- 1Department of Surgery, The First Affiliated Hospital of China Medical University, Shenyang, China
- 2Department of Gastrointestinal Surgery, The First Affiliated Hospital of China Medical University, Shenyang, China
With the continuous development of bioinformatics and public database, more and more genes that play a role in cancers have been discovered. Synaptotagmins (SYTs) are abundant, evolutionarily conserved integral membrane proteins composed of a short N-terminus, a variable linker domain, a single transmembrane domain, and two C2 domains, and they constitute a family of 17 isoforms. The synaptotagmin family members are known to regulate calcium-dependent membrane fusion events. Some SYTs play roles in hormone secretion or neurotransmitter release or both, and much evidence supports SYTs as Ca2+ sensors of exocytosis. Since 5 years ago, an increasing number of studies have found that SYTs also played important roles in the occurrence and development of lung cancer, gastric cancer, colon cancer, and other cancers. Down-regulation of SYTs inhibited cell proliferation, migration, and invasion of cancer cells, but promoted cell apoptosis. Growth of peritoneal nodules is inhibited and survival is prolonged in mice administrated with siSYTs intraperitoneally. Therefore, most studies have found SYTs serve as an oncogene after overexpression and may become potential prognostic biomarkers for multiple cancers. This article provides an overview of recent studies that focus on SYT family members’ roles in cancers and highlights the advances that have been achieved.
Introduction
Synaptotagmins (SYTs) are a family of membrane-trafficking proteins composed of a short N-terminus, a variable linker domain, a single transmembrane domain, and two C2 domains (C2A and C2B domains) (1). The C2 domains are binding sites of Ca2+. SYT1–3, 5–7, and 9 have C2 domains, while the others do not (2). SYTs are known to regulate calcium-dependent membrane fusion events. At present, it has been found that humans have 17 synaptotagmin isoforms whose structure can be predicted by AlphaFold Protein Structure Database (3, 4) (Figure 1). The structure of some SYTs has been resolved for a long time (5–7). SYTs are involved in postsynaptic receptor endocytosis (8), synaptic vesicle exocytosis (9), synaptic plasticity (10), and vesicle trafficking (11). Since 5 years ago, an increasing number of studies have found that SYTs also play an important role in the occurrence and development of lung cancer, gastric cancer (GC), colon cancer, and other cancers (12–15) (Supplementary Figure 1). This article provides an overview of recent studies that focus on SYT family members’ roles in cancers and highlights the advances that have been achieved (Table 1).
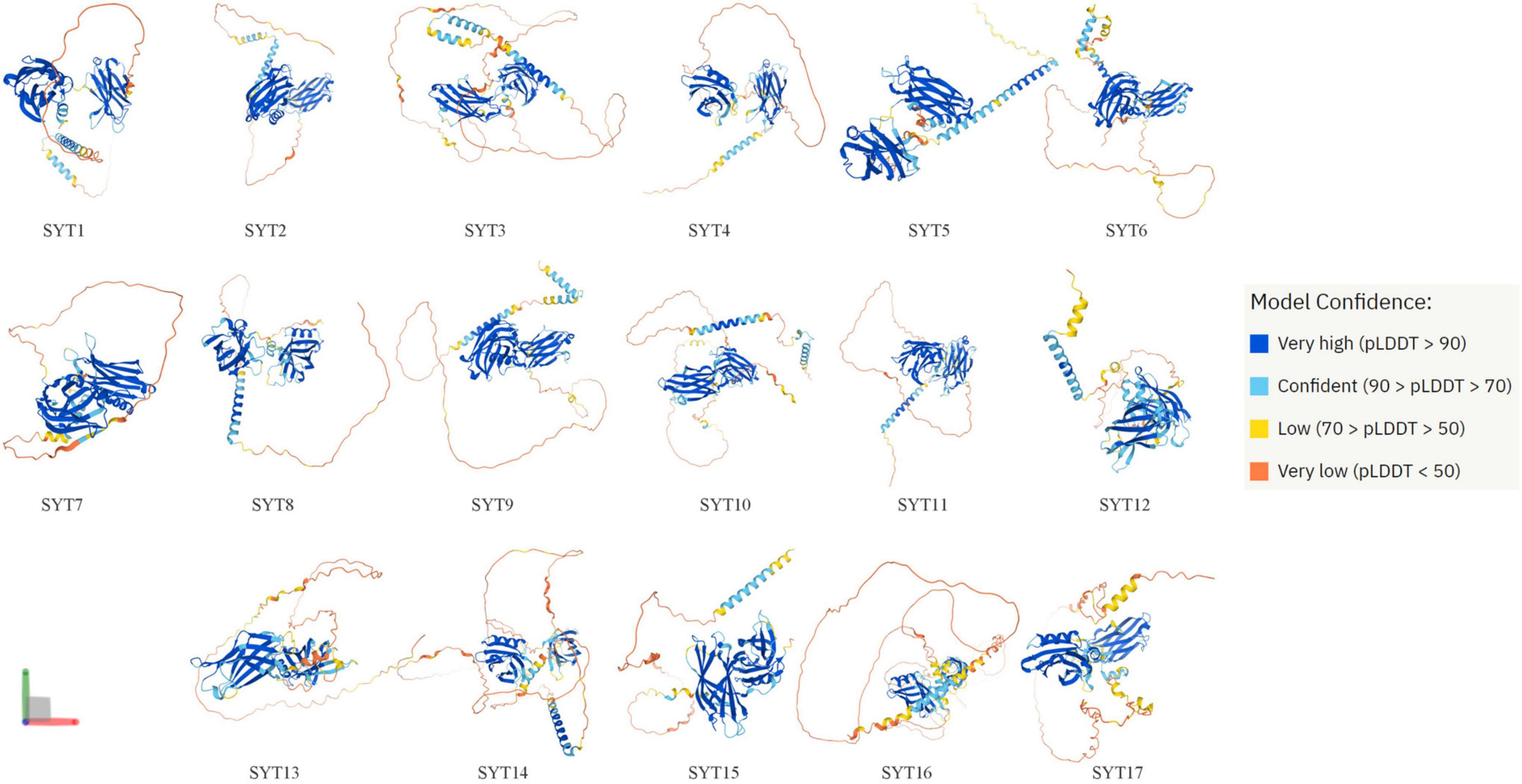
Figure 1. Structure of 17 human synaptotagmin family members predicted by AlphaFold. AlphaFold is an AI system developed by DeepMind that predicts a protein’s 3D structure from its amino acid sequence. AlphaFold produces a per-residue confidence score (pLDDT) between 0 and 100. Some regions below 50 pLDDT may be unstructured in isolation.
Roles of synaptotagmin family members in cancers
SYT1
SYT1 is located at the vesicular membrane of nerve and endocrine cells. It is considered to be the main Ca2+ sensor in neurotransmission and hormone secretion processes and plays a vital role in Ca2+-induced secretion processes (16). In a recent study, downregulation of SYT1 significantly suppressed the proliferation, invasion, and migration of colon cancer cells, but induced cell apoptosis. These results suggested that SYT1 may serve as an oncogene in colon cancer (17).
SYT7
SYT7 resides on human chromosome 11q12.2 and encodes a predicted single-pass 46-kDa transmembrane protein (18). SYT7 encodes a protein that plays a central role in the regulation of calcium-dependent lysosome exocytosis (19), facilitation of central synapses (20, 21), and the regulation of membrane trafficking during synaptic transmission (20, 22). SYT7 is currently the most studied isoform in oncological diseases.
Compared with adjacent normal tissues, SYT7 was found overexpressed in tissues of GC with hepatic metastasis. Meanwhile, high expression of SYT7 in primary GC tissues was closely correlated with hepatic recurrence, metastasis (stage IV GC), and adverse prognostic characteristics. Knockdown of SYT7 suppressed the proliferation of GC cells and attenuated the invasion, migration, and adhesion ability of cancer (13). It has also been reported that SYT7 is overexpressed in colorectal cancer (CRC). The higher level of SYT7 was significantly associated with a higher pathological stage of CRC. Downregulation of SYT7 inhibited RKO cell proliferation and colony formation but promoted G2/M arrest and subsequent apoptosis (15). Another study showed that SYT7 was significantly overexpressed in hepatocellular carcinoma (HCC) and was closely correlated with tumor size, differentiation, vascular invasion, and lymph node metastasis. Meanwhile, SYT7 was also identified as a risk factor for disease-free survival (DFS) and overall survival (OS). Additionally, knockdown of SYT7 in HCC could inhibit cell proliferation and colony-forming ability as well as induce cell cycle arrest (23, 24).
One recent study showed that SYT7 was upregulated in non-small-cell lung cancer (NSCLC), and its high expression was positively correlated with T stage and tumor differentiation. Patients with lower SYT7 expression had longer survival than those with higher expression (25). Liu et al. reported that SYT7 served as an oncogene in NSCLC in vitro, including promoting proliferation, invasion, and migration, but inhibiting apoptosis of cancer cells. It was also demonstrated that shSYT7 significantly blocked the growth of NSCLC tumor cells in a xenograft model. The expression of Vimentin and N-cadherin in cultured cells was decreased after the knockdown of SYT7, while E-cadherin levels increased (14). The above results revealed that SYT7 played a vital role in promoting tumorigenesis by activating epithelial-mesenchymal transition (EMT) in NSCLC. Another study demonstrated that the expression levels of SYT7 were elevated in both lung cancer tissues and cell lines. In addition, SYT7 was shown to inhibit senescence and promote growth and colony-forming capacity in lung cancer cells. The interaction between SYT7 and P53 further potentiated the interaction between P53 and its E3 ligase MDM2 (26).
Downregulation of SYT7 can promote cellular apoptosis and subsequently inhibit the growth of glioblastoma (27). One study by Wu et al. demonstrated that the expression levels of SYT7 in osteosarcoma tissues had a positive correlation with tumor stage. Functional assays showed that SYT7 silencing could significantly suppress cell proliferation as well as the colony-forming ability of osteosarcoma in vitro with time independence. Furthermore, knockdown of SYT7 could also increase cell apoptosis rates, induce cell cycle arrest with a decreased proportion of S phase and increased G2 phase, and inhibit the invasion and migration capability (28). Fu et al. discovered that downregulation of SYT7 obviously inhibited the migration and tumor growth of head and neck squamous cell carcinoma (HNSCC) in vivo. They also found that ΔNp63α could affect HNSCC cells by downregulating the expression of SYT7 in vitro, including inhibiting proliferation, promoting apoptosis, and reducing the proportion of cancer cells in G1 phase. Therefore, the ΔNp63α/SYT7 axis might be a potential clinically effective target for the treatment of HNSCC (29).
In summary, SYT7 may serve as an oncogene in GC, CRC, HCC, lung cancer, glioblastoma, osteosarcoma, and HNSCC. High expression of SYT7 predicted a poor prognosis in GC, HCC, and HNSCC. Nevertheless, the precise mechanisms underlying the biological activity of SYT7 remain to be elaborated on in these cancers.
SYT8
There are two forms of SYT8 (40 and 50 kDa). The 40-kDa form was present in the cytosol in the brain, in clonal beta-cells, and in PC12 cells, while the 50-kDa form was localized in very typical clusters. Further research found that SYT8 is not a Ca2+ sensor in exocytotic membrane fusion in endocrine cells (30). There are few studies on SYT8 function; therefore, the possible functions of SYT8 in the brain or other organs are still unknown. However, in recent years, several studies have shown that abnormal expression of SYT8 may affect the occurrence and development of tumor diseases. SYT8 was overexpressed in tumor tissues of pancreatic cancer and played important roles in promoting cell proliferation, invasion, and migration both in vivo and in vitro. Furthermore, the authors also identified SYT8 to be involved in signaling via the TNNI2/ERRα/SIRT1 axis (31).
High expression scores of the dual-marker expression panel (MAGED2 and SYT8) were significantly correlated with higher tumor stage, more lymph node or peritoneal metastasis, and more vascular invasion. Moreover, compared with single markers, the C-index of the combination panel was obviously higher. Patients with GC can be precisely stratified into high, intermediate and low risk by this dual-marker predictive signature after gastrectomy (32). The authors also found that the optimal expression panel comprised four constituents (SYT8, MAGED2, FAM461, and BTG) among 32,767 combinations with a C-index value of 0.793. Both OS and DFS decreased incrementally with increasing expression scores (33). Kanda et al. found that the expression levels of SYT8 were higher in GC tissues of patients with peritoneal recurrence or metastasis. Downregulation of SYT8 in GC cells was correlated with inhibition of cell invasion, migration, and fluorouracil resistance. The growth of peritoneal nodules was significantly suppressed by intraperitoneal administration of SYT8-siRNA into nude mice engrafted with GC cells, and survival was also prolonged (34).
Finally, it may be concluded that SYT8 may serve as an oncogene in pancreatic cancer and GC. The underlying mechanism in pancreatic cancer could be involved in TNNI2/ERRα/SIRT1 signaling pathway.
SYT12
SYT12 encodes proteins involved in regulating transmitter release in the nervous system (35). Moreover, SYT12 phosphorylation by cAMP-dependent protein kinase is essential for hippocampal mossy fiber long-term potentiation (36). In the field of oncology, a prospective cohort study investigated the effect of biomarkers (SYT12, ITGA2, and CDH3) on outcomes of papillary thyroid cancer (PTC) patients. SYT12 as a single marker provided the best prediction performance of initial metastasis (specificity: 54%; sensitivity: 72%) compared with ITGA2 and CDH3. For long-term outcomes, the best performance was obtained by combining American Thyroid Association risk stratification with SYT12, with a specificity and sensitivity of 73 and 88%, respectively (37). The above results suggest that SYT12 may serve as a prognostic biomarker in PTC but warrants to be further validation in larger populations. In The Cancer Genome Atlas (TCGA) cohort, SYT12 was significantly upregulated in PTC. Moreover, the expression levels of SYT12 were positively related to the incidence of lymph node metastasis. Functional experiments in vitro showed that knockdown of SYT12 inhibited cell colony formation, proliferation, migration, and invasion ability of PTC cell lines, but accelerated the process of apoptosis (12).
Eizuka et al. found that SYT12 was overexpressed in both oral squamous cell carcinoma (OSCC)-derived cell lines and primary OSCC tissues. Knockdown of SYT12 in OSCC inhibited cellular proliferation, invasion, and migration and arrested the cell cycle in G1 phase. Meanwhile, L-dopa (L-3,4-dihydroxyphenylalanine), which has been approved for Parkinson’s disease, could reduce cellular SYT12 expression, allowing cells to acquire a cellular phenotype similar to SYT12 knockdown. Therefore, L-dopa is expected to become a new drug for the clinical therapy of OSCC by regulating the expression level of SYT12 (38). Analysis through the public database TCGA database revealed that SYT12 expression was significantly increased in tissues of lung adenocarcinoma (LUAD). Moreover, SYT12 was confirmed to be correlated with advanced tumor stage and poor prognosis. SYT12 also promoted LUAD cell proliferation and migration in vitro and increased the weight and volume of tumors in mice xenograft models. In parallel, SYT12 could activate the PI3K/AKT/mTOR signaling pathway by increasing the level of phosphorylation of PIK3R3 (39).
To summarize, SYT12 may serve as an oncogene in PTC, OSCC, and LUAD. High expression of SYT12 predicted poor prognosis in LUAD. The underlying mechanism in LUAD could be involved in signaling via the PI3K/AKT/mTOR axis.
SYT13
SYT13 locates at human chromosome 11p11.2 and encodes a predicted single-pass 47-kDa transmembrane protein (40). SYT13 also serves as a neuroendocrine marker in the pancreas, intestine, and brain (41). In an analysis of 200 GC patients, the expression levels of SYT13 mRNA in patients with peritoneal recurrence or metastasis were significantly higher than those in Stage I GC patients. SYT13 knockdown in a GC cell line obviously reduced cell migration and invasion but did not alter proliferation and apoptosis. Meanwhile, the growth of peritoneal nodules is inhibited and survival is prolonged in mice administrated with siSYT13 intraperitoneally (42). Further analysis revealed that SYT13 expression was correlated with shorter peritoneal recurrence-free survival and OS. Multivariate analysis demonstrated that SYT13 positivity in lavage fluid was a vital prognostic factor for predicting GC peritoneal recurrence (P = 0.0246, HR = 3.69, 95% CI = 1.18–12.74) (43). Kanda et al. constructed a mouse xenograft model with GC peritoneal metastasis and discovered that intraperitoneal administration of amido-bridged nucleic acid-modified anti-SYT13 antisense oligonucleotides could inhibit the formation of peritoneal metastatic nodules and significantly prolong survival (44).
SYT13 was overexpressed in CRC samples compared with the adjacent normal samples. In vitro experiments showed that silencing of SYT13 could depress the activity of the CRC cell lines RKO and HCT116, including proliferation, colony formation, invasion, and migration. In vivo assays also showed the role of SYT13 in promoting tumor growth. In addition, after SYT13 knockdown, N-cadherin, Vimentin, and Snail expression were all inhibited, suggesting that downregulation of SYT13 may inhibit the occurrence of EMT (45). Keck et al. analyzed RNA isolated from matched primary neuroendocrine tumors of the small bowel (SBNETs), liver metastases, and normal small bowel tissue in 12 patients by utilizing RNA-Seq and whole transcriptome expression microarrays (46). The results showed that SYT13 was overexpressed in tumorous tissues and was associated with the progression of SBNETs. However, more laboratory and clinical investigations are warranted.
Downregulation of SYT13 in the LUAD cell lines A549 and H1299 could successfully suppress cell proliferation and clonal activity, but enhance apoptosis. Moreover, the knockdown of SYT13 decreased the migration capacity of H1299 cells. These results demonstrated that SYT13 was a vital promoter in the development of LUAD (47). Furthermore, SYT13 is overexpressed in both clinical specimens and cell lines of estrogen receptor (ER)-positive breast cancer. SYT13 was also revealed to be positively correlated with several oncogenes predominantly expressed in ER-positive breast cancer by PCR array analysis (48). These results suggest that SYT13 has a positive correlation with ER-related signaling pathways in breast cancer.
In conclusion, SYT13 may serve as an oncogene in GC, CRC, SBNETs, LUAD, and ER-positive breast cancer. High expression of SYT13 predicted poor prognosis in GC. More investigations of the mechanism underlying the biological activity of SYT13 in these cancers are warranted.
SYT14
Aberrant SYT14 was associated with neurodevelopmental abnormalities (49) and psychomotor retardation (50). Sheng et al. knocked down the expression of SYT14 in the human glioma cell line U87MG via RNAi, resulting in significant inhibition of cell proliferation and colony formation but a modest promotion of apoptosis. In parallel, more G2/M phase cells and fewer S phase cells were observed (51). These results reveal that SYT14 is upregulated in glioma cells and may participate in the occurrence and development of glioma.
SYT16
SYT16 lacks calcium-sensing as well as a transmembrane domain (52). Bioinformatic analysis of TCGA database revealed that the expression levels of SYT16 in glioma samples were significantly lower than that in normal samples. Moreover, SYT16 was only expressed in grade II and grade III glioma and was positively correlated with tumor grade. The higher the histological grade, the lower the expression. Multivariate analysis showed that SYT16 was a significant prognostic factor for glioma (53). Nevertheless, more laboratory studies should be conducted to further validate the biological activity of SYT16.
Other isoforms
Through bioinformatics analysis in online databases, the expression levels of SYT4, SYT9, and SYT14 were found to be upregulated in GC tissues compared with normal tissues and were negatively correlated with their methylation levels. Both the hypomethylation levels of SYT4, SYT9, and SYT14 and their high expression contributed to the suboptimal OS and DFS in GC. The expression of these three isoforms also played a key role in immune cell infiltration in GC (54). These findings suggested that these three isoforms might be reliable prognostic indicators and potential immunotherapeutic targets in GC patients.
Future prospects
Although the results from previous studies appear to be promising, supporting evidence of SYTs as a tumor marker is still lacking since most studies were retrospective. In the future, large-scale prospective studies are needed to further assess the value of SYTs in the diagnosis and prognostic prediction. We suggest the following for further research: (1) At present, we still do not understand the mechanism by which SYTs affect the occurrence and development of cancer. More work is required to elucidate the proteins and pathways that interact with SYTs to promote tumor growth and metastasis for further understanding of the biological functions of SYTs in cancers. (2) With the continuous improvement of public databases, more and more tumor markers or potential prognostic biomarkers have been discovered (55–58). For example, many SYTs were found significantly associated with poor prognosis in GC when analyzed using the Kaplan–Meier plotter (KM plotter) database (Supplementary Figure 2). However, the vast majority of studies in cancer are focused on SYT 7, 8, 12, and 13 currently. Thus, the following studies could be further extended to other isoforms in the future. (3) In addition, more than two isoforms of SYT expression abnormalities have been found in some tumors. For example, the expression of SYT7, SYT8, and SYT13 is increased in GC tissues, and all three isoforms can promote the proliferation, migration, and invasion of GC cells (13, 34, 42). Whether there is an intrinsic link between them also requires further research. (4) The classical function of SYTs mainly relies on their interaction with Ca2+ via the C2 domain. Is this Ca2+ sensing activity of SYTs involved in cancer? Do SYTs affect cancer via Ca2+ binding or independent of Ca2+ binding? In-depth studies of these issues can give us a better understanding of the anti-cancer mechanism of SYTs. (5) To develop SYT-targeted therapy, the dose, route, frequency, and duration of administration of siSYTs or inhibitors should be optimized. In addition, the existence of a synergistic effect of siSYTs in combination with existing chemotherapeutic agents also needs to be confirmed. Through the above research, we will obtain a better understanding of the functions of the SYTs and may find a new target for anticancer therapy.
Conclusion
In the past 5 years, an increasing number of studies have demonstrated that SYTs play important roles in the occurrence and development of cancers. At present, most studies have shown that SYTs serve as oncogenes after overexpression. Downregulation of SYTs can inhibit the proliferation, migration, and invasion of cancer cells, but promote apoptosis. Through more in-depth research, SYTs may become a new target for the treatment of tumor diseases in the future.
Author contributions
HS and NX searched the literature for recent advances in the field. HS wrote the manuscript. KW designed the study and edited and revised the manuscript. All authors approved the final version to be published.
Funding
Natural Science Foundation of Education Department of Liaoning Province (QNZR2020008) and Youth Elite Support Program of China Medical University (QGZ2018043).
Conflict of interest
The authors declare that the research was conducted in the absence of any commercial or financial relationships that could be construed as a potential conflict of interest.
Publisher’s note
All claims expressed in this article are solely those of the authors and do not necessarily represent those of their affiliated organizations, or those of the publisher, the editors and the reviewers. Any product that may be evaluated in this article, or claim that may be made by its manufacturer, is not guaranteed or endorsed by the publisher.
Supplementary material
The Supplementary Material for this article can be found online at: https://www.frontiersin.org/articles/10.3389/fmed.2022.968081/full#supplementary-material
Supplementary Figure 1 | Synaptotagmins currently known to play an important role in the occurrence and development of cancers. All these SYTs in this figure play as oncogenes. SYTs, synaptotagmins; NSCLC, non-small cell lung cancer; HNSCC, head and neck squamous cell carcinoma; OSCC, oral squamous cell carcinoma; LUAD, lung adenocarcinoma; PTC, papillary thyroid cancer.
Supplementary Figure 2 | Correlation between the expression levels of SYTs and patient survival. Expression data were analyzed using KM plotter (http://kmplot.com/). Patients were split by median expression. HRs (hazardous ratios), 95% CIs (confidence intervals), and log-rank P-values are indicated. (A) The relationship between overall survival and expression levels of SYTs in gastric cancer patients. (B) The relationship between relapse-free survival and expression levels of SYTs in gastric cancer patients.
References
1. Bowers MR, Reist NE. Synaptotagmin: Mechanisms of an electrostatic switch. Neurosci Lett. (2020) 722:134834. doi: 10.1016/j.neulet.2020.134834
2. Pang ZP, Südhof TC. Cell biology of Ca2+-triggered exocytosis. Curr Opin Cell Biol. (2010) 22:496–505. doi: 10.1016/j.ceb.2010.05.001
3. Jumper J, Evans R, Pritzel A, Green T, Figurnov M, Ronneberger O, et al. Highly accurate protein structure prediction with AlphaFold. Nature. (2021) 596:583–9. doi: 10.1038/s41586-021-03819-2
4. Varadi M, Anyango S, Deshpande M, Nair S, Natassia C, Yordanova G, et al. AlphaFold protein structure database: Massively expanding the structural coverage of protein-sequence space with high-accuracy models. Nucleic Acids Res. (2022) 50:D439–439. doi: 10.1093/nar/gkab1061
5. Sutton RB, Davletov BA, Berghuis AM, Südhof TC, Sprang SR. Structure of the first C2 domain of synaptotagmin I: A novel Ca2+/phospholipid-binding fold. Cell. (1995) 80:929–38. doi: 10.1016/0092-8674(95)90296-1
6. Sutton RB, Ernst JA, Brunger AT. Crystal structure of the cytosolic C2A-C2B domains of synaptotagmin III. Implications for Ca(+2)-independent snare complex interaction. J Cell Biol. (1999) 147:589–98. doi: 10.1083/jcb.147.3.589
7. Ruchala P, Waring AJ, Cilluffo M, Whitelegge JP, Gundersen CB. Insights into the structure and molecular topography of the fatty acylated domain of synaptotagmin-1. Biochim Biophys Acta Biomembr. (2019) 1861:677–84. doi: 10.1016/j.bbamem.2018.12.019
8. Awasthi A, Ramachandran B, Ahmed S, Benito E, Shinoda Y, Nitzan N, et al. Synaptotagmin-3 drives AMPA receptor endocytosis, depression of synapse strength, and forgetting. Science. (2019) 363:eaav1483. doi: 10.1126/science.aav1483
9. Bello OD, Jouannot O, Chaudhuri A, Stroeva E, Coleman J, Volynski KE, et al. Synaptotagmin oligomerization is essential for calcium control of regulated exocytosis. Proc Natl Acad Sci U.S.A. (2018) 115:E7624–31. doi: 10.1073/pnas.1808792115
10. Fujii T, Sakurai A, Littleton JT, Yoshihara M. Synaptotagmin 7 switches short-term synaptic plasticity from depression to facilitation by suppressing synaptic transmission. Sci Rep. (2021) 11:4059. doi: 10.1038/s41598-021-83397-5
11. Shimojo M, Madara J, Pankow S, Liu X, Yates J III, Südhof TC, et al. Synaptotagmin-11 mediates a vesicle trafficking pathway that is essential for development and synaptic plasticity. Genes Dev. (2019) 33:365–76. doi: 10.1101/gad.320077.118
12. Jin L, Zheng D, Chen D, Xia E, Guan Y, Wen J, et al. SYT12 is a novel oncogene that promotes thyroid carcinoma progression and metastasis. J Cancer. (2021) 12:6851–60. doi: 10.7150/jca.62555
13. Kanda M, Tanaka H, Shimizu D, Miwa T, Umeda S, Tanaka C, et al. SYT7 acts as a driver of hepatic metastasis formation of gastric cancer cells. Oncogene. (2018) 37:5355–66. doi: 10.1038/s41388-018-0335-8
14. Liu X, Li C, Yang Y, Liu X, Li R, Zhang M, et al. Synaptotagmin 7 in twist-related protein 1-mediated epithelial – Mesenchymal transition of non-small cell lung cancer. EBioMedicine. (2019) 46:42–53. doi: 10.1016/j.ebiom.2019.07.071
15. Wang K, Xiao H, Zhang J, Zhu D. Synaptotagmin7 is overexpressed in colorectal cancer and regulates colorectal cancer cell proliferation. J Cancer. (2018) 9:2349–56. doi: 10.7150/jca.25098
16. Banerjee A, Lee J, Nemcova P, Liu C, Kaeser PS. Synaptotagmin-1 is the Ca(2+) sensor for fast striatal dopamine release. Elife. (2020) 9:e58359. doi: 10.7554/eLife.58359
17. Lu H, Hao L, Yang H, Chen J, Liu J. miRNA-34a suppresses colon carcinoma proliferation and induces cell apoptosis by targeting SYT1. Int J Clin Exp Pathol. (2019) 12:2887–97.
18. Segovia M, Alés E, Montes MA, Bonifas I, Jemal I, Lindau M, et al. Push-and-pull regulation of the fusion pore by synaptotagmin-7. Proc Natl Acad Sci U.S.A. (2010) 107:19032–7. doi: 10.1073/pnas.1014070107
19. MacDougall DD, Lin Z, Chon NL, Jackman SL, Lin H, Knight JD, et al. The high-affinity calcium sensor synaptotagmin-7 serves multiple roles in regulated exocytosis. J Gen Physiol. (2018) 150:783–807. doi: 10.1085/jgp.201711944
20. Jackman SL, Turecek J, Belinsky JE, Regehr WG. The calcium sensor synaptotagmin 7 is required for synaptic facilitation. Nature. (2016) 529:88–91. doi: 10.1038/nature16507
21. Luo F, Südhof TC. Synaptotagmin-7-mediated asynchronous release boosts high-fidelity synchronous transmission at a central synapse. Neuron. (2017) 94:826–39.e3. doi: 10.1016/j.neuron.2017.04.020
22. Liu H, Bai H, Hui E, Yang L, Evans CS, Wang Z, et al. Synaptotagmin 7 functions as a Ca2+-sensor for synaptic vesicle replenishment. Elife. (2014) 3:e01524. doi: 10.7554/eLife.01524
23. Jin H, Pang Q, Fang M, Wang Y, Man Z, Tan Y, et al. Syt-7 overexpression predicts poor prognosis and promotes cell proliferation in hepatocellular carcinoma. Future Oncol. (2020) 16:2809–19. doi: 10.2217/fon-2020-0262
24. Jin H, Xu G, Zhang Q, Pang Q, Fang M. Synaptotagmin-7 is overexpressed in hepatocellular carcinoma and regulates hepatocellular carcinoma cell proliferation via Chk1-p53 signaling. Onco Targets Ther. (2017) 10:4283–93. doi: 10.2147/OTT.S143619
25. Han Q, Zou D, Lv F, Wang S, Yang C, Song J, et al. High SYT7 expression is associated with poor prognosis in human non-small cell lung carcinoma. Pathol Res Pract. (2020) 216:153101. doi: 10.1016/j.prp.2020.153101
26. Fei Z, Gao W, Xie R, Feng G, Chen X, Jiang Y. Synaptotagmin-7, a binding protein of P53, inhibits the senescence and promotes the tumorigenicity of lung cancer cells. Biosci Rep. (2019) 39:BSR20181298. doi: 10.1042/BSR20181298
27. Xiao B, Li J, Fan Y, Ye M, Lv S, Xu B, et al. Downregulation of SYT7 inhibits glioblastoma growth by promoting cellular apoptosis. Mol Med Rep. (2017) 16:9017–22. doi: 10.3892/mmr.2017.7723
28. Wu Z, Sun Z, Huang R, Zang D, Wang C, Yan X, et al. Silencing of synaptotagmin 7 regulates osteosarcoma cell proliferation, apoptosis, and migration. Histol Histopathol. (2020) 35:303–12. doi: 10.14670/HH-18-174
29. Fu Y, Tian G, Zhang Z, Yang X. SYT7 acts as an oncogene and a potential therapeutic target and was regulated by ΔNp63α in HNSCC. Cancer Cell Int. (2021) 21:696. doi: 10.1186/s12935-021-02394-w
30. Monterrat C, Boal F, Grise F, Hémar A, Lang J. Synaptotagmin 8 is expressed both as a calcium-insensitive soluble and membrane protein in neurons, neuroendocrine and endocrine cells. Biochim Biophys Acta. (2006) 1763:73–81. doi: 10.1016/j.bbamcr.2005.11.008
31. Fu Z, Liang X, Shi L, Tang L, Chen D, Liu A, et al. SYT8 promotes pancreatic cancer progression via the TNNI2/ERRα/SIRT1 signaling pathway. Cell Death Discov. (2021) 7:390. doi: 10.1038/s41420-021-00779-4
32. Kanda M, Murotani K, Tanaka H, Miwa T, Umeda S, Tanaka C, et al. A novel dual-marker expression panel for easy and accurate risk stratification of patients with gastric cancer. Cancer Med. (2018) 7:2463–71. doi: 10.1002/cam4.1522
33. Kanda M, Murotani K, Tanaka H, Miwa T, Umeda S, Tanaka C, et al. Integrated multigene expression panel to prognosticate patients with gastric cancer. Oncotarget. (2018) 9:18775–85. doi: 10.18632/oncotarget.24661
34. Kanda M, Shimizu D, Tanaka H, Tanaka C, Kobayashi D, Hayashi M, et al. Significance of SYT8 for the detection, prediction, and treatment of peritoneal metastasis from gastric cancer. Ann Surg. (2018) 267:495–503. doi: 10.1097/SLA.0000000000002096
35. Maximov A, Shin OH, Liu X, Südhof TC. Synaptotagmin-12, a synaptic vesicle phosphoprotein that modulates spontaneous neurotransmitter release. J Cell Biol. (2007) 176:113–24. doi: 10.1083/jcb.200607021
36. Kaeser-Woo YJ, Younts TJ, Yang X, Zhou P, Wu D, Castillo PE, et al. Synaptotagmin-12 phosphorylation by cAMP-dependent protein kinase is essential for hippocampal mossy fiber LTP. J Neurosci. (2013) 33:9769–80. doi: 10.1523/JNEUROSCI.5814-12.2013
37. Jonklaas J, Murthy S, Liu D, Klubo-Gwiezdzinska J, Krishnan J, Burman KD, et al. Novel biomarker SYT12 may contribute to predicting papillary thyroid cancer outcomes. Future Sci OA. (2018) 4:FSO249. doi: 10.4155/fsoa-2017-0087
38. Eizuka K, Nakashima D, Oka N, Wagai S, Takahara T, Saito T, et al. SYT12 plays a critical role in oral cancer and may be a novel therapeutic target. J Cancer. (2019) 10:4913–20. doi: 10.7150/jca.32582
39. Liu K, Luo J, Shao C, Ren Z, Sun S, Zhu Y, et al. Synaptotagmin 12 (SYT12) gene expression promotes cell proliferation and progression of lung adenocarcinoma and involves the phosphoinositide 3-kinase (PI3K)/AKT/mammalian target of rapamycin (mTOR) pathway. Med Sci Monit. (2020) 26:e920351. doi: 10.12659/MSM.920351
40. von Poser C, Südhof TC. Synaptotagmin 13: Structure and expression of a novel synaptotagmin. Eur J Cell Biol. (2001) 80:41–7. doi: 10.1078/0171-9335-00133
41. Tarquis-Medina M, Scheibner K, González-García I, Bastidas-Ponce A, Sterr M, Jaki J, et al. Synaptotagmin-13 is a neuroendocrine marker in brain, intestine and pancreas. Int J Mol Sci. (2021) 22:12526. doi: 10.3390/ijms222212526
42. Kanda M, Shimizu D, Tanaka H, Tanaka C, Kobayashi D, Hayashi M, et al. Synaptotagmin XIII expression and peritoneal metastasis in gastric cancer. Br J Surg. (2018) 105:1349–58. doi: 10.1002/bjs.10876
43. Nakanishi K, Kanda M, Umeda S, Tanaka C, Kobayashi D, Hayashi M, et al. The levels of SYT13 and CEA mRNAs in peritoneal lavages predict the peritoneal recurrence of gastric cancer. Gastric Cancer. (2019) 22:1143–52. doi: 10.1007/s10120-019-00967-3
44. Kanda M, Kasahara Y, Shimizu D, Miwa T, Umeda S, Sawaki K, et al. Amido-bridged nucleic acid-modified antisense oligonucleotides targeting SYT13 to treat peritoneal metastasis of gastric cancer. Mol Ther Nucleic Acids. (2020) 22:791–802. doi: 10.1016/j.omtn.2020.10.001
45. Li Q, Zhang S, Hu M, Xu M, Jiang X. Silencing of synaptotagmin 13 inhibits tumor growth through suppressing proliferation and promoting apoptosis of colorectal cancer cells. Int J Mol Med. (2020) 45:234–44. doi: 10.3892/ijmm.2019.4412
46. Keck KJ, Breheny P, Braun TA, Darbro B, Li G, Dillon JS, et al. Changes in gene expression in small bowel neuroendocrine tumors associated with progression to metastases. Surgery. (2018) 163:232–9. doi: 10.1016/j.surg.2017.07.031
47. Zhang L, Fan B, Zheng Y, Lou Y, Cui Y, Wang K, et al. Identification SYT13 as a novel biomarker in lung adenocarcinoma. J Cell Biochem. (2020) 121:963–73. doi: 10.1002/jcb.29224
48. Ichikawa T, Shibata M, Inaishi T, Soeda I, Kanda M, Hayashi M, et al. Synaptotagmin 13 is highly expressed in estrogen receptor-positive breast cancer. Curr Oncol. (2021) 28:4080–92. doi: 10.3390/curroncol28050346
49. Quintero-Rivera F, Chan A, Donovan DJ, Gusella JF, Ligon AH. Disruption of a synaptotagmin (SYT14) associated with neurodevelopmental abnormalities. Am J Med Genet A. (2007) 143A:558–63. doi: 10.1002/ajmg.a.31618
50. Doi H, Yoshida K, Yasuda T, Fukuda M, Fukuda Y, Morita H, et al. Exome sequencing reveals a homozygous SYT14 mutation in adult-onset, autosomal-recessive spinocerebellar ataxia with psychomotor retardation. Am J Hum Genet. (2011) 89:320–7. doi: 10.1016/j.ajhg.2011.07.012
51. Sheng B, Jiang Y, Wu D, Lai N, Ye Z, Zhang B, et al. RNAi-mediated SYT14 knockdown inhibits the growth of human glioma cell line U87MG. Brain Res Bull. (2018) 140:60–4. doi: 10.1016/j.brainresbull.2018.04.002
52. Fukuda M. Molecular cloning, expression, and characterization of a novel class of synaptotagmin (Syt XIV) conserved from Drosophila to humans. J Biochem. (2003) 133:641–9. doi: 10.1093/jb/mvg082
53. Chen J, Wang Z, Wang W, Ren S, Xue J, Zhong L, et al. SYT16 is a prognostic biomarker and correlated with immune infiltrates in glioma: A study based on TCGA data. Int Immunopharmacol. (2020) 84:106490. doi: 10.1016/j.intimp.2020.106490
54. Yang MF, Long XX, Hu HS, Bin YL, Chen XM, Wu BH, et al. Comprehensive analysis on the expression profile and prognostic values of synaptotagmins (SYTs) family members and their methylation levels in gastric cancer. Bioengineered. (2021) 12:3550–65. doi: 10.1080/21655979.2021.1951059
55. Kim J. In silico analysis of differentially expressed genesets in metastatic breast cancer identifies potential prognostic biomarkers. World J Surg Oncol. (2021) 19:188. doi: 10.1186/s12957-021-02301-7
56. Lánczky A, Gyõrffy B. Web-based survival analysis tool tailored for medical research (KMplot): Development and implementation. J Med Internet Res. (2021) 23:e27633. doi: 10.2196/27633
57. Nagy Á, Munkácsy G, Gyõrffy B. Pancancer survival analysis of cancer hallmark genes. Sci Rep. (2021) 11:6047. doi: 10.1038/s41598-021-84787-5
Keywords: synaptotagmin, cancer, overexpression, biomarker, prognosis, oncogene
Citation: Suo H, Xiao N and Wang K (2022) Potential roles of synaptotagmin family members in cancers: Recent advances and prospects. Front. Med. 9:968081. doi: 10.3389/fmed.2022.968081
Received: 16 June 2022; Accepted: 18 July 2022;
Published: 08 August 2022.
Edited by:
Luigi Tornillo, University of Basel, SwitzerlandReviewed by:
Renhao Xue, Tongji University, ChinaCopyright © 2022 Suo, Xiao and Wang. This is an open-access article distributed under the terms of the Creative Commons Attribution License (CC BY). The use, distribution or reproduction in other forums is permitted, provided the original author(s) and the copyright owner(s) are credited and that the original publication in this journal is cited, in accordance with accepted academic practice. No use, distribution or reproduction is permitted which does not comply with these terms.
*Correspondence: Kewei Wang, d2FuZ2tld2VpLTgzQDE2My5jb20=