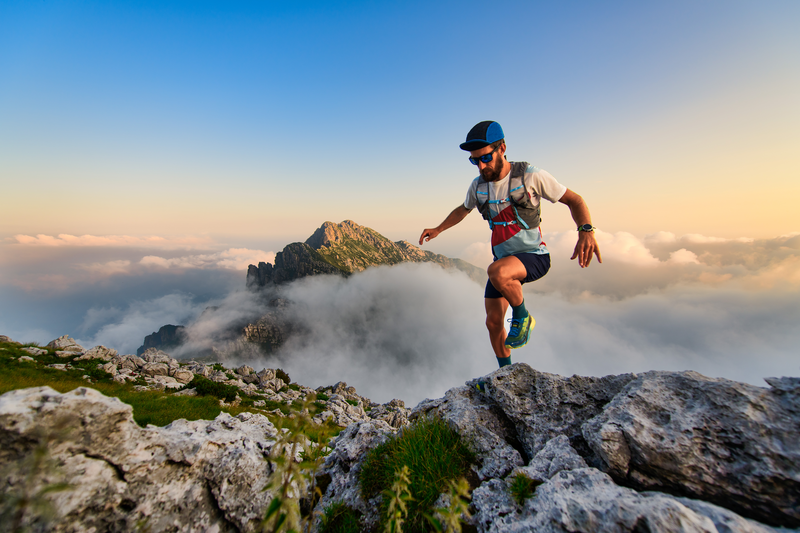
94% of researchers rate our articles as excellent or good
Learn more about the work of our research integrity team to safeguard the quality of each article we publish.
Find out more
ORIGINAL RESEARCH article
Front. Med. , 23 March 2023
Sec. Ophthalmology
Volume 10 - 2023 | https://doi.org/10.3389/fmed.2023.1037919
This article is part of the Research Topic Vascular Involvement in Eye Diseases View all 15 articles
Importance: Optical coherence tomography angiography (OCTA) has been widely applied into children, however, few studies have assessed the repeatability and reproducibility of papillary and peripapillary VD in healthy children.
Objective: To assess the precision of papillary and peripapillary vascular density (VD) measurements using optical coherence tomography angiography (OCTA) and analyze the effects of the signal strength index (SSI) and axial length (AL) on precision estimates.
Design, setting, and participants: This was a prospective observational study. Seventy-eight children aged 6–16 years underwent 4.5 × 4.5 mm OCTA (RTVue XR Avanti) disc scans: two scans by one examiner (repeatability) and two additional scans by another examiner (reproducibility). Within-subject standard deviation (Sw), test-retest reproducibility (TRT), within-subject coefficient of variation (CoV), intraclass correlation coefficient (ICC), and Bland–Altman analysis were performed.
Main outcomes and measures: In repeatability measurement, the fluctuation ranges (minimum to maximum) of VD between intraexaminer A/B in Sw, TRT, CoV, and ICC were (1.05–2.17)% / (1.16–2.32)%, (2.9–6)% / (3.21–6.44)%, (1.9–4.47)% / (2.08–5)%, and (0.588–0.783)% / (0.633–0.803)%, respectively. In reproducibility measurement, the fluctuation ranges of VD in Sw, TRT, CoV, and ICC were 1.11–2.13%, 3.07–5.91%, 1.99–4.41%, and 0.644–0.777%, respectively. VD was negatively correlated with SSI in most sectors of the peripapillary (e.g., inferior nasal, temporal inferior, temporal superior, superior temporal, and superior nasal). AL was positively correlated with inferior temporal VD and negatively correlated with superior nasal VD.
Conclusion and relevance: Optical coherence tomography angiography showed moderate-to-good repeatability and reproducibility for papillary and peripapillary perfusion measurements in healthy children. The SSI value affects most of the peripapillary VD, while AL affects only the temporal inferior and nasal superior peripapillary VD.
- To assess the precision of papillary and peripapillary vascular density (VD) measurements using optical coherence tomography angiography (OCTA), 78 children aged 6–16 years underwent 4.5 × 4.5 mm OCTA disc scans.
- OCTA showed moderate-to-good repeatability and reproducibility for papillary and peripapillary perfusion measurements in healthy children.
Examination of papillary and peripapillary blood flow in children can help understand the pathological mechanisms of some optic nerve diseases, such as juvenile glaucoma and optic neuritis (1, 2). Consequently, precise estimates of the parameters of the papillary and peripapillary blood flow are imperative.
With the advent of optical coherence tomography angiography (OCTA), it is now possible to non-invasive images and quantify macular, papillary and peripapillary vascular networks through fast scanning and image processing algorithms (3–5). Repeated measurements of blood flow parameters, such as vascular density (VD), will often fluctuate near the true value for the same retinal location of the same individual. It is important to assess the precision of repeated measurements to determine clinical changes in these blood flow parameters; therefore, they are considered when evaluating individual or longitudinal measurements.
Previous studies have shown that OCTA has high repeatability and reproducibility in measuring the area of the foveal avascular zone, superficial and deep retinal VD, and papillary and peripapillary VD in healthy adults (6–11). However, only a few studies have assessed the repeatability and reproducibility of these parameters in healthy children. Zhang et al. (12) reported that OCTA is reliable for evaluating macular perfusion in 8–16 years old children, but the algorithm was not applied to the measurement of papillary and peripapillary VD. Other studies have evaluated the impact of image magnification, associations with ocular biometry, and the effect of patient-specific factors on OCTA measurements (13–15).
In this study, the precision (repeatability and reproducibility) of OCTA was evaluated in measuring papillary and peripapillary VD in 6–16 years old healthy Chinese children in a large sample. Additional objectives were to provide reference values for clinical use and analyze the effects of the signal strength index (SSI) and axial length (AL) on the VD.
This prospective observational study was conducted at the Eye Hospital of Wenzhou Medical University. The study protocol was approved by the Medical Ethics Committee of the Eye Hospital of Wenzhou Medical University. Written informed consent was obtained from the legal guardian of each child.
Children aged 6–16 years attending the hospital were invited to participate in this study. The inclusion criteria were as follows: (1) monocular best-corrected distance visual acuity 20/20 or better; (2) refractive spheres between −5.00 D and +5.00 D and cylinder within ± 3 D; (3) intraocular pressure between 10 and 21 mmHg; (4) no retinal pathology; (5) no history of systemic disease.
The papillary and peripapillary VDs were generated by OCTA using the RTVue XR Avanti device (software RTVue, version 2018.1.1.63; Optovue Inc., Fremont, CA, USA) with the Angio Disc (4.5 × 4.5 mm) mode. The measurement principle of angiography and flow imaging has been reported in previous studies (6, 16).
Examiner A (WWL) acquired two repeated scans of the right eye for each patient. Examiner B (LHL) acquired two additional scans of the right eye. Two measurements were taken from previous studies to avoid the fatigue effect in children (17, 18). After the baseline scan was used as the reference scan, the follow-up scan option was chosen to automatically track the same location.
The software automatically segmented the papillary and peripapillary VD (Figure 1) between the inner limiting membrane and the nerve fiber layer. The VD was defined as the ratio of the area of the large and capillary vessels divided by the total area measured sectors. The capillary VD was the VD, except for the large vessels. According to the Garway-Heath Partition Method, the peripapillary area is subdivided into eight sectors: nasal superior, nasal inferior, inferior nasal, inferior tempo, tempo inferior, tempo superior, superior tempo, and superior nasal. Eyes with poor-quality images with OCTA (quality score < 8), significant motion, or blink artifacts were excluded from the analysis. The AL was measured using an IOLMaster 700 (Zeiss, Germany).
Statistical analyses were performed using Statistical Product and Service Solution (SPSS) statistical software V24.0. Only the right eye was analyzed. Kolmogorov–Smirnov analysis was used to test the normality of the data distribution. Normally distributed variables were described as mean ± standard deviation (SD). Median and interquartile ranges were used to describe non-normally distributed parameters.
The within-subject standard deviation (Sw) (19), test-retest repeatability (TRT) (20, 21), within-subject coefficient of variation (CoV) (22, 23), and intraclass correlation coefficient (ICC) (23, 24) were used to evaluate repeatability and reproducibility. The Sw value was taken as the square root of the residual mean. The TRT value was equal to the Sw multiplied by 2.77. An ICC value > 0.8 or < 0.4 indicates good or poor reproducibility between every two repeated measurements. The CoV value is Sw divided by the average measurement and is expressed as a percentage. A lower CoV indicates greater repeatability (25).
In addition, the Bland–Altman analysis was used to plot the differences in VD and SSI between interexaminer measurements and their means. The method uses the mean difference and 95% limits of agreement (LoAs) between the two examiner measurements. The significance level was set at P < 0.05.
To assess interexaminer reproducibility, the two examiners’ mean values between two measurements of each parameter were included in the analysis.
Generalized estimation equations were used to analyze the effects of SSI and AL on precision estimates.
Eighty-five children were enrolled in this study; seven were excluded due to motion and blink artifacts (two children) or poor image quality (five children). Therefore, the study included 78 children with a median age (interquartile range) of 10 (9–12) years (range, 6–16 years). The mean (± SD) of AL was 24.72 ± 1.26 mm. The mean spherical equivalent refraction was −2.72 ± 1.94 D.
For the repeatability of two measurements within intra-examiners A and B, the mean ± SD, Sw, TRT, CoV, and ICC of SSI of the whole images were 73.57 ± 8.03 and 72.46 ± 8.02, 3.91, and 3.49, 10.83, and 9.66, 5.31, and 4.81, 0.788 (0.686–0.859), and 0.827 (0.741–0.886), respectively. Table 1 and Figures 2, 3 show the intraexaminer repeatability in measuring papillary and peripapillary VD. The fluctuation ranges of VD between examiners A and B in Sw, TRT, CoV, and ICC were (1.05–2.17)% / (1.16–2.32)%, (2.9–6)% / (3.21–6.44)%, (1.9–4.47)% / (2.08–5)%, and (0.588–0.783) / (0.633–0.803), respectively.
Table 1. Intra-examiner repeatability of the OCTA in measuring the papillary and peripapillary vascular density.
Figure 2. Test-retest repeatability of papillary and peripapillary vascular density measurements intraexaminer and interexaminer.
Figure 3. Within-subject coefficient of papillary and peripapillary vascular density measurements intraexaminer and interexaminer.
In the interexaminer reproducibility measurement, the mean ± SD, Sw, TRT, CoV, and ICC of SSI were 73.01 ± 8.02, 4.74, 13.14, 6.50, and 0.652 (0.505–0.763). The fluctuation ranges of VD in Sw, TRT, CoV, and ICC were 1.11–2.13%, 3.07–5.91%, 1.99–4.41%, and 0.644–0.777, respectively, as shown in Table 2 and Figures 2, 3.
Table 2. Inter-examiner agreement of the OCTA in measuring the papillary and peripapillary vascular density.
In the reproducibility measurement of papillary and peripapillary VD, the interexaminer 95% LoA range for whole, whole capillary, disc, disc capillary, peripapillary, and peripapillary capillary was 6.0, 6.1, 9.9, 11.3, 6.6, and 7.2%, respectively, and other sectors were shown in Figure 4.
Figure 4. Panels (A–S) shows the Bland–Altman plots of reproducibility of interexaminer on papillary and peripapillary vascular density measurement. A solid line represents the mean difference. The upper and lower lines represent the 95% limit of agreement.
The effects of SSI and AL on the repeatability measurements for two examiners of papillary and peripapillary VD are shown in Tables 3, 4. Overall, the disc, disc capillary, and VD increased with increasing SSI. This relationship was the opposite in most sectors of the peripapillary, including the inferior nasal, temporal inferior, temporal superior, superior temporal, and superior nasal. In other sectors, there is no relationship between the two.
Table 3. Effect of signal strength index on the repeatability measurement of the papillary and peripapillary vascular density.
Table 4. Effect of axial length on the repeatability measurement of the papillary and peripapillary vascular density.
Axial length was positively correlated with VD in the temporal inferior sector, while in the nasal superior sector it was the opposite. In most other sectors, the AL did not correlate with the VD. There was no correlation between age and the repeatability of the papillary and peripapillary vascular density measurement (all P > 0.05), except for superior temporal sector in measurement of the examiner A, shown in Table 5.
Table 5. Effect of age on the repeatability measurement of the papillary and peripapillary vascular density.
Many previous studies have reported that OCTA showed moderate-to-good repeatability and reproducibility in adult measurements with small samples (1, 26–29). Although the OCTA measurement scan is fast, it should also be performed in some patients with poor cooperation, such as children. It is rarely reported in larger samples, particularly in the papillary and peripapillary regions of children. The present study showed moderate-to-good repeatability and reproducibility of the OCTA measurements of papillary and peripapillary VD in healthy Chinese children using comprehensive and systematic evaluation indicators.
In repeatability measurement, Venugopal et al. (26) reported that in 30 normal adult eyes (aged 57 years old), Sw, TRT, CoV, ICC of the papillary and each peripapillary sector were 1.3–2.6%, 3.3–7.0%, 2.4–4.4%, and 0.71–0.85, respectively, for three repeated measurements (1). Manalastas et al. (9) found that in two continuous optic nerve head scans of 15 healthy adults aged approximately 70 years old, the Sw, CoV, and ICC of the papillary and each peripapillary sector were 1.58–3.61%, 2.9–5.8%, and 0.42–0.80, respectively. Consistent with this study, Sw, TRT, CoV, and ICC of the papillary and each peripapillary sector in intraexaminer A/B for two repeated measurements were 1.09–2.17% / 1.16–2.32%, 2.94–6.0% / 3.21–6.44%, 1.9–4.47% / 2.08–5.00%, and 0.588–0.783 / 0.633–0.785. Venugopal et al. (26) reported that a TRT < 6% had no clinical significance. The TRT measured for reproducibility and repeatability in this study were lower than this value. Using the same OCTA equipment, the reliability of repeated measurements of papillary and peripapillary VD in children is close to that in adults.
Capillary density (CD) has a potential value in the evaluation of glaucoma (27). Mansoori et al. (28) visualized the radial peripapillary capillary network using OCTA. Repeatability measurements of CD have also been reported in the adult macula (18, 29, 30). Pappelis and Jansonius (17) reported two measurements of pericapillary CD in 30 healthy adult eyes using Canon OCT-HS 100 angiography; the ICC and TRT were 0.79 and 1.8%, respectively. Consistent with this study, the intra-examiner ICC of examiners A/B was 0.757 / 0.691, and the TRT was 3.54% / 4.18%. This shows that as the operational proficiency of the examiner and cooperation of children increases, the repeatability measurement of pericapillary CD in children approaches that of adults.
Rao et al. (31) found that the use of Cirrus HD-OCT (software version 11.0.0.29946) follow-up scans can make repeatability measurements more reliable compared to non-referenced scans in 33 normal adults (48 eyes), and they reported that the nasal, superior, temporal, and inferior peripapillary were 2.2, 2.2, 2.7, and 1.9% for TRT, 1.8, 2.0, 2.1, and 1.8% for CoV, 0.92, 0.92, 0.85, and 0.97, respectively. Venugopal et al. (26) included 46 eyes of 33 healthy adults using non-referenced high-density scans of RTVue-XR SD-OCT (AngioVue, V.2016.2.0.35) and reported that the repeatability estimates of the papillary and peripapillary sections ranged from 1.1 to 1.8% for Sw, 3.0 to 4.9% for TRT, and 2.0 to 3.1% for CoV. Based on follow-up scanning, this study found that the nasal superior, nasal inferior, inferior nasal, inferior temporal, temporal inferior, temporal superior, superior temporal, and superior nasal peripapillaries of intraexaminer A were 1.89, 2.06, 2.15, 2.17, 1.74, 1.62, 2.09, and 1.96% in Sw; 5.24, 5.72, 5.96, 6, 4.82, 4.5, 5.79, and 5.44% in TRT; 4.03, 4.44, 4.47, 3.96, 3.19, 2.9, 3.9, and 4.08% in CoV; and 0.732, 0.763, 0.765, 0.675, 0.717, 0.783, 0.711, and 0.747 in ICC. Differences in the results may be attributed to different sample sizes, including subjects, scanning mode, devices, and algorithms.
In assessing the reproducibility of interexaminer measurements, She et al. (32) reported that CoV was < 2% and ICC was > 0.8 in different sectors of the peripapillary in normal adults. The Bland–Altman plots showed that the 95% LoA range was 12.2% in the superotemporal, 10.1% in the temporal, 11.3% in the inferotemporal, 12.0% in the inferonasal, 8.0% in the nasal, and 7.4% in the superior peripapillary (32). In our study, CoV and TRT were < 4.5% and < 6%, respectively, and ICC was > 0.64. The Bland–Altman plots showed that the 95% LoA range was 9.3% in the nasal superior, 10.3% in the nasal inferior, 10.9% in the inferior nasal, 11.5% in the inferior temporal, 8.7% in the temporal superior, 9.1% in the temporal superior, and 10.8% in the superior temporal, 11.8% in the superior nasal regions. This shows that the reproducibility measurement for children is acceptable but remains lower than that for adults. The unstable fixation in children may affect the image quality and reliability of the measurement. She et al. (32) also found that the reproducibility and repeatability of measurements in different sectors were different, and this study also had similar findings. However, the reason for this difference is unknown.
Some studies have reported that SSI is positively correlated with macular and peripapillary VD, and clinicians should consider the effect of SSI on VD during follow-up studies (12, 15). Based on repeated measurements, Venugopal et al. (26) claimed that SSI was positively correlated with papillary and sectoral peripapillary VD. Lim et al. (33) showed that SSI was positively correlated with peripapillary microvascular VD through a 3 × 3 mm angiography scan. Interestingly, in the sample of children in this study, considering the two examiners repeated measurement factors, SSI was negatively correlated with VD in most peripapillary sectors. Similarly, Rao et al. (31) used Cirrus OCTA and found that SSI was only negatively correlated with peripapillary temporal VD, while there was no correlation in other sectors. Due to differences in scan area, partition algorithm, equipment, and included subjects, further research is needed on the influence of SSI on peripapillary VD measurement. In addition, the influence of physiological factors, such as small fluctuations in perfusion pressure, should also be considered.
Based on repeatability measurements, Lei et al. (34) reported that AL was only positively correlated with peripapillary temporal VD in Optovue and Triton OCTA; this correlation did not exist in other sectors. In the Spectralis and Cirrus OCTA, the positive correlation between AL and VD was reflected in the superior and inferior sectors, and there was no such correlation in other sectors (34). This study also showed that AL was only positively correlated with inferior temporal VD in Optovue OCTA. However, previous studies have demonstrated a negative correlation between peripapillary VD and AL (35, 36), and Sampson et al. (13) suggested that attention should be paid to the correction of AL in VD calculations. This study included healthy children and excluded subjects with high myopia and analyzed them based on repeated measurements by two examiners, which may be the source of differences in the results of the study.
This study has some limitations. First, this study only included Chinese children who used an OCTA device. The reliability of children’s measurements of multiple devices, including other ethnic groups, requires further research. Second, this study removed images with low-quality scores or significant motion and blinking artifacts. Previous studies have reported a large number of low quality OCTA images (37, 38). The latest version of the OCTA algorithm reduces image defects using real-time tracking. Finally, the repeatability and reproducibility of OCTA depend on multiple factors, including system parameters, imaging protocols, and subject compliance. System parameters include optical or mechanical stability and algorithm robustness. Imaging protocols include scan location, scan speed, and field of view: wide-field (39) or narrow field. Lastly, young/elderly subjects (26) and ocular disease (40) can lower the OCTA precision. We need to further observe the consistency of the measurements with a long-term follow-up.
This study reports the reliability of papillary and peripapillary VD measurements in healthy Chinese children. Our results demonstrated low intraexaminer and interexaminer variations in papillary and peripapillary perfusion parameters. Considering the factors of repeatability measurement by the two examiners, SSI affects most of the peripapillary VD, while AL only affects the temporal inferior and nasal peripapillary VD.
The original contributions presented in this study are included in the article/supplementary material, further inquiries can be directed to the corresponding authors.
The studies involving human participants were reviewed and approved by the Eye Hospital of Wenzhou Medical University. Written informed consent to participate in this study was provided by the participants’ legal guardian/next of kin. Written informed consent was obtained from the individual(s), and minor(s)’ legal guardian/next of kin, for the publication of any potentially identifiable images or data included in this article.
RC, XL, MY, QW, SC, and JH contributed to the conception and design of the study. ZZ, XyC, ZL, XC, and MS organized the database. HL performed the statistical analysis. RC wrote the first draft of the manuscript. WL, YY, CM, QW, SC, and JH wrote sections of the manuscript. All authors contributed to manuscript revision, read, and approved the submitted version.
This work was supported in part by Science and Technology Planning Project of Zhejiang Province (LGF19H120002); the Eye and ENT Hospital of Fudan University High-level Talents Program (2021318); the Zhejiang National Natural Science Foundation (LQ16B010002); the Wenzhou Bureau of Science and Technology (Y20160061 and Y2020037); the Medical and Health Science and Technology Program of Zhejiang Province (2019KY111); the Program for Professor of Special Appointment (Eastern Scholar) at Shanghai Institutions of Higher Learning; Multidisciplinary innovation team of traditional Chinese medicine for the treatment of myopia and amblyopia in children in Zhejiang Province; and the funders had no role in study design, data collection and analysis, decision to publish, or reparation of the manuscript.
The authors declare that the research was conducted in the absence of any commercial or financial relationships that could be construed as a potential conflict of interest.
All claims expressed in this article are solely those of the authors and do not necessarily represent those of their affiliated organizations, or those of the publisher, the editors and the reviewers. Any product that may be evaluated in this article, or claim that may be made by its manufacturer, is not guaranteed or endorsed by the publisher.
1. Werner A, Shen LQ. A review of OCT angiography in glaucoma. Semin Ophthalmol. (2019) 34:279–86. doi: 10.1080/08820538.2019.1620807
2. Abdelrahman A, Eltanamly R, Elsanabary Z, Hassan L. Optical coherence tomography angiography in juvenile open angle glaucoma: correlation between structure and perfusion. Int Ophthalmol. (2021) 41:883–9. doi: 10.1007/s10792-020-01643-7
3. Spaide RF, Klancnik JM Jr, Cooney MJ. Retinal vascular layers imaged by fluorescein angiography and optical coherence tomography angiography. JAMA Ophthalmol. (2015) 133:45–50. doi: 10.1001/jamaophthalmol.2014.3616
4. Jia Y, Bailey S, Hwang T, McClintic S, Gao S, Pennesi M, et al. Quantitative optical coherence tomography angiography of vascular abnormalities in the living human eye. Proc Natl Acad Sci USA. (2015) 112:E2395–402. doi: 10.1073/pnas.1500185112
5. Savastano MC, Lumbroso B, Rispoli M. In vivo characterization of retinal vascularization morphology using optical coherence tomography angiography. Retina. (2015) 35:2196–203. doi: 10.1097/iae.0000000000000635
6. Coscas F, Sellam A, Glacet-Bernard A, Jung C, Goudot M, Miere A, et al. Normative data for vascular density in superficial and deep capillary plexuses of healthy adults assessed by optical coherence tomography angiography. Invest Ophthalmol Vis Sci. (2016) 57:OCT211–23. doi: 10.1167/iovs.15-18793
7. Yu J, Jiang C, Wang X, Zhu L, Gu R, Xu H, et al. Macular perfusion in healthy Chinese: an optical coherence tomography angiogram study. Invest Ophthalmol Vis Sci. (2015) 56:3212–7. doi: 10.1167/iovs.14-16270
8. Iafe N, Phasukkijwatana N, Chen X, Sarraf D. Retinal capillary density and foveal avascular zone area are age-dependent: quantitative analysis using optical coherence tomography angiography. Invest Ophthalmol Vis Sci. (2016) 57:5780–7. doi: 10.1167/iovs.16-20045
9. Manalastas P, Zangwill L, Saunders L, Mansouri K, Belghith A, Suh M, et al. Reproducibility of optical coherence tomography angiography macular and optic nerve head vascular density in glaucoma and healthy eyes. J Glaucoma. (2017) 26:851–9. doi: 10.1097/IJG.0000000000000768
10. You Q, Freeman W, Weinreb R, Zangwill L, Manalastas P, Saunders L, et al. Reproducibility of vessel density measurement with optical coherence tomography angiography in eyes with and without retinopathy. Retina. (2017) 37:1475–82. doi: 10.1097/IAE.0000000000001407
11. Shahlaee A, Samara W, Hsu J, Say E, Khan M, Sridhar J, et al. In vivo assessment of macular vascular density in healthy human eyes using optical coherence tomography angiography. Am J Ophthalmol. (2016) 165:39–46. doi: 10.1016/j.ajo.2016.02.018
12. Zhang Z, Huang X, Meng X, Chen T, Gu Y, Wu Y, et al. In vivo assessment of macula in eyes of healthy children 8 to 16 years old using optical coherence tomography angiography. Sci Rep. (2017) 7:8936. doi: 10.1038/s41598-017-08174-9
13. Sampson D, Gong P, An D, Menghini M, Hansen A, Mackey D, et al. Axial length variation impacts on superficial retinal vessel density and foveal avascular zone area measurements using optical coherence tomography angiography. Invest Ophthalmol Vis Sci. (2017) 58:3065–72. doi: 10.1167/iovs.17-21551
14. Tai E, Li L, Wan-Hazabbah W, Wong T, Shatriah I. Effect of axial eye length on retinal vessel parameters in 6 to 12-year-old malay girls. PLoS One. (2017) 12:e0170014. doi: 10.1371/journal.pone.0170014
15. Rao H, Pradhan Z, Weinreb R, Reddy H, Riyazuddin M, Sachdeva S, et al. Determinants of peripapillary and macular vessel densities measured by optical coherence tomography angiography in normal eyes. J Glaucoma. (2017) 26:491–7. doi: 10.1097/IJG.0000000000000655
16. Bazvand F, Mirshahi R, Fadakar K, Faghihi H, Sabour S, Ghassemi F. The quantitative measurements of vascular density and flow area of optic nerve head using optical coherence tomography angiography. J Glaucoma. (2017) 26:735–41. doi: 10.1097/IJG.0000000000000722
17. Pappelis K, Jansonius N. Quantification and repeatability of vessel density and flux as assessed by optical coherence tomography angiography. Transl Vis Sci Technol. (2019) 8:3. doi: 10.1167/tvst.8.3.3
18. Al-Sheikh M, Tepelus T, Nazikyan T, Sadda S. Repeatability of automated vessel density measurements using optical coherence tomography angiography. Br J Ophthalmol. (2017) 101:449–52. doi: 10.1136/bjophthalmol-2016-308764
19. Maassen G. The two errors of using the within-subject standard deviation (Wsd) as the standard error of a reliable change index. Arch Clin Neuropsychol. (2010) 25:451–6. doi: 10.1093/arclin/acq036
20. Huang J, Savini G, Wu F, Yu X, Yang J, Yu A, et al. Repeatability and reproducibility of ocular biometry using a new noncontact optical low-coherence interferometer. J. Cataract Refract Surg. (2015) 41:2233–41. doi: 10.1016/j.jcrs.2015.10.062
21. Huang J, Ding X, Savini G, Pan C, Feng Y, Cheng D, et al. A comparison between scheimpflug imaging and optical coherence tomography in measuring corneal thickness. Ophthalmology. (2013) 120:1951–8. doi: 10.1016/j.ophtha.2013.02.022
22. Tian L. Inferences on the within-subject coefficient of variation. Stat Med. (2006) 25:2008–17. doi: 10.1002/sim.2330
23. Huang J, Savini G, Hu L, Hoffer K, Lu W, Feng Y, et al. Precision of a new scheimpflug and placido-disk analyzer in measuring corneal thickness and agreement with ultrasound pachymetry. J Cataract Refract Surg. (2013) 39:219–24. doi: 10.1016/j.jcrs.2012.10.034
24. Xue C, Yuan J, Lo G, Chang A, Poon D, Wong O, et al. Radiomics feature reliability assessed by intraclass correlation coefficient: a systematic review. Quant Imaging Med Surg. (2021) 11:4431–60. doi: 10.21037/qims-21-86
25. Wang Q, Ding X, Savini G, Chen H, Feng Y, Pan C, et al. Anterior chamber depth measurements using scheimpflug imaging and optical coherence tomography: repeatability, reproducibility, and agreement. J Cataract Refract Surg. (2015) 41:178–85. doi: 10.1016/j.jcrs.2014.04.038
26. Venugopal J, Rao H, Weinreb R, Dasari S, Riyazuddin M, Pradhan Z, et al. Repeatability and comparability of peripapillary vessel density measurements of high-density and non-high-density optical coherence tomography angiography scans in normal and glaucoma eyes. Br J Ophthalmol. (2019) 103:949–54. doi: 10.1136/bjophthalmol-2018-312401
27. Rao H, Kadambi S, Weinreb R, Puttaiah N, Pradhan Z, Rao D, et al. Diagnostic ability of peripapillary vessel density measurements of optical coherence tomography angiography in primary open-angle and angle-closure glaucoma. Br J Ophthalmol. (2017) 101:1066–70. doi: 10.1136/bjophthalmol-2016-309377
28. Mansoori T, Sivaswamy J, Gamalapati J, Agraharam S, Balakrishna N. Measurement of radial peripapillary capillary density in the normal human retina using optical coherence tomography angiography. J Glaucoma. (2017) 26:241–6. doi: 10.1097/ijg.0000000000000594
29. Lei J, Durbin M, Shi Y, Uji A, Balasubramanian S, Baghdasaryan E, et al. Repeatability and reproducibility of superficial macular retinal vessel density measurements using optical coherence tomography angiography en face images. JAMA Ophthalmol. (2017) 135:1092–8. doi: 10.1001/jamaophthalmol.2017.3431
30. Alnawaiseh M, Brand C, Bormann E, Sauerland C, Eter N. Quantification of macular perfusion using optical coherence tomography angiography: repeatability and impact of an eye-tracking system. BMC Ophthalmol. (2018) 18:123. doi: 10.1186/s12886-018-0789-z
31. Rao HL, Dasari S, Riyazuddin M, Lavanya R, Nk P, Pradhan ZS, et al. Referenced scans improve the repeatability of optical coherence tomography angiography measurements in normal and glaucoma eyes. Br J Ophthalmol. (2020) 105:1542–7. doi: 10.1136/bjophthalmol-2020-316480
32. She X, Guo J, Liu X, Zhu H, Li T, Zhou M, et al. Reliability of vessel density measurements in the peripapillary retina and correlation with retinal nerve fiber layer thickness in healthy subjects using optical coherence tomography angiography. Ophthalmologica. (2018) 240:183–90. doi: 10.1159/000485957
33. Lim H, Kim Y, Nam K, Ryu C, Jo Y, Kim J. Signal strength as an important factor in the analysis of peripapillary microvascular density using optical coherence tomography angiography. Sci Rep. (2019) 9:16299. doi: 10.1038/s41598-019-52818-x
34. Lei J, Pei C, Wen C, Abdelfattah N. Repeatability and reproducibility of quantification of superficial peri-papillary capillaries by four different optical coherence tomography angiography devices. Sci Rep. (2018) 8:17866. doi: 10.1038/s41598-018-36279-2
35. Wang X, Kong X, Jiang C, Li M, Yu J, Sun X. Is the peripapillary retinal perfusion related to myopia in healthy eyes? A prospective comparative study. BMJ Open. (2016) 6:e010791. doi: 10.1136/bmjopen-2015-010791
36. Mo J, Duan A, Chan S, Wang X, Wei W. Vascular flow density in pathological myopia: an optical coherence tomography angiography study. BMJ Open. (2017) 7:e013571. doi: 10.1136/bmjopen-2016-013571
37. Spaide R, Fujimoto J, Waheed N. Image artifacts in optical coherence tomography angiography. Retina. (2015) 35:2163–80. doi: 10.1097/IAE.0000000000000765
38. Suh M, Zangwill L, Manalastas P, Belghith A, Yarmohammadi A, Medeiros F, et al. Optical coherence tomography angiography vessel density in glaucomatous eyes with focal lamina cribrosa defects. Ophthalmology. (2016) 123:2309–17. doi: 10.1016/j.ophtha.2016.07.023
39. Hong J, Tan B, Quang N, Gupta P, Lin E, Wong D, et al. Intra-session repeatability of quantitative metrics using widefield optical coherence tomography angiography (OCTA) in elderly subjects. Acta Ophthalmol. (2019) 98:e570–8. doi: 10.1111/aos.14327
Keywords: vascular density, optical coherence tomography angiography, repeatability, reproducibility, children
Citation: Chen R, Liu X, Yao M, Zou Z, Chen X, Li Z, Chen X, Su M, Lian H, Lu W, Yang Y, McAlinden C, Wang Q, Chen S and Huang J (2023) Precision (repeatability and reproducibility) of papillary and peripapillary vascular density measurements using optical coherence tomography angiography in children. Front. Med. 10:1037919. doi: 10.3389/fmed.2023.1037919
Received: 06 September 2022; Accepted: 27 February 2023;
Published: 23 March 2023.
Edited by:
Fabrizio Giansanti, University of Florence, ItalyReviewed by:
Bingyao Tan, Nanyang Technological University, SingaporeCopyright © 2023 Chen, Liu, Yao, Zou, Chen, Li, Chen, Su, Lian, Lu, Yang, McAlinden, Wang, Chen and Huang. This is an open-access article distributed under the terms of the Creative Commons Attribution License (CC BY). The use, distribution or reproduction in other forums is permitted, provided the original author(s) and the copyright owner(s) are credited and that the original publication in this journal is cited, in accordance with accepted academic practice. No use, distribution or reproduction is permitted which does not comply with these terms.
*Correspondence: Qinmei Wang, d2FuZ3FtNTVAMTI2LmNvbQ==; Shihao Chen, Y2hlbmxlQHJvY2tldG1haWwuY29t; Jinhai Huang, dmlwOTk5dmlwQDE2My5jb20=
†These authors have contributed equally to this work and share first authorship
Disclaimer: All claims expressed in this article are solely those of the authors and do not necessarily represent those of their affiliated organizations, or those of the publisher, the editors and the reviewers. Any product that may be evaluated in this article or claim that may be made by its manufacturer is not guaranteed or endorsed by the publisher.
Research integrity at Frontiers
Learn more about the work of our research integrity team to safeguard the quality of each article we publish.