- 1Laboratory of Tumor Immunology and Immunotherapy, Department of Oncology, Leuven Cancer Institute, KU Leuven, Leuven, Belgium
- 2Oncoinvent AS, Oslo, Norway
- 3Department of Neurosurgery, Mont-Godinne Hospital, UCL Namur, Yvoir, Belgium
- 4Division of Gynecological Oncology, Department of Obstetrics and Gynecology, Leuven Cancer Institute, University Hospitals Leuven, Leuven, Belgium
- 5Department of Oncology, Gynecological Oncology, KU Leuven, Leuven, Belgium
A novel alpha-therapy consisting of 224Ra-labeled calcium carbonate microparticles (224Ra-CaCO3-MP) has been designed to treat micrometastatic peritoneal disease via intraperitoneal (IP) administration. This preclinical study aimed to evaluate its efficacy and tolerability when given as a single treatment or in combination with standard of care chemotherapy regimens, in a syngeneic model of ovarian cancer in immune competent mice. Female C57BL/6 mice bearing ID8-fLuc ovarian cancer were treated with 224Ra-CaCO3-MP 1 day after IP tumor cell inoculation. The activity dosages of 224Ra ranged from 14 to 39 kBq/mouse. Additionally, 224Ra-CaCO3-MP treatment was followed by either carboplatin (80 mg/kg)-pegylated liposomal doxorubicin (PLD, 1.6 mg/kg) or carboplatin (60 mg/kg)-paclitaxel (10 mg/kg) on day 14 post tumor cell inoculation. All treatments were administered via IP injections. Readouts included survival, clinical signs, and body weight development over time. There was a slight therapeutic benefit after single treatment with 224Ra-CaCO3-MP compared to the vehicle control, with median survival ratios (MSRs) ranging between 1.1 and 1.3. The sequential administration of 224Ra-CaCO3-MP with either carboplatin-paclitaxel or carboplatin-PLD indicated a synergistic effect on overall survival at certain 224Ra activities. Moreover, the combinations tested appeared well tolerated in terms of weight assessment in the first 4 weeks after treatment. Overall, this research supports the further evaluation of 224Ra-CaCO3-MP in patients with ovarian cancer. However, the most optimal chemotherapy regimen to combine with 224Ra-CaCO3-MP should be identified to fully exploit its therapeutic potential.
Introduction
Ovarian cancer is the eight leading cause of cancer related deaths within the female population worldwide (1, 2). The most dominant subtype is high-grade serous ovarian cancer (HGSOC), which has an epithelial origin (3, 4). Due to the absence of symptoms at earlier stages of the disease, patients are often diagnosed at an advanced disease stage (International Federation of Gynecology and Obstetrics (FIGO) stage III and IV). In first line, the current standard of care of advanced ovarian cancer consists of a cytoreductive debulking surgery combined with platinum-based chemotherapy and eventually bevacizumab and/or poly (ADP-ribose) polymerase (PARP) inhibitors (5). The carboplatin-paclitaxel chemotherapy combination is the preferred regimen in a first-line treatment setting. However, the choice of chemotherapy at recurrence depends on tumor characteristics and whether platinum is again an option or not. At the time of recurrence, both platinum and non-platinum agents such as paclitaxel, gemcitabine, pegylated liposomal doxorubicin (PLD) and topotecan, as well as targeted therapies such as PARP inhibitors and bevacizumab can be used as single agents or in combination schedules (6). Nevertheless, HGSOC patients who get diagnosed in an advanced disease stage have a poor 5 year survival of only 20%-41% (7).
In most patients, recurrence of the disease involves the presence of metastases confined to the peritoneal cavity. In the ongoing search for more effective treatment strategies to locally target this peritoneal disease, the rapidly evolving research field of radionuclide therapy is of interest. Historically, the main focus in the context of ovarian cancer was on the investigation of β-particle emitters, with various success rates. In the past, radiocolloids containing 32P or 198Au have been used for IP treatment of patients with ovarian cancer (8–11). However, its use was limited in time due to the increased incidence of adverse effects, and replacement with chemotherapy treatment was recommended (9). Additionally, antibody guided 90Y has been explored in the context of ovarian cancer, but it did not proceed from phase III clinical trials (12, 13). Alternative types of radiotherapy options that have been explored for ovarian cancer include proton beam therapy and carbon ion therapy with successful outcomes in two case reports of patients with recurrent ovarian cancer (14, 15).
The use of α-particle emitters is assumed to have advantages over the prior β-therapies. They are particularly of interest for the treatment of micrometastatic cancer dissemination in body cavities, one of the characteristics of peritoneal carcinomatosis in patients with ovarian cancer (16). Alpha-emitters are highly cytotoxic for the cancer cells residing in the abdominal cavity, while sparing surrounding radiosensitive organs because of their short penetration depth, thus limiting toxicities compared to β-emitters. To date, Xofigo® (223RaCl2) remains the only α-emitting radiopharmaceutical approved by the European Medicines Agency and the Food and Drug Administration, and is currently used for the treatment of skeletal metastases of castration-resistant prostate cancer (17). However, α-emitters, such as 212Pb and 211At have been investigated for ovarian cancer in phase I clinical trials, where feasibility of this type of treatment was confirmed without apparent signs of dose-limiting toxicities (18, 19).
The α-emitter 224Ra, when adsorbed onto the microparticle drug carrier CaCO3, has shown therapeutic potential in immunodeficient ovarian cancer xenograft mouse models, when administered as an IP treatment (20, 21). Based on these promising data, the 224Ra-CaCO3-MP are currently being assessed in phase I clinical trials for both ovarian cancer (22) and colorectal carcinoma (23), two cancer types characterized by the presence of a widespread metastatic disease within the peritoneal cavity.
The current paper focusses on the evaluation of 224Ra-CaCO3-MP in terms of its potential to treat peritoneally disseminated ovarian cancer in an immune competent mouse model. The aim was to examine the tolerability and efficacy of combinations with chemotherapy regimens commonly used in patients with ovarian cancer.
Materials and methods
Ovarian cancer tumor model
The ID8-fLuc cell line was transduced with a lentiviral vector (pCHMWS_CMV-fluc-I-PuroR) by the Laboratory of Molecular Virology and Gene Therapy and Leuven Viral Vector Core in our institute (KU Leuven, Belgium) (24). Female C57BL/6 mice (Envigo, Horst, The Netherlands) of seven to 9 weeks of age were inoculated IP with 5 x 106 ID8-fLuc ovarian cancer cells on day 0 of the experiment, in the lower right quadrant of the abdomen. All animal experiments were approved by the ethical committee of the KU Leuven (P123/2017) and followed the most recent ethical standards (NIH guidelines for the Care and Use of Laboratory Animals and EU Directive 2010/63/EU as amended by Regulation (EU) 2019/1010) and the ARRIVE (Animal Research: Reporting of In Vivo Experiments) guidelines (25, 26). All mice in experiment were monitored at least three times per week in terms of body weight and clinical signs of disease, and drained from ascites when mice reached 32 grams. When pre-defined humane endpoints were reached [previously published (24)], mice were euthanized by cervical dislocation.
224Ra-CaCO3-MP preparation and treatment in mice
Two product formulations of 224Ra-CaCO3-MP have been used: 224Ra-CaCO3-MP-1 from the early-phase of development and the optimized 224Ra-CaCO3-MP-2, developed for clinical use in humans. The preparation of the 224Ra-CaCO3-MP-1 product formulation is described as second generation microparticles (27), whereas the preparation of the 224Ra-CaCO3-MP-2 formulation can be found in a separate publication where they are described as layer-encapsulated microparticles (28). In brief, CaCO3 microparticles were prepared by a spontaneous precipitation method which yielded particles with a mainly spherical geometry with a volume-based median diameter of approximately 6 μm when measured by laser diffraction (Mastersizer 3000, Malvern Instruments Ltd, Worcestershire, UK). For radiolabeling, 224RaCl2 solution was added to a suspension of CaCO3 microparticles in the presence of Ba2+ and SO (0.004% and 0.6% (w/w) relative to CaCO3 respectively) for the coprecipitation of 224Ra. After the radiolabeling process, the 224Ra-CaCO3-MP-1 were dispersed to a concentration of approximately 12.5 mg/ml in 0.9% NaCl. To fulfill the requirements for the clinical use of the radiopharmaceutical, it was necessary to control the size of microparticles in suspension over time and introduce a sterilization process. Hence, for the 224Ra-CaCO3-MP-2, an additional layer of CaCO3 was precipitated on the microparticles before they were dispersed to 25 mg/ml in 0.9% NaCl and 2.4% (w/w) EDTMPA [ethylenediamine tetra(methylenephosphonic acid)] and sterilized in an autoclave at 121 °C for 20 min. The 224Ra-CaCO3-MP-2 product was diluted to a final concentration of 12.5 mg/ml with Plasmalyte (Baxter, Deerfield, IL, USA) prior to treatment administration in mice. Radium-224 labeled microparticles were administered via an IP injection at a volume of 0.4 ml on day 1 post tumor cell inoculation at a mass dose of 5 mg CaCO3 and an activity dose ranging between 14 and 39 kBq/mouse 224Ra (805 and 2118 kBq/kg, respectively).
Chemotherapy preparation and treatment in mice
Carboplatin and paclitaxel (Hospira, ONCO-TAIN, Pfizer, New York, NY, USA) were dissolved in Dulbecco's phosphate-buffered saline (DPBS, Thermo Fisher Scientific, Waltham, MA, USA) and administered IP at a dose of 60 or 80 mg/kg and 10 mg/kg, respectively, calculated for an average body weight of 20 g per mouse. Pegylated liposomal doxorubicin (Caelyx/Doxil®, Janssens Cilag International NV, Beerse, Belgium) was administered IP at a dose of 1.6 mg/kg. All chemotherapy doses used in this manuscript were determined previously via in vivo dosage experiments for each of the combination schedules in the ID8-fLuc mouse model for ovarian cancer (unpublished results).
Experimental design
All 224Ra-CaCO3-MP treatments were administered on day 1 post tumor cell inoculation (Figures 1A, 2A, 3A). This time point was chosen to mimic minimal residual disease after a cytoreductive debulking surgery in patients, a situation highly relevant to target micro-metastatic disseminations in the peritoneal cavity. Chemotherapy administration in all experiments was performed at day 14 post tumor cell inoculation (Figures 2A, 3A), to mimic the adjuvant chemotherapy initiation in patients. All treatments were administered via IP injections. For all injection time points, control mice received either DPBS, 0.9% NaCl or Plasmalyte without additives as the appropriate vehicle control solution for their respective experimental treatment.
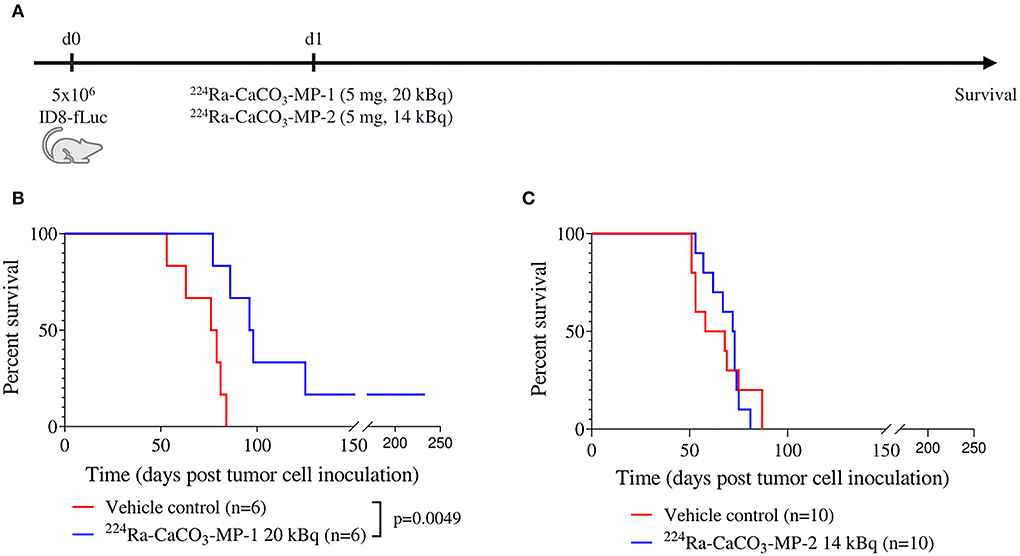
Figure 1. Experimental set-up (A) with corresponding Kaplan-Meier curves (B,C) for survival of mice injected IP with vehicle control and 224Ra-CaCO3-MP-1 (5 mg, 20 kBq) or 224Ra-CaCO3-MP-2 (5 mg, 14 kBq) on day 1 post tumor cell inoculation.
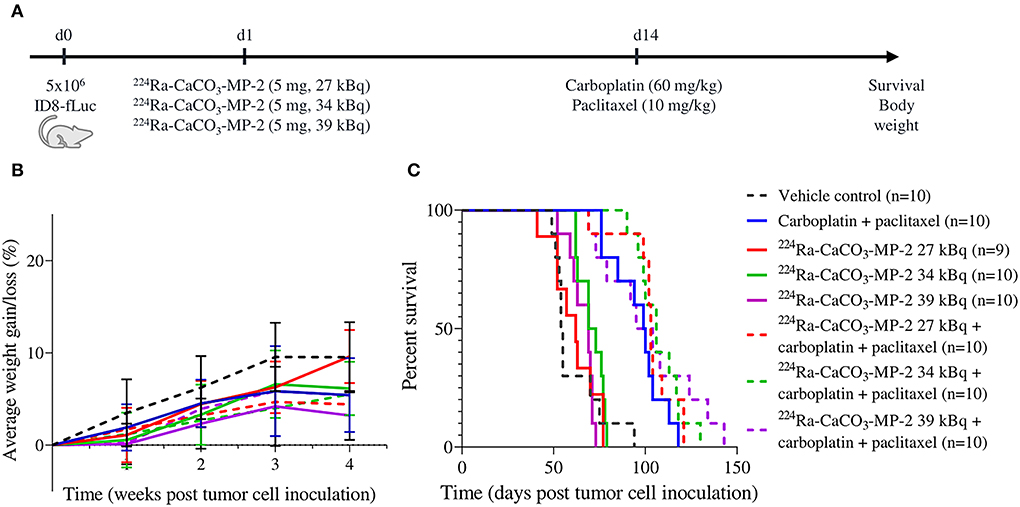
Figure 2. Experimental set-up (A) with corresponding body weight evolution as a percentage of starting weight (B) and Kaplan-Meier curve for survival (C) of mice injected IP with vehicle control, 224Ra-CaCO3-MP-2 (5 mg, 27/34/39 kBq) on day 1 post tumor cell inoculation and/or carboplatin (60 mg/kg) and paclitaxel (10 mg/kg) on day 14 post tumor cell inoculation. Due to procedural complications during tumor cell inoculation (injection in visceral peritoneum instead of peritoneal cavity), one animal allocated to the 224Ra-CaCO3-MP-2 27 kBq single treatment group was excluded from all further data analysis.
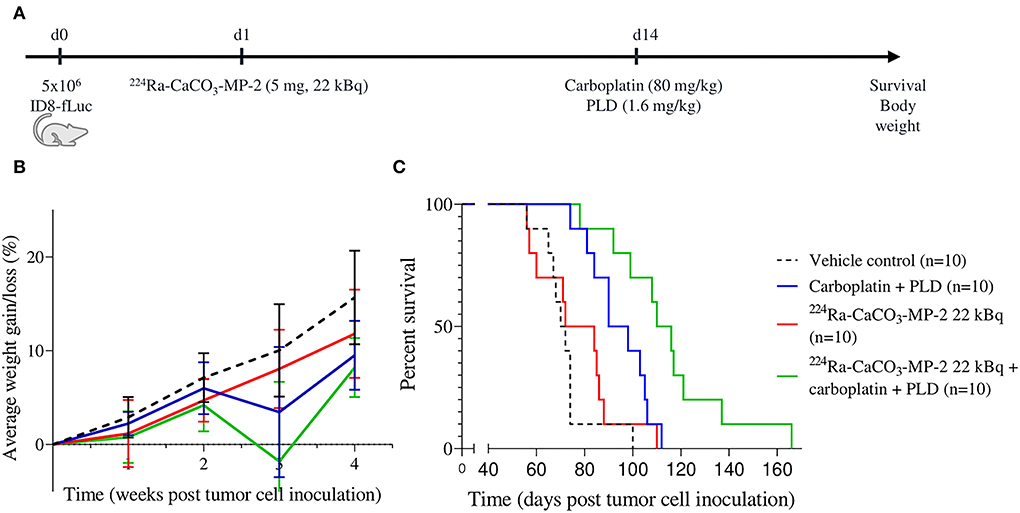
Figure 3. Experimental set-up (A) with corresponding body weight evolution as a percentage of starting weight (B) and Kaplan-Meier curve for survival (C) of mice injected IP with vehicle control, 224Ra-CaCO3-MP-2 (5 mg, 22 kBq) on day 1 post tumor cell inoculation and/or carboplatin (80 mg/kg) and PLD (1.6 mg/kg) on day 14 post tumor cell inoculation.
Data analysis
A statistical power analysis was performed to determine sample sizes for all experiments. A power of at least 0.80 was reached with 6 to 10 mice per treatment group, depending on the type of experiment. Survival curves were compared using the log-rank (Mantel-Cox) test. Adjustment for multiple comparisons was performed with the Benjamini-Hochberg procedure (with Q = 5%). The comparisons made for the different experiments can be found in Supplementary Tables S1, S2. Median survival ratios (MSR) were calculated as the median survival of the experimental group divided by the median survival of the respective control group and served as an additional measure for efficacy. A linear mixed model was fitted to assess the effects of the 224Ra-CaCO3-MP on the weight changes of the mice in the first 4 weeks after treatment administration, with data points taken at 1 week intervals. An (adjusted) p < 0.05 was considered significant. Synergy between chemotherapy treatment and 224Ra-CaCO3-MP was assessed using the Bliss analysis method (29). For this, a Cox proportional-hazards model was fitted to the survival data. Synergy is evaluated based on the hazard ratios (HRs) of the interaction of groups treated with both chemotherapy and 224Ra-CaCO3-MP and the monotherapy treatment groups. Interaction values lower than 1 are considered synergistic, with statistical significance defined by a p < 0.05 and by the confidence interval not including 1.
The HR in case of synergy (HRcombination) is calculated by multiplying the HRs of both single treatment and the HR of the interaction, and the HR in case of an additive effect (HRadditive) is calculated by multiplying only the HRs of the single treatments.
The power analysis, Bliss analysis and linear mixed model fitting were performed using R version 4.1.0 (R Foundation for Statistical Computing, Vienna, Austria, https://www.R-project.org/), all other statistical analyses were performed using GraphPad Prism version 8.2.1 (GraphPad Software, San Diego, CA, USA).
Results
Therapeutic potential of 224Ra-CaCO3-MP as an IP treatment in the immune competent ID8-fLuc mouse model for ovarian cancer
Two product formulations of 224Ra-CaCO3-MP were evaluated in the immune competent ID8-fLuc mouse model for ovarian cancer. Treatment with the early-phase development product formulation 224Ra-CaCO3-MP-1 was able to significantly prolong survival compared to vehicle control mice (median survival of 97 and 77.5 days, respectively, p = 0.0049) and cured 17% of the mice at an activity dose of 20 kBq/mouse with an average of 1,004 kBq/kg body weight (Figure 1B). In a follow-up experiment with the 224Ra-CaCO3-MP-2 formulation developed for clinical use, where control of microparticle size over time was achieved and a sterilization procedure was introduced [20], there was no effect on overall survival at an activity dose of 14 kBq/mouse with an average of 805 kBq/kg body weight (Figure 1C). Even though the activity dose in this experiment was lower (14 compared to 20 kBq/mouse), higher activity doses that were used in the combination studies discussed below (ranging between 22 and 39 kBq/mouse) had a similar outcome (Figures 2C, 3C). The MSRs of all single treatments with 224Ra-CaCO3-MPs, were higher than 1 in all investigated conditions (Table 1).
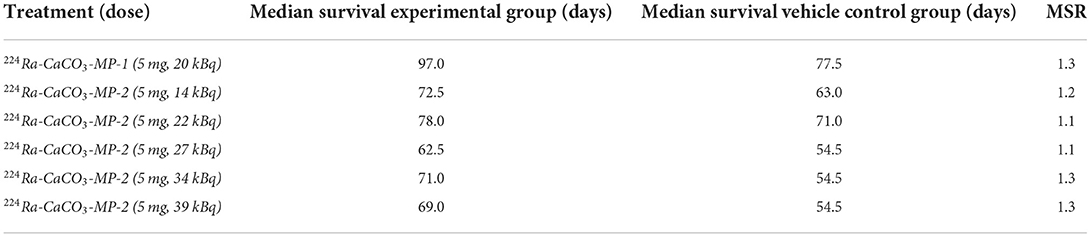
Table 1. Median survival ratios (MSRs) for 224Ra-CaCO3-MP as a single treatment compared to vehicle control.
Therapeutic synergistic effect of 224Ra-CaCO3-MP combined with chemotherapy
224Ra-CaCO3-MPs were combined with two different chemotherapy regimens commonly used in clinical practice. In these studies, our first readout included therapeutic efficacy in terms of survival. In a first experiment we combined the first-line chemotherapy regimen carboplatin-paclitaxel with different activity doses of 224Ra-CaCO3-MP: 27, 34 and 39 kBq/mouse with an average of 1,466, 1,847 and 2,118 kBq/kg body weight. None of the activity dose levels of 224Ra-CaCO3-MP-2 in combination with carboplatin-paclitaxel were able to significantly improve survival compared to mice treated with carboplatin-paclitaxel alone (Figure 2C). No statistically significant synergistic effects were observed for any of the activity doses and the carboplatin-paclitaxel chemotherapy regimen. However, there is a tendency toward synergism for the highest activity dose level of 224Ra-CaCO3-MP-2 (39 kBq) when comparing the HRcombination (0.0667) with the HRadditive (0.1206). An overview of all HRs can be found in Table 2.
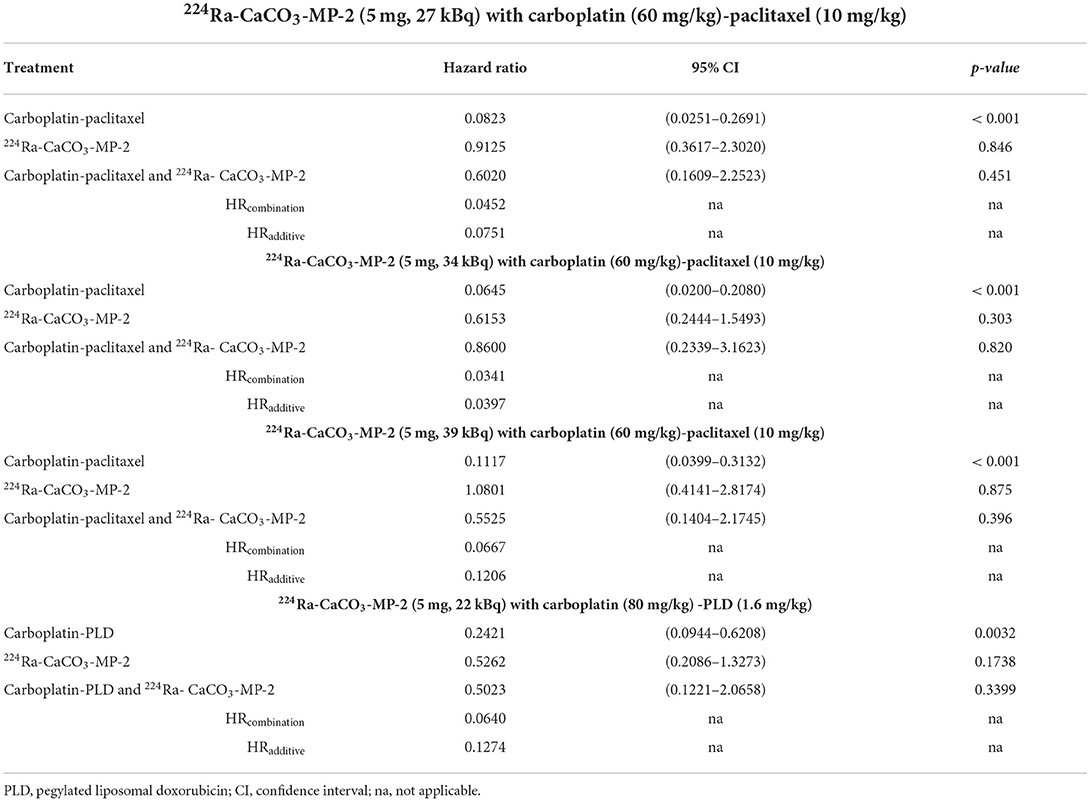
Table 2. Assessment of synergistic effect between 224Ra-CaCO3-MP-2 and carboplatin-paclitaxel or carboplatin-PLD.
Subsequently, we assessed a combination with carboplatin-PLD as an example of a second-line chemotherapy regimen. Only one activity dose of 224Ra-CaCO3-MP was included: 22 kBq/mouse with an average of 1,300 kBq/kg body weight. While 224Ra-CaCO3-MP-2 as a single treatment was not able to prolong survival, the combination of 224Ra-CaCO3-MP-2 combined with the carboplatin-PLD resulted in a prolonged survival compared to mice that received chemotherapy alone (median survival of 114 and 94.5 days, respectively, padj = 0.0102) (Figure 3C). However, the biologically observed synergistic effect between carboplatin-PLD and 224Ra-CaCO3-MP-2 treatment is not supported by a statistically significant effect (p = 0.3399), although there is a tendency toward synergism when comparing the HRcombination (0.0640) and the HRadditive (0.1274). An overview of the different HRs can be found in Table 2.
The MSRs were also determined for the combinations of 224Ra-CaCO3-MP-2 with the two different chemotherapy regimens, when compared to mice treated with chemotherapy alone. All MSRs ranged between 1 and 1.2 (Table 3).
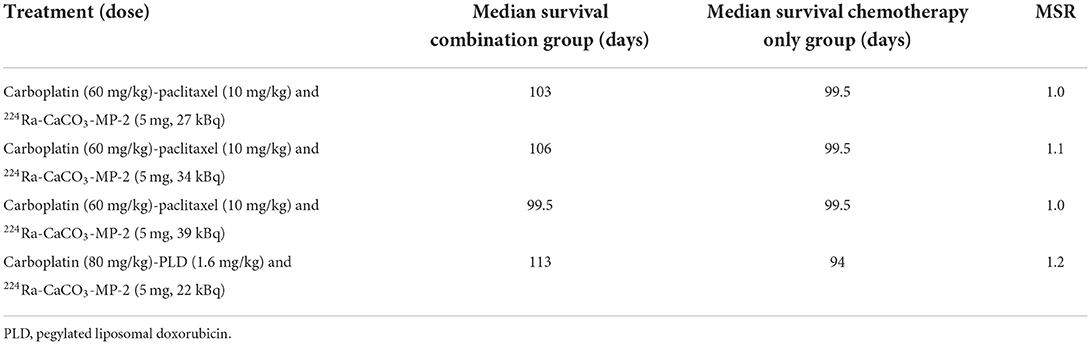
Table 3. Median survival ratios (MSRs) for 224Ra-CaCO3-MP combined with chemotherapy compared to chemotherapy as a single treatment.
Combination of 224Ra-CaCO3-MPs with standard of care chemotherapy regimens is feasible in terms of tolerability
Changes in body weight over time was assessed as a measure of tolerability. From the body weight curves (Supplementary Figures S1, S2), there were no indications of persistent treatment related effects. A transient loss in body weight was observed in the days following both treatment with 224Ra-CaCO3-MP-2 and chemotherapy, with longest time to recovery (approximately 1 week) for the carboplatin and PLD regime. When body weight development over time was analyzed by fitting a linear mixed model, a statistically significant delay in body weight gain was found for the mice treated with the highest activity doses of 34 and 39 kBq/mouse compared to vehicle control mice (p = 0.047 and p < 0.001, respectively). However, 224Ra-CaCO3-MP-2 treatment was not inferior to chemotherapy treatment in terms of body weight development over time. If anything, treatment with carboplatin-paclitaxel resulted in a delayed weight progression compared to mice that received 224Ra-CaCO3-MP-2 at a dose of 27 kBq/mouse (p=0.045). More importantly, none of the groups receiving a combination of chemotherapy with the 224Ra-CaCO3-MP-2 treatment presented with delayed weight progression compared to mice that received chemotherapy alone, irrespective of the chemotherapy regimen (Figures 2B, 3B, Table 4). In addition, no apparent clinical signs of toxicity were observed in any of the mice in the duration of the studies.
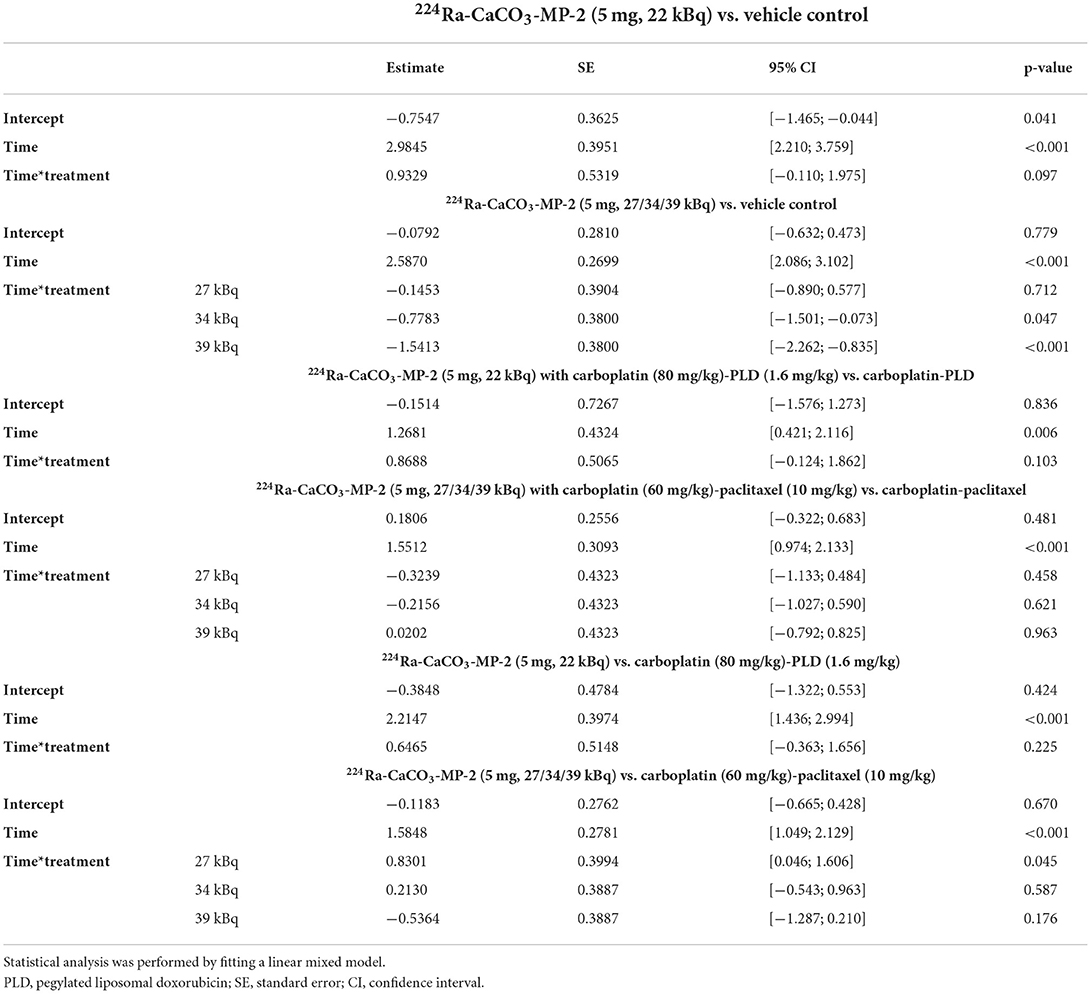
Table 4. Overview of weight change assessment in mice that received 224Ra-CaCO3-MPs as a single treatment and in combination with both carboplatin-paclitaxel and carboplatin-PLD chemotherapy regimens.
Discussion
In the search for more effective treatment strategies for ovarian cancer, α-emitting radionuclide therapies are emerging. The high energy deposition in combination with limited penetration depth can be exploited to target residual microscopic disease without affecting the surrounding radiosensitive organs. These micrometastases remain present within the peritoneal cavity after cytoreductive debulking surgery and are often related to the high recurrence rate of the disease. In this study, we specifically investigated the therapeutic potential of a newly developed α-emitting radiopharmaceutical which consists of 224Ra adsorbed onto CaCO3 microparticles, and the safety to use this in combination with chemotherapy regimens commonly used in clinical practice. We provide proof-of-principle of the therapeutic potential of 224Ra-CaCO3-MPs in a syngeneic model of ovarian cancer in immune competent mice. Furthermore, the sequential administration of 224Ra-CaCO3-MPs with two different standard of care chemotherapy regimens indicated that a synergistic effect can be obtained, however, the synergism was more pronounced with carboplatin-PLD compared to carboplatin-paclitaxel. In general, the various treatment combinations appeared well-tolerated in the mice.
The therapeutic potential of 224Ra-CaCO3-MPs in the immune compromised ES-2 and SKOV3 mouse model for ovarian cancer have previously been demonstrated. Different product formulations of 224Ra-CaCO3-MPs were able to prolong survival with MSRs ranging between 1.5 and 2.8 (20, 21, 28), while the MSRs observed in the current study ranged between 1.1 and 1.3. An important factor that might negatively influence the therapeutic efficacy in the immune competent ID8-fLuc mouse model for ovarian cancer is the reaction of the tumor immune microenvironment to the particle drug carrier (CaCO3 microparticles). It has been shown that IP injections of microparticle drug carriers, including but not limited to CaCO3 microparticles, elicit an immune suppressive and tumor promoting effect in the ID8-fLuc model, mediated by innate immune suppressive cells such as myeloid-derived suppressor cells and M2-like macrophages (30). Both cell types are known to be involved in ovarian cancer development and progression [recently reviewed (31)]. We believe that the slight survival benefit in the ID8-fLuc model can be explained by the fact that the immune-related tumor promoting mechanisms in response to the CaCO3 microparticles partially counteract the therapeutic effect of the 224Ra. In another syngeneic mouse model of disseminated peritoneal disease, albeit of colorectal origin (CT26.WT), treatment with the 224Ra-CaCO3-MPs was able to significantly prolong survival (MSR of 1.8) (28), indicating that the tumor-promoting mechanisms are not universal among different disease models.
One novelty with the current study is the use of the fully immune competent ID8-fLuc mouse model for ovarian cancer. Previously published work on the 224Ra-CaCO3-MPs in ovarian cancer models was performed in immune compromised mouse models (ES-2 and SKOV3). Since the strong immune suppressive tumor microenvironment in patients with ovarian cancer is an important factor in the disease progression (31), we provide additional proof of the therapeutic potential of the 224Ra-CaCO3-MPs in a mouse model that closely resembles this clinical situation. The study design was aimed to mimic the clinically relevant standard of care chemotherapy regimens, although, it should be noted that the IP administration route of the different chemotherapeutics differs from the standard administration route in patients with ovarian cancer (intravenous administration).
However, we recognize that our study encounters some limitations. With the current data, we are not able to provide a mechanistic explanation as to why two chemotherapy chemotherapy regimens result in a different outcome when combined with 224Ra-CaCO3-MPs. Several mechanisms can be responsible for creating synergistic or additive effects between chemotherapy and radiation therapy. The mechanism of action for the specific chemotherapeutic drug may radiosensitize tumor cells to α-radiation to a varying degree. In addition, it is known that different chemotherapy regimens have different effects on the ovarian cancer immune microenvironment in mice (32, 33). Hence, the immune response caused by the 224Ra-CaCO3-MPs and the chemotherapeutics may favor some but not all combinations and dosages. A future characterization of both the cytotoxic mechanisms and immunological responses of the combined 224Ra-CaCO3-MPs and chemotherapy treatment might therefore aid with identifying the most optimal combination regimens.
In the past, other applications with α-particle emitters have been evaluated for the treatment for ovarian cancer. Preclinical evaluation of IP treatment with 211At-labeled monoclonal antibodies showed a high therapeutic efficacy in treating micrometastatic growth in the OVCAR-3 ovarian cancer mouse model (34, 35). Additionally, the α-emitter 212Pb has been evaluated as an IP treatment in the immunodeficient ES-2 and A2780cp20 mouse models for ovarian cancer showing a therapeutic potential when labeled to a monoclonal antibody or CaCO3 microparticles (36, 37). Additionally, 212Pb and 211At colloids have also been investigated previously in a preclinical setting for IP ovarian cancer dissemination, where they have proven their therapeutic potential (38, 39). No immediate and/or late signs of local radiation-induced toxicities were observed in the phase I clinical evaluation of 211At- or 212Pb-labeled antibody treatments in patients with ovarian cancer (18, 19, 40, 41). These results are as expected with the limited range of tissue penetration of α-emitters, preventing irradiation of other radiosensitive organs within the peritoneal cavity.
Furthermore, the combined effects of α-therapies and chemotherapeutics on weight development in mice as a measure for toxicity have been evaluated previously. Milenic and colleagues reported a modest weight loss in mice treated sequentially with 212Pb-trastuzumab and gemcitabine compared to mice that received gemcitabine alone in a model for colon carcinoma (LS-174T) (42), which is in contrast to what we observed in our study. However, the same group reported no difference in weight development between mice that received paclitaxel and 213Bi-trastuzumab or paclitaxel alone in the same tumor model (43), indicating a differential response to combinations of different types and dosages of chemotherapy and radionuclides. Both combination regimens described above also produced synergistic therapeutic effects that could not be reached by these therapeutics separately (42, 43).
We provide proof-of-principle for the therapeutic efficacy 224Ra-CaCO3-MPs in an immune competent mouse model for ovarian cancer, both alone and in combination with chemotherapy. Furthermore, the results indicate a safe sequential administration with two different chemotherapy regimens often used in clinical practice. The results support further evaluation of 224Ra-CaCO3-MPs in patients with ovarian cancer. However, further investigations remain to identify the most optimal chemotherapy regimen to combine with 224Ra-CaCO3-MPs and the sequence of therapies to fully exploit a potential synergistic effect.
Data availability statement
The raw data supporting the conclusions of this article will be made available by the authors, without undue reservation.
Ethics statement
The animal study was reviewed and approved by Katholieke Universiteit Leuven.
Author contributions
Conceptualization and interpretation of results: RW, SW, AC, and TB. Design of experiments: RW and SW. In vivo experiments: RW, YB, and MR. Analysis of results: RW and MR. Statistics: RW and JC. Manuscript writing: RW. Manuscript proof-reading: JC, YB, MR, SW, TB, IV, and AC. Supervision: SW, TB, IV, and AC. Funding acquisition: TB and AC. All authors have read and agreed to the published version of the manuscript.
Funding
This research was funded by the Norwegian Research Council (with project number 304591) and Oncoinvent AS.
Acknowledgments
The authors would like to thank Ann Vankerckhoven, Gitte Thirion, and Katja Vandenbrande for their support in the execution and follow-up of the animal experiments described in this manuscript. Additionally, the R&D department of Oncoinvent AS is acknowledged for the production, shipping, and activity measurements of the 224Ra-labeled CaCO3 microparticles used in these experiments.
Conflict of interest
RW was employed by Oncoinvent AS. AC was a contracted researcher for Oncoinvent AS and Novocure and a consultant for Sotio a.s. SW was employed by and a shareholder of Oncoinvent AS. TB was employed by and a shareholder of Oncoinvent AS. IV is a consultant for Agenus, Akesobio, AstraZeneca, Bristol Myers Squibb, Deciphera Pharmaceuticals, Eisai, Elevar Therapeutics, F. Hoffmann-La Roche, Genmab, GSK, Immunogen, Jazzpharma, Karyopharm, Mersana, MSD, Novocure, Novartis, Oncoinvent, OncXerna, Sanofi, Seagen, Sotio, Verastem Oncology and Zentalis, was a contracted researcher for Oncoinvent AS, performs corporate sponsored research for Amgen and Roche, and receives accommodation and travel expenses from Karyopharm, Genmab and Novocure. The funders (The Norwegian Research Council (project 304591) and Oncoinvent AS) provided support in the form of salaries for authors RW, SW, and TB but did not have any additional role in the study design, data collection, analysis or interpretation, preparation of the manuscript, or decision to publish.
The remaining authors declare that the research was conducted in the absence of any commercial or financial relationships that could be construed as a potential conflict of interest.
Publisher's note
All claims expressed in this article are solely those of the authors and do not necessarily represent those of their affiliated organizations, or those of the publisher, the editors and the reviewers. Any product that may be evaluated in this article, or claim that may be made by its manufacturer, is not guaranteed or endorsed by the publisher.
Supplementary material
The Supplementary Material for this article can be found online at: https://www.frontiersin.org/articles/10.3389/fmed.2022.995325/full#supplementary-material
References
1. Bray F, Ferlay J, Soerjomataram I, Siegel RL, Torre LA, Jemal A. Global cancer statistics 2018: GLOBOCAN estimates of incidence and mortality worldwide for 36 cancers in 185 countries. CA Cancer J Clin. (2018) 68:394–424. doi: 10.3322/caac.21492
2. Cabasag CJ, Fagan PJ, Ferlay J, Jerome V, Mathieu L, Lihua L, et al. Ovarian cancer today and tomorrow: A global assessment by world region and Human Development Index using GLOBOCAN 2020. Int J Cancer. (2022) 151:1535–41. doi: 10.1002/ijc.34002
3. Siegel RL, Miller KD, Jemal A. Cancer statistics 2016. CA Cancer J Clin. (2016) 66:7–30. doi: 10.3322/caac.21332
4. Prat J. Staging classification for cancer of the ovary, fallopian tube, and peritoneum. Int J Gynecol Obstet. (2014) 124:1–5. doi: 10.1016/j.ijgo.2013.10.001
5. Vergote I, Gonzalez-Martin A, Lorusso D, Gourley C, Mirza MR, Kurtz J-E, et al. Clinical research in ovarian cancer: consensus recommendations from the Gynecologic Cancer InterGroup. Lancet Oncol. (2022) 23:e374–84. doi: 10.1016/S1470-2045(22)00139-5
6. Baert T, Ferrero A, Sehouli J, O'donnell DM, González-Martín A, Joly F, et al. The systemic treatment of recurrent ovarian cancer revisited. (2021) 32:710–25. doi: 10.1016/j.annonc.2021.02.015
7. Torre LA, Trabert B, DeSantis CE, Miller KD, Samimi G, Runowicz CD, et al. Ovarian cancer statistics, 2018. CA Cancer J Clin. (2018) 68:284–96. doi: 10.3322/caac.21456
8. Bakri YN, Given FT, Peeples WJ, Frazier AB. Complications from intraperitoneal radioactive phosphorus in ovarian malignancies. Gynecol Oncol. (1985) 21:294–9. doi: 10.1016/0090-8258(85)90266-5
9. Vergote IB, Vergote-De Vos LN, Abeler VM, Aas M, Lindegaard MW, Kjmstad KE, et al. Randomized trial comparing cisplatin with radioactive phosphorus or whole-abdomen irradiation as adjuvant treatment of ovarian cancer. Cancer. (1992) 69:741–9.3.0.CO;2-G“ target=”_blank“>https://doi.org/10.1002/1097-0142(19920201)69:3<741::AID-CNCR2820690322>3.0.CO;2-G
10. Rosenshein NB, Leichner PK, Vogelsang G. Radiocolloids in the treatment of ovarian cancer. Obstet Gynecol Surv. (1979) 34:708–20. doi: 10.1097/00006254-197909000-00028
11. Aure J, Høeg K, Kolstad P. Radioactive colloidal gold in the treatment of ovarian carcinoma. Acta Radiol Ther Phys Biol. (1971) 10:399–407. doi: 10.3109/02841867109130785
12. Verheijen RH, Massuger LF, Benigno BB, Epenetos AA, Lopes A, Soper JT, et al. Phase iii trial of intraperitoneal therapy with yttrium-90-labeled hmfg1 murine monoclonal antibody in patients with epithelial ovarian cancer after a surgically defined complete remission. J Clin Oncol. (2006) 24:571–8. doi: 10.1200/JCO.2005.02.5973
13. Epenetos AA, Hird V, Lambert H, Mason P, Coulter C. Long term survival of patients with advanced ovarian cancer treated with intraperitoneal radioimmunotherapy. Int J Gynecol Cancer. (2000) 10 (Suppl. 1):44–6. doi: 10.1046/j.1525-1438.2000.99510.x
14. Kino T, Ando N, Ogawara Y, Shigeta H. Proton beam therapy for recurrent ovarian carcinoma: A case report. J Obstet Gynaecol Res. (2019) 45:1952–6. doi: 10.1111/jog.14036
15. Nawa A, Suzuki K, Kato S, Fujiwara S, Kajiyama H, Shibata K, et al. Carbon beam therapy in recurrent ovarian cancer. Ann Oncol. (2008) 19:192–4. doi: 10.1093/annonc/mdm553
16. Vergote I, Larsen RH, De Vos L, Nesland JM, Bruland Ø, Bjørgum J, et al. Therapeutic efficacy of the α-emitter 211At bound on microspheres compared with 90Y and 32P colloids in a murine intraperitoneal tumor model. Gynecol Oncol. (1992) 47:366–72. doi: 10.1016/0090-8258(92)90141-5
17. Parker C, Nilsson S, Heinrich D, Helle SI, O'Sullivan JM, Fosså SD, et al. Alpha emitter radium-223 and survival in metastatic prostate cancer. N Engl J Med. (2013) 369:213–23. doi: 10.1056/NEJMoa1213755
18. Hallqvist A, Bergmark K, Bäck T, Andersson H, Dahm-Kähler P, Johansson M, et al. Intraperitoneal α-emitting radioimmunotherapy with 211At in relapsed ovarian cancer: long-term follow-up with individual absorbed dose estimations. J Nucl Med. (2019) 60:1073–9. doi: 10.2967/jnumed.118.220384
19. Meredith RF, Torgue JJ, Rozgaja TA, Banaga EP, Bunch PW, Alvarez RD, et al. Safety and outcome measures of first-in-human intraperitoneal α radioimmunotherapy with 212Pb-TCMC-trastuzumab. Am J Clin Oncol. (2018) 41:716. doi: 10.1097/COC.0000000000000353
20. Westrøm S, Bønsdorff TB, Bruland ØS, Larsen RH. Therapeutic effect of α-emitting 224 ra-labeled calcium carbonate microparticles in mice with intraperitoneal ovarian cancer. Transl Oncol. (2018) 11:259–67. doi: 10.1016/j.tranon.2017.12.011
21. Li RG, Napoli E, Jorstad IS, Bønsdorff TB, Juzeniene A, Bruland ØS, et al. Calcium carbonate microparticles as carriers of 224ra: impact of specific activity in mice with intraperitoneal ovarian cancer. Curr Radiopharm. (2020) 13. doi: 10.2174/18744710MTEx5OTYyy
22. National Library of Medicine (US). Identifier NCT03732768. Study of Radspherin® in Recurrent Ovarian Cancer Subjects With Peritoneal Carcinomatosis. ClinicalTrials.gov.
23. National Library of Medicine (US). Identifier NCT03732781. Study of Radspherin® in Colorectal Carcinoma Subjects With Peritoneal Carcinomatosis Treated With HIPEC. ClinicalTrials.gov.
24. Baert T, Verschuere T, Van Hoylandt A, Gijsbers R, Vergote I, Coosemans A. The dark side of ID8-Luc2: pitfalls for luciferase tagged murine models for ovarian cancer. J Immunother Cancer. (2015) 3:57. doi: 10.1186/s40425-015-0102-0
25. Kilkenny C, Browne WJ, Cuthill IC, Emerson M, Altman DG. Improving bioscience research reporting: The arrive guidelines for reporting animal research. PLoS Biol. (2010) 8:e1000412. doi: 10.1371/journal.pbio.1000412
26. Council NR. Guide for the Care and Use of Laboratory Animals. Guide for the Care and Use of Laboratory Animals. Washington, DC: National Academies Press. (2011).
27. Westrøm S, Malenge M, Jorstad IS, Napoli E, Bruland ØS, Bønsdorff TB, et al. Ra-224 labeling of calcium carbonate microparticles for internal α-therapy: Preparation, stability, and biodistribution in mice. J Labelled Comp Radiopharm. (2018) 61:472–86. doi: 10.1002/jlcr.3610
28. Li RG, Lindland K, Tonstad SK, Bønsdorff TB, Juzeniene A, Westrøm S, et al. Improved formulation of 224Ra-labeled calcium carbonate microparticles by surface layer encapsulation and addition of EDTMP. Pharmaceutics. (2021) 13:5. doi: 10.3390/pharmaceutics13050634
29. Demidenko E, Miller TW. Statistical determination of synergy based on Bliss definition of drugs independence. PLoS ONE. (2019) 14:e0224137. doi: 10.1371/journal.pone.0224137
30. Wouters R, Westrøm S, Vankerckhoven A, Thirion G, Ceusters J, Claes S, et al. Effect of particle carriers for intraperitoneal drug delivery on the course of ovarian cancer and its immune microenvironment in a mouse model. Pharm. (2022) 14:687. doi: 10.3390/pharmaceutics14040687
31. Fucikova J, Coosemans A, Orsulic S, Cibula D, Vergote I, Galluzzi L, et al. Immunological configuration of ovarian carcinoma: features and impact on disease outcome. J Immunother Cancer. (2021) 9:2873. doi: 10.1136/jitc-2021-002873
32. Vankerckhoven A, Baert T, Riva M, De Bruyn C, Thirion G, Vandenbrande K, et al. Type of chemotherapy has substantial effects on the immune system in ovarian cancer. Transl Oncol. (2021) 14:101076. doi: 10.1016/j.tranon.2021.101076
33. Zitvogel L, Apetoh L, Ghiringhelli F, Kroemer G. Immunological aspects of cancer chemotherapy. Nat Rev Immunol. (2008) 8:59–73. doi: 10.1038/nri2216
34. Elgqvist J, Andersson H, Bäck T, Hultborn R, Jensen H, Karlsson B, et al. Therapeutic efficacy and tumor dose estimations in radioimmunotherapy of intraperitoneally growing OVCAR-3 cells in nude mice with 211At-labeled monoclonal antibody MX35. J Nucl Med. (2005) 46.
35. Elgqvist J, Andersson H, Jensen H, Kahu H, Lindegren S, Warnhammar E, et al. Repeated intraperitoneal alpha-radioimmunotherapy of ovarian cancer in mice. J Oncol. (2010) 2010:394913. doi: 10.1155/2010/394913
36. Kasten BB, Arend RC, Katre AA, Kim H, Fan J, Ferrone S, et al. B7-H3-targeted 212Pb radioimmunotherapy of ovarian cancer in preclinical models. Nucl Med Biol. (2017) 47:23–30. doi: 10.1016/j.nucmedbio.2017.01.003
37. Li RG, Lindland K, Bønsdorff TB, Westrøm S, Larsen RH. A novel single-step-labeled 212Pb-CaCO3 microparticle for internal alpha therapy: preparation, stability, and preclinical data from mice. Materials (Basel). (2021) 14:7130. doi: 10.3390/ma14237130
38. Bloomer WD, McLaughlin WH, Neirinckx RD, Adelstein SJ, Gordon PR, Ruth TJ, et al. Astatine-211—tellurium radiocolloid cures experimental malignant ascites. Science. (1981) 212:340–1. doi: 10.1126/science.7209534
39. Rotmensch J, Atcher RW, Schlenker R, Hines J, Grdina D, Block BS, et al. The effect of the α-emitting radionuclide lead-212 on human ovarian carcinoma: A potential new form of therapy. Gynecol Oncol. (1989) 32:236–9. doi: 10.1016/S0090-8258(89)80040-X
40. Meredith R, Torgue J, Shen S, Fisher DR, Banaga E, Bunch P, et al. Dose escalation and dosimetry of first-in-human α radioimmunotherapy with 212Pb-TCMC-trastuzumab. J Nucl Med. (2014) 55:1636–42. doi: 10.2967/jnumed.114.143842
41. Meredith RF, Torgue J, Azure MT, Shen S, Saddekni S, Banaga E, et al. Pharmacokinetics and imaging of 212Pb-TCMC-trastuzumab after intraperitoneal administration in ovarian cancer patients. Cancer Biother Radiopharm. (2014) 29:12. doi: 10.1089/cbr.2013.1531
42. Milenic DE, Garmestani K, Brady ED, Albert PS, Abdulla A, Flynn J, et al. Potentiation of high-LET radiation by gemcitabine: targeting HER2 with trastuzumab to treat disseminated peritoneal disease. Clin Cancer Res. (2007) 13:1926–35. doi: 10.1158/1078-0432.CCR-06-2300
Keywords: ovarian cancer, alpha therapy, radium-224, chemotherapy, carboplatin, paclitaxel, pegylated liposomal doxorubicin
Citation: Wouters R, Westrøm S, Berckmans Y, Riva M, Ceusters J, Bønsdorff TB, Vergote I and Coosemans A (2022) Intraperitoneal alpha therapy with 224Ra-labeled microparticles combined with chemotherapy in an ovarian cancer mouse model. Front. Med. 9:995325. doi: 10.3389/fmed.2022.995325
Received: 15 July 2022; Accepted: 21 September 2022;
Published: 10 October 2022.
Edited by:
Giorgio Treglia, Ente Ospedaliero Cantonale (EOC), SwitzerlandReviewed by:
Weijun Wei, Shanghai Jiao Tong University, ChinaRonghua Liu, Huazhong University of Science and Technology, China
Copyright © 2022 Wouters, Westrøm, Berckmans, Riva, Ceusters, Bønsdorff, Vergote and Coosemans. This is an open-access article distributed under the terms of the Creative Commons Attribution License (CC BY). The use, distribution or reproduction in other forums is permitted, provided the original author(s) and the copyright owner(s) are credited and that the original publication in this journal is cited, in accordance with accepted academic practice. No use, distribution or reproduction is permitted which does not comply with these terms.
*Correspondence: Roxanne Wouters, roxanne.wouters@kuleuven.be