- 1State Key Laboratory of Respiratory Disease, National Clinical Research Center for Respiratory Disease, Guangzhou Institute of Respiratory Health, First Affiliated Hospital of Guangzhou Medical University, Guangzhou, China
- 2Respiratory Mechanics Laboratory, Guangzhou Institute of Respiratory Health, First Affiliated Hospital of Guangzhou Medical University, Guangzhou, China
- 3Key Laboratory of Shenzhen Respiratory Diseases, Institute of Shenzhen Respiratory Diseases, Shenzhen People’s Hospital (The First Affiliated Hospital of Southern University of Science and Technology, The Second Clinical Medical College of Jinan University), Shenzhen, Guangdong, China
Background: JAK (Janus kinases) inhibitors have been proposed as a promising treatment option for the coronavirus disease-2019 (COVID-19). However, the benefits of JAK inhibitors and the optimum thereof for COVID-19 have not been adequately defined.
Methods: Databases were searched from their inception dates to 17 June 2022. Eligible studies included randomized controlled trials and observational studies. Extracted data were analyzed by pairwise and network meta-analysis. The primary outcome was the coefficient of mortality.
Results: Twenty-eight studies of 8,206 patients were included and assessed qualitatively (modified Jadad and Newcastle–Ottawa Scale scores). A pairwise meta-analysis revealed that JAK inhibitors effectively reduced the mortality (OR = 0.54; 95% CI: 0.46–0.63; P < 0.00001; I2 = 32%) without increasing the risk of adverse events (OR = 1.02; 95% CI: 0.88–1.18; P = 0.79; I2 = 12%). In a network meta-analysis, clinical efficacy benefits were seen among different types of JAK inhibitors (baricitinib, ruxolitinib, and tofacitinib) without the observation of a declined incidence of adverse events. The assessment of rank probabilities indicated that ruxolitinib presented the greatest likelihood of benefits regarding mortality and adverse events.
Conclusion: JAK inhibitors appear to be a promising treatment for COVID-19 concerning reducing mortality, and they do not increase the risk of adverse events vs. standard of care. A network meta-analysis suggests that mortality benefits are associated with specific JAK inhibitors, and among these, ruxolitinib presents the greatest likelihood of having benefits for mortality and adverse events.
Systematic review registration: [www.crd.york.ac.uk/prospero], identifier [CRD42022343338].
Background
The severe acute respiratory syndrome-coronavirus-2 [SARS-CoV-2/coronavirus disease-2019 (COVID-19)] pandemic has emerged as an extraordinary challenge to public health. According to the World Health Organization’s most recent weekly epidemiological update on COVID-19 (17 June 2022), the cumulative number of cases reported globally since 2019 exceeds 535 million, and the number of deaths caused by this infection has surpassed 6.31 million. The number of people diagnosed has been increasing globally as variants continue to emerge, even as vaccines have been administered in multiple countries.
The primary cause of death from COVID-19 is acute respiratory distress syndrome, while cytokine release syndrome (CRS), characterized by increased interleukin (IL)-6, IL-2, IL-7, IL-10, is thought to be the main reason for multiple organ failure (1). Therefore, treatment of cytokine storm has been proposed as a key part of rescuing severe COVID-19 disease. Several cytokines associated with COVID-19 disease employ a unique intracellular signaling pathway mediated by Janus kinase (JAK). JAK inhibition provides an attractive therapeutic strategy for CRS (2). Accordingly, effective inhibition of cytokine storms is crucial for preventing severe COVID-19 complications and reducing mortality (3).
JAK-signal transducer and activator of transcription (STAT) signaling is critical to multiple cellular processes, including survival, differentiation, and proliferation (4). Over the past decade, JAK inhibitors have known a wide range of applications in the clinic and have been constantly designed for new molecules. Ruxolitinib, one of the oldest JAK inhibitors, is the drug most commonly used in patients with hematologic disorders, while other JAK inhibitors such as baricitinib and tofacitinib are more commonly used in systemic rheumatic diseases (5). JAK-STAT inhibitors can block many proinflammatory and anti-inflammatory cytokines and have good pharmacodynamics. At the same time because of its short half-life, the extent and duration of cytokine arrest can be easily monitored by adjusting the dose and duration of treatment (6, 7).
Four previous meta-analyses have reported that JAK inhibitors could be beneficial in the treatment of COVID-19 (8–11). However, all of these studies were pairwise meta-analyses comparing the JAK inhibitors with the standard of care (SOC). There was no comparison of efficacy and safety between JAK inhibitors in COVID-19 patients. To solve this problem, this research sought to further explore the efficacy and safety of JAK inhibitors in patients with COVID-19 by updating the latest relevant evidence and using a network meta-analysis (NMA) approach to assess the efficacy and safety of three JAK inhibitors (baricitinib, ruxolitinib and tofacitinib) through indirect comparison. The primary outcome was mortality. Because many symptoms of COVID-19 overlap with the adverse events of JAK inhibitors, in all relevant cohort studies, it has not been clarified whether the adverse events were caused by symptoms of COVID-19 disease itself or by taking JAK inhibitors (12, 13). Therefore, this study only focused on whether treatment of JAK inhibitors increased the overall risk of these adverse events compared with SOC, and the adverse events analyzed in this study were based on the data of the included studies.
Methods
This systematic review and meta-analysis were performed under the instruction of PRISMA guidance,1 and the protocol for research was registered at PROSPERO (CRD42022343338).
Search strategy
This was a pairwise and Bayesian network meta-analysis study of clinical trials and observational studies. Published studies included in the meta-analysis were those that fit within the following PICO framework (P: Populations, hospitalized COVID-19 patients; I: Interventions, treatment with JAK inhibitors; C: Comparator/Control, a group of patients who only received SOC therapy and was not treated with JAK inhibitors; O: Outcomes, mortality and the risk of adverse events), and met the Preferred Reporting Items for pairwise and Bayesian network meta-analysis (PRISMA) criteria (14).
PubMed, Embase, Clinical Trial, and Web of Science were each searched from their inception to 17 June 2022 by three independent reviewers. The keywords “JAK inhibitors OR Baricitinib OR Ruxolitinib OR Tofacitinib” AND “SARS-CoV-2 OR coronavirus disease 2019 OR COVID-19” were used. The investigators independently screened titles and abstracts generated by the search. The search was reworked before the final analyses to review the latest studies.
Inclusion and exclusion criteria
The comparative trials that met all the following criteria was included: (1) The studies were in English; (2) a JAK inhibitor (baricitinib, ruxolitinib, tofacitinib or nezulcitinib) was used alone or with other therapies in the experimental group who were suffering from COVID-19, while the SOC was applied in the control group. SOC in the control groups included patients with the application of oxygen via low-flow or high-flow devices, invasive or non-invasive ventilation and other medication like steroids or remdesivir; (3) the survey was conducted among adults; (4) Clinical outcomes of interest (all-cause mortality and adverse events) were reported; (5) the included researches were cohort, randomized or non-randomized clinical trial research; (6) the included researches were correspondence or review articles, case-series or case report studies.
Data extraction and study quality assessment
After selection, the full text articles in electronic versions were then carefully evaluated for data extraction. Two independent reviewers extracted data independently using predefined standardized forms and one independent reviewer joined when any disagreements existed. Each full article that met the inclusion criteria was carefully reviewed, and the following baseline information was extracted: first author, publication year, study type, basic information of the participants (age, severity), the total number of participants, number of participants receiving JAK inhibitors (baricitinib, ruxolitinib, tofacitinib and nezulcitinib), number of participants receiving SOC, the regimen of JAK inhibitors or SOC and the modified Jadad (15) or Newcastle–Ottawa Scale (NOS) score (16). The outcome measures were mortality and the risk of adverse events.
Three independent reviewers independently assessed the quality of each study involved in this review. Randomized clinical trials (RCTs) and clinical trials included in the final analyses were scored by one independent reviewer to formally assess the risk of bias using the modified Jadad score. Observational studies included in the final analyses were scored by one independent reviewer using the NOS score. There was adjudication by one independent reviewer when there was disagreement.
The modified Jadad scale was used to evaluate the quality of RCTs and clinical trials, including the presence of randomization (0 or 2), blinding (0 or 2), description of withdrawals and dropouts (0 or 1), inclusion/exclusion criteria (0 or 1), adverse effects (0 or 1) and statistical analysis (0 or 1) for each study. The studies were scored from zero to eight, with 1–3 signifying low quality and 4–8 signifying high quality (17). The NOS score was used to evaluate the quality of observational studies. The representativeness of the exposed cohort (0 or 1), selection of the non-exposed cohort (0 or 1), ascertainment of exposure (0 or 1), whether the subjects had the disease they were studying at the start of the study (0 or 1), comparability (0 or 1), non-comparability (0 or 1), method (0 or 1), follow-up time (0 or 1) and adequacy of follow-up of cohorts (0 or 1) were reported for the NOS score. Research is graded as good quality if it scores ≥ 7 (18).
Statistical analysis
Review Manager V.5.3 software (Cochrane Collaboration, Oxford, UK) for pairwise meta-analysis was used. To calculate the odds ratio (OR) and its 95% confidence interval (95% CI) for dichotomous outcomes (mortality and adverse events), the Mantel-Haenszel formula was utilized. Heterogeneity was assessed using the Cochran Q statistic and the I2 statistic. The I2 values ≤ 50% were considered an acceptable heterogeneity between studies, and the fixed-effects model was selected. Otherwise, determine the source of heterogeneity through subgroup analysis, and conduct sensitivity analysis or use random effect model. For subgroup analyses, the studies were split into four subgroups according to different drugs and applied a pairwise meta-analysis model separately in each subgroup.
The R software (version 3.6.1) and R package “gemtc” were mainly employed to construct the Bayesian network meta-analysis with 3 chains simulated for 50,000 iterations using The Markov Chain Monte Carlo method (17). Fixed-effect model was utilized, and the random-effect model was applied when the heterogeneity was high. The Bayesian approach also provided overall ranking probabilities for each treatment, making it possible to rank each outcome measurement from the best to the worst, and were then visualized by rankogram. The drug with the largest proportion of dark gray rectangles (best treatment) represents the drug that is considered to have the highest probability of the most negative value of mortality or adverse events.
Results
Selection and characteristics of the studies
The PRISMA flow diagram was shown in Figure 1. A total of 3,310 articles were identified initially. 103 highly relevant articles were identified by searching titles and abstracts and eliminating repetitions. After examining the content further, 28 studies (19–46) comprising 8,826 patients remained. Of the 28 studies, nine were double-blind, RCTs, two were non-randomized clinical trials, and 17 were prospective cohort or retrospective cohort studies (Table 1). The quality assessment for these studies was shown in Figure 2.
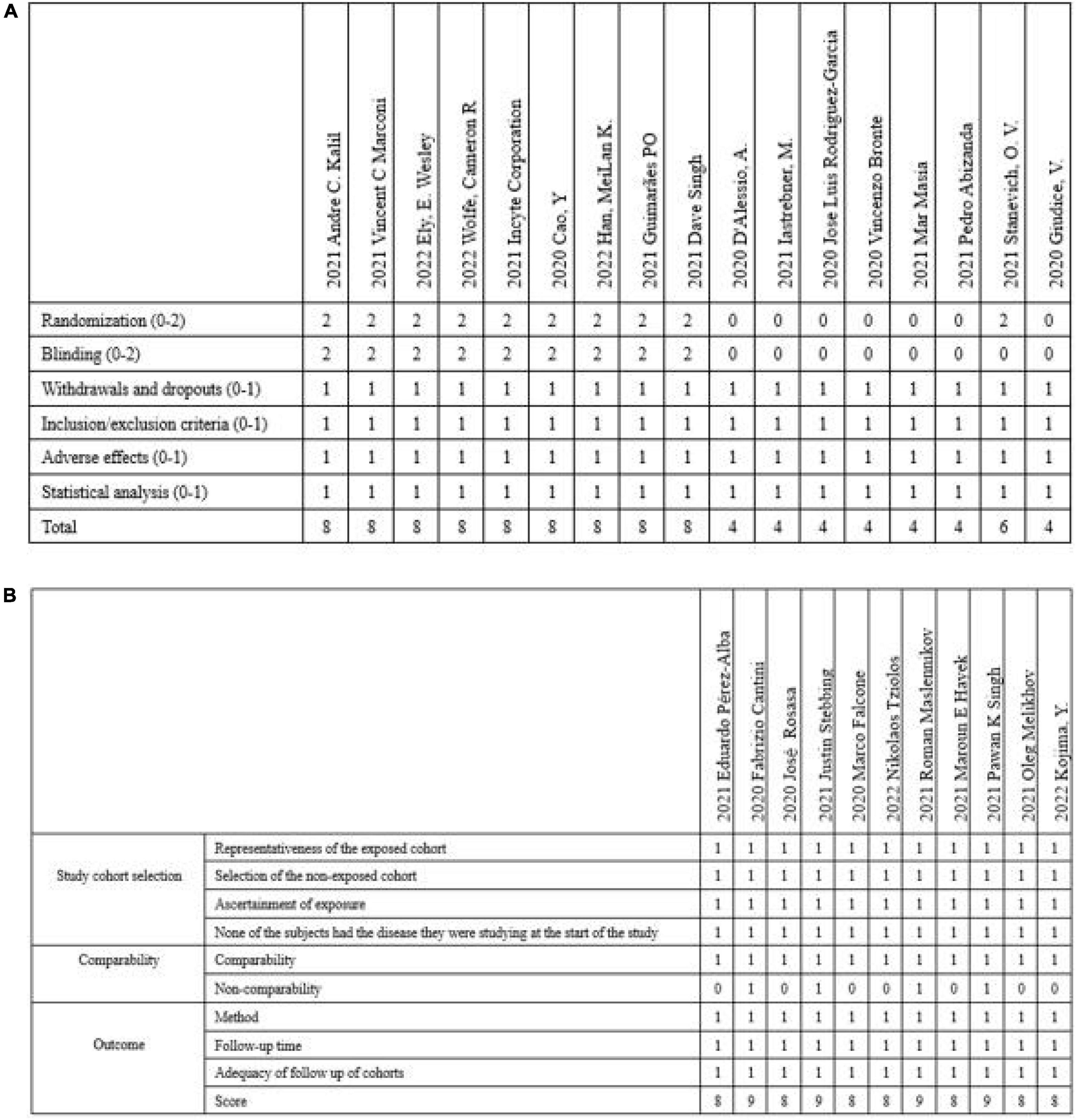
Figure 2. The quality assessment for these studies included in the article. (A) Modified Jadad score was used to score RCTs and clinical trials included. (B) NOS score was used to score observational studies. RCTs, randomized clinical trials; NOS, Newcastle–Ottawa Scale.
Baseline characteristics of the included studies
Data on the first author, sample size, study design, age and severity of patients, outcome, the specific regimen of JAK-inhibitor used in the experimental group, and the specific regimen of SOC used in the control group were shown in Table 1.
Study quality assessment
The modified Jadad scale was used to evaluate the quality of RCTs and clinical trials. And the NOS score was used to evaluate the quality of observational studies (Figure 2).
Pairwise meta-analysis results
Mortality
The outcomes of mortality in the JAK inhibitors groups vs. the SOC groups were shown in Figure 3. Twenty-six studies including 8,206 patients reported the mortality (19–40, 43–47). Pooled results showed that treatment with JAK inhibitors was associated with significantly lower mortality compared with that in the SOC groups (OR = 0.54; 95% CI: 0.46–0.63; P < 0.00001; Figure 3). There was low statistical heterogeneity in the fixed effect model (I2 = 32%).
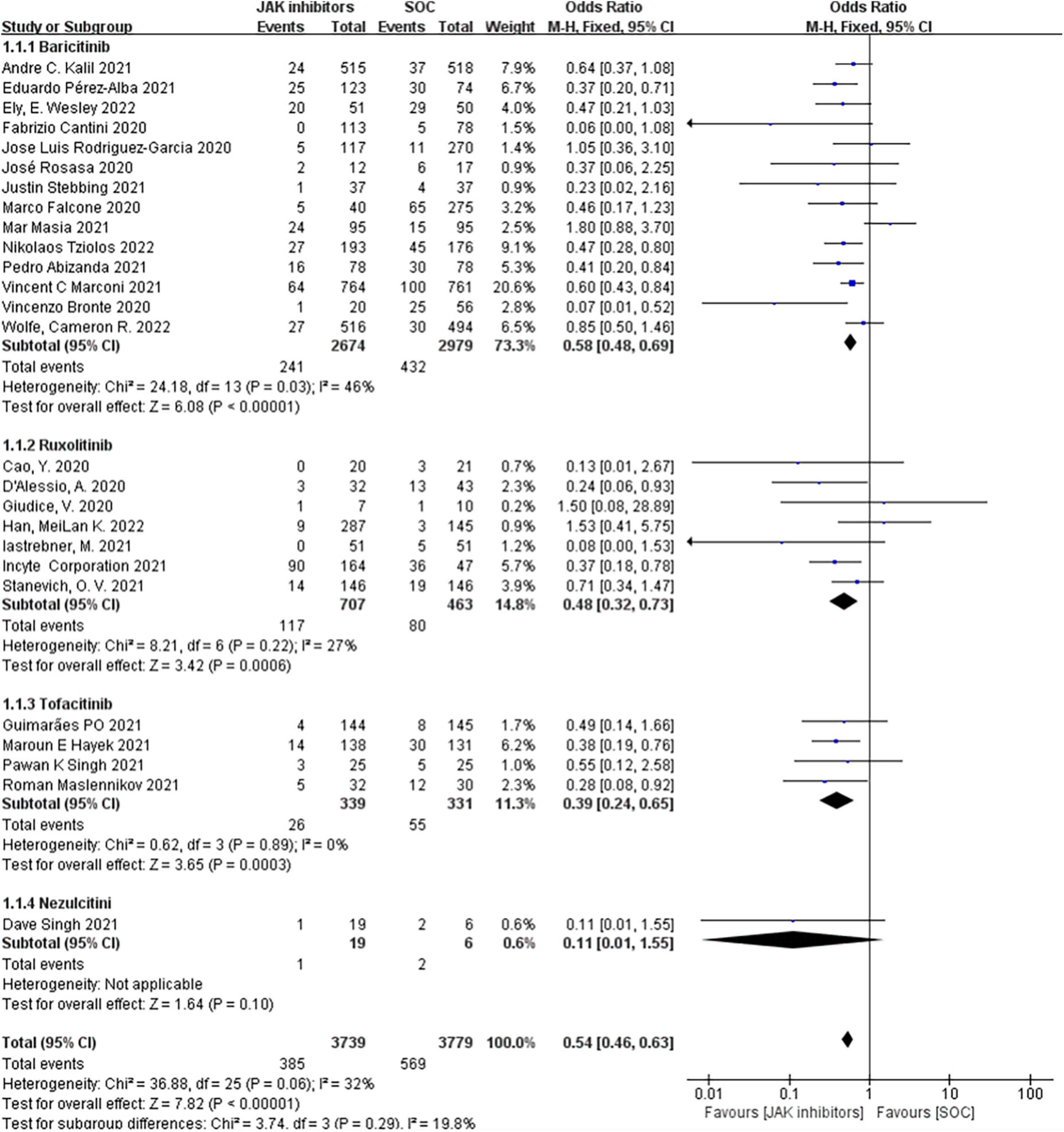
Figure 3. Forest plots of effect of JAK inhibitors compared to SOC on mortality. The study was divided into four subgroups by different drugs. SOC, standard of care.
Our subgroup analyzed by different types of JAK inhibitors found that in the 14 studies of 5,653 patients treated with baricitinib (19, 23–26, 32–37, 43, 45, 47), the effect vs. SOC in patients produced an OR of 0.58 (95% CI: 0.48–0.69; P < 0.00001; I2 = 46%; Figure 3); the seven studies of 1,170 ruxolitinib-treated patients (21, 27–30, 40, 44) reported an OR of 0.48 (95% CI: 0.32–0.73; P = 0.0006; I2 = 27%; Figure 3) vs. SOC; four studies of 670 patients treated with tofacitinib (22, 31, 38, 39) reported an OR of 0.39 (95% CI: 0.24–0.65; P = 0.0003; I2 = 0%; Figure 3) vs. SOC; and only one study of 25 patients treated with nezulcitini (44) reported an OR of 0.11 (95% CI: 0.01–1.55; P = 0.10; Figure 3).
Adverse events
When examining adverse events associated with JAK inhibitor treatment in patients with COVID-19, the most frequent adverse events were infections, embolisms, liver dysfunction, renal and urinary disorders, and mental disorders. Among these, infection and thrombosis after receiving the drug were the most concerned adverse events for doctors. Therefore, this meta-analysis focused on the selection of the incidence of them during data screening and discussed the comparison with the control.
Nineteen studies including 6,173 patients investigated the effect of JAK inhibitors on adverse events. The pooled results showed no significant differences between the JAK inhibitors group and the control groups in the overall adverse event (OR = 1.02; 95% CI: 0.88–1.18; P = 0.79; I2 = 12%; Figure 4). The subgroup analyzed by type of JAK inhibitor showed no difference in the adverse event from standard treatment for any of the three drugs.
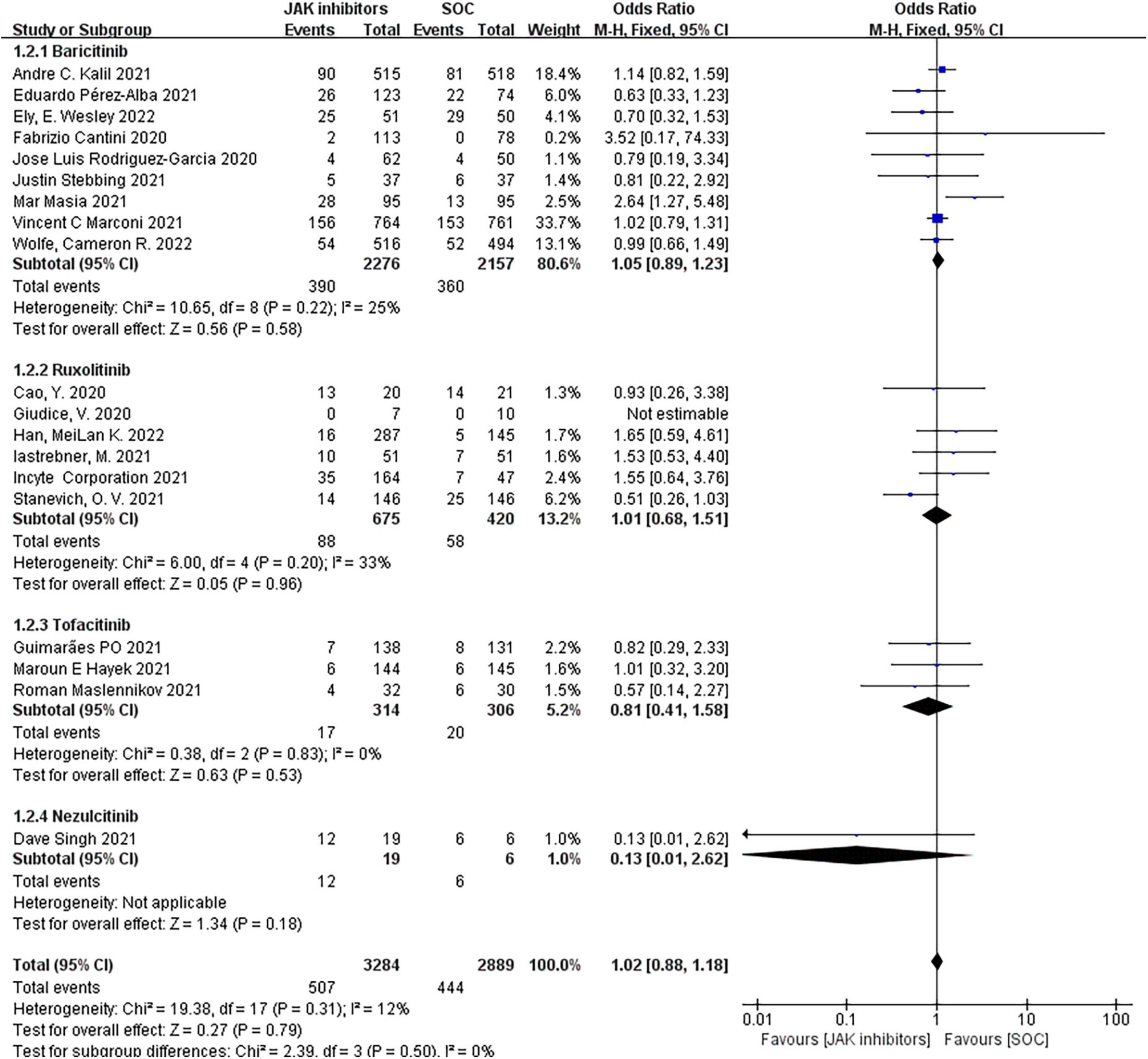
Figure 4. Forest plots of effect of JAK inhibitors compared to SOC on adverse events. The study was divided into four subgroups by different drugs. SOC, standard of care.
Publication bias and sensitivity analyses
Funnel plot analysis for mortality and the incidence of adverse events presented a relatively symmetrical inverted plot (Supplementary Figure 1), indicating no publication bias. Sensitivity analyses were also conducted to assess the impact of each study on the pooled OR, and the statistical results were not remarkably altered after removing any study (Supplementary Figure 2).
Network meta-analysis results
Figure 5 showed a comparison of network evidence from studies of mortality involved in treatment with multiple JAK inhibitors. The thickness of connecting lines represented the number of trials between each comparator, and the size of each node corresponded to the number of participants who received the same intervention (sample size).
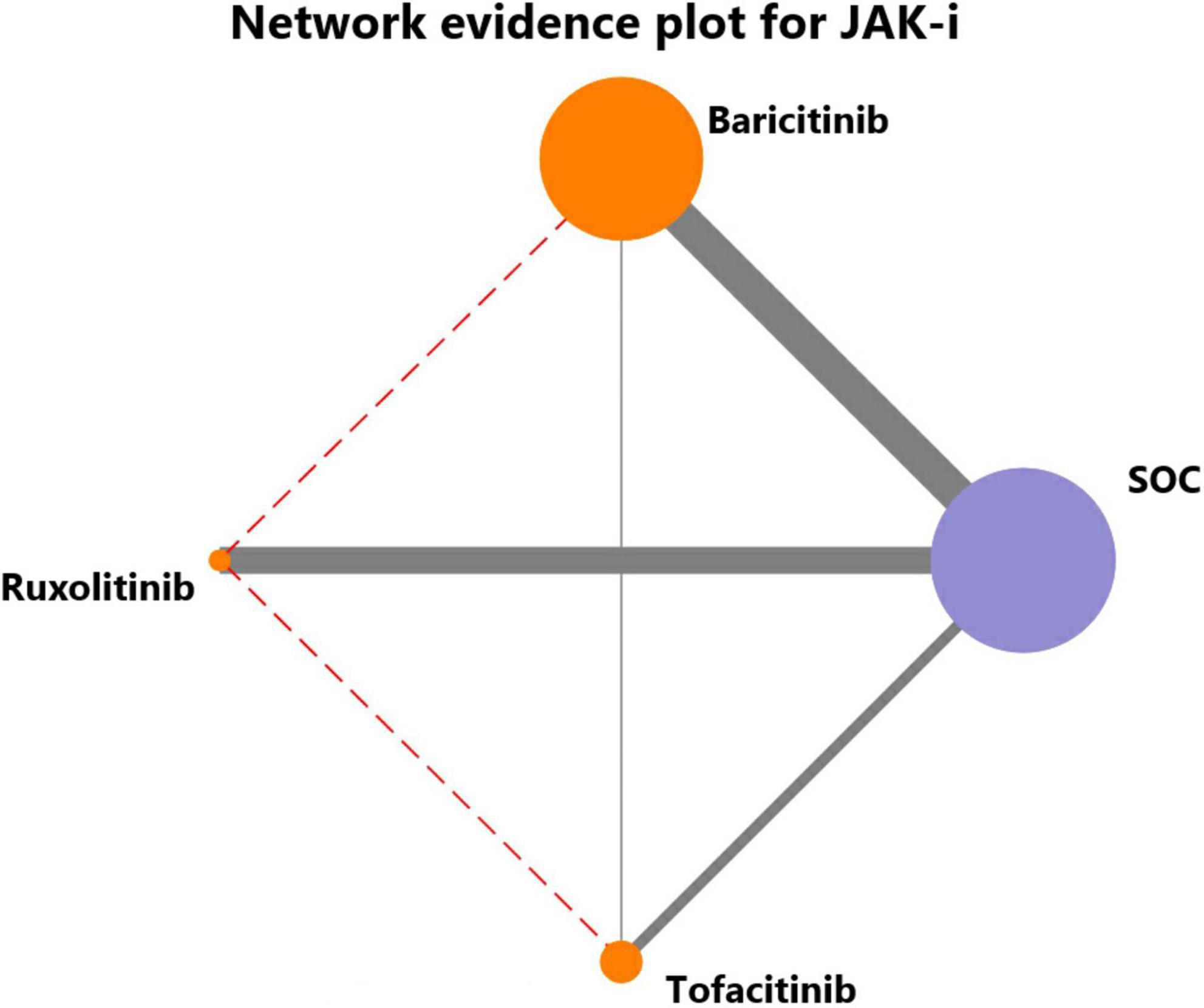
Figure 5. Network evidence for JAK inhibitors to treat COVID-19. The thickness of connecting lines represented the number of trials between each comparator, and the size of each node corresponded to the number of participants who received the same intervention (sample size).
Mortality
Fifteen trials were comparing the coefficient of mortality among baricitinib-treated patients, seven studies among ruxolitinib-treated patients, and four trials among tofacitinib-treated patients. Thus, this research first pooled direct comparisons to obtain indirect comparisons by comparing baricitinib vs. SOC (19, 23–26, 32–37, 43, 45, 47), ruxolitinib vs. SOC (21, 27–30, 40, 44), tofacitinib vs. SOC (22, 31, 38, 39), and tofacitinib vs. baricitinib (41, 42).
In individual comparisons for mortality using SOC as the reference, baricitinib, ruxolitinib and tofacitinib were all more likely to reduce the mortality (NMA: OR = 0.46, 95% CI: 0.29–0.65; OR = 0.43, 95% CI: 0.20–0.84; OR = 0.60, 95% CI: 0.31–1.2, respectively; Figure 6A). There were no significant differences among the other comparisons of the NMA for mortality. The assessment of rank probabilities indicated that ruxolitinib (51.5% probability) presented the greatest likelihood of reducing mortality among the evaluated JAK inhibitors, followed by baricitinib (36.9% probability) and then tofacitinib (11.5% probability) (Figure 6B).
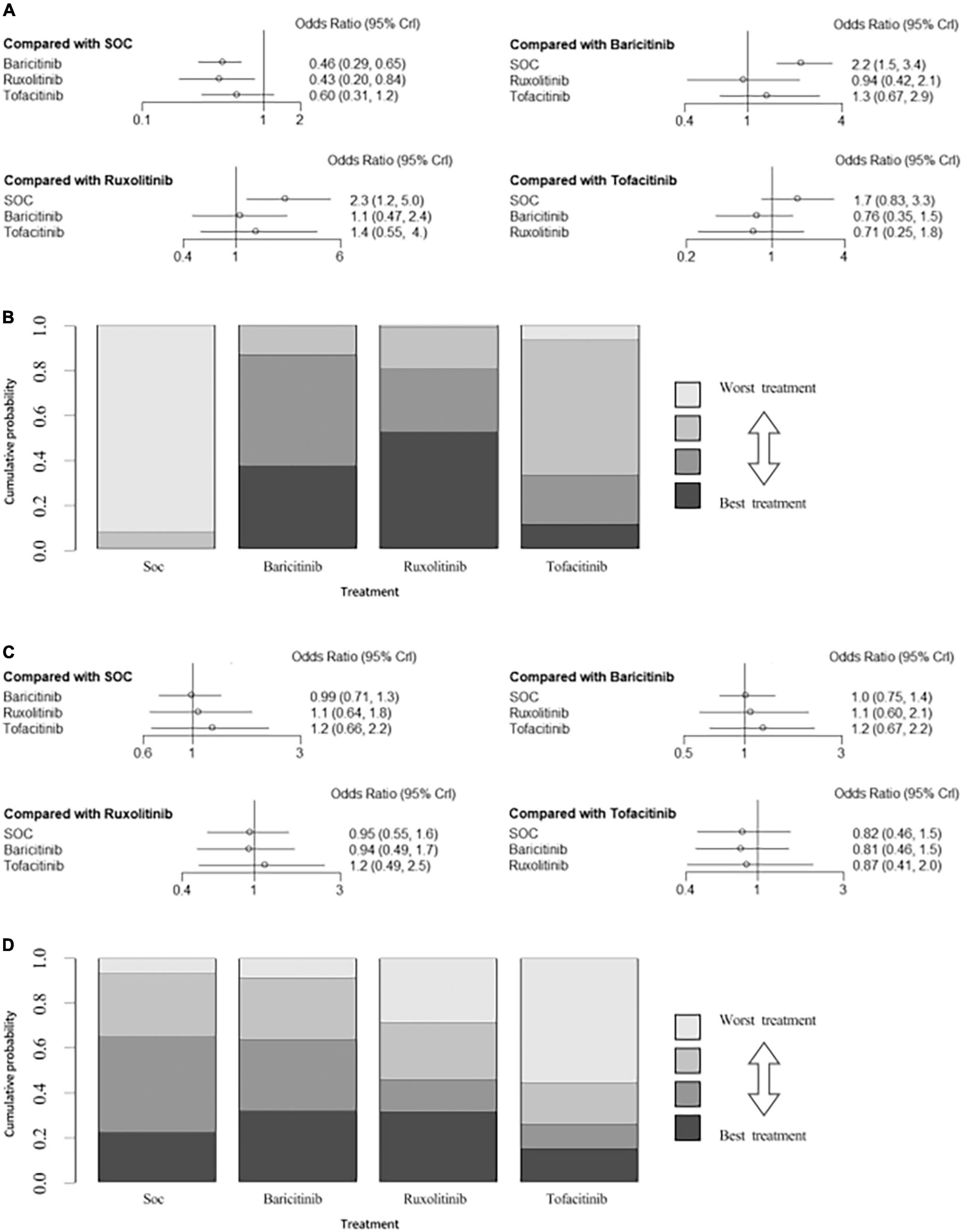
Figure 6. (A) Network estimates mortality among JAK inhibitors. (B) Rank probabilities among JAK inhibitors for mortality based on the network meta-analysis. (C) Network estimates adverse events among JAK inhibitors. (D) Rank probabilities among JAK inhibitors for adverse events based on the network meta-analysis. CI, confidence interval.
Adverse events
There were no significant differences among all comparisons of the network meta-analysis for adverse events (Figure 6C). Ranking analysis revealed that baricitinib presented the lowest rate of adverse events (31.7% probability), followed by ruxolitinib (31.3% probability) and then tofacitinib (14.8% probability) (Figure 6D).
Two-dimensional plot of primary outcomes
The pooled estimates of two primary outcomes were projected to a two-dimensional (2D) plot, thus hierarchical information about efficacy and incidence of adverse events could be obtained simultaneously and help us understand the optimal choice for COVID-19. The 2D plot revealed ruxolitinib as the optimal choice among all JAK inhibitors (Figure 7). Baricitinib could be an alternative option as well.
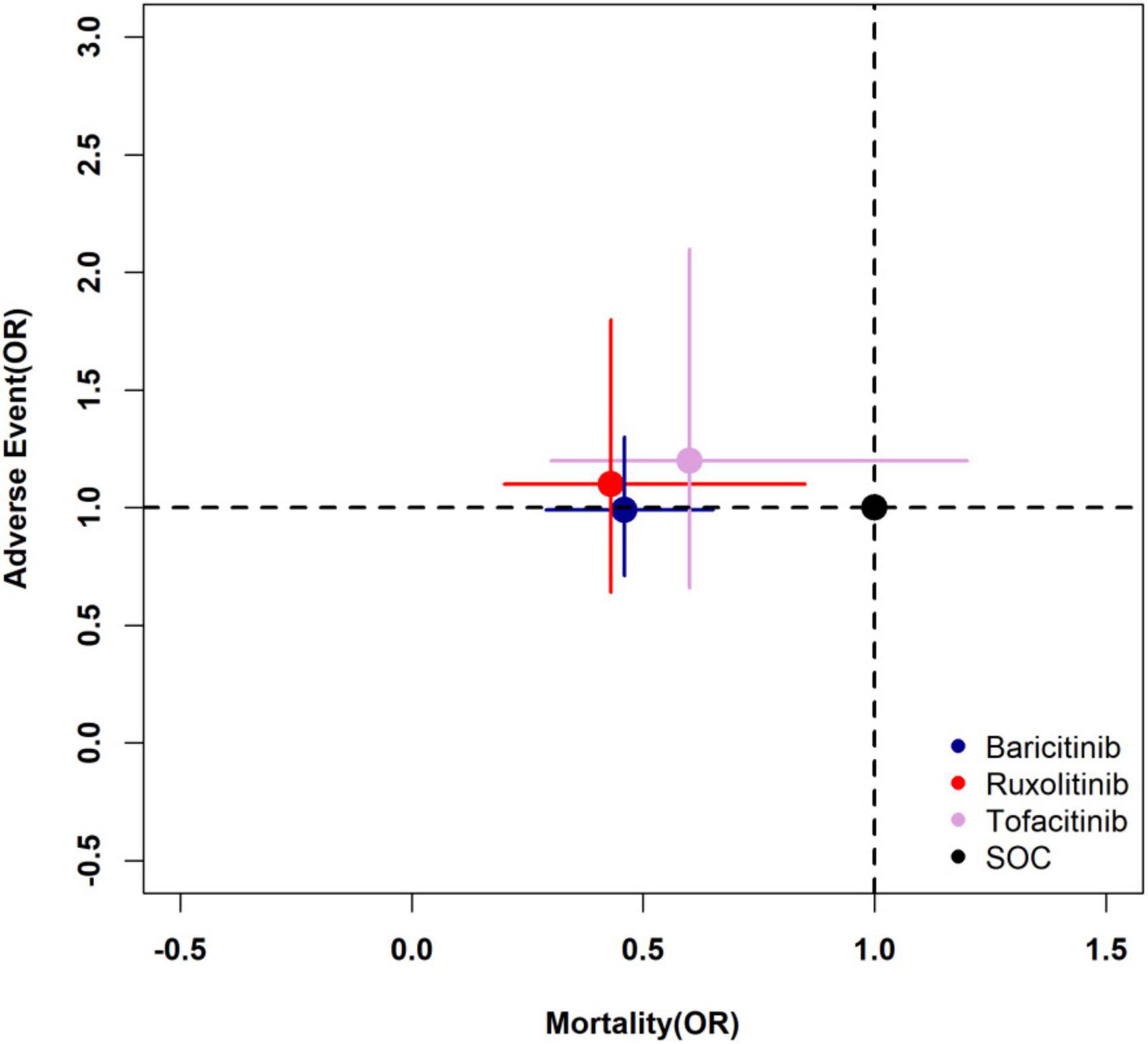
Figure 7. Two-dimensional plot of primary outcomes. The longitudinal coordinate and horizontal ordinate are the odds ratio of mortality and adverse events of each drug to SOC (1.0, 1.0). SOC, standard of care.
Discussion
To the best of our knowledge, this was the first Bayesian network meta-analysis of the efficacy and safety of JAK inhibitors as a potential therapeutic candidate for SARS-CoV-2 specifically. Using JAK inhibitors was associated with a significant reduction in mortality in patients with COVID-19, without clinically meaningful differences in safety from SOC. The present Bayesian network meta-analysis and ranking analysis suggested the best JAK inhibitor in terms of reducing mortality and adverse events for COVID-19 were likely to be ruxolitinib.
In contrast to the four previously published meta-analyses that have summarized published studies of JAK inhibitors (10–13), this research included the latest random control trials and observational studies on the efficacy of JAK inhibitors, and also conducted a systematic pairwise meta-analysis. In the recently published meta-analysis that only included RCTs, the meta-analysis results of mortality were the same as ours, but the subjects of baricitinib included in the study accounted for 97% of the total subjects, so the results of the study were mainly affected by baricitinib. We believe that, as a meta-analysis to discuss the efficacy of JAK inhibitors on COVID-19, the article should increase the research involving other drugs, while ensuring the high quality of the included research. The discussion on safety in this article focused on the occurrence of any grade adverse events or serious adverse events but did not deliberately analyze the occurrence of certain adverse events that deserve attention and were closely related to JAK inhibitors (13). By contrast, our research had a better understanding of treatment-related adverse events in this group of patients.
The safety of JAK inhibitors is a topic of current concern and has significant implications for the treatment of COVID-19. Known adverse events of JAK inhibitors have been described in phase 3 trials with ruxolitinib in myelofibrosis and polycythemia vera (48, 49). Given that JAK2 is essential for the formation of red blood cells and platelets, administration of JAK2 inhibitors induces anemia and low platelet counts. In addition, JAK is involved in immune responses, particularly through interferon-γ, so some infection events, such as hepatitis B infection and reactivation of tuberculosis, are common potential adverse effects (49). Therefore, the thromboembolic and infection risk of JAK inhibitors has been extensively studied and debated since their clinical development (50). In April 2017, the US Food and Drug Administration (FDA) expressed concern about the observed imbalance in thromboembolic events (deep vein thrombosis and pulmonary embolism) in a placebo-controlled clinical trial of baricitinib (51). In September 2021, the FDA issued an updated warning regarding the increased risk of serious heart-related events, cancer, blood clots, and death in JAK inhibitors. As mentioned above, based on the included studies, this study focused on the two most common adverse events, namely thrombotic events and infection. In the study, we found no statistical difference in the incidence of adverse events between the above three JAK inhibitors and SOC. We assumed that it might be related to the shorter course of treatment of JAK inhibitors for COVID-19. As the above FDA warning on adverse events of JAK inhibitors, the drug is usually treated for more than 24 weeks. The warming is based on long-term (up to 72 months) safety data of tofacitinib in patients with rheumatoid arthritis (52). Nevertheless, in the relevant studies we included, most JAK inhibitors have been used only for 7–14 days (53).
Additionally, the mortality and side effects of different JAK inhibitors were compared, specifically in depth by using Bayesian network meta-analysis, which provided a more specific reference for the clinical treatment of COVID-19 patients. Without adding other types of treatment to interfere with the results in this NMA, ruxolitinib is the most likely best treatment, which could significantly reduce all-cause mortality in patients with COVID-19, with no difference in the incidence of adverse events compared with SOC. Although nezulcitinib has also been tried to treat this type of patient, there was only one relevant study and a small number of included people. After the study was included in the NMA, the sensitivity analysis showed that it had a great impact on the original NMA results: the assessment of rank probabilities of the NMA showed that the probability of nezulcitinib being the best treatment was 82.9%, ruxolitinib was 9.52%, baricitinib was 5.74%, and tofacitinib was 1.86% (Supplementary Figure 3), while the rank probabilities assessment results of the original NMA showed that the probability of ruxolitinib being the best treatment was 51.5%, baricitinib was 36.9%, and tofacitinib was 11.5%. Therefore, nezulcitinib was not included in this NMA analysis.
Besides, by comparing the basic characteristics of the included studies, we found that dose of most of the same drugs was consistent. Except for baricitinib, there were four studies (25, 26, 37, 42) that did not provide useful data on dose and one study (29) that used ruxolitinib 5–10 mg daily, which is different from the 10 mg twice a day used in other studies. Therefore, the sensitivity analysis was conducted after the above studies were excluded, and NMA results showed that there was no significant difference between the results before and after exclusion. In terms of efficacy, ruxolitinib still had the largest possibility of the best treatment (58.9% probability) (Supplementary Figure 4), while in terms of safety, three JAK inhibitors had no statistical difference with SOC, and baricitinib was still the best (37.9% probability) (Supplementary Figure 5).
A comprehensive evaluation of different drugs should be made by combining efficacy and safety. In Figure 7, the longitudinal coordinate and horizontal ordinate are odds ratio of mortality and adverse events. Compared with SOC, the treatment with the coordinate point closer to the lower left quadrant in the figure is more likely to be the best treatment. It is true that ruxolitinib and baricitinib are obviously better than tofacitinib in terms of efficacy, but the comparison between them is not obvious. Similar conclusions can also be found through the Bayesian network meta-analysis rank possibility assessment. Among the three drugs, ruxolitinib is the most likely one (51.5%), baricitinib is the second most likely (36.9%), and tofacitinib is the least likely (11.5%). At the same time, it can be found in Figure 7 that there is no significant difference between the three JAK inhibitors and SOC in causing drug-related adverse events in patients. Therefore, based on the comparison between the efficacy and safety of the three drugs, we believe that ruxolitinib is most likely to be the best treatment, while baricitinib can be used in clinical practice as an alternative to ruxolitinib.
The experience with severe SARS-CoV-1 points to a major feature of these infections namely delayed cytokine storm after initial induction and insufficient type I interferon (IFN-I) action (54). The same situation is observed in SARS-CoV-2. During SARS-CoV-2 infection, it induces biphasic disease, first, a flu-like stage, then pulmonary and systemic disease, followed by a potentially suppressed or delayed innate immune response, followed by an emergency signal that may be triggered by sustained viral replication. This could eventually lead to cytokine storms that lead to severe evolution of COVID-19, possibly leading to acute respiratory distress syndrome (ARDS) (13). JAK inhibitors have recently been used to treat inflammatory diseases, such as moderate to severe rheumatoid arthritis and psoriatic arthritis. These disorders are characterized by abnormal activation of the JAK-STAT signaling pathway, which plays a key role in the coordination of immune system responses (55). In addition, JAK/STAT signaling cascades are involved in the control of cell proliferation and survival (56). Therefore, JAK inhibitors hold the potential to cut - off pathological reactions in COVID-19.
Compared with other COVID-19 treatment candidates, all JAK inhibitors have the advantage of being taken orally or inhaled directly (nezulcitinib) and have favorable pharmacokinetic characteristics: short half-life; low plasma protein binding, and minimal interference with Cytochrome P450-mediated biotransformation pathways. JAK inhibitors are mostly eliminated by the kidneys as complete drugs, and partial or slight liver metabolism occurs (57).
However, different JAK inhibitors still have different mechanisms. Ruxolitinib inhibits JAK1 and JAK2 by terminating kinase activity, thus preventing STAT activation and nuclear translocation. Baricitinib is a kinase inhibitor that competes with adenosine triphosphate to efficiently and reversibly inhibit JAK1 and JAK2. Tofacitinib inhibits mainly JAK1 and JAK3 and to a lesser range the JAK2. The inhibition of JAK, especially JAK1 and JAK3, can block the signaling of multiple interleukins, thus reducing inflammatory cascade (55). The differences in the mechanisms of the three drugs may be the main reason for the differences in clinical outcomes. Besides, it has been reported in the previous literature that among the JAK inhibitors, baricitinib shows a particularly high affinity for AP-2 Associated Kinase 1 (AAK1) and is the only drug that can effectively inhibit AAK1 and Cyclin G-Associated Kinase at therapeutic concentrations. Ruxolitinib also shows a relatively high affinity for AKK1, but only tofacitinib does not show significant inhibition on AAK1 (58).
At present, the timing of JAK inhibitor use is also considered to be the key to the success of treatment. Winthrop have proposed a clinical staging system consisting of three COVID-19 stages in terms of illness development (59). The third stage is marked by severe extrapulmonary systemic hyperinflammation syndrome, ARDS, systemic inflammatory response syndrome, and impending multi-organ failure. It is believed that JAK inhibitors should be considered before multi-organ dysfunction. However, there is still no standard for the classification of disease development. Most of the included studies involved patients classified as moderate or severe according to the oxygenation index. The previous relevant meta-analysis also included subgroups classified by other patient severity scores. Current studies are insufficient to perform a network meta-analysis of study outcomes in patients with different disease severity receiving JAK inhibitors. Perhaps there will be differences in the efficacy of different drugs in patients of different severity.
There are some limitations to this research. First, to ensure the integrity of the included studies, in addition to RCTs, this study also included relevant high-quality observational studies. Although the evidence value of such observational studies is low, it is believed that the observational studies that have passed the screening nonetheless have high reference values. Second, the current JAK inhibitor-related network evidence map shows that the baricitinib-related studies account for the majority of all JAK inhibitors studies (14/28), hence also the majority of subjects were treated with that drug. However, because the data leading to the conclusion of better efficacy with ruxolitinib were drawn from relatively few studies, which may have affected the results of this network analysis, more high-quality RCTs on ruxolitinib are looked forward to. Finally, our analysis compares only the efficacy of the drug components but was not designed to consider specific drug doses. At present, most of JAK inhibitors used to treat COVID-19 use their own conventional treatment doses. We hoped that relevant RCT results can be published in the future. In addition, we have collected the ongoing clinical studies of related drugs (Supplementary Table 1), which will be tracked by us continuously.
Conclusion
JAK inhibitors appear to be a promising treatment for reducing mortality from COVID-19 and do not appear to increase the risk of adverse events. This network meta-analysis suggests that mortality benefits are associated with JAK inhibitors, and among these, ruxolitinib presents the greatest likelihood of having benefits for mortality and adverse events.
Data availability statement
The original contributions presented in this study are included in the article/Supplementary material, further inquiries can be directed to the corresponding authors.
Author contributions
RC, LZ, and LG contributed to determining the outline and content of the pairwise and Bayesian network meta-analysis. JN and ZL contributed to retrieving literature. JN, ZL, and ZH contributed to writing a draft of this manuscript. All authors contributed to revising the draft critically for important intellectual content, providing final confirmation of the revised version, being responsible for all aspects of the work, and read and approved the final manuscript.
Funding
This work was supported by the State Key Laboratory of Respiratory Disease (grant no. SKLRD-Z-202203), the Basic Research Program of Guangzhou (grant no. 202102010224), the Clinical Transformation Program of the First Affiliated Hospital of Guangzhou Medical University (grant nos. ZH201802 and ZH201914), the High-level University Program of Guangzhou Medical University (grant no. 2017[160]), and the Opening Project of State Key Laboratory of Respiratory Disease (grant no. SKLRD-0P-202115).
Acknowledgments
We thank John Daniel from Liwen Bianji (Edanz) (www.liwenbianji.cn) for editing the English text of a draft of this manuscript.
Conflict of interest
The authors declare that the research was conducted in the absence of any commercial or financial relationships that could be construed as a potential conflict of interest.
Publisher’s note
All claims expressed in this article are solely those of the authors and do not necessarily represent those of their affiliated organizations, or those of the publisher, the editors and the reviewers. Any product that may be evaluated in this article, or claim that may be made by its manufacturer, is not guaranteed or endorsed by the publisher.
Supplementary material
The Supplementary Material for this article can be found online at: https://www.frontiersin.org/articles/10.3389/fmed.2022.973688/full#supplementary-material
Abbreviations
JAK, Janus kinases; COVID-19, coronavirus disease-2019; SARS-CoV, severe acute respiratory syndrome-coronavirus; CRS, cytokine release syndrome; IL, interleukin; STAT, signal transducer and activator of transcription; SOC, standard of care; NMA, network meta-analysis; PRISMA, Preferred Reporting Items for meta-analysis; NOS, Newcastle–Ottawa Scale; RCTs, Randomized clinical trials; OR, odds ratio; 2D, two-dimensional; FDA, Food and Drug Administration; IFN-I, type I interferon (IFN-I); ARDS, acute respiratory distress syndrome.
Footnotes
References
1. Kim JS, Lee JY, Yang JW, Lee KH, Effenberger M, Szpirt W, et al. Immunopathogenesis and treatment of cytokine storm in COVID-19. Theranostics. (2021) 11:316–29. doi: 10.7150/thno.49713
2. Luo W, Li YX, Jiang LJ, Chen Q, Wang T, Ye DW. Targeting JAK-STAT signaling to control cytokine release syndrome in COVID-19. Trends Pharmacol Sci. (2020) 41:531–43. doi: 10.1016/j.tips.2020.06.007
3. Ye Q, Wang B, Mao J. The pathogenesis and treatment of the ‘Cytokine Storm’ in COVID-19. J Infect. (2020) 80:607–13. doi: 10.1016/j.jinf.2020.03.037
4. Doshi PB, Whittle JS, Dungan G II, Volakis LI, Bublewicz M, Kearney J, et al. The ventilatory effect of high velocity nasal insufflation compared to non-invasive positive-pressure ventilation in the treatment of hypercapneic respiratory failure: A subgroup analysis. Heart Lung. (2020) 49:610–5. doi: 10.1016/j.hrtlng.2020.03.008
5. McLornan DP, Pope JE, Gotlib J, Harrison CN. Current and future status of JAK inhibitors. Lancet. (2021) 398:803–16. doi: 10.1016/s0140-6736(21)00438-4
6. Meletiadis J, Tsiodras S, Tsirigotis P. Interleukin-6 Blocking vs. JAK-STAT inhibition for prevention of lung injury in patients with COVID-19. Infect Dis Ther. (2020) 9:707–13. doi: 10.1007/s40121-020-00326-1
7. Huang J, Zhou C, Deng J, Zhou J. JAK inhibition as a new treatment strategy for patients with COVID-19. Biochem Pharmacol. (2022) 202:115162. doi: 10.1016/j.bcp.2022.115162
8. Zhang X, Shang L, Fan G, Gu X, Xu J, Wang Y, et al. The efficacy and safety of janus kinase inhibitors for patients with COVID-19: A living systematic review and meta-analysis. Front Med (Lausanne). (2021) 8:800492. doi: 10.3389/fmed.2021.800492
9. Chen CX, Wang JJ, Li H, Yuan LT, Gale RP, Liang Y. JAK-inhibitors for coronavirus disease-2019 (COVID-19): a meta-analysis. Leukemia. (2021) 35:2616–20. doi: 10.1038/s41375-021-01266-6
10. Limen RY, Sedono R, Sugiarto A, Hariyanto TI. Janus kinase (JAK)-inhibitors and coronavirus disease 2019 (Covid-19) outcomes: a systematic review and meta-analysis. Exp Rev Anti Infect Ther. (2022) 20:425–34. doi: 10.1080/14787210.2021.1982695
11. Kramer A, Prinz C, Fichtner F, Fischer AL, Thieme V, Grundeis F, et al. Janus kinase inhibitors for the treatment of COVID-19. Cochrane Database Syst Rev. (2022) 6:CD015209. doi: 10.1002/14651858.CD015209
12. Xiang M, Wu X, Jing H, Liu L, Wang C, Wang Y, et al. The impact of platelets on pulmonary microcirculation throughout COVID-19 and its persistent activating factors. Front Immunol. (2022) 13:955654. doi: 10.3389/fimmu.2022.955654
13. Levy G, Guglielmelli P, Langmuir P, Constantinescu SN. JAK inhibitors and COVID-19. J Immunother Cancer. (2022) 10:e002838. doi: 10.1136/jitc-2021-002838
14. Moher D, Liberati A, Tetzlaff J, Altman DG, Prisma Group. Preferred reporting items for systematic reviews and meta-analyses: the PRISMA statement. PLoS Med. (2009) 6:e1000097. doi: 10.1371/journal.pmed.1000097
15. Olivo SA, Macedo LG, Gadotti IC, Fuentes J, Stanton T, Magee DJ. Scales to assess the quality of randomized controlled trials: a systematic review. Phys Ther. (2008) 88:156–75. doi: 10.2522/ptj.20070147
16. Lo CK, Mertz D, Loeb M. Newcastle-Ottawa Scale: comparing reviewers’ to authors’ assessments. BMC Med Res Methodol. (2014) 14:45. doi: 10.1186/1471-2288-14-45
17. Oremus M, Wolfson C, Perrault A, Demers L, Momoli F, Moride Y. Interrater reliability of the modified jadad quality scale for systematic reviews of Alzheimer’s Disease drug trials. Dement Geriatr Cogn Disord. (2001) 12:232–6. doi: 10.1159/000051263
18. Margulis AV, Pladevall M, Riera-Guardia N, Varas-Lorenzo C, Hazell L, Berkman ND, et al. Quality assessment of observational studies in a drug-safety systematic review, comparison of two tools: the Newcastle-Ottawa Scale and the RTI item bank. Clin Epidemiol. (2014) 6:359–68. doi: 10.2147/CLEP.S66677
19. Kalil AC, Patterson TF, Mehta AK, Tomashek KM, Wolfe CR, Ghazaryan V, et al. Baricitinib plus remdesivir for hospitalized adults with Covid-19. N Engl J Med. (2021) 384:795–807. doi: 10.1056/NEJMoa2031994
20. Marconi VC, Ramanan AV, de Bono S, Kartman CE, Krishnan V, Liao R, et al. Efficacy and safety of baricitinib for the treatment of hospitalised adults with COVID-19 (COV-BARRIER): a randomised, double-blind, parallel-group, placebo-controlled phase 3 trial. Lancet Respir Med. (2021) 9:1407–18. doi: 10.1016/S2213-2600(21)00331-3
21. Cao Y, Wei J, Zou L, Jiang T, Wang G, Chen L, et al. Ruxolitinib in treatment of severe coronavirus disease 2019 (COVID-19): A multicenter, single-blind, randomized controlled trial. J Allergy Clin Immunol. (2020) 146:137–46. doi: 10.1016/j.jaci.2020.05.019
22. Guimarães PO, Quirk D, Furtado RH, Maia LN, Saraiva JF, Antunes MO, et al. Tofacitinib in Patients Hospitalized with Covid-19 Pneumonia. N Engl J Med. (2021) 385:406–15. doi: 10.1056/NEJMoa2101643
23. Rodriguez-Garcia JL, Sanchez-Nievas G, Arevalo-Serrano J, Garcia-Gomez C, Jimenez-Vizuete JM, Martinez-Alfaro E. Baricitinib improves respiratory function in patients treated with corticosteroids for SARS-CoV-2 pneumonia: an observational cohort study. Rheumatology (Oxford). (2021) 60:399–407. doi: 10.1093/rheumatology/keaa587
24. Bronte V, Ugel S, Tinazzi E, Vella A, De Sanctis F, Canè S, et al. Baricitinib restrains the immune dysregulation in patients with severe COVID-19. J Clin Invest. (2020) 130:6409–16. doi: 10.1172/JCI141772
25. Masia M, Padilla S, Garcia JA, Garcia-Abellan J, Navarro A, Guillen L, et al. Impact of the addition of baricitinib to standard of care including tocilizumab and corticosteroids on mortality and safety in severe COVID-19. Front Med. (2021) 8:749657. doi: 10.3389/fmed.2021.749657
26. Abizanda P, Calbo Mayo JM, Mas Romero M, Cortés Zamora EB, Tabernero Sahuquillo MT, Romero Rizos L, et al. Baricitinib reduces 30-day mortality in older adults with moderate-to-severe COVID-19 pneumonia. J Am Geriatr Soc. (2021) 69:2752–8. doi: 10.1111/jgs.17357
27. D’Alessio A, Del Poggio P, Bracchi F, Cesana G, Sertori N, Di Mauro D, et al. Low-dose ruxolitinib plus steroid in severe SARS-CoV-2 pneumonia. Leukemia. (2021) 35:635–8. doi: 10.1038/s41375-020-01087-z
28. Iastrebner M, Castro J, García Espina E, Lettieri C, Payaslian S, Cuesta MC, et al. Ruxolitinib in severe COVID-19: Results of a multicenter, prospective, single arm, open-label clinical study to investigate the efficacy and safety of ruxolitinib in patients with COVID-19 and severe acute respiratory syndrome. Rev Fac Cien Med Univ Nac Cordoba. (2021) 78:294–302. doi: 10.31053/1853.0605.v78.n3.32800
29. Stanevich OV, Fomina DS, Bakulin IG, Galeev SI, Bakin EA, Belash VA, et al. Ruxolitinib versus dexamethasone in hospitalized adults with COVID-19: multicenter matched cohort study. BMC Infect Dis. (2021) 21:1277. doi: 10.1186/s12879-021-06982-z
30. Giudice V, Pagliano P, Vatrella A, Masullo A, Poto S, Polverino BM, et al. Combination of ruxolitinib and eculizumab for treatment of severe SARS-CoV-2-Related acute respiratory distress syndrome: A controlled study. Front Pharmacol. (2020) 11:857. doi: 10.3389/fphar.2020.00857
31. Maslennikov R, Ivashkin V, Vasilieva E, Chipurik M, Semikova P, Semenets V, et al. Tofacitinib reduces mortality in coronavirus disease 2019 Tofacitinib in COVID-19. Pulm Pharmacol Ther. (2021) 69:102039. doi: 10.1016/j.pupt.2021.102039
32. Pérez-Alba E, Nuzzolo-Shihadeh L, Aguirre-García GM, Espinosa-Mora J, Lecona-Garcia JD, Flores-Pérez RO, et al. Baricitinib plus dexamethasone compared to dexamethasone for the treatment of severe COVID-19 pneumonia: A retrospective analysis. J Microbiol Immunol Infect. (2021) 54:787–93. doi: 10.1016/j.jmii.2021.05.009
33. Rosas J, Liaño FP, Cantó ML, Barea JMC, Beser AR, Rabasa JTA, et al. Experience with the use of baricitinib and tocilizumab monotherapy or combined, in patients with interstitial pneumonia secondary to coronavirus COVID19: A real-world study. Reumatol Clin (Engl Ed). (2020) 28:150–6. doi: 10.1016/j.reuma.2020.10.009
34. Cantini F, Niccoli L, Nannini C, Matarrese D, Natale MED, Lotti P, et al. Beneficial impact of Baricitinib in COVID-19 moderate pneumonia; multicentre study. J Infect. (2020) 81:647–79. doi: 10.1016/j.jinf.2020.06.052
35. Stebbing J, Sánchez Nievas G, Falcone M, Youhanna S, Richardson P, Ottaviani S, et al. JAK inhibition reduces SARS-CoV-2 liver infectivity and modulates inflammatory responses to reduce morbidity and mortality. Sci Adv. (2021) 7:eabe4724. doi: 10.1126/sciadv.abe4724
36. Tziolos N, Karofylakis E, Grigoropoulos I, Kazakou P, Koullias E, Savva A, et al. Real-Life effectiveness and safety of baricitinib as adjunctive to standard-of-care treatment in hospitalized patients with Severe Coronavirus Disease 2019. Open Forum Infect Dis. (2021) 9:ofab588. doi: 10.1093/ofid/ofab588
37. Falcone M, Tiseo G, Barbieri G, Galfo V, Russo A, Virdis A, et al. Role of low-molecular-weight heparin in hospitalized patients with severe acute respiratory syndrome coronavirus 2 Pneumonia: A prospective observational study. Open Forum Infect Dis. (2020) 7:ofaa563. doi: 10.1093/ofid/ofaa563
38. Hayek ME, Mansour M, Ndetan H, Burkes Q, Corkern R, Dulli A, et al. Anti-Inflammatory treatment of COVID-19 Pneumonia with tofacitinib alone or in combination with dexamethasone is safe and possibly superior to dexamethasone as a single agent in a predominantly african american cohort. Mayo Clin Proc Innov Qual Outcomes. (2021) 5:605–13. doi: 10.1016/j.mayocpiqo.2021.03.007
39. Singh PK, Lalwani LK, Govindagoudar MB, Aggarwal R, Chaudhry D, Kumar P, et al. Tofacitinib associated with reduced intubation rates in the management of severe COVID-19 pneumonia: A preliminary experience. Indian J Crit Care Med. (2021) 25:1108–12. doi: 10.5005/jp-journals-10071-23964
40. Incyte Corporation. Assessment of efficacy and safety of ruxolitinib in participants with COVID-19-Associated ARDS Who Require Mechanical Ventilation (RUXCOVID-DEVENT).ClinicalTrials.gov.NCT04377620. (2021) Wilmington: Incyte Corporation.
41. Melikhov O, Kruglova T, Lytkina K, Melkonyan G, Prokhorovich E, Putsman G, et al. Use of Janus kinase inhibitors in COVID-19: a prospective observational series in 522 individuals. Ann Rheum Dis. (2021) 80:1245–6. doi: 10.1136/annrheumdis-2021-220049
42. Kojima Y, Nakakubo S, Takei N, Kamada K, Yamashita Y, Nakamura J, et al. Comparative efficacy of tocilizumab and baricitinib administration in COVID-19 treatment: A retrospective cohort study. Medicina (Kaunas). (2022) 58:513. doi: 10.3390/medicina58040513
43. Wolfe CR, Tomashek KM, Patterson TF, Gomez CA, Marconi VC, Jain MK, et al. Baricitinib versus dexamethasone for adults hospitalised with COVID-19 (ACTT-4): a randomised, double-blind, double placebo-controlled trial. Lancet Respir Med. (2022) 10:888–99. doi: 10.1016/S2213-2600(22)00088-1
44. Han MK, Antila M, Ficker JH, Gordeev I, Guerreros A, Bernus AL, et al. Ruxolitinib in addition to standard of care for the treatment of patients admitted to hospital with COVID-19 (RUXCOVID): a randomised, double-blind, placebo-controlled, phase 3 trial. Lancet Rheumatol. (2022) 4:e351–61. doi: 10.1016/S2665-9913(22)00044-3
45. Ely EW, Ramanan AV, Kartman CE, de Bono S, Liao R, Piruzeli MLB, et al. Efficacy and safety of baricitinib plus standard of care for the treatment of critically ill hospitalised adults with COVID-19 on invasive mechanical ventilation or extracorporeal membrane oxygenation: an exploratory, randomised, placebo-controlled trial. Lancet Respir Med. (2022) 10:327–36. doi: 10.1016/S2213-2600(22)00006-6
46. Singh D, Bogus M, Moskalenko V, Lord R, Moran EJ, Crater GD, et al. A phase 2 multiple ascending dose study of the inhaled pan-JAK inhibitor nezulcitinib (TD-0903) in severe COVID-19. Eur Respir J. (2021) 58:2100673. doi: 10.1183/13993003.00673-2021
47. Mavridis D, Salanti G. A practical introduction to multivariate meta-analysis. Stat Methods Med Res. (2013) 22:133–58. doi: 10.1177/0962280211432219
48. Harrison C, Kiladjian JJ, Al-Ali HK, Gisslinger H, Waltzman R, Stalbovskaya V, et al. JAK inhibition with ruxolitinib versus best available therapy for myelofibrosis. N Engl J Med. (2012) 366:787–98. doi: 10.1056/NEJMoa1110556
49. Vannucchi AM, Kiladjian JJ, Griesshammer M, Masszi T, Durrant S, Passamonti F, et al. Ruxolitinib versus standard therapy for the treatment of polycythemia vera. N Engl J Med. (2015) 372:426–35. doi: 10.1056/NEJMoa1409002
50. Mehta P, Ciurtin C, Scully M, Levi M, Chambers RC. JAK inhibitors in COVID-19: the need for vigilance regarding increased inherent thrombotic risk. Eur Respir J. (2020) 56:2001919. doi: 10.1183/13993003.01919-2020
51. Scott IC, Hider SL, Scott DL. Thromboembolism with Janus Kinase (JAK) inhibitors for rheumatoid arthritis: How real is the risk? Drug Saf. (2018) 41:645–53. doi: 10.1007/s40264-018-0651-5
52. Ytterberg SR, Bhatt DL, Mikuls TR, Koch GG, Fleischmann R, Rivas JL, et al. ORAL surveillance investigators. Cardiovascular and cancer risk with tofacitinib in rheumatoid arthritis. N Engl J Med. (2022) 386:316–26. doi: 10.1056/NEJMoa2109927
53. Florescu DF, Kalil AC. Janus Kinase inhibitors for the treatment of hospitalized patients with COVID-19. Curr Opin Crit Care. (2021) 27:493–6. doi: 10.1097/MCC.0000000000000869
54. Channappanavar R, Fehr AR, Zheng J, Wohlford-Lenane C, Abrahante JE, Mack M, et al. IFN-I response timing relative to virus replication determines MERS coronavirus infection outcomes. J Clin Invest. (2019) 129:3625–39. doi: 10.1172/JCI126363
55. Spinelli FR, Meylan F, O’Shea JJ, Gadina M. JAK inhibitors: Ten years after. Eur J Immunol. (2021) 51:1615–27. doi: 10.1002/eji.202048922
56. Lin CM, Cooles FA, Isaacs JD. Basic Mechanisms of JAK Inhibition. Mediterr J Rheumatol. (2020) 31(Suppl 1):100–4. doi: 10.31138/mjr.31.1.100
57. Veeravalli V, Dash RP, Thomas JA, Babu RJ, Madgula LMV, Srinivas NR. Critical assessment of pharmacokinetic drug-drug interaction potential of tofacitinib, baricitinib and upadacitinib, the three approved janus kinase inhibitors for rheumatoid arthritis treatment. Drug Saf. (2020) 43:711–25. doi: 10.1007/s40264-020-00938-z
58. Stebbing J, Phelan A, Griffin I, Tucker C, Oechsle O, Smith D, et al. COVID-19: combining antiviral and anti-inflammatory treatments. Lancet Infect Dis. (2020) 20:400–2. doi: 10.1016/S1473-3099(20)30132-8
Keywords: COVID-19, JAK inhibitors, mortality, adverse events, network meta-analysis
Citation: Niu J, Lin Z, He Z, Yang X, Qin L, Feng S, Guan L, Zhou L and Chen R (2022) Janus kinases inhibitors for coronavirus disease-2019: A pairwise and Bayesian network meta-analysis. Front. Med. 9:973688. doi: 10.3389/fmed.2022.973688
Received: 20 June 2022; Accepted: 03 November 2022;
Published: 23 November 2022.
Edited by:
Reza Lashgari, Shahid Beheshti University, IranReviewed by:
Pin-Kuei Fu, Taichung Veterans General Hospital, TaiwanAnnika Åstrand, AstraZeneca, Sweden
Ralph Budd, University of Vermont, United States
Copyright © 2022 Niu, Lin, He, Yang, Qin, Feng, Guan, Zhou and Chen. This is an open-access article distributed under the terms of the Creative Commons Attribution License (CC BY). The use, distribution or reproduction in other forums is permitted, provided the original author(s) and the copyright owner(s) are credited and that the original publication in this journal is cited, in accordance with accepted academic practice. No use, distribution or reproduction is permitted which does not comply with these terms.
*Correspondence: Rongchang Chen, Y2hlbnJjQHZpcC4xNjMuY29t; Luqian Zhou, emhseDA5QDE2My5jb20=; Lili Guan, ZHJfbmlja2d1YW5AMTYzLmNvbQ==
†These authors have contributed equally to this work