- 1Department of Medical Psychology and Ethics, School of Basic Medical Sciences, Cheeloo College of Medicine, Shandong University, Jinan, China
- 2Department of Geriatric Medicine, Tan Tock Seng Hospital, Singapore, Singapore
- 3Lee Kong Chian School of Medicine, Nanyang Technological University, Singapore, Singapore
- 4The Third Hospital of Jinan, Jinan, China
- 5Gerontology Research Programme, Department of Psychological Medicine, National University Health System, Yong Loo Lin School of Medicine, National University of Singapore, Singapore, Singapore
- 6Department of Medicine, Research Center on Aging, University of Sherbrooke, Sherbrooke, QC, Canada
- 7Department of Endocrinology, Khoo Teck Puat Hospital, Singapore, Singapore
- 8Biology of Ageing Laboratory, Singapore Immunology Network (SIgN), Agency for Science Technology and Research (A*STAR), Biopolis, Singapore, Singapore
Background: Sarcopenia is common among older individuals with and without type 2 diabetes mellitus (T2DM). There are conflicting evidence in support of the role of insulin in the development of age-related and T2DM-related sarcopenia. We investigated the relationships between the levels of fasting insulin and other blood biomarkers related to insulin or lipid metabolism with the presence of sarcopenia in two independent studies.
Materials and methods: In 246 pre-frail frail older individuals with (n = 41) and without T2DM (n = 205) in the Singapore Frailty Interventional Trial, sarcopenia was defined by low appendicular lean mass (ALM) relative to total body mass (skeletal muscle index, SMI = ALM/height2) and low lower limb strength or gait speed according to the Asian Working Group for Sarcopenia (AWGS) criteria released in 2019, and related to levels of fasting insulin and glucose, C-peptide, IGF-1, leptin, and active ghrelin. This investigation was validated in another independent study sample of 189 robust and pre-frail frail elderly in the Singapore Longitudinal Aging Study Wave 2 (SLAS-2).
Results: Compared to non-sarcopenic individuals, those with sarcopenia and possible sarcopenia showed significantly lower fasting insulin (p < 0.05) in pre-frail/frail and non-frail older individuals. Consistent trends of relationships were observed for serum levels of C-peptide, IGF-1, leptin, and active ghrelin. In multivariable logistic regression models, sarcopenia was independently associated with low insulin (p < 0.05). Levels of fasting insulin, C-peptide, and leptin were also significantly associated with BMI, SMI, knee extension strength, gait speed, and physical activity score.
Conclusion: Dysregulated insulin secretion in diabetic and non-diabetic older individuals may play an important role in age-related and diabetes-related sarcopenia.
Introduction
Sarcopenia is an incipient manifestation of aging characterized by progressive loss of skeletal muscle mass and function. It is reported that sarcopenia predicts physical frailty, late-life disability, and mortality (1, 2). In a world with an accelerated population aging, the rapidly growing number of older individuals with sarcopenia and physical frailty makes it an important geriatric syndrome in clinical care. The prevalence of sarcopenia is 8–36% worldwide in individuals below 60 years and 10–27% in those equal to or above 60 years. Population-based prevalence of frailty varies by age, gender, and frailty classification, with 12% using physical frailty and 24% using the deficit accumulation model among those aged ≥50 years across 62 countries (3, 4). The underlying pathophysiology of sarcopenia is complex and far from being fully understood, involving nutritional deficiency, reduced physical activity, insulin resistance, atherosclerosis, and changes in inflammatory and endocrine functions (5, 6).
Disordered β cell functioning and insulin resistance are hallmarks of the development of type 2 diabetes mellitus (T2DM), which is prominently associated with sarcopenia (7). The association of insulin level and muscle mass and function decline in old age and those with T2DM is complex. Based on the classical view that insulin resistance is central to the development of T2DM, there are contrary hypotheses that insulin resistance facilitates the development of sarcopenia (8) and conversely that sarcopenia is a risk factor for insulin resistance and T2DM (9). Increasing evidence strongly points to disordered insulin secretion rather than insulin resistance playing a central role in driving the development of T2DM (10–12). Patients with diabetes may be vulnerable to sarcopenia due to primary changes in insulin level and activity (9, 13, 14). As an anabolic hormone, insulin stimulates protein synthesis via the uptake of amino acids into muscle tissues. Reduced insulin signaling in aging and diabetes hinders muscle protein synthesis (MPS) and promotes muscle protein degradation, leading to loss of muscle mass and eventually sarcopenia. Sarcopenia in turn further decreases the targeting mass of insulin action, reduces insulin sensitivity, and induces glucose dysregulation (15, 16).
Empirical evidence suggests the critical role of sufficient insulin in promoting protein synthesis, maintaining muscle function, and preventing muscle mass loss and sarcopenia (17, 18). Insulin exerts its anabolic action on skeletal muscles via stimulating glucose disposal (19) and inhibiting protein catabolism (20). A meta-analysis of human studies demonstrates that insulin has a permissive role in MPS in the presence of elevated amino acids and a major anti-catabolic effect in alleviating muscle protein breakdown (MPB) (21). In an interventional study, insulin treatmentattenuated annual decline of skeletal muscle index (SMI), especially in the lower extremities in Japanese patients with T2DM (22). There is an age-related decrease in insulin-mediated peripheral glucose utilization (23) and suppression of proteolysis (24) that are associated with sarcopenia. However, few studies have yet investigated the association of insulin and sarcopenia in older individuals with T2DM and those without (25, 26), with conflicting results generated. In the Baltimore Longitudinal Study of Aging, higher fasting and oral glucose tolerance test (OGTT) levels of insulin were associated with lower muscle mass in non-diabetic older individuals (27). On the other hand, Tanaka et al. (28), in a study of 191 male elderly with T2DM, suggested that endogenous insulin reduction is an independent risk factor of sarcopenia. Furthermore, supraphysiological hyperinsulinemia was reported to play an important role in the stimulation of MPS and anabolic signaling in the elderly (29). A possible explanation of the divergences may be related to the different ways of lean mass measurement (30). In individuals with sarcopenia, appendicular muscle mass index defined as muscle mass relative to height is mostly found positively associated with insulin resistance, while this correlation is negative in terms of muscle mass relative to body weight (31). Thus it is imperative to the standardized criteria to classify sarcopenia, in order to draw clear conclusion.
The present study aimed to ascertain whether sarcopenia is associated with the level of fasting insulin and other biomarkers related to insulin or lipid metabolism in diabetic and non-diabetic older individuals. Sarcopenia and physical frailty share core features such as impaired physical function, especially mobility, high prevalence, and intimate association with adverse health-related outcomes including disability and mortality in the elderly, and potential reversibility, and thus have been studied in parallel since the beginning (32, 33). We therefore studied the relationship of sarcopenia and fasting insulin level in a group of community-living pre-frail frail older persons. Furthermore, we validated and extended the results derived from this group to another independent study of elderly consisting of both robust and pre-frail frail elderly. We hypothesized that older individuals with sarcopenia may have lower levels of fasting insulin than those without sarcopenia, considering the anabolic role of insulin in maintaining muscle mass and function. The present study investigated for the first time, to the best of our knowledge, the association of sarcopenia and fasting insulin level in elderly with and without T2DM, and thus extend the findings from previous research through its broader relevance to community-living elderly without T2DM.
Materials and methods
Study design and participants
Participant recruitment
The subjects in the exploratory study were participants in the Singapore Frailty Interventional Trial, a randomized controlled trial of nutritional, physical, and cognitive interventions among community-living pre-frail frail older persons (clinicaltrial.gov identifier NCT00973258) (34). We used the baseline data from the participants who were 246 pre-frail or frail older individuals (aged 65 years and above) as determined from the Fried criteria for frailty syndrome (35) comprising of 5 components (unintentional weight loss, slowness, weakness, exhaustion, and physical inactivity). We validated the study in an independent validation sample of 189 robust and pre-frail frail elderly from Singapore Longitudinal Aging Study Wave 2 (SLAS-2) (36). The difference between the exploratory study and the validation study lies in that the exploratory study emphasizes 246 pre-frail or frail older individuals, and the validation study covers the full spectrum of 189 robust and pre-frail frail elderly. The participants from both studies were from the same Chinese Singaporean population.
Study design
In both studies, sarcopenia was determined from measures of muscle mass, strength, and function using dual-energy x-ray absorptiometry (DXA) whole body scan, Physiological Profile Assessment (PPA) (37), and the 6-meter fast gait speed test. Serum (in the exploratory study) or plasma (in the validation study) was isolated from blood samples and stored in −80°C until measurements. The studies were approved by National Health Group (NHG) Domain Specific Review Board (DSRB) of Singapore, and all participants provided written informed consent. All methods were performed in accordance with the approved protocol and relevant guidelines and regulations.
Settings
Community-living Singaporean Chinese.
Participant inclusion/exclusion criteria
Inclusion criteria for both studies: (1) community residents in the southwest region of Singapore; (2) aged 65 years and above; (3) able to ambulate without personal assistance; (4) living at home. Exclusion criteria: (1) significant cognitive impairment (Mini Mental State Examination score ≤ 23); (2) major depression; (3) severe audiovisual impairment; (4) any progressive, degenerative neurologic disease; (5) terminal illness with life expectancy < 12 months. Additional inclusion criteria for the exploratory study: (1) prefrail and frail older adults identified based on Fried criteria defining physical frailty. Additional exclusion criteria for the exploratory study: (1) simultaneous participation in other interventional studies; (2) unavailable to participate for the full duration of the study. The recruitment procedures are summarized in Figures 1, 2, respectively, for the exploratory study and the validation study.
Frailty measurements
Frailty was assessed by the presence of: (1) Involuntary or unintentional weight loss: body mass index (BMI) < 18.5 kg/m2 and/or unintentional weight loss > 10 pounds (4.5 kg) in the past 6 months; (2) Slowness: sex and height stratified lowest quintile values from the average of two measurements of the 6 m fast gait speed test; (3) Weakness: dominant knee extension strength measured from the average value from three trials using Lord’s strap and strain gauge assembly component of the PPA, falling within the lowest sex and BMI standardized quintile; (4) Exhaustion: exhaustion score was generated from the vitality domain in the SF-12 and those falling within the lowest quintile of energy score [below 10 out of 15, derived in a previous population-based study of frailty (38)] were classified as having exhaustion; (5) Physical inactivity: Physical activity was assessed by the Longitudinal Ageing Physical Activity Questionnaire (LAPAQ) which measured the frequency and duration (in minutes) of six different activities (walking outside, bicycling, gardening, light and heavy household activities, and sports activities) during the past 2 weeks. A participant was considered physically inactive if his/her overall average daily time spent on physical activities fell within the sex-specific lowest quintile. A participant with three and more components was determined to be frail, one or two components as pre-frail, and none of the components as robust.
Dual-energy x-ray absorptiometry scans of body composition
Total and regional lean body mass and body fat was measured by dual energy X-ray absorptiometry (DXA) with the use of Hologic® densitometer. Tests were performed by operators in accordance with the manufacturer’s protocol in Department of Diagnostic Radiology, National University Hospital of Singapore. The participant was instructed to lay in a supine position on the DXA table with limbs close to the body. The whole-body lean soft tissue mass was divided into the regions of arms, legs, and the trunk. Appendicular lean mass (ALM) was calculated by summing lean mass (kg) of the two upper limbs and two lower limbs, with the adjustment of limb cut lines according to specific anatomical landmarks as described by Heymsfield et al. (39).
Measurement of lower limb strength
Lower limb strength was assessed by knee extension strength using the strap and strain gauge assembly component of the PPA described by Lord et al. (37), and a mean value from three trials (standardized by sex and BMI) was calculated. Low lower limb strength was classified as less than or equal to 18 kg for men and less than or equal to 16 kg for women.
Gait speed test
The 6 m fast gait speed test was performed following standardized procedures described by Nelson et al. (40). Briefly, participants were instructed to stand with their toes touching the taped start line, and complete the 6 m walking as fast as possible. Total course time was recorded using a second watch from the moment their foot crossed the start line until the moment their foot crossed the stop line. The average speed of two trials was calculated and presented as meters/second (m/s).
Definition of sarcopenia
Sarcopenia was identified in accordance with the recommendations from the Asian Working Group for Sarcopenia (AWGS) criteria released in 2019 (41). SMI was calculated as the ratio of ALM assessed by DXA scan over height squared. Participants were considered to have low SMI if the ratio was <7.0 kg/m2 in men or <5.4 kg/m2 in women. Low gait speed was defined as the average speed of two trials < 1.0 m/s. Participants were categorized as having sarcopenia if they had both low SMI and low lower limb strength or gait speed, possible sarcopenia if they had either low lower limb strength or gait speed but not low SMI, and non-sarcopenia if they had neither low SMI nor low lower limb strength or gait speed.
Diabetes status
The presence of diabetes was ascertained by the subjects’ self-reports of a doctor’s diagnosis and treatment, with additional examination of their medication packages. Participants were determined as having diabetes when the use of appropriate diabetes medications was verified by the interviewer. The cross tables of number of participants with different status of sarcopenia and diabetes are summarized below:
Exploratory study:
No diabetes | Diabetes | |
Non-sarcopenia | 25 | 3 |
Possible sarcopenia | 98 | 21 |
Sarcopenia | 82 | 17 |
Validation study:
No diabetes | Diabetes | |
Non-sarcopenia | 28 | 4 |
Possible sarcopenia | 49 | 15 |
Sarcopenia | 79 | 14 |
Blood biomarkers
Venous blood was drawn from overnight fasting participants and serum (in the exploratory study) or plasma (in the validation study) was isolated and stored in –80°C freezer until measurements. The levels of fasting insulin, C-peptide, leptin, and active ghrelin were tested using high throughput Luminex technology (Cat no.: HMHEMAG-34K-04 and HIGFMAG-52K-01; Millipore Corp., Billerica, MA, United States) following the manufacturers’ instructions. Experiments were performed by the platform operators. After an overnight incubation, the plates were read on a Flexmap 3D instrument (Luminex Corporation, Austin, TX, United States) and data were analyzed using Bioplex Manager 6.0 software (Bio-Rad Laboratories, Hercules, CA, United States). The concentration of IGF-1 was measured using Milliplex Map Human IGFI-II Magnetic Bead Panel (Cat no.: HIGFMAG-52K-01) in the exploratory sample and an enzyme-linked immunosorbent assay (ELISA) kit from Raybiotech Inc. (Norcross, GA, USA; Cat no.: ELH-IGF1) in the validation sample. The level of fasting glucose was measured by Department of Laboratory Medicine, National University Hospital of Singapore.
Statistical analysis
Data analysis was performed using IBM SPSS 22 software (IBM, New York, NY, USA). We compared the differences in demographics, physical and functional status, and biomarkers among the sarcopenia, possible sarcopenia, and non-sarcopenia study participants using chi-squared test or one-way analysis of variance (ANOVA) with post-hoc Bonferroni correction for multiple comparisons as appropriate, in the whole group and among non-diabetic and diabetic participants separately. Logistic regressions were performed on each blood biomarker to estimate odds ratio of association with the presence of sarcopenia. Blood biomarkers were analyzed as continuous variables as well as in tertiles, with adjustment for age, sex, diabetes status, and percentage of whole body fat mass to control for potential confounding. P for trends across tertiles were calculated by assigning ordinal scores to the tertiles and repeating the logistic regressions. Linear regression models were used to examine the relations of each blood biomarker concentration to clinical and functional measures of sarcopenia and frailty (BMI, SMI, lower limb strength, exhaustion score, gait speed, and physical activity score) in the whole and stratified samples of non-diabetic and diabetic participants after adjusting for potential confounders. The level of statistical significance was set at p < 0.05 with a two-sided distribution.
Results
The 246 pre-frail frail study participants in the exploratory study and the 189 robust and pre-frail frail elderly in the validation study were all Chinese and had average ages of 70.0 years (SD: 4.7 years) and 73.2 years (SD: 5.3 years), respectively. The exploratory and validatory samples had similar sex proportions, with 151 (61.4%) and 119 (63.0%) females, respectively. In the exploratory study, there were 41 (16.7%) elderly who had diabetes and 205 (83.3%) who did not have diabetes. The validatory study sample had a similar proportion of participants who had diabetes [33 (17.5%)]. Participants with diabetes had a mean disease history of 10 and 12 years, respectively, in the exploratory and validation studies, respectively, with all but five and four, respectively, taking anti-diabetic medications regularly. The commonest medication used was simvastatin and metformin, respectively. None of them were on insulin sensitizer drugs or treated with insulin.
In both studies, participants with sarcopenia had significantly lower BMI, SMI, knee extension strength, and gait speed in the whole sample and among subgroups of non-diabetic and diabetic individuals. Sarcopenia was associated with significantly higher exhaustion in the validation sample in the whole sample and among the subgroups (Table 1).
As shown in Table 2, sarcopenia was associated with lower fasting insulin (p < 0.05) in comparison to non-sarcopenia and possible sarcopenia in the whole exploratory sample of pre-frail/frail elderly. Among participants without diabetes, there was a positive trend of association between sarcopenia with lower fasting insulin, but this did not reach statistical significance, possibly due to the relatively large standard deviation (p = 0.055). In the validation study sample of robust and pre-frail/frail elderly, sarcopenic elderly had significantly lower insulin level than the non-sarcopenia and possible sarcopenia elderly in the whole sample and the non-diabetic group (p < 0.001). There was no difference in insulin levels among the sarcopenia subgroups of diabetic elderly, given the small sample size of the sarcopenic group. Lower C-peptide was observed in the sarcopenia group than in the non-sarcopenia and possible sarcopenia groups in the whole sample and the non-diabetic group. Sarcopenia was associated with lower leptin level than the non-sarcopenia group. No difference was observed in serum or plasma levels of fasting glucose, IGF-1 or active ghrelin among the sarcopenic subgroups (Supplementary Tables 1, 2).
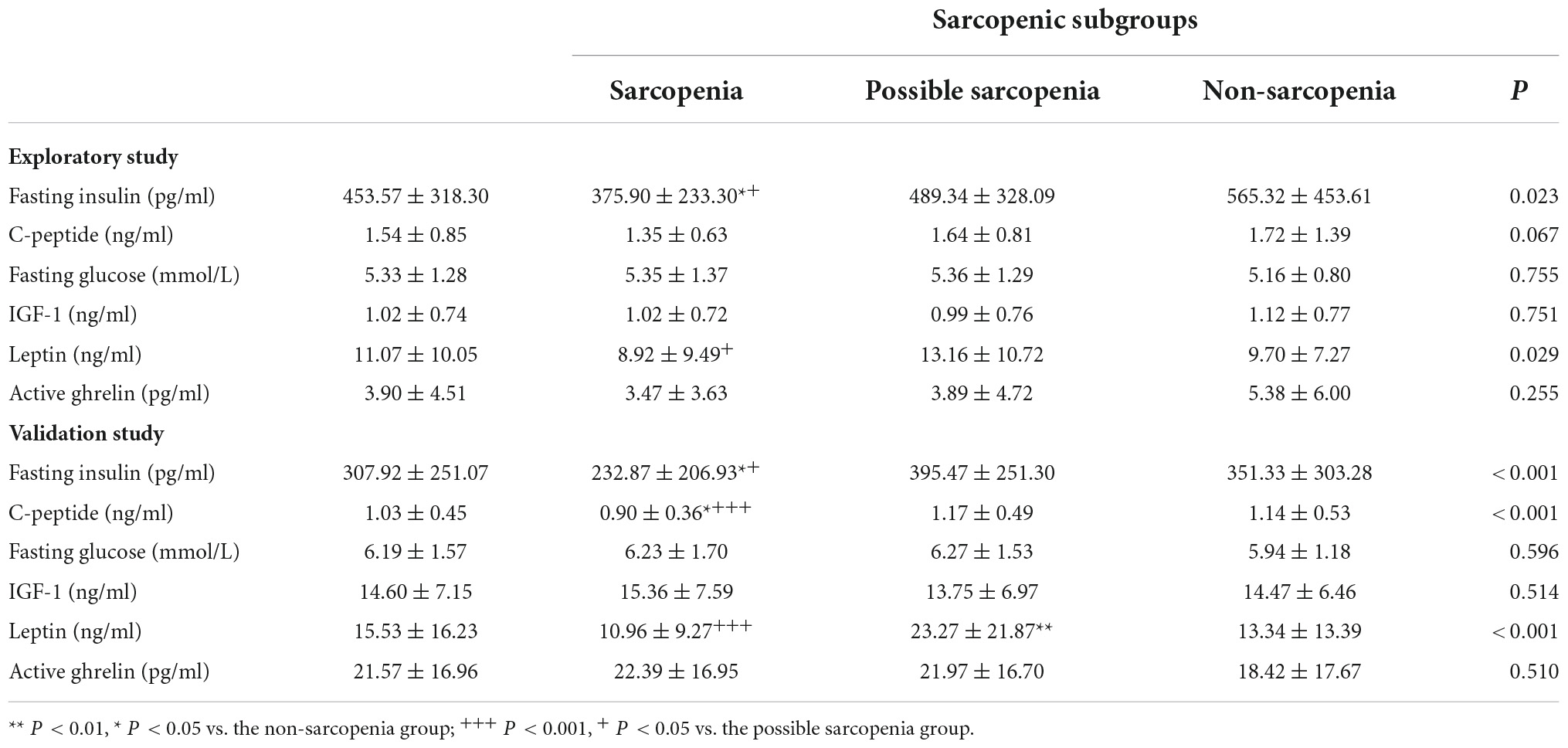
Table 2. Blood biomarker concentrations of sarcopenia groups in frailty intervention trial (FIT) study subjects and Singapore longitudinal ageing studies (SLAS) subjects.
Table 3 shows the results of logistic regression analyses of the association of blood biomarkers with the presence of sarcopenia that were adjusted for age, sex, and diabetes status. The levels of insulin were borderlinely or significantly inversely associated with the presence of sarcopenia in the exploratory sample and the validation sample. The top tertile of fasting insulin was associated with lower risk of sarcopenia relative to the bottom tertile, with an OR of 0.297. A similar trend was observed in the validation study sample.
In linear regression models (Table 4), we found significant independent associations of blood biomarker concentrations with clinical and functional measures of sarcopenia and frailty. In the whole sample, the levels of fasting insulin and C-peptide were positively associated with BMI, SMI, and knee extension strength. IGF-1 was positively associated with knee extension strength. We also found that the level of leptin was positively associated with BMI, SMI, and physical activity score. Similar trends were observed in both non-diabetic and diabetic subgroups as shown in Supplementary Tables 3, 4, respectively.
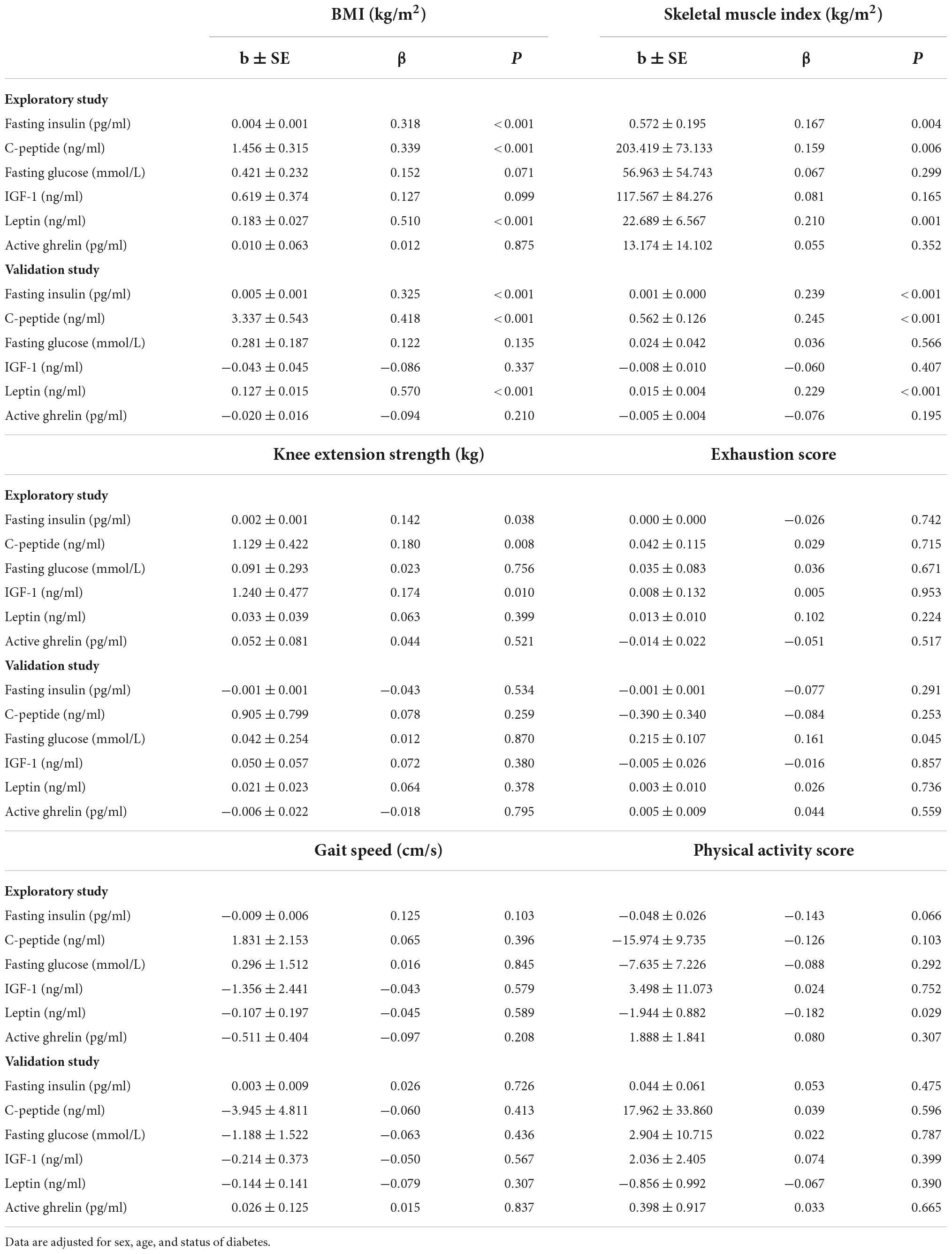
Table 4. Relations of blood biomarker concentrations to clinical and functional measures of sarcopenia and frailty.
Discussion
This study shows for the first time that the association of sarcopenia with low fasting insulin in the elderly is independent of whether the subjects have diabetes or not. Furthermore, fasting insulin, C-peptide, and leptin were significantly associated with one or more clinical and functional measures of sarcopenia and frailty including total body mass, muscle mass, knee extension strength, gait speed, and physical activity. The results are consistent with studies which showed that measures of endogenous insulin secretion were inversely associated with the presence of sarcopenia in individuals with type 2 diabetes (28) and that insulin treatment attenuates skeletal muscle mass loss (21, 22). Our results suggest that insulin is clearly involved in the biological process of sarcopenia (18, 42) in the elderly.
The mechanisms underlying low insulin in sarcopenia in diabetic and non-diabetic older individuals is not completely understood. The hypothesis that age-related changes in the number and size of Type I versus Type 2 muscle fibers favoring a tendency for altered insulin sensitivity may explain the observed association in this study (9). In diabetes, the pathogenetic role and mechanisms of insulin is highly complex, and a large volume of literature now strongly favor the view that β cell dysfunction but not insulin resistance may be the central defect responsible for the development of Type 2 diabetes (11). Disordered insulin secretion contributes to the development of insulin resistance and may be an initiating factor in the progression to T2DM (43, 44). The loss of β cell function is progressive throughout the course of diabetes, beginning with defect in first-phase insulin secretion, followed by a decreasing maximal capacity of insulin secretion, before a reduction of steady-state and basal insulin secretion and complete β cell failure requiring insulin treatment (45). Reduction of insulin level and dysregulated insulin signaling activity both in diabetic or non-diabetic older individuals diminishes the anabolic capacity of insulin to alleviate MPB in skeletal muscles and may possibly be a primary mechanism shared by age-related sarcopenia and diabetes.
Taken together, a reduced level of endogenous insulin secretion may thus be a risk factor for sarcopenia development in both Japanese and Chinese diabetic elderly. This is particularly so given that it is widely recognized that T2DM in East Asians is characterized primarily by β cell dysfunction (12). As diabetes is a highly heterogeneous condition across different populations, whether this may explain the contrary findings from Western population studies of non-diabetic older persons (27, 46), showing hyperinsulinemia to be associated with lower muscle mass and strength, should be investigated in further studies.
IGF-1 has been shown to have an anabolic effect on muscle tissues. In line with report from previous studies (28, 47), our study found that the level of IGF-1 was associated with increased muscle strength. The serum level of IGF-1 decreases during aging, together with decreased tissue responsiveness and intracellular signaling (48). Previous studies also reported IGF-1 to be associated with various physical performance and mobility tasks, and all-cause mortality in the elderly (49, 50). In mouse models, exogenous systemic administration of IGF-1 improves contractile function, facilitates the functional recovery of injured skeletal muscle, and enhances muscle oxidative enzymes (51, 52). This may be explained by the mediating role of IGF-1 in muscle growth and subsequent regeneration: IGF-1 promotes the stimulation of muscle cell proliferation and differentiation, and MPS while inhibiting its degradation (53). The levels of c-peptide are found lower in participants with sarcopenia and positively associated with BMI, SMI, and knee extension strength in the whole sample and those without diabetes. C-peptide level is not affected by insulin injections or liver metabolization and are thus considered a better measure of portal insulin secretion than insulin itself (54, 55). Leptin is an adipokine secreted by white adipose tissue. Leptin acts on hypothalamic neurons to regulate food consumption and body fat (56). Moreover, leptin impacts on pancreatic beta cells, immune cells and muscle cells and modulate glucose metabolism, inflammatory processes and insulin resistance (57, 58). Considering the proinflammatory properties of leptin, previous studies have investigated the relationship between serum/plasma leptin level and sarcopenia, with generation of conflicting results. Our results of lower leptin level in sarcopenic older individuals corroborate the findings of previous studies which demonstrated an inverse association between serum leptin level and sarcopenia in hemodialysis patients (59). Consistently, frail hospitalized patients with a lower mid-arm muscle area were found to have lower leptin levels than their healthy counterparts (60). The discrepancies in these studies may be caused by disparate effects of leptin on different populations such as obese individuals, hemodialysis subjects, and individuals with different ethnicities. These findings taken together thus also support the direct and indirect role of insulin in increasing muscle mass and function, regardless of diabetes status.
The present study defined sarcopenia according to the latest population-specific standardized criteria for Asian elderly: AWGS 2019. Furthermore, we validated our results derived from pre-frail frail older individuals in another independent study sample of robust and pre-frail frail older individuals, thus supporting the robustness of these findings. However, there are limitations in the cross-sectional study design and future studies employing longitudinal measurements should be undertaken to elucidate its temporal causal relationship. There is also limited sample size in this study. Insulin secretion and impaired insulin signaling may be a primary defect in sarcopenia associated with their prognosis and quality of life in both diabetic and non-diabetic individuals. Moderate-intensity exercise-based interventions, in combination with appropriate nutritional supplementation, are recommended to improve both muscle mass and performance and insulin levels in the elderly.
Data availability statement
The raw data supporting the conclusions of this article will be made available by the authors, without undue reservation.
Ethics statement
The studies involving human participants were reviewed and approved by National Health Group (NHG) Domain Specific Review Board (DSRB) of Singapore. The patients/participants provided their written informed consent to participate in this study.
Author contributions
YL did the statistical analyses and drafted the manuscript. TN designed the study and directed the data collection as the principal investigator of the study. All authors contributed to the study concept and design, interpretation of data, and critical review of the manuscript and approved the final version.
Funding
This work was supported by a research grant from the National Medical Research Council (NMRC/1108/2007).
Acknowledgments
We thank the following voluntary welfare organizations for their support in kind: Geylang East Home for the Aged, Presbyterian Community Services, St Luke’s Eldercare Services, Thye Hua Kwan Moral Society (Moral Neighbourhood Links), Yuhua Neighbourhood Link, Henderson Senior Citizens’ Home, NTUC Eldercare Co-op. Ltd., Thong Kheng Seniors Activity Centre (Queenstown Centre), and Redhill Moral Seniors Activity Centre.
Conflict of interest
The authors declare that the research was conducted in the absence of any commercial or financial relationships that could be construed as a potential conflict of interest.
Publisher’s note
All claims expressed in this article are solely those of the authors and do not necessarily represent those of their affiliated organizations, or those of the publisher, the editors and the reviewers. Any product that may be evaluated in this article, or claim that may be made by its manufacturer, is not guaranteed or endorsed by the publisher.
Supplementary material
The Supplementary Material for this article can be found online at: https://www.frontiersin.org/articles/10.3389/fmed.2022.971622/full#supplementary-material
References
1. Kalyani RR, Corriere M, Ferrucci L. Age-related and disease-related muscle loss: the effect of diabetes, obesity, and other diseases. Lancet Diab Endocrinol. (2014) 2:819–29. doi: 10.1016/s2213-858770034-8
2. Drew L. Fighting the inevitability of ageing. Nature. (2018) 555:S15–7. doi: 10.1038/d41586-018-02479-z
3. Wiedmer P, Jung T, Castro JP, Pomatto LCD, Sun PY, Davies KJA, et al. Sarcopenia – molecular mechanisms and open questions. Ageing Res Rev. (2021) 65:101200. doi: 10.1016/j.arr.2020.101200
4. Petermann-Rocha F, Balntzi V, Gray SR, Lara J, Ho F, Pell JP, et al. Global prevalence of sarcopenia and severe sarcopenia: a systematic review and meta-analysis. J Cachexia Sarcopenia Muscle. (2022) 13:86–99. doi: 10.1002/jcsm.12783
5. Sarcopenia E. A fate worth challenging. Lancet Diab Endocrinol. (2014) 2:183. doi: 10.1016/s2213-858770055-5
6. Cruz-Jentoft AJ, Sayer AA. Sarcopenia. Lancet. (2019) 393:2636–46. doi: 10.1016/S0140-673631138-9
7. Volpato S, Bianchi L, Lauretani F, Lauretani F, Bandinelli S, Guralnik JM, et al. Role of muscle mass and muscle quality in the association between diabetes and gait speed. Diab Care. (2012) 35:1672–9. doi: 10.2337/dc11-2202
8. Yang C-W, Li C-I, Li T-C, Liu C-S, Lin C-H, Lin W-Y, et al. The joint association of insulin sensitivity and physical activity on the skeletal muscle mass and performance in community-dwelling older adults. Exp Gerontol. (2017) 95:34–8. doi: 10.1016/j.exger.2017.05.006
9. Khamseh ME, Malek M, Aghili R, Emami Z. Sarcopenia and diabetes: pathogenesis and consequences. Br J Diab Vasc Dis. (2011) 11:230–4.
10. Schofield CJ, Sutherland C. Disordered insulin secretion in the development of insulin resistance and type 2 diabetes. Diabet Med. (2012) 29:972–9. doi: 10.1111/j.1464-5491.2012.03655.x
11. Yabe D, Seino Y, Fukushima M, Seino S. Beta cell dysfunction versus insulin resistance in the pathogenesis of type 2 diabetes in east asians. Curr Diab Rep. (2015) 15:602. doi: 10.1007/s11892-015-0602-9
12. Yabe D, Seino Y. Type 2 diabetes via beta-cell dysfunction in east asian people. Lancet Diabet Endocrinol. (2016) 4:2–3. doi: 10.1016/s2213-858700389-7
13. Umegaki H. Sarcopenia and diabetes: hyperglycemia is a risk factor for age-associated muscle mass and functional reduction. J Diabet Invest. (2015) 6:623–4. doi: 10.1111/jdi.12365
14. Jang HC. Sarcopenia, frailty, and diabetes in older adults. Diabet Metab J. (2016) 40:182–9. doi: 10.4093/dmj.2016.40.3.182
15. Park MH, Kim DH, Lee EK, Kim ND, Im DS, Lee J, et al. Age-related inflammation and insulin resistance: a review of their intricate interdependency. Arch Pharmacal Res. (2014) 37:1507–14. doi: 10.1007/s12272-014-0474-6
16. Aleman-Mateo H, Lopez Teros MT, Ramirez FA, Astiazaran-Garcia H. Association between insulin resistance and low relative appendicular skeletal muscle mass: evidence from a cohort study in community-dwelling older men and women participants. J Gerontol Ser A Biol Sci Med Sci. (2014) 69:871–7. doi: 10.1093/gerona/glt193
17. Prod’homme M, Rieu I, Balage M, Dardevet D, Grizard J. Insulin and amino acids both strongly participate to the regulation of protein metabolism. Curr Opin Clin Nutr Metab Care. (2004) 7:71–7. doi: 10.1097/00075197-200401000-00012
18. Cleasby ME, Jamieson PM, Atherton PJ. Insulin resistance and sarcopenia: mechanistic links between common co-morbidities. J Endocrinol. (2016) 229:R67–81. doi: 10.1530/joe-15-0533
19. DeFronzo RA, Tripathy D. Skeletal muscle insulin resistance is the primary defect in type 2 diabetes. Diabet Care. (2009) 32:S157–63. doi: 10.2337/dc09-S302
20. Guillet C, Zangarelli A, Gachon P, Morio B, Giraudet C, Rousset P, et al. Whole body protein breakdown is less inhibited by insulin, but still responsive to amino acid, in nondiabetic elderly subjects. J Clin Endocrinol Metab. (2004) 89:6017–24. doi: 10.1210/jc.2003-031323
21. Abdulla H, Smith K, Atherton PJ, Idris I. Role of insulin in the regulation of human skeletal muscle protein synthesis and breakdown: a systematic review and meta-analysis. Diabetologia. (2016) 59:44–55. doi: 10.1007/s00125-015-3751-0
22. Bouchi R, Fukuda T, Takeuchi T, Nakano Y, Murakami M, Minami I, et al. Insulin treatment attenuates decline of muscle mass in japanese patients with type 2 diabetes. Calcified Tiss Int. (2017) 101:1–8. doi: 10.1007/s00223-017-0251-x
23. Groen BB, Hamer HM, Snijders T, van Kranenburg J, Frijns D, Vink H, et al. Skeletal muscle capillary density and microvascular function are compromised with aging and type 2 diabetes. J Appl Physiol. (2014) 116:998–1005. doi: 10.1152/japplphysiol.00919.2013
24. Wilkes EA, Selby AL, Atherton PJ, Patel R, Rankin D, Smith K, et al. Blunting of insulin inhibition of proteolysis in legs of older subjects may contribute to age-related sarcopenia. Am J Clin Nutr. (2009) 90:1343–50. doi: 10.3945/ajcn.2009.27543
25. Wang T, Feng X, Zhou J, Gong H, Xia S, Wei Q, et al. Type 2 diabetes mellitus is associated with increased risks of sarcopenia and pre-sarcopenia in chinese elderly. Sci Rep. (2016) 6:38937. doi: 10.1038/srep38937
26. Mesinovic J, Zengin A, De Courten B, Ebeling PR, Scott D. Sarcopenia and type 2 diabetes mellitus: a bidirectional relationship. Diabet Metab Syndr Obes Targets Ther. (2019) 12:1057–72. doi: 10.2147/dmso.s186600
27. Kalyani RR, Metter EJ, Ramachandran R, Chia CW, Saudek CD, Ferrucci L. Glucose and insulin measurements from the oral glucose tolerance test and relationship to muscle mass. J Gerontol Ser A Biol Sci Med Sci. (2012) 67:74–81. doi: 10.1093/gerona/glr022
28. Tanaka K, Kanazawa I, Sugimoto T. Reduction in endogenous insulin secretion is a risk factor of sarcopenia in men with type 2 diabetes mellitus. Calcified Tissue Int. (2015) 97:385–90. doi: 10.1007/s00223-015-9990-8
29. Fujita S, Glynn EL, Timmerman KL, Rasmussen BB, Volpi E. Supraphysiological hyperinsulinaemia is necessary to stimulate skeletal muscle protein anabolism in older adults: evidence of a true age-related insulin resistance of muscle protein metabolism. Diabetologia. (2009) 52:1889–98. doi: 10.1007/s00125-009-1430-8
30. Tosato M, Marzetti E, Cesari M, Savera G, Miller RR, Bernabei R, et al. Measurement of muscle mass in sarcopenia: from imaging to biochemical markers. Aging Clin Exp Res. (2017) 29:19–27. doi: 10.1007/s40520-016-0717-0
31. Lagace JC, Brochu M, Dionne IJ. A counterintuitive perspective for the role of fat-free mass in metabolic health. Phys Ther. (2020) 11:343–7. doi: 10.1002/jcsm.12520
32. Cesari M, Landi F, Vellas B, Bernabei R, Marzetti E. Sarcopenia and physical frailty: two sides of the same coin. Front Aging Neurosci. (2014) 6:192. doi: 10.3389/fnagi.2014.00192
33. Clegg A, Young J, Iliffe S, Rikkert MO, Rockwood K. Frailty in elderly people. Lancet. (2013) 381:752–62. doi: 10.1016/s0140-673662167-9
34. Ng TP, Feng L, Nyunt MS, Feng L, Niti M, Tan BY, et al. Nutritional, physical, cognitive, and combination interventions and frailty reversal among older adults: a randomized controlled trial. Am J Med. (2015) 128:1225–36.e1. doi: 10.1016/j.amjmed.2015.06.017
35. Fried LP, Tangen CM, Walston J, Newman AB, Hirsch C, Gottdiener J, et al. Frailty in older adults: evidence for a phenotype. J Gerontol Ser A Biol Sci Med Sci. (2001) 56:M146–56.
36. Lu Y, Tan CT, Nyunt MS, Mok EW, Camous X, Kared H, et al. Inflammatory and immune markers associated with physical frailty syndrome: findings from singapore longitudinal aging studies. Oncotarget. (2016) 7:28783–95. doi: 10.18632/oncotarget.8939
37. Lord SR, Menz HB, Tiedemann A. A physiological profile approach to falls risk assessment and prevention. Phys Ther. (2003) 83:237–52. doi: 10.1093/ptj/83.3.237
38. Ng TP, Feng L, Nyunt MS, Larbi A, Yap KB. Frailty in older persons: multisystem risk factors and the frailty risk index (fri). J Am Med Dir Assoc. (2014) 15:635–42. doi: 10.1016/j.jamda.2014.03.008
39. Heymsfield SB, Smith R, Aulet M, Bensen B, Lichtman S, Wang J, et al. Appendicular skeletal muscle mass: measurement by dual-photon absorptiometry. Am J Clin Nutr. (1990) 52:214–8.
40. Nelson HD, Nevitt MC, Scott JC, Stone KL, Cummings SR. Smoking, alcohol, and neuromuscular and physical function of older women. study of osteoporotic fractures research group. JAMA. (1994) 272:1825–31. doi: 10.1001/jama.1994.03520230035035
41. Chen LK, Woo J, Assantachai P, Auyeung TW, Chou MY, Iijima K, et al. Asian working group for sarcopenia: 2019 consensus update on sarcopenia diagnosis and treatment. J Am Med Dir Assoc. (2019) 21:300–7. doi: 10.1016/j.jamda.2019.12.012
42. Lee SW, Youm Y, Lee WJ, Choi W, Chu SH, Park YR, et al. Appendicular skeletal muscle mass and insulin resistance in an elderly korean population: the korean social life, health and aging project-health examination cohort. Diabet Metab J. (2015) 39:37–45. doi: 10.4093/dmj.2015.39.1.37
43. Halban PA, Polonsky KS, Bowden DW, Hawkins MA, Ling C, Mather KJ, et al. B -cell failure in type 2 diabetes: postulated mechanisms and prospects for prevention and treatment. J Clin Endocrinol Metab. (2014) 99:1983–92. doi: 10.1210/jc.2014-1425
44. Ohn JH, Kwak SH, Cho YM, Lim S, Jang HC, Park KS, et al. 10-year trajectory of β-cell function and insulin sensitivity in the development of type 2 diabetes: a community-based prospective cohort study. Lancet Diabet Endocrinol. (2016) 4:27–34. doi: 10.1016/S2213-858700336-8
45. Gotthardt M, Eizirik DL, Cnop M, Brom M. Beta cell imaging – a key tool in optimized diabetes prevention and treatment. Trends Endocrinol Metab. (2014) 25:375–7. doi: 10.1016/j.tem.2014.02.002
46. Barzilay JI, Cotsonis GA, Walston J, Schwartz AV, Satterfield S, Miljkovic I, et al. Insulin resistance is associated with decreased quadriceps muscle strength in nondiabetic adults aged >or=70 years. Diabet Care. (2009) 32:736–8. doi: 10.2337/dc08-1781
47. Ascenzi F, Barberi L, Dobrowolny G, Villa Nova Bacurau A, Nicoletti C, Rizzuto E, et al. Effects of Igf-1 isoforms on muscle growth and sarcopenia. Aging Cell. (2019) 18:e12954. doi: 10.1111/acel.12954
48. Barclay RD, Burd NA, Tyler C, Tillin NA, Mackenzie RW. The role of the Igf-1 signaling cascade in muscle protein synthesis and anabolic resistance in aging skeletal muscle. Front Nutr. (2019) 6:146. doi: 10.3389/fnut.2019.00146
49. Bian A, Ma Y, Zhou X, Guo Y, Wang W, Zhang Y, et al. Association between sarcopenia and levels of growth hormone and insulin-like growth factor-1 in the elderly. BMC Muscul Dis. (2020) 21:214. doi: 10.1186/s12891-020-03236-y
50. Zhang WB, Aleksic S, Gao T, Weiss EF, Demetriou E, Verghese J, et al. Insulin-like growth factor-1 and Igf binding proteins predict all-cause mortality and morbidity in older adults. Cells. (2020) 9:6. doi: 10.3390/cells9061368
51. Schertzer JD, Ryall JG, Lynch GS. Systemic administration of Igf-I enhances oxidative status and reduces contraction-induced injury in skeletal muscles of mdx dystrophic mice. Am J Physiol Endocrinol Metab. (2006) 291:E499–505. doi: 10.1152/ajpendo.00101.2006
52. Gregorevic P, Plant DR, Leeding KS, Bach LA, Lynch GS. Improved contractile function of the mdx dystrophic mouse diaphragm muscle after insulin-like growth factor-I administration. Am J Pathol. (2002) 161:2263–72. doi: 10.1016/S0002-944064502-6
53. Yoshida T, Delafontaine P. Mechanisms of Igf-1-mediated regulation of skeletal muscle hypertrophy and atrophy. Cells. (2020) 9:70. doi: 10.3390/cells9091970
54. Clark PM. Assays for insulin, proinsulin(S) and C-peptide. Ann Clin Biochem. (1999) 36:541–64. doi: 10.1177/000456329903600501
55. Shapiro ET, Tillil H, Rubenstein AH, Polonsky KS. Peripheral insulin parallels changes in insulin secretion more closely than c-peptide after bolus intravenous glucose administration. J Clin Endocrinol Metab. (1988) 67:1094–9. doi: 10.1210/jcem-67-5-1094
56. Morrison CD. Leptin signaling in brain: a link between nutrition and cognition? Biochim Biophys Acta. (2009) 1792:401–8. doi: 10.1016/j.bbadis.2008.12.004
57. Bjørbaek C, Kahn BB. Leptin signaling in the central nervous system and the periphery. Recent Prog Horm Res. (2004) 59:305–31. doi: 10.1210/rp.59.1.305
58. Kwon H, Pessin JE. Adipokines mediate inflammation and insulin resistance. Front Endocrinol. (2013) 4:71. doi: 10.3389/fendo.2013.00071
59. Lin YL, Wang CH, Lai YH, Kuo CH, Syu RJ, Hsu BG. Negative correlation between leptin serum levels and sarcopenia in hemodialysis patients. Int J Clin Exp Pathol. (2018) 11:1715–23.
Keywords: sarcopenia, diabetes, frailty, insulin, aging
Citation: Lu Y, Lim WS, Jin X, Zin Nyunt MS, Fulop T, Gao Q, Lim SC, Larbi A and Ng TP (2022) Lower insulin level is associated with sarcopenia in community-dwelling frail and non-frail older adults. Front. Med. 9:971622. doi: 10.3389/fmed.2022.971622
Received: 17 June 2022; Accepted: 07 November 2022;
Published: 22 November 2022.
Edited by:
Tzvi Dwolatzky, Technion - Israel Institute of Technology, IsraelReviewed by:
Siti Setiati, University of Indonesia, IndonesiaGuilherme Eustaquio Furtado, Instituto Politécnico da Guarda, Portugal
Copyright © 2022 Lu, Lim, Jin, Zin Nyunt, Fulop, Gao, Lim, Larbi and Ng. This is an open-access article distributed under the terms of the Creative Commons Attribution License (CC BY). The use, distribution or reproduction in other forums is permitted, provided the original author(s) and the copyright owner(s) are credited and that the original publication in this journal is cited, in accordance with accepted academic practice. No use, distribution or reproduction is permitted which does not comply with these terms.
*Correspondence: Tze Pin Ng, cGNtbmd0cEBudXMuZWR1LnNn