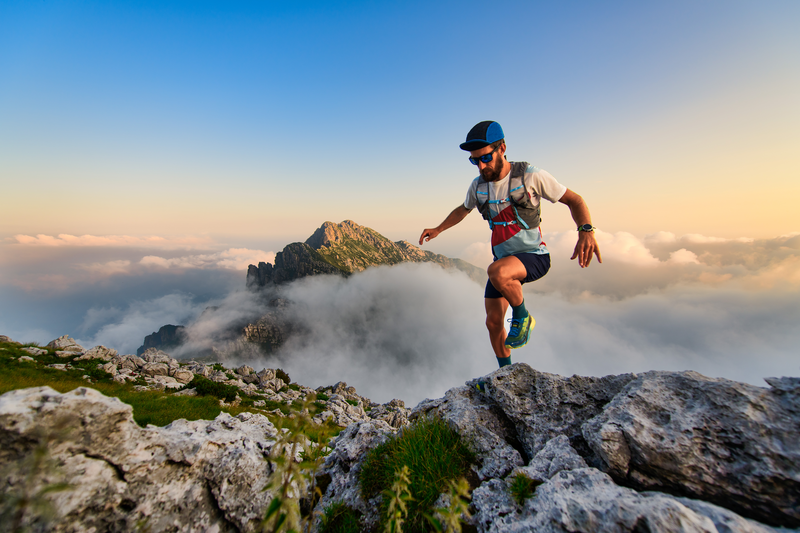
94% of researchers rate our articles as excellent or good
Learn more about the work of our research integrity team to safeguard the quality of each article we publish.
Find out more
SYSTEMATIC REVIEW article
Front. Med. , 16 December 2022
Sec. Intensive Care Medicine and Anesthesiology
Volume 9 - 2022 | https://doi.org/10.3389/fmed.2022.999225
This article is part of the Research Topic Learning to Live With a Persistent Pandemic: Challenges of Balancing Resources between COVID-19 and Non-COVID-19 Patients In a New Era of Healthcare View all 9 articles
Background and aim: With the Coronavirus Disease 2019 (COVID-19) pandemic continuing to impact healthcare systems around the world, healthcare providers are attempting to balance resources devoted to COVID-19 patients while minimizing excess mortality overall (both COVID-19 and non-COVID-19 patients). To this end, we conducted a systematic review (SR) to describe the effect of the COVID-19 pandemic on all-cause excess mortality (COVID-19 and non-COVID-19) during the pandemic timeframe compared to non-pandemic times.
Methods: We searched EMBASE, Cochrane Database of SRs, MEDLINE, Cumulative Index to Nursing and Allied Health Literature (CINAHL) and Cochrane Controlled Trials Register (CENTRAL), from inception (1948) to December 31, 2020. We used a two-stage review process to screen/extract data. We assessed risk of bias using Newcastle-Ottawa Scale (NOS). We used Critical Appraisal and Grading of Recommendations Assessment, Development and Evaluation (GRADE) methodology.
Results: Of 11,581 citations, 194 studies met eligibility. Of these studies, 31 had mortality comparisons (n = 433,196,345 participants). Compared to pre-pandemic times, during the COVID-19 pandemic, our meta-analysis demonstrated that COVID-19 mortality had an increased risk difference (RD) of 0.06% (95% CI: 0.06–0.06% p < 0.00001). All-cause mortality also increased [relative risk (RR): 1.53, 95% confidence interval (CI): 1.38–1.70, p < 0.00001] alongside non-COVID-19 mortality (RR: 1.18, 1.07–1.30, p < 0.00001). There was “very low” certainty of evidence through GRADE assessment for all outcomes studied, demonstrating the evidence as uncertain.
Interpretation: The COVID-19 pandemic may have caused significant increases in all-cause excess mortality, greater than those accounted for by increases due to COVID-19 mortality alone, although the evidence is uncertain.
Systematic review registration: [https://www.crd.york.ac.uk/prospero/#recordDetails], identifier [CRD42020201256].
– Question: What was the total burden of all-cause mortality during the COVID-19 pandemic, inclusive of COVID-19 mortality, non-COVID-19 mortality, and all-cause mortality?
– Findings: In this systematic review of 31 observational studies (n = 433,196,345 participants), there was a significant increase in all-cause excess mortality through the COVID-19 pandemic as compared to pre-pandemic time periods. This increase in excess mortality is only partially explained by COVID-19 deaths, as there was a substantial number of non-COVID-19 patients affected by the pandemic time period as well.
– Meaning: The COVID-19 pandemic may have caused a significant amount of death, both from COVID-19 related illness and non-COVID-19 illness alike. Monitoring all-cause excess mortality may be a better measure to ascertain the COVID-19 pandemic’s full impact on mortality.
As of June 23, 2022, the World Health Organization (WHO) has reported over 545 million cases and over 6.3 million deaths from coronavirus disease 2019 (COVID-19) (1). However, assessing the true mortality from COVID-19 is challenging. Confounding factors include: (1) lack of testing availability or policies leading to undertesting (2); (2) varying COVID-19 diagnostic criteria (e.g., suspected cases vs. confirmed cases, nucleic acid vs. antigen vs. antibody testing) (3); (3) testing sensitivity and specificity (e.g., rapid antigen test vs. polymerase chain reaction) (4–6). This can create uncertainty in producing accurate mortality data surrounding the pandemic. There is evidence to suggest non-COVID-19 mortality was excessive during the COVID-19 pandemic, due to disruptions in healthcare provisions and changes in acute care hospitalizations, leading to increased all-cause morbidity and mortality (7). Therefore, following COVID-19 mortality alone does not accurately estimate the full impact of the pandemic.
It is crucial to evaluate total excess (e.g., avoidable or unanticipated) mortality during the pandemic, as compared to non-pandemic times (8, 9). Multiple studies have demonstrated that COVID-19 deaths can be underestimated (10–12), where measuring total excess mortality can better capture the total impact of the pandemic, and total excess mortality may be a worthwhile measure to calculate attributable mortality from the pandemic (13). The ability to adapt and innovate during these periods of global disruption is key to mitigating the adverse effects of the pandemic on global health, both related to the immediate and prolonged complications of COVID-19, alongside any negative pandemic influences on non-COVID-19 mortality (7).
To this end, we conducted a systematic review (SR) to describe the total burden of all-cause excess mortality during the pandemic (inclusive of both COVID-19 and non-COVID-19 mortality). The purpose of this SR is to inform clinicians, health policymakers (e.g., public health officials, ethicists, politicians), and patients to ensure that policies enacted are representative of the full breadth of impact of the pandemic for all patients.
In populations around the world [population], what impact did the COVID-19 pandemic (intervention) as compared to the pre-pandemic era (comparator) have on excess mortality of both COVID-19 and non-COVID-19 patients (outcomes) during pre-pandemic and pandemic periods (time)?
This SR was conducted in line with Preferred Reporting Items for Systematic Reviews and Meta-Analyses (PRISMA) guidelines (14), with International Prospective Register of Systematic Reviews (PROSPERO) on September 2, 2020 (CRD42020201256). Our PRISMA checklist is included in Supplementary Table 1. This paper specifically focused on COVID-19 related excess mortality alongside all-cause excess mortality (with non-COVID-19 mortality) (7).
We systematically searched Ovid EMBASE, Cochrane Database of Systematic Reviews, MEDLINE, Cumulative Index to Nursing and Allied Health Literature (CINAHL) and Cochrane Controlled Trials Register (CENTRAL), from inception (1948) to December 31, 2020. Searches were performed by a clinical librarian (DKL) and underwent Peer Review Electronic Search Strategy (PRESS) (15) by a second researcher (MS). The search criteria are summarized in Supplementary Appendix 1.
The following keywords (topic/subject and keywords) were used, alongside alternative word spellings and endings: excess mortality; pandemic; non-pandemic time periods; outcomes. For each specific electronic database, individual search parameters were adjusted for syntax, field names, and search terms. Supplementary searches and bibliographies of relevant studies were also explored.
The COVID-19 pandemic exposure time period was defined as December 31, 2019 (first initial reports of pneumonia of unknown etiology to the WHO) (16), and forward. The non-COVID-19 pandemic control time period was defined as before December 31, 2019 (7). There was variability among the time periods cited, hence a broader definition was chosen to include studies that used data early in the pandemic.
Inclusion criteria were: (1) randomized control trials (RCTs), (2) observational studies and case series with control groups; (3) adult patients (>18 years old); and (4) must compare mortality outcomes in time periods during and before the COVID-19 pandemic. All animal and pediatric studies were excluded. We excluded all non-peer reviewed websites and non-research conference abstracts. No language restrictions were applied.
At least two independent reviewers (DL, SD, HC, PG, BM, AV, KL, BK, DC, and AA) evaluated and assessed for eligibility of each of the citations and utilized the previous eligibility criteria. Any citations selected by either reviewer was advanced to the full-text screening second stage. In the second stage, at least two reviewers reviewed the full-text articles for inclusion. Discussion with a third reviewer (VL) was used to resolve conflicts, if necessary. To manage screening and selection of studies, Covidence (Veritas Health Innovation, Melbourne, VIC, Australia) was used (17).
We developed an a priori data abstraction tool, which was piloted among all data abstractors (most demographics and baseline characteristics were extractable, however, excess mortality data were not routinely reported for pandemic and pre-pandemic time periods, limiting the number of studies pooled for analysis). We abstracted the following data points from included articles: study characteristics (title, author), patient group demographic/clinical data, interventions and comparators, clinical outcome data: mortality and jurisdiction(s) in which the study was performed. Supplementary Tables 2, 3 outline our data abstraction.
We used the Newcastle-Ottawa Scale (NOS) to evaluate the risk of bias (RoB) in non-randomized observational case-control and cohort studies. The following domains were assessed: selection (maximum score: four), comparability (maximum: two), and exposure (maximum: three). Each NOS scales for case-control/cohort studies are outlined in the footnotes of Supplementary Tables 4A, B (18). Study quality was deemed either: poor (selection domain: 0–1 star, comparability: 0, exposure: 0–1); fair (selection: 2, comparability: 1–2, exposure: 2–3); or good (selection: 3–4 domain, comparability: 1–2, exposure: 2–3) (18).
Grading of Recommendations Assessment, Development, and Evaluation (GRADE) methodology was used to assess publication bias, inconsistency, imprecision, indirectness, and RoB for the clinical outcomes of all-cause mortality, COVID-19 mortality, and non-COVID-19 mortality. Certainty of evidence was rated as high, moderate, low, or very low, with RCTs initially rated as high, and observational studies initially rated as low (19–21).
Continuous variables are displayed as medians and inter-quartile ranges (IQR) and/or means and standard deviations (SD), where appropriate. Comparisons were performed using a Wilcoxon rank sum test. Categorical variables were presented as counts and proportions and were assessed using Fischer’s exact tests or Pearson’s chi-squared test, where appropriate.
RevMan version 5.4 software (Copenhagen: The Nordic Cochrane Centre, Cochrane Collaboration 2014) was used to perform meta-analysis. The DerSimonian and Laird methodology was used to pool effect sizes. Study weights were measured using the inverse variance method using a random-effects model (22). Results were presented as relative risk (RR) or mean difference with 95% confidence intervals (CIs) (23). Heterogeneity was evaluated using the I2 statistic (>50% demonstrating significant heterogeneity). We also assessed the χ2 test for homogeneity (p < 0.1 demonstrating substantial heterogeneity). Subgroup analyses were attempted to determine if there were any methodological or clinical sources of heterogeneity. Publication bias was not formally assessed because in the meta-analysis there were fewer than 10 studies per outcome (23–25). However, we do present the funnel plots in Supplementary Figures 2A–C.
Due to the variations in reporting among the included studies, meta-analysis was completed for a subset of studies. The reasons for exclusion are outlined in Figure 1, which included inappropriate data format (did not include COVID-19 death, non-COVID-19 deaths, or all-cause deaths), duplication of data sets such as overlapping jurisdictions (only included the study with the largest number of participants), and incomplete data. We narratively summarized the eligible studies in terms of point estimates or proportions with p-values or 95% CIs. Significance was set at 0.05.
Potential methodologic or clinical sources of heterogeneity were examined to determine if significant heterogeneity existed. If enough trials were available, we attempted pre-specified subgroup analyses (hypothesized direction in parentheses) were considered for comparison:
• Low-middle income countries (LMIC) vs. high-income countries (HIC), as per the World Bank definition (26) (outcomes would favor high-income countries during both pandemic and non-pandemic times).
• High vs. low RoB studies (high RoB studies would favor non-pandemic usual care management outcomes).
• Medical vs. surgical vs. medical/surgical case-mixes (surgical cases would be favored during pandemic times compared to medical cases).
• Randomized control trials vs. observational studies (observational studies would favor non-pandemic usual care mortality outcomes).
• Pandemic time periods vs. non-pandemic time periods (would favor non-pandemic time period outcomes).
If a subgroup’s effects were deemed to be credible, outcomes were presented separately for each subgroup.
We attempted to contact the study authors for any missing data. If unavailable, we presented what data is available, and commented in the discussion on the potential impact of the missing data.
We identified 11,581 articles during our database search. Of these, 336 full texts were reviewed, with 31 eligible studies reporting mortality data (Figure 1). Table 1 presents a summary of the study characteristics. Supplementary Tables 2, 3 show complete study data, demographics, baseline characteristics, subgroups, and outcomes.
Table 1. Summary statistics of study design and characteristics of the studies included in COPES (n = 31).
Of the 31 studies (10, 27–56) reporting mortality data, 27 reported (10, 27–31, 33–41, 43–46, 48, 50–56) on all-cause mortality. Another 16 studies (10, 27, 28, 30, 31, 33–35, 38, 41, 47, 51–53, 55, 56) reported non-COVID-19 mortality data. There were 30 peer-reviewed publications (96.8%) (10, 27–49, 51–56) and 1 pre-print (3.2%) (10). There were 30 (96.8%) cohort observational studies (10, 27–46, 48–56), and 1 (3.2%) case-control (47) observational study with no randomized control trials. Research ethics board (REB) approval and consent were obtained in 6 (19.4%) studies (29, 36, 37, 45, 50, 53), 14 (45.2%) studies (10, 32, 35, 38–44, 47–49, 54) stated formal ethics approval was not required or the study was exempted while 11 (35.2%) studies (27, 28, 30, 33, 34, 46, 51, 52, 54–56) did not report REB approval or were not applicable (stated as none with no explanation). The setting for these studies were either acute care hospitals (7 studies, 22.6%) (37, 40, 42, 44, 47, 49, 50) or jurisdictional or national studies (24 studies, 77.4%) (10, 27–36, 38, 39, 41, 43, 45, 46, 48, 51–56). Nearly half of the studies (15 studies, 48.4%) had no funding or no funding declared (27, 31–35, 37, 38, 42, 46–48, 52, 55, 56). The majority of studies were performed in a single country (28/31 studies, 90.3%) (10, 27, 29–48, 50–53, 55, 56). There were no missing data from the studies included in the meta-analysis.
Supplementary Tables 4A, B demonstrate RoB for cohort and case-control studies, respectively (using the NOS).
For cohort studies (Supplementary Table 4A), full scores for NOS were found in only 1/30 studies (3.3%) (37). Common deficiencies were demonstrated in the following areas: lack of adequate length of follow-up (13 studies, 43.3%), lack of proper follow-up overall (19 studies, 63.3%), and lack of cohort comparability (19 studies, 63.3%).
For case-control studies (Supplementary Table 4B), 1/1 study (100%) had a full NOS score (47).
Supplementary Table 3 shows study outcomes, which demonstrated significant changes in mortality (primary outcome). GRADE assessment is shown in Supplementary Table 5. Supplementary Table 6 further summarized outcomes from Supplementary Table 3 in terms of demographics. There were increases in mortality, which disproportionally affected older individuals, males, racial minorities, those with comorbidities, and those with lower socioeconomic status.
Overall, 31 studies reported mortality comparisons between the COVID-19 pandemic and non-pandemic times, with 7 studies with sufficient data for meta-analysis for COVID-19 and non-COVID-19 mortality and 8 studies for all-cause mortality. These Forest plots are presented in Figures 2–4. For COVID-19 mortality (Figure 2), 7 studies (243,685 deaths out of 430,940,442 patients) were included in a meta-analysis, which demonstrated a significant increase in mortality [risk difference (RD): 0.06, 95% CI: 0.06–0.06%, p < 0.00001, τ2 = 0.0, I2 = 100%, “very low” certainty], as a proportion of the total population. This analysis was completed to quantify the COVID-19 mortality impact on the population and hence represented as a risk difference.
Figure 2. Forest plot for COVID-19 mortality. CI, confidence intervals; COVID-19, coronavirus disease 2019; IV, inverse variance.
Figure 3. Forest plot for all–cause excess mortality. CI, confidence intervals; COVID-19, coronavirus disease 2019; IV, inverse variance.
Figure 4. Forest plot for non-COVID-19 mortality. CI, confidence intervals; COVID-19, coronavirus disease 2019; IV, inverse variance.
For all-cause mortality (Figure 3), 8 studies (0.64% pandemic mortality vs. 0.54% pre-pandemic mortality) also demonstrated a significant increase in all-cause mortality when compared to baseline pre-pandemic mortality (RR: 1.53, 95% CI: 1.38–1.70, p < 0.00001, τ2 = 0.02, I2 = 100%, “very low” certainty).
For non-COVID-19 mortality (Figure 4), 7 studies (0.57% pandemic mortality vs. 0.54% pre-pandemic mortality) showed significant increases in non-COVID-19 mortality during the pandemic when compared to baseline pre-pandemic mortality (RR: 1.18, 1.07–1.30, p < 0.00001, τ2 = 0.02, I2 = 100%, “very low” certainty).
Supplementary Figure 1 shows a pre-specified subgroup Forest plot for mortality examining HIC vs. LMIC countries. A significant increase in all-cause mortality was shown in both HIC and LMIC during the COVID-19 pandemic as compared to non-COVID-19 pandemic historical controls. However, there was a greater increase in all-cause excess mortality in LMIC (RR 2.22, 95% CI: 1.55–3.19; p < 0.00001, τ2 = 0.07, I2 = 99%, “very low” certainty) as compared to HIC (RR 1.33, 95% CI: 1.26–1.41; p < 0.00001, τ2 = 0.0, I2 = 100%, “very low” certainty). The other subgroups were not subject to meta-analysis due to a lack of data.
Visual inspection of funnel plots was not formally assessed due to the limited number of studies (<10 studies) that can be used in the meta-analysis. The diagrams can be found in Supplementary Figures 2A–C which suggest the potential for publication bias due to the asymmetry of the plot.
This SR demonstrates the COVID-19 pandemic likely had increased excess all-cause mortality over and above attributable deaths from COVID-19 mortality alone, indicating non-COVID-19 mortality was also likely affected, although the strength of the evidence is very uncertain. In subgroup analyses, LMIC also had higher all-cause excess mortality as compared to HIC, demonstrating further inequities between rich and poor countries. Overall, all-cause mortality and non-COVID-19 mortality increased during the pandemic when compared to historical controls, although the certainty in the level of evidence is very low (the majority of the included observational studies had high RoB) and high heterogeneity makes any conclusions very uncertain.
During the pandemic, differences in COVID-19 mortality rates were found depending on location, case mix, population structure, health system responses, and capacity, alongside individual jurisdictional healthcare policies (e.g., lockdowns, vaccine access, etc.) (7, 57–60). Early during the pandemic, only hospitalized patients could have confirmatory testing for infection (61), which caused underreporting of COVID-19 cases by a factor of 2–3 times (62), with estimated COVID-19 deaths up 6–10 times higher than officially reported (63, 64). This underestimation was magnified in subsequent waves of COVID-19 variants, due to cases outstripping testing capabilities (65, 66), even in HIC (67). For this reason, it may be beneficial to contextualize COVID-19 mortality alongside all-cause excess mortality, which has been promoted prior by other authors (68, 69). This would allow a greater understanding of the full scope and impact the pandemic has had on COVID-19 and non-COVID-19 patients alike. The use of all-cause mortality in conjunction with COVID-19 mortality may serve to be a more effective metric, emphasizing the need to monitor all-cause excess mortality alongside COVID-19 mortality, and perhaps allow prioritization of resources to prevent excess avoidable deaths.
This SR reinforces the degree and scale of unintended consequences of the COVID-19 pandemic, adding to growing literature demonstrating the impact on all-cause excess and non-COVID-19 mortality (7, 10, 28, 30, 33–35, 43, 51–53, 69), in addition to COVID-19 mortality. The COVID-19 pandemic has already surpassed the death toll of many major world events (e.g., World War II 1939, H1N1 2009), and only outpaced by larger plagues of the past (e.g., 1918 Spanish Flu) (70). The impact of COVID-19 on mortality is highly stratified by country. Certain countries (e.g., India, USA, Brazil) had the highest excess mortality, while others (e.g., Australia, New Zealand, Taiwan, Singapore) have reported negative excess mortalities (69), likely due to their COVID-19 containment strategies and differences in healthcare resource allocation with decreased non-COVID-19 mortality from their populations staying home. Each jurisdictional strategy (e.g., COVID-19 elimination vs. mitigation, access to vaccinations and medications, demographic and geographic factors) has likely led to discrepancies in the global impact of all-cause excess mortality alongside COVID-19 mortality (7), which has varied throughout different waves of the pandemic (28, 69). The continued pandemic has had profound effects on the health of non-COVID-19 patients (7, 71), as pandemic patients have utilized resources, caused invoking of pandemic control measures, and disrupted care throughout the entire healthcare system (7). Furthermore, there are likely lasting impacts on our healthcare system (72, 73), and on those who have valiantly served during the pandemic, including mental health effects for patients and healthcare workers (72, 74, 75). There are high reported rates of burnout from the COVID-19 pandemic (74), particularly in frontline workers (75), which has led to attrition and further exacerbated staffing shortages (76), from which the healthcare system may never fully recover. This could further exacerbate all-cause excess mortality.
Our strengths include rigorous adherence to SR methodology, consisting of: (1) broad eligibility criteria (2) study selection by independent adjudicators using a priori criteria to minimize selection bias; and (3) comprehensive search strategy to minimize publication bias. Data abstraction and critical appraisal were conducted independently and in duplicate from established PRISMA recommendations. We performed a rigorous assessment of study quality using NOS tools for observation cohort/case-control studies. Moreover, we performed rigorous assessment and summation of the level of certainty using GRADE. While mostly observational in nature, the meta-analyzed studies included rely primarily on government databases that cover multiple jurisdictions and millions of people.
This SR has several limitations, the majority of which relate to the outcomes reported in the studies. Consequently, only a subset of the studies with large population-based data was included in the meta-analysis for COVID-19 mortality, all-cause mortality, and non-COVID-19 mortality. Other studies were excluded from our meta-analysis if they had incomplete data, duplicate data from overlapping jurisdictional areas in which we selected the study with the most participants, or if they contained mortality data in formats that could not be meta-analyzed. Our heterogeneity was high, which is unsurprising given the initial spread of COVID-19, testing capabilities, economic disparity, the ranges of policy responses from across the world, and diverse populations which all could have impacted the number of deaths recorded (77). All outcomes’ GRADE certainty of evidence was very low, precluding definitive conclusions from being drawn. All the studies included were observational in nature and GRADE certainty was downgraded due to a high proportion of the studies with high RoB. In addition, the calculation of non-COVID-19 mortality can be skewed by a lack of testing.
The COVID-19 pandemic has resulted in all-cause excess mortality greater than the number that can be accounted for by increases due to mortality from COVID-19 alone. Monitoring all-cause excess mortality may help health policy decision-makers and governments alike understand the true overall impacts of the COVID-19 pandemic on mortality.
The original contributions presented in this study are included in the article/Supplementary material, further inquiries can be directed to the corresponding author.
DL, OR, SB, HS, DZ, MS, KL, HC, SD, PG, BM, AV, BK, DC, AA, DN, KF, and VL have assisted with drafting this manuscript, alongside critical revisions for intellectual content, made substantial contributions to: design and/or development of this SR, data acquisition, meta-analysis and data interpretation, and gave final approval of the published version of this manuscript (Supplementary Appendix 2). All authors contributed to the article and approved the submitted version.
SB was supported by a Canada Research Chair in Critical Care Outcomes and Systems Evaluation.
We are thankful to Diane Keto-Lambert (University of Alberta, Faculty of Medicine and Dentistry) for her expertise during the SR search and PRESS process.
The authors declare that the research was conducted in the absence of any commercial or financial relationships that could be construed as a potential conflict of interest.
All claims expressed in this article are solely those of the authors and do not necessarily represent those of their affiliated organizations, or those of the publisher, the editors and the reviewers. Any product that may be evaluated in this article, or claim that may be made by its manufacturer, is not guaranteed or endorsed by the publisher.
The Supplementary Material for this article can be found online at: https://www.frontiersin.org/articles/10.3389/fmed.2022.999225/full#supplementary-material
1. World Health Organization. Coronavirus Disease 2019 (COVID-19) Situation Report. Geneva: World Health Organization (2021).
2. McElfish PA, Purvis R, James LP, Willis DE, Andersen JA. Perceived barriers to COVID-19 testing. Int J Environ Res Public Health. (2021) 18:2278. doi: 10.3390/ijerph18052278
3. Wang YY, Jin YH, Ren XQ, Li YR, Zhang XC, Zeng XT, et al. Updating the diagnostic criteria of COVID-19 “suspected case” and “confirmed case” is necessary. Mil Med Res. (2020) 7:17. doi: 10.1186/s40779-020-00245-9
4. Kahn M, Schuierer L, Bartenschlager C, Zellmer S, Frey R, Freitag M, et al. Performance of antigen testing for diagnosis of COVID-19: a direct comparison of a lateral flow device to nucleic acid amplification based tests. BMC Infect Dis. (2021) 21:798. doi: 10.1186/s12879-021-06524-7
5. Zhan Z, Li J, Cheng ZJ. Rapid antigen test combine with nucleic acid detection: a better strategy for COVID-19 screening at points of entry. J Epidemiol Glob Health. (2022) 12:13–5. doi: 10.1007/s44197-021-00030-4
6. Chau CH, Strope JD, Figg WD. COVID-19 clinical diagnostics and testing technology. Pharmacother J Hum Pharmacol Drug Ther. (2020) 40:857–68. doi: 10.1002/phar.2439
7. Lau VI, Dhanoa S, Cheema H, Lewis K, Geeraert P, Lu D, et al. Non-COVID outcomes associated with the coronavirus disease-2019 (COVID-19) pandemic effects study (COPES): a systematic review and meta-analysis. PLoS One. (2022) 17:e0269871. doi: 10.1371/journal.pone.0269871
8. Pasquariello P, Stranges S. Excess mortality from COVID-19: a commentary on the Italian experience. Int J Public Health. (2020) 65:529–31. doi: 10.1007/s00038-020-01399-y
9. Banerjee A, Pasea L, Harris S, Gonzalez-Izquierdo A, Torralbo A, Shallcross L, et al. Estimating excess 1-year mortality associated with the COVID-19 pandemic according to underlying conditions and age: a population-based cohort study. Lancet. (2020) 395:1715–25. doi: 10.1016/S0140-6736(20)30854-0
10. Stokes AC, Lundberg DJ, Hempstead K, Elo IT, Preston SH. Assessing the impact of the Covid-19 pandemic on US Mortality: a county-level analysis. medRxiv [Preprint]. (2020) doi: 10.1101/2020.08.31.20184036
11. Kung S, Doppen M, Black M, Braithwaite I, Kearns C, Weatherall M, et al. Underestimation of COVID-19 mortality during the pandemic. ERJ Open Res. (2021) 7:00766–2020. doi: 10.1183/23120541.00766-2020
12. Iuliano AD, Chang HH, Patel NN, Threlkel R, Kniss K, Reich J, et al. Estimating under-recognized COVID-19 deaths, United States, march 2020-may 2021 using an excess mortality modelling approach. Lancet Reg Health Am. (2021) 1:100019. doi: 10.1016/j.lana.2021.100019
13. Kiang MV, Irizarry RA, Buckee CO, Balsari S. Every body counts: measuring mortality from the COVID-19 pandemic. Ann Intern Med. (2020) 173:1004–7. doi: 10.7326/M20-3100
14. Moher D, Liberati A, Tetzlaff J, Altman DG. Preferred reporting items for systematic reviews and meta-analyses: the PRISMA Statement. J Clin Epidemiol. (2009) 62:1006–12. doi: 10.1016/j.jclinepi.2009.06.005
15. McGowan J, Sampson M, Salzwedel DM, Cogo E, Foerster V, Lefebvre C. PRESS peer review of electronic search strategies: 2015 Guideline Statement. J Clin Epidemiol. (2016) 75:40–6. doi: 10.1016/j.jclinepi.2016.01.021
16. World Health Organization. Pneumonia of Unknown Cause – China. Geneva: World Health Organization (2020).
17. Veritas Health Innovation. Covidence Systematic Review Software. London: Veritas Health Innovation (2019).
18. Wells G, Shea B, O’Connell D, Peterson J, Welch V, Losos M, et al. The Newcastle-Ottawa Scale (NOS) for Assessing the Quality of Nonrandomised Studies in Meta-Analyses. Ottawa, ON: Ottawa Hospital Research Institute (2019).
19. Guyatt GH, Oxman AD, Kunz R, Vist GE, Falck-Ytter Y, Schünemann HJ, et al. What is “quality of evidence” and why is it important to clinicians? BMJ. (2008) 336:995–8. doi: 10.1136/bmj.39490.551019.BE
20. Guyatt GH, Oxman AD, Vist GE, Kunz R, Falck-Ytter Y, Alonso-Coello P, et al. GRADE: an emerging consensus on rating quality of evidence and strength of recommendations. BMJ. (2008) 336:924–6. doi: 10.1136/bmj.39489.470347.AD
21. Schünemann H, Brożek J, Guyatt G, Oxman A. GRADE Handbook for Grading Quality of Evidence and Strength of Recommendations. Toronto: GRADEpro (2013).
22. DerSimonian R, Laird N. Meta-analysis in clinical trials. Control Clin Trials. (1986) 7:177–88. doi: 10.1016/0197-2456(86)90046-2
23. Higgins JP, Green S. Cochrane Handbook for Systematic Reviews of Interventions : Cochrane Book Series. London: Cochrane Collaboration (2011).
24. Begg CB, Mazumdar M. Operating characteristics of a rank correlation test for publication bias. Biometrics. (1994) 50:1088–101. doi: 10.2307/2533446
25. Free C, Phillips G, Felix L, Galli L, Patel V, Edwards P. The effectiveness of M-health technologies for improving health and health services: a systematic review protocol. BMC Res Notes. (2010) 3:250. doi: 10.1186/1756-0500-3-250
26. The World Bank. World Bank Country and Lending Groups – World Bank Data Help Desk. Washington, DC: The World Bank (2022).
27. Alicandro G, Remuzzi G, La Vecchia C. COVID-19 pandemic and total mortality in the first six months of 2020 in Italy. Med Lav. (2020) 111:351–3.
28. Bilinski A, Emanuel EJ. COVID-19 and excess all-cause mortality in the US and 18 comparison countries. JAMA. (2020) 324:2100. doi: 10.1001/jama.2020.20717
29. Birkmeyer JD, Barnato A, Birkmeyer N, Bessler R, Skinner J. The impact of the COVID-19 pandemic on hospital admissions in the United States. Health Aff. (2020) 39:2010–7. doi: 10.1377/hlthaff.2020.00980
30. Blangiardo M, Cameletti M, Pirani M, Corsetti G, Battaglini M, Baio G. Estimating weekly excess mortality at sub-national level in Italy during the COVID-19 pandemic. PLoS One. (2020) 15:e0240286. doi: 10.1371/journal.pone.0240286
31. Bustos Sierra N, Bossuyt N, Braeye T, Leroy M, Moyersoen I, Peeters I, et al. All-cause mortality supports the COVID-19 mortality in Belgium and comparison with major fatal events of the last century. Arch Public Health. (2020) 78:117. doi: 10.1186/s13690-020-00496-x
32. Cates J. Risk for in-hospital complications associated with COVID-19 and Influenza — Veterans Health Administration, United States, October 1, 2018–May 31, 2020. MMWR Morb Mortal Wkly Rep. (2020) 69:1528–34. doi: 10.15585/mmwr.mm6942e3
33. Cevallos-Valdiviezo H, Vergara-Montesdeoca A, Zambrano-Zambrano G. Measuring the impact of the COVID-19 outbreak in Ecuador using preliminary estimates of excess mortality, March 17–October 22, 2020. Int J Infect Dis. (2021) 104:297–9. doi: 10.1016/j.ijid.2020.12.045
34. Conti S, Ferrara P, Mazzaglia G, D’Orso MI, Ciampichini R, Fornari C, et al. Magnitude and time-course of excess mortality during COVID-19 outbreak: population-based empirical evidence from highly impacted provinces in northern Italy. ERJ Open Res. (2020) 6:00458–2020. doi: 10.1183/23120541.00458-2020
35. Cusack DA. COVID-19 pandemic: coroner’s database of death inquiries with clinical epidemiology and total and excess mortality analyses in the District of Kildare March to June 2020. J Forensic Leg Med. (2020) 76:102072. doi: 10.1016/j.jflm.2020.102072
36. Friedman J, Calderón-Villarreal A, Bojorquez I, Vera Hernández C, Schriger DL, Tovar Hirashima E. Excess out-of-hospital mortality and declining oxygen saturation: the sentinel role of emergency medical Services data in the COVID-19 Crisis in Tijuana, Mexico. Ann Emerg Med. (2020) 76:413–26. doi: 10.1016/j.annemergmed.2020.07.035
37. Habonimana D, Ouedraogo L, Ndirahisha E, Misago N, Ciza R, Niyomwungere D, et al. Understanding the influence of the COVID-19 pandemic on hospital-based mortality in Burundi: a cross-sectional study comparing two time periods. Epidemiol Infect. (2020) 148:e280. doi: 10.1017/S0950268820002770
38. Jacobson SH, Jokela JA. Non–COVID-19 excess deaths by age and gender in the United States during the first three months of the COVID-19 pandemic. Public Health. (2020) 189:101–3. doi: 10.1016/j.puhe.2020.10.004
39. Lerner EB, Newgard CD, Mann NC. Effect of the coronavirus disease 2019 (COVID-19) Pandemic on the U.S. emergency medical services system: a preliminary report. Acad Emerg Med. (2020) 27:693–9. doi: 10.1111/acem.14051
40. Little CD, Kotecha T, Candilio L, Jabbour RJ, Collins GB, Ahmed A, et al. COVID-19 pandemic and STEMI: pathway activation and outcomes from the pan-London heart attack group. Open Heart. (2020) 7:e001432. doi: 10.1136/openhrt-2020-001432
41. Mannucci E, Nreu B, Monami M. Factors associated with increased all-cause mortality during the COVID-19 pandemic in Italy. Int J Infect Dis. (2020) 98:121–4. doi: 10.1016/j.ijid.2020.06.077
42. McGuinness G, Zhan C, Rosenberg N, Azour L, Wickstrom M, Mason DM, et al. Increased incidence of barotrauma in patients with COVID-19 on invasive mechanical ventilation. Radiology. (2020) 297:E252–62. doi: 10.1148/radiol.2020202352
43. Michelozzi P, de’Donato F, Scortichini M, Pezzotti P, Stafoggia M, De Sario M, et al. Temporal dynamics in total excess mortality and COVID-19 deaths in Italian cities. BMC Public Health. (2020) 20:1238. doi: 10.1186/s12889-020-09335-8
44. Miles A, Webb TE, Mcloughlin BC, Mannan I, Rather A, Knopp P, et al. Outcomes from COVID-19 across the range of frailty: excess mortality in fitter older people. Eur Geriatr Med. (2020) 11:851–5. doi: 10.1007/s41999-020-00354-7
45. Nef HM, Elsässer A, Möllmann H, Abdel-Hadi M, Bauer T, Brück M, et al. Impact of the COVID-19 pandemic on cardiovascular mortality and catherization activity during the lockdown in central Germany: an observational study. Clin Res Cardiol. (2021) 110:292–301. doi: 10.1007/s00392-020-01780-0
46. Orellana JDY, da Cunha GM, Marrero L, Horta BL, da Leite IC. Explosion in mortality in the Amazonian epicenter of the COVID-19 epidemic 19. Cad Saude Publica. (2020) 36:e00120020. doi: 10.1590/0102-311x00120020
47. Perkin MR, Heap S, Crerar-Gilbert A, Albuquerque W, Haywood S, Avila Z, et al. Deaths in people from Black, Asian and minority ethnic communities from both COVID-19 and non-COVID causes in the first weeks of the pandemic in London: a hospital case note review. BMJ Open. (2020) 10:e040638. doi: 10.1136/bmjopen-2020-040638
48. Piccininni M, Rohmann JL, Foresti L, Lurani C, Kurth T. Use of all cause mortality to quantify the consequences of covid-19 in Nembro, Lombardy: descriptive study. BMJ. (2020) 369:m1835. doi: 10.1136/bmj.m1835
49. Richards-Belle A, Orzechowska I, Gould DW, Thomas K, Doidge JC, Mouncey PR, et al. COVID-19 in critical care: epidemiology of the first epidemic wave across England, Wales and Northern Ireland. Intensive Care Med. (2020) 46:2035–47. doi: 10.1007/s00134-020-06267-0
50. Riley B, Packer M, Gallier S, Sapey E, Atkin C. Acute, non-COVID related medical admissions during the first wave of COVID-19: a retrospective comparison of changing patterns of disease. Acute Med. (2020) 19:176–82. doi: 10.52964/AMJA.0825
51. Rossen LM. Excess deaths associated with COVID-19, by age and race and ethnicity — United States, January 26–October 3, 2020. MMWR Morb Mortal Wkly Rep. (2020) 69:1522–7. doi: 10.15585/mmwr.mm6942e2
52. Saglietto A, D’ascenzo F, Cavarretta E, Frati G, Anselmino M, Versaci F, et al. Excess all-cause mortality during COVID-19 outbreak: potential role of untreated cardiovascular disease. Minerva Cardioangiol. (2022) 70:160–6. doi: 10.23736/S2724-5683.20.05349-9
53. Strang P, Fürst P, Schultz T. Excess deaths from COVID-19 correlate with age and socio-economic status. a database study in the Stockholm region. Ups J Med Sci. (2020) 125:297–304. doi: 10.1080/03009734.2020.1828513
54. Vestergaard LS, Nielsen J, Richter L, Schmid D, Bustos N, Braeye T, et al. Excess all-cause mortality during the COVID-19 pandemic in Europe – preliminary pooled estimates from the EuroMOMO network, March to April 2020. Eurosurveillance. (2020) 25:2001214. doi: 10.2807/1560-7917.ES.2020.25.26.2001214
55. Vieira A, Peixoto VR, Aguiar P, Abrantes A. Rapid estimation of excess mortality during the COVID-19 pandemic in portugal -beyond reported deaths. J Epidemiol Glob Health. (2020) 10:209–13. doi: 10.2991/jegh.k.200628.001
56. Woolf SH, Chapman DA, Sabo RT, Weinberger DM, Hill L. Excess deaths from COVID-19 and other causes, March-April 2020. JAMA. (2020) 324:510. doi: 10.1001/jama.2020.11787
57. Ioannidis JPA. Infection fatality rate of COVID-19 inferred from seroprevalence data. Bull World Health Organ. (2021) 99:19F–33F. doi: 10.2471/BLT.20.265892
58. Ghisolfi S, Almås I, Sandefur JC, von Carnap T, Heitner J, Bold T. Predicted COVID-19 fatality rates based on age, sex, comorbidities and health system capacity. BMJ Glob Health. (2020) 5:e003094. doi: 10.1136/bmjgh-2020-003094
59. Atalan A. Is the lockdown important to prevent the COVID-19 pandemic? Effects on psychology, environment and economy-perspective. Ann Med Surg. (2020) 56:38–42. doi: 10.1016/j.amsu.2020.06.010
60. Burki T. Global COVID-19 vaccine inequity. Lancet Infect Dis. (2021) 21:922–3. doi: 10.1016/S1473-3099(21)00344-3
61. Yang S, Cao P, Du P, Wu Z, Zhuang Z, Yang L, et al. Early estimation of the case fatality rate of COVID-19 in mainland China: a data-driven analysis. Ann Transl Med. (2020) 8:128–128. doi: 10.21037/atm.2020.02.66
62. Karlinsky A, Kobak D. The world mortality dataset: tracking excess mortality across countries during the COVID-19 pandemic. medRxiv [Preprint]. (2021) doi: 10.1101/2021.01.27.21250604
63. Jha P, Deshmukh Y, Tumbe C, Suraweera W, Bhowmick A, Sharma S, et al. COVID mortality in India: National survey data and health facility deaths. Science. (2022) 375:667–71. doi: 10.1126/science.abm5154
64. Adam D. The pandemic’s true death toll: millions more than official counts. Nature. (2022) 601:312–5. doi: 10.1038/d41586-022-00104-8
65. Lau H, Khosrawipour T, Kocbach P, Ichii H, Bania J, Khosrawipour V. Evaluating the massive underreporting and undertesting of COVID-19 cases in multiple global epicenters. Pulmonology. (2021) 27:110–5. doi: 10.1016/j.pulmoe.2020.05.015
66. Whittaker C, Walker PGT, Alhaffar M, Hamlet A, Djaafara BA, Ghani A, et al. Under-reporting of deaths limits our understanding of true burden of covid-19. BMJ. (2021) 375:n2239. doi: 10.1136/bmj.n2239
67. Rahmandad H, Lim TY, Sterman J. Estimating COVID-19 under-reporting across 86 nations: implications for projections and control. medRxiv [Preprint]. (2020) doi: 10.1101/2020.06.24.20139451
68. Muscatello DJ, Cretikos MA, MacIntyre CR. All-cause mortality during first wave of pandemic (H1N1) 2009, New South Wales, Australia, 2009. Emerg Infect Dis. (2010) 16:1396–402. doi: 10.3201/eid1609.091723
69. Wang H, Paulson KR, Pease SA, Watson S, Comfort H, Zheng P, et al. Estimating excess mortality due to the COVID-19 pandemic: a systematic analysis of COVID-19-related mortality, 2020–21. Lancet. (2022) 399:1513–36.
70. Patterson GE, McIntyre KM, Clough HE, Rushton J. Societal impacts of pandemics: comparing COVID-19 with history to focus our response. Front Public Health. (2021) 9:630449. doi: 10.3389/fpubh.2021.630449
71. TIME. The White House Wants to Achieve Herd Immunity By Letting the Virus Rip. That is Dangerous and Inhumane. Secunderabad: TIME (2022).
72. Blumenthal D, Fowler EJ, Abrams M, Collins SR. Covid-19 — Implications for the Health Care System. N Engl J Med. (2020) 383:1483–8. doi: 10.1056/NEJMsb2021088
73. Moynihan R, Sanders S, Michaleff ZA, Scott AM, Clark J, To EJ, et al. Impact of COVID-19 pandemic on utilisation of healthcare services: a systematic review. BMJ Open. (2021) 11:e045343. doi: 10.1136/bmjopen-2020-045343
74. Sasangohar F, Jones SL, Masud FN, Vahidy FS, Kash BA. Provider burnout and fatigue during the COVID-19 Pandemic: lessons learned from a high-volume intensive care unit. Anesth Analg. (2020) 131:106–11. doi: 10.1213/ANE.0000000000004866
75. Sheehan O, Sheehan M, Rau RI, Sullivan IO, McMahon G, Payne A. Burnout on the frontline: the impact of COVID-19 on emergency department staff wellbeing. Ir J Med Sci. (2022) 191:2325–33. doi: 10.1007/s11845-021-02795-w
76. Wu H, Soe MM, Konnor R, Dantes R, Haass K, Dudeck MA, et al. Hospital capacities and shortages of healthcare resources among US hospitals during the coronavirus disease 2019 (COVID-19) pandemic, National Healthcare Safety Network (NHSN), March 27–July 14, 2020. Infect Control Hosp Epidemiol. (2022) 43:1473–6. doi: 10.1017/ice.2021.280
Keywords: excess mortality, COVID-19, non-COVID-19 mortality, pandemic (COVID-19), outcomes
Citation: Lu D, Dhanoa S, Cheema H, Lewis K, Geeraert P, Merrick B, Vander Leek A, Sebastianski M, Kula B, Chaudhuri D, Basmaji J, Agrawal A, Niven D, Fiest K, Stelfox HT, Zuege DJ, Rewa OG, Bagshaw SM and Lau VI (2022) Coronavirus disease 2019 (COVID-19) excess mortality outcomes associated with pandemic effects study (COPES): A systematic review and meta-analysis. Front. Med. 9:999225. doi: 10.3389/fmed.2022.999225
Received: 20 July 2022; Accepted: 02 December 2022;
Published: 16 December 2022.
Edited by:
Rebecca De Lorenzo, Vita-Salute San Raffaele University, ItalyReviewed by:
Gloria Vazquez Grande, University of Manitoba, CanadaCopyright © 2022 Lu, Dhanoa, Cheema, Lewis, Geeraert, Merrick, Vander Leek, Sebastianski, Kula, Chaudhuri, Basmaji, Agrawal, Niven, Fiest, Stelfox, Zuege, Rewa, Bagshaw and Lau. This is an open-access article distributed under the terms of the Creative Commons Attribution License (CC BY). The use, distribution or reproduction in other forums is permitted, provided the original author(s) and the copyright owner(s) are credited and that the original publication in this journal is cited, in accordance with accepted academic practice. No use, distribution or reproduction is permitted which does not comply with these terms.
*Correspondence: Vincent I. Lau, ✉ dmluY2UubGF1QHVhbGJlcnRhLmNh
Disclaimer: All claims expressed in this article are solely those of the authors and do not necessarily represent those of their affiliated organizations, or those of the publisher, the editors and the reviewers. Any product that may be evaluated in this article or claim that may be made by its manufacturer is not guaranteed or endorsed by the publisher.
Research integrity at Frontiers
Learn more about the work of our research integrity team to safeguard the quality of each article we publish.