- 1Department of Clinical Laboratory, The Second Hospital of Tianjin Medical University, Tianjin, China
- 2Institute of Infectious Diseases, The Second Hospital of Tianjin Medical University, Tianjin, China
- 3Department of Emergency Medicine, The Second Hospital of Tianjin Medical University, Tianjin, China
- 4Department of Cardiology, The Second Hospital of Tianjin Medical University, Tianjin, China
- 5Department of Clinical Laboratory, Yanda Hospital, Langfang, China
- 6Medical Security Center, The No. 983 Hospital of the Joint Service Support Force, Tianjin, China
Background: Hyperinflammation and coagulopathy are hallmarks of COVID-19 and synergistically contribute to illness progression. Antiplatelet agents have been proposed as candidate drugs for COVID-19 treatment on the basis of their antithrombotic and anti-inflammatory properties. A systematic review and meta-analysis that included early observational studies and recent randomized controlled trials (RCTs) was performed to summarize and compare evidence on this issue.
Methods: PubMed, Embase, and the Cochrane Central Register of Controlled Trials (CENTRAL) were searched to identify studies published up to Nov 7, 2021, and the results of registered clinical trials were followed up to Mar 30, 2022. We included RCTs and observational studies assessing the effect of antiplatelet therapy in adult patients with COVID-19. Data on baseline patient characteristics, interventions, controls, and outcomes were extracted by two independent reviewers. The primary outcome was mortality. Data were pooled using a random-effects model.
Results: Twenty-seven studies were included, of which 23 observational studies were pooled in a meta-analysis, and the remaining four RCTs (ACTIV-4B, RECOVERY, ACTIV-4a, and REMAP-CAP) were narratively synthesized. Based on 23 observational studies of 87,824 COVID-19 patients, antiplatelet treatment favors a lower risk of mortality [odds ratio (OR) 0.72, 95% confidence interval (CI) 0.61–0.85; I2 = 87.0%, P < 0.01]. The narrative synthesis of RCTs showed conflicting evidence, which did not support adding antiplatelet therapy to the standard care, regardless of the baseline illness severity and concomitant anticoagulation intensity.
Conclusion: While the rationale for using antiplatelet treatment in COVID-19 patients is compelling and was supported by the combined result of early observational studies, evidence from RCTs did not confirm this approach. Several factors that could explain this inconsistency were highlighted alongside perspectives on future research directions.
Introduction
Dysregulated inflammation and coagulopathy are hallmarks of severe COVID-19 and contribute to an increased risk of thromboembolic complications and mortality (1–4). Platelets are anucleate cell fragments derived from megakaryocytes that are not simply involved in thrombosis and immune response but also exert a hub function bridging these two processes as a new mechanism, termed immunothrombosis (5–7). The multifaceted role of platelets in immunothrombosis has been well-documented in previous literature and further highlighted in the current COVID-19 situation (8–11). A hyperactive platelet phenotype, as characterized by increased platelet surface markers [e.g., P-selectin, platelet Factor 4 (PF4), and CD40L], platelet-derived soluble mediators [e.g., thromboxane B2 (TxB2) and 5-hydroxytryptamine (5-HT)], and platelet homotypic and heterotypic aggregates, has been extensively identified in COVID-19 patients (9, 12–16). Likewise, data from transcriptome and proteome analyses indicate that platelet hyperactivity is a predominant cellular signature in response to SARS-CoV-2 infection (17, 18), thus suggesting a possible role of platelets in this novel viral disease. Moreover, activated platelets can trigger the formation of neutrophil extracellular traps (NETs) (19, 20), which have recently been recognized as pivotal players in thrombosis (21–23). Autopsy reports of COVID-19 patients revealed microthrombi with platelet and NET deposition in inflamed lung tissues, along with endothelial disruption (23–25).
Given the possible role of hyperactive platelets in the pathological mechanism of COVID-19, antiplatelet agents, such as aspirin and P2Y12 inhibitors, have been proposed as a potential treatment strategy for COVID-19 patients on the basis of their antithrombotic and anti-inflammatory properties (26–28). Additionally, significant thrombotic events have been observed despite anticoagulant treatment in clinical trials, implying that antiplatelet agents could be potential candidates for additional adjunctive antithrombotic therapy (29–31). In fact, an association between antiplatelet drug use and improved outcomes for COVID-19 patients has been reported in early observational studies (32–34). However, recently completed RCTs failed to confirm the effectiveness of antiplatelet treatment in preventing COVID-19 progression. While RCTs are considered to be more reliable than observational studies in evaluating interventions, the latter has helped us establish an initial foundation, which is particularly significant in an urgent situation (35). In the present circumstances, there is a need for findings to be assessed in the context of existing evidence in order to ensure reasonable interpretation of all studies (36). Here, we perform a systematic review and meta-analysis that included both RCTs and observational studies to provide an overview of existing evidence on antiplatelet therapy in patients with COVID-19. Furthermore, several study elements (e.g., baseline illness severity, the timing of antiplatelet therapy, and concomitant anticoagulation intensity) that might contribute to discrepancies among current lines of evidence and should be taken into consideration in future research are discussed.
Methods
This systematic review was performed following the PRISMA statement (37). The study protocol is provided in Supplementary material 1. Briefly, PubMed, Embase, and Cochrane CENTRAL were searched to identify studies published up to Nov 7, 2021, and the results of registered clinical trials were followed up to Mar 30, 2022. Details of the search strategies are provided in Supplementary material 1. The inclusion criteria were adult COVID-19 patients confirmed by laboratory testing, administration of antiplatelet therapy at any time or dose, comparison between patients with and without antiplatelet therapy, and availability of English or Chinese full texts. Studies involving patients with a particular illness or emergency conditions were excluded (e.g., cancer and pregnancy). When studies had significant overlapping data, the most comprehensive study was included.
The results of observational studies and RCTs were separately synthesized and compared (35). For pooled analysis, we selected all-cause mortality as the primary outcome for effect estimates. Considering that all the included studies for quantitative pooled analysis were retrospective in design, the odds ratio (OR) was used as the common measure of association across studies. Hazard ratios (HRs) and relative risks (RRs) were directly considered as ORs. A random-effect model was selected to account for clinical heterogeneity. Heterogeneity across studies was assessed using the Q statistic with its P-value and I2 statistic (38). Subgroup analyses were conducted to investigate variation in estimates according to original effect size, study center, illness severity, antiplatelet drugs, the timing of drug administration, and concomitant anticoagulant use. Sensitivity analysis was performed on the primary outcome by omitting one study at a time to assess the robustness of the results (39). A funnel plot was drawn to assess publication bias. The quality of the included observational studies for meta-analysis was evaluated following the Newcastle–Ottawa scale (NOS) by two independent reviewers (40). Studies with NOS scores of 8 or 9, 6 or 7, and < 6 were judged as having a low, medium, and high risk of bias, respectively. Discrepancies in data extraction and quality assessment were resolved through discussion with a third author. Statistical analyses were performed using RStudio software.
Results
Study identification
Our search yielded 1,228 records. After initial screening and full-text review, 23 observational studies (41–63) and 4 RCTs (64–67) (ACTIV-4B, RECOVERY, ACTIV-4a, and REMAP-CAP) were finally included for evidence synthesis and comparison (Figure 1). Observational studies were mostly performed in the first half of 2020, and RCTs were subsequently conducted between late 2020 and early 2021. For observational studies, the overall risk of bias was determined to be medium (Supplementary Table 1). Adjusted estimates could be determined for all observational studies even though the adjusted factors were slightly different. Among all studies, aspirin is the most common antiplatelet drug. Tables 1, 2 show details of the included observational studies and RCTs, respectively.
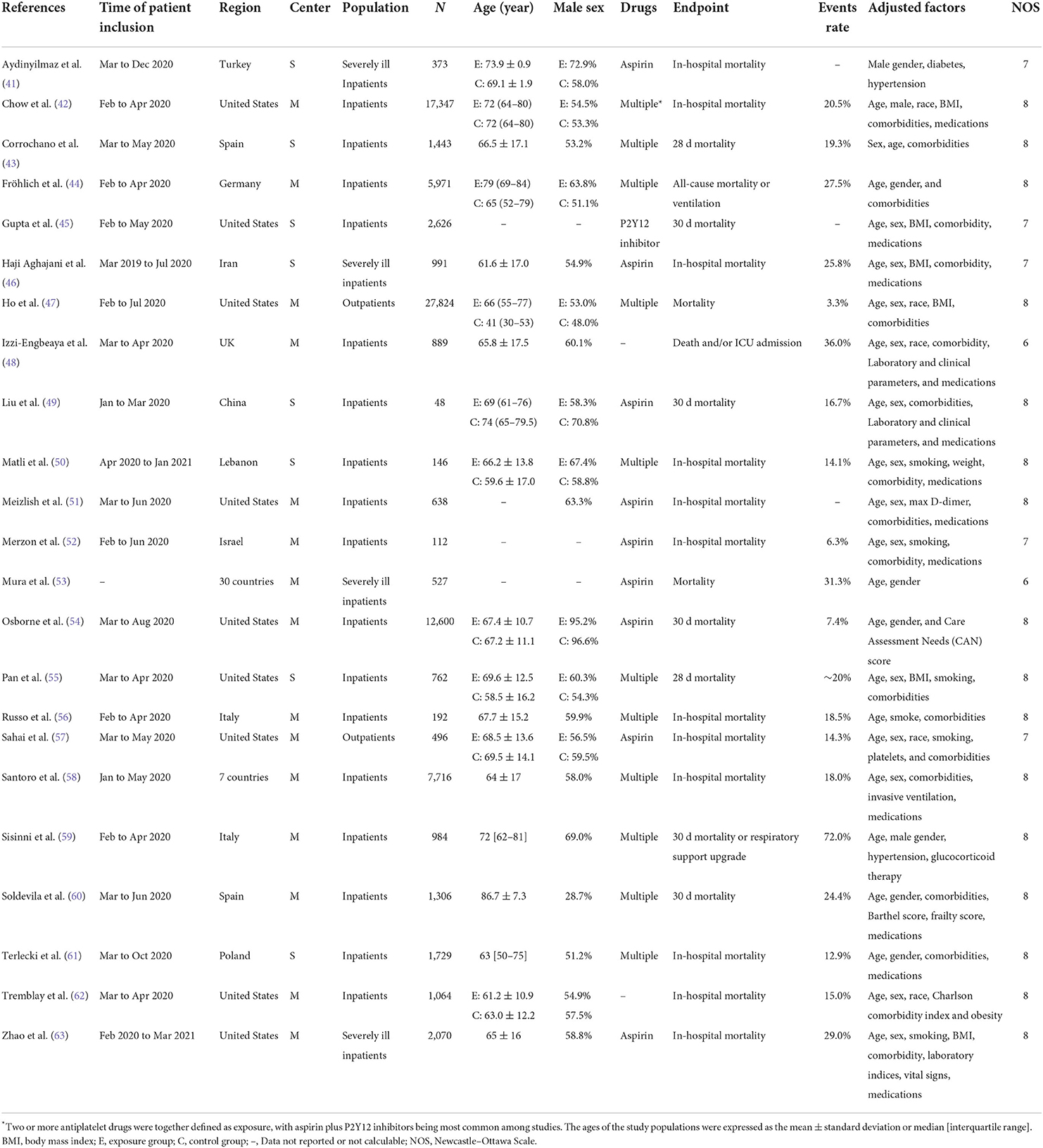
Table 1. Details of observational studies included in this meta-analysis of the association between antiplatelet treatment and mortality.
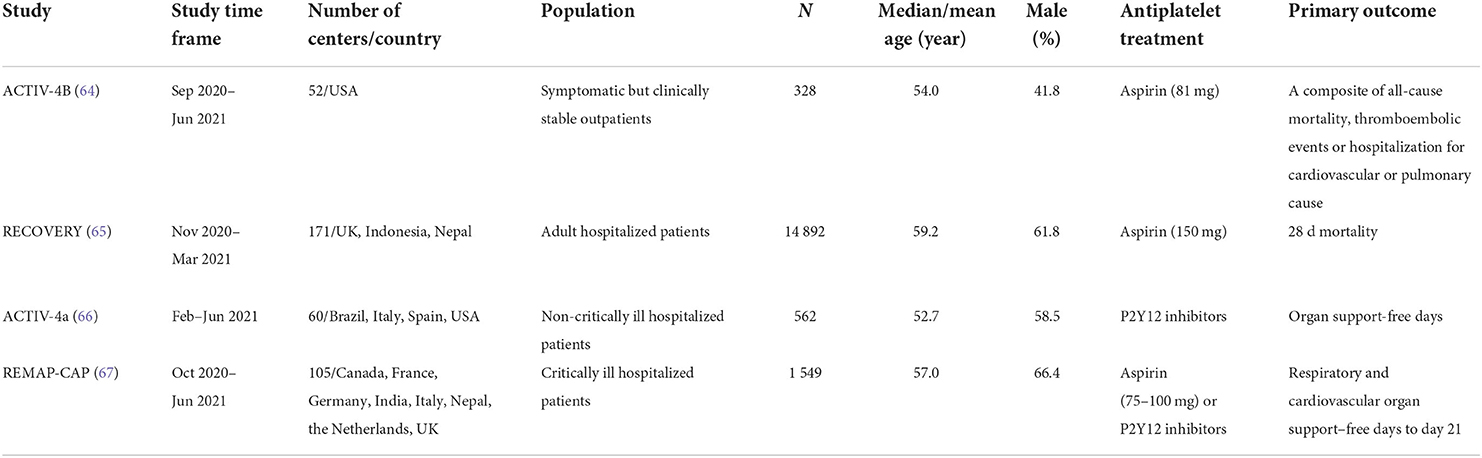
Table 2. Details of RCTs investigating the effect of antiplatelet treatment for patients with COVID-19.
Meta-analysis of observational studies
We first performed a meta-analysis of the 23 included observational studies and obtained a combined OR of 0.72 (95% CI: 0.61–0.85), suggesting that antiplatelet treatment favors a lower risk of mortality in patients with COVID-19 (Figure 2). Although significant heterogeneity was evident (P < 0.01, I2 = 87%), the combined estimates were consistent in the random- and fixed-effect models, and the sensitivity analysis suggested that our result was stable (Supplementary Figure 1), probably driven by a large number of participants (n = 87,824). To investigate the variation of combined evidence among observational studies and improve comparability with RCTs, pre-specified subgroup analyses were further conducted. All results of the subgroup analyses are summarized in Figure 3 (forest plots are shown in Supplementary Figures 2–7). The only significant antiplatelet treatment-covariate interaction identified in subgroup analyses was concomitant anticoagulant use, with OR = 0.64 (95% CI: 0.50–0.83) among patients with anticoagulant use (including partial use) and OR = 1.07 (95% CI: 0.94–1.21) among patients without anticoagulation treatment. There was no evidence to suggest a differential treatment effect for any other subgroups. The asymmetric shape of the funnel plot shows some evidence of publication bias among the evaluated studies (Supplementary Figure 8).
RCTs
Currently, one outpatient trial (ACTIV-4B) (64) and three inpatient trials (RECOVERY, ACTIV-4a, and REMAP-CAP) (65–67) have released their results. As there was obvious heterogeneity in the study population, antiplatelet treatment regimen, and concomitant anticoagulation intensity among these studies, their results were narratively synthesized (Table 2). The ACTIV-4B trial aimed to assess whether antiplatelet therapy (aspirin 81 mg) can safely reduce major adverse cardiopulmonary outcomes among symptomatic but clinically stable outpatients with COVID-19. The study was terminated early because of an event rate (0.7%) lower than anticipated and no evidence of efficacy when comparing aspirin with placebo.
RECOVERY (65) is the current largest randomized study investigating the effect of antiplatelet therapy in COVID-19, with 14,892 participants from 171 centers. This study found that in a mixed population of patients with mild, moderate, and severe COVID-19, adding 150 mg aspirin to standard care did not reduce 28-day mortality [relative risk (RR) = 0.96, 95% CI: 0.89–1.04] or the probability of progression to the composite of invasive mechanical ventilation or death (RR = 0.96; 95% CI: 0.90–1.03).
In recently completed multiplatform trials (ATTACC, ACTIV-4a, and REMAP-CAP), therapeutic-dose heparin vs. conventional thromboprophylaxis has been found to improve organ support-free days in hospitalized non-critically ill patients (30) but is not beneficial for critically ill patients (29). Subsequently, the ACTIV-4a trial (66) tested whether the addition of a P2Y12 inhibitor to anticoagulant therapy would further change clinical outcomes in non-critically ill patients hospitalized for COVID-19. After 562 patients completed the trial, no significant differences were found in the primary outcome (the composite of organ support-free days evaluated on an ordinal scale combined with in-hospital death) or in the secondary outcome (the composite of major thrombotic events or death by 28 days).
In parallel with ACTIV-4a, the REMAP-CAP trial (67) aimed to examine the add-on effect of antiplatelet therapy [aspirin, 75–100 mg; n = 565 or P2Y12 inhibitors (clopidogrel, 75 mg; ticagrelor, 60 mg; or prasugrel, 60 mg); n = 455] alongside prophylactic dose anticoagulation in severe COVID-19 patients. First, this trial observed equivalence between the aspirin and P2Y12 inhibitor groups (OR = 1.00; 95% CI, 0.8–1.27; >90% posterior probability of equivalence). In a subsequent adaptive pooled analysis of the two antiplatelet treatment groups in comparison with controls, the median for organ support-free days was 7 in both the antiplatelet and control groups (median-adjusted OR = 1.02; 95% CI, 0.86–1.23; 95.7% posterior probability of futility). Although a modest benefit on the secondary endpoint of 90-day mortality was determined (HR = 1.22; 95% CI, 1.06–1.40; 99.7% posterior probability of efficacy), the median number of organ support-free days was again equal (14 days) among survivors in both groups. Additionally, the authors reported a small but certain increased risk of major bleeding in the antiplatelet group (2.1 vs. 0.4%; adjusted OR = 2.97; 95% CI, 1.23–8.28; 99.4% probability of harm).
Discussion
This systematic review summarized and compared current evidence regarding antiplatelet treatment for patients with COVID-19. The combined effect estimates of observational studies suggested that antiplatelet therapy favors a lower risk of mortality, and the results were consistent in all pre-specified subgroup analyses in addition to those based on anticoagulant use. Nevertheless, subsequent RCTs did not confirm this association. A series of well-conducted randomized studies found no additional effect when adding antiplatelet therapy to standard care, regardless of baseline illness severity and concomitant anticoagulation intensity. The reason for this inconsistency may be multiple. First, since all observational studies included for pooled analysis were retrospective in design, selection bias might have occurred in the selection of exposed subjects according to an antecedent prescription of antiplatelet medication. Additionally, while adjusted estimates could be determined for all studies, potential cofounding associated with both exposure and outcome cannot be definitively excluded (68, 69).
In addition to the limitations ascribed to the study design per se, another noteworthy factor is the timing of antiplatelet treatment. For most observational studies (41–45, 47, 52, 54–57, 59–62) included for evidence synthesis, antiplatelet therapy was initiated before COVID-19 diagnosis in contrast to during hospitalization in RCTs (65–67). Possibly, the baseline suboptimal platelet reactivity due to prior chronic antiplatelet therapy restrains illness progression and aggravation. At the time of hospitalization because of moderate or severe illness, platelet activation may have already reached a maximum level, for which antiplatelet treatment is too late (66). Additionally, as mentioned above, the rationale for antiplatelet medication in COVID-19 is based on the antithrombotic and anti-inflammatory properties. However, there is evidence that the distribution of platelets is not limited only to intravascular compartments but also to alveolar translocation (70–73). Moreover, platelets differentially bind to neutrophils and Treg cells at distinct time points to orchestrate both the initiation and resolution of pulmonary inflammation. These interactions prevent excessive lung damage after infection (70). In sum, platelets still offer a candidate treatment target for infection-related thrombosis, yet the treatment timing may be of great relevance and warrant further investigation at the clinical level.
Among currently completed RCTs, ACTIV-4B is the only outpatient trial. In addition to its negative finding, this trial also reported a markedly low rate of events (a composite of all-cause mortality, thromboembolism, myocardial infarction, stroke, or hospitalization for cardiovascular or pulmonary cause), namely, 0.7% among the study populations, which is much lower than that reported by early epidemiological data (64). The significant decline in adverse event incidence among mildly ill outpatient populations could partially be attributed to aggressive vaccination and progress in medical care since the pandemic outbreak (74, 75). Correspondingly, the recently updated COVID-19 treatment guidelines recommend against the use of anticoagulants and antiplatelet therapy in the outpatient setting, unless the patient has other indications for the therapy (76). Another ongoing trial (OLA COVID; NCT04937088) (77) that tests whether a novel, liquid aspirin formulation can reduce COVID-19-related hospitalizations in old populations will provide more evidence on this issue.
For hospitalized patients with COVID-19, thrombotic complications have been reported to be common despite conventional thromboprophylaxis and therapeutic anticoagulation (31). In this setting, the RECOVERY, ACTIV-4a, and REMAP-CAP trials sought to examine the additional effect and risk of antiplatelet treatment on the basis of thromboprophylaxis and anticoagulation therapy. Overall, the results of these well-conducted trials found no additional effect when antiplatelet therapy was added to anticoagulation (mostly Low-molecular-weight heparin, LMWH) in hospitalized patients with COVID-19, despite a slightly increased risk of bleeding. The reason for these negative results is not obvious. A possible explanation is that the anticipated antithrombotic effect of antiplatelet drugs was partially masked by LMWH (78).
While the mechanism of COVID-19-related coagulopathy has not yet been elucidated, a major cause is tissue factor overexpression on the surface of damaged endothelial cells and immune cells, which further initiates coagulation cascades and leads to thrombin generation (8). This opinion can be supported by anticoagulation trials that found the superiority of heparin/LMWH by targeting thrombin. However, thrombin is not only a central enzyme involved in coagulation cascades but also a potent inducer of platelet activation (78, 79). In a more recent study, the TF/thrombin pathway was found to be pivotal for platelet activation in an ex vivo SARS-CoV-2 infection model (80). The authors concluded that TF activity from SARS-CoV-2-infected cells activates thrombin, which signals to protease-activated receptors (PARs) on platelets (80). Taken together, it is plausible to speculate that the key upstream pathway that promotes platelet activation during SARS-CoV-2 infection is inhibited by heparin through disturbing thrombin ligation to platelet Glycoprotein Ib (GP Ib) and PARs (78, 80), whereby the anticipated antithrombotic effects of aspirin and P2Y12 inhibitors in the above trials were diluted.
To date, our successful experience in the combined use of heparin and antiplatelet agents is almost confined to thrombotic disease, with most valid evidence in arterial thrombosis, yet, under the premise that antiplatelet treatment per se is effective (81). Whether antiplatelet therapy alone can prevent illness progression for hospitalized patients with COVID-19 is still unclear. This question is difficult to clarify in future trials, as it is unethical to abrogate proven beneficial anticoagulation for patients to measure the effect of single antiplatelet therapy. Alternatively, early observational studies could shine a light on this issue. In the subgroup analysis of observational studies by anticoagulant intensity, we identified four studies (43, 44, 47, 62) including 36,302 patients without anticoagulant use. The combined OR of 1.07 (95% CI, 0.94–1.21; I2 = 0%, P = 0.32) suggested that single antiplatelet treatment is not associated with lower mortality (see Figure 3). However, this result may be limited by the lack of sufficient direct comparisons and should be regarded with extreme caution. In contrast to targeting platelets per se, there is an ongoing arm of the ACTIV-4a trial that aims to test whether inhibiting the cross-talk between platelets and immune as well as endothelial cells, by using Crizanlizumab (82, 83) or sodium-glucose cotransporter-2 (SGLT2) inhibitor (84), will further improve the hypercoagulable state of patients with COVID-19, and the results are eagerly anticipated.
Conclusion
This paper provides an overview of existing evidence on antiplatelet therapy for patients with COVID-19. In summary, while the rationale for using antiplatelet drugs to prevent COVID-19 progression is compelling and was supported by combined evidence from early observational studies, recently completed RCTs do not support this approach. The consistent negative results of such RCTs have supplied more valid evidence against adding antiplatelet therapy to standard care for COVID-19 patients in either community or hospital settings. In terms of directions for future study, the optimal antithrombotic regimen for patients with COVID-19 should be individualized (85) and guided by biomarkers, such as urinary 11-dehydrothromboxane B2, platelet reactivity, platelet-platelet aggregates, and platelet-leukocyte aggregates detected by new microscopic techniques (16, 31). Moreover, several factors that could explain the inconsistency among the current evidence and advocate for further investigation were highlighted in the current review.
Data availability statement
The datasets used during the current study are available from the corresponding author upon reasonable request.
Author contributions
XZ contributed to the conception and design of the study, acquisition of data, analysis and interpretation of the data, and drafting of the manuscript. ZL, WW, DW, and ZC reviewed and revised the manuscript. XW, YL, and ZL contributed to the acquisition as well as to the analysis and interpretation of the data. All authors gave final approval and agree to be accountable for all aspects of the work ensuring integrity and accuracy.
Funding
This work was supported by the Tianjin Health Science and Technology Project (KJ20092), the Youth Training Program, the Second Hospital of Tianjin Medical University (2019ydey28), Tianjin Health and Family Planning Industry Young Medical Talents Project, Integrated Chinese and Western Medicine Project (2021207), and Hebei Health Science and Technology Projects (20191066 and 20191061).
Conflict of interest
The authors declare that the research was conducted in the absence of any commercial or financial relationships that could be construed as a potential conflictof interest.
Publisher's note
All claims expressed in this article are solely those of the authors and do not necessarily represent those of their affiliated organizations, or those of the publisher, the editors and the reviewers. Any product that may be evaluated in this article, or claim that may be made by its manufacturer, is not guaranteed or endorsed by the publisher.
Supplementary material
The Supplementary Material for this article can be found online at: https://www.frontiersin.org/articles/10.3389/fmed.2022.965790/full#supplementary-material
Abbreviations
5-HT, 5-Hydroxytryptamine; CI, Confidence intervals; COVID-19, Coronavirus Disease; GP Ib, Glycoprotein Ib; HR, Hazard ratio; LMWH, Low-molecular-weight heparin; NETs, Neutrophil extracellular traps; NOS, Newcastle–Ottawa Scale; OR, Odds ratio; PARs, Protease-activated receptors; PF4, Platelet Factor 4; RCTs, Randomized controlled trials; RR, Relative risk; SARS-CoV-2, Severe acute respiratory syndrome coronavirus 2; SGLT2, Sodium-glucose cotransporter-2; TxB2, Thromboxane B2.
References
1. Consortium. A blood atlas of COVID-19 defines hallmarks of disease severity and specificity. Cell. (2022) 185:916–38.e58. doi: 10.1016/j.cell.2022.01.012
2. Lazzaroni MG, Piantoni S, Masneri S, Garrafa E, Martini G, Tincani A, et al. Coagulation dysfunction in COVID-19: the interplay between inflammation, viral infection and the coagulation system. Blood Rev. (2021) 46:100745. doi: 10.1016/j.blre.2020.100745
3. Lamers MM, Haagmans BL. SARS-CoV-2 pathogenesis. Nat Rev Microbiol. (2022) 20:270–84. doi: 10.1038/s41579-022-00713-0
4. Bikdeli B, Madhavan MV, Jimenez D, Chuich T, Dreyfus I, Driggin E, et al. COVID-19 and thrombotic or thromboembolic disease: implications for prevention, antithrombotic therapy, and follow-up: JACC state-of-the-art review. J Am Coll Cardiol. (2020) 75:2950–73. doi: 10.1016/j.jacc.2020.04.031
5. Van Der Meijden PEJ, Heemskerk JWM. Platelet biology and functions: new concepts and clinical perspectives. Nat Rev Cardiol. (2019) 16:166–79. doi: 10.1038/s41569-018-0110-0
6. Martinod K, Deppermann C. Immunothrombosis and thromboinflammation in host defense and disease. Platelets. (2021) 32:314–24. doi: 10.1080/09537104.2020.1817360
7. Patel P, Michael JV, Naik UP, Mckenzie SE. Platelet FcγRIIA in immunity and thrombosis: adaptive immunothrombosis. J Thromb Haemost. (2021) 19:1149–60. doi: 10.1111/jth.15265
8. Iba T, Levy JH, Connors JM, Warkentin TE, Thachil J, Levi M. The unique characteristics of COVID-19 coagulopathy. Critical Care. (2020) 24:3077. doi: 10.1186/s13054-020-03077-0
9. Taus F, Salvagno G, Canè S, Fava C, Mazzaferri F, Carrara E, et al. Platelets promote thromboinflammation in SARS-CoV-2 pneumonia. Arterioscler Thromb Vasc Biol. (2020) 40:2975–89. doi: 10.1161/ATVBAHA.120.315175
10. Iffah R, Gavins FNE. Thromboinflammation in coronavirus disease 2019: the clot thickens. Br J Pharmacol. (2021). doi: 10.1111/bph.15594
11. Zong X, Gu Y, Yu H, Li Z, Wang Y. Thrombocytopenia is associated with COVID-19 severity and outcome: an updated meta-analysis of 5,637 patients with multiple outcomes. Lab Med. (2021) 52:10–5. doi: 10.1093/labmed/lmaa067
12. Barrett TJ, Lee AH, Xia Y, Lin LH, Black M, Cotzia P, et al. Platelet and vascular biomarkers associate with thrombosis and death in coronavirus disease. Circ Res. (2020) 127:945–7. doi: 10.1161/CIRCRESAHA.120.317803
13. Goshua G, Pine AB, Meizlish ML, Chang CH, Zhang H, Bahel P, et al. Endotheliopathy in COVID-19-associated coagulopathy: evidence from a single-centre, cross-sectional study. Lancet Haematol. (2020) 7:e575–82. doi: 10.1016/S2352-3026(20)30216-7
14. Zaid Y, Puhm F, Allaeys I, Naya A, Oudghiri M, Khalki L, et al. Platelets can associate with SARS-CoV-2 RNA and are hyperactivated in COVID-19. Circ Res. (2020) 127:1404–18. doi: 10.1161/CIRCRESAHA.120.317703
15. Petrey AC, Qeadan F, Middleton EA, Pinchuk IV, Campbell RA, Beswick EJ. Cytokine release syndrome in COVID-19: innate immune, vascular, and platelet pathogenic factors differ in severity of disease and sex. J Leukoc Biol. (2021) 109:55–66. doi: 10.1002/JLB.3COVA0820-410RRR
16. Gorog DA, Storey RF, Gurbel PA, Tantry US, Berger JS, Chan MY, et al. Current and novel biomarkers of thrombotic risk in COVID-19: a consensus statement from the International COVID-19 Thrombosis Biomarkers Colloquium. Nat Rev Cardiol. (2022). doi: 10.1038/s41569-021-00665-7
17. Manne BK, Denorme F, Middleton EA, Portier I, Rowley JW, Stubben C, et al. Platelet gene expression and function in patients with COVID-19. Blood. (2020) 136:1317–29. doi: 10.1182/blood.2020007214
18. Shen B, Yi X, Sun Y, Bi X, Du J, Zhang C, et al. Proteomic and metabolomic characterization of COVID-19 patient sera. Cell. (2020) 182:59–72 e15. doi: 10.1016/j.cell.2020.05.032
19. Clark SR, Ma AC, Tavener SA, Mcdonald B, Goodarzi Z, Kelly MM, et al. Platelet TLR4 activates neutrophil extracellular traps to ensnare bacteria in septic blood. Nat Med. (2007) 13:463–9. doi: 10.1038/nm1565
20. Mcdonald B, Davis RP, Kim SJ, Tse M, Esmon CT, Kolaczkowska E, et al. Platelets and neutrophil extracellular traps collaborate to promote intravascular coagulation during sepsis in mice. Blood. (2017) 129:1357–67. doi: 10.1182/blood-2016-09-741298
21. Jiménez-Alcázar M, Rangaswamy C, Panda R, Bitterling J, Simsek YJ, Long AT, et al. Host DNases prevent vascular occlusion by neutrophil extracellular traps. Science. (2017) 358:1202–6. doi: 10.1126/science.aam8897
22. Perdomo J, Leung HHL, Ahmadi Z, Yan F, Chong JJH, Passam FH, et al. Neutrophil activation and NETosis are the major drivers of thrombosis in heparin-induced thrombocytopenia. Nat Commun. (2019) 10:1322. doi: 10.1038/s41467-019-09160-7
23. Middleton EA, He XY, Denorme F, Campbell RA, Ng D, Salvatore SP, et al. Neutrophil extracellular traps contribute to immunothrombosis in COVID-19 acute respiratory distress syndrome. Blood. (2020) 136:1169–79. doi: 10.1182/blood.2020007008
24. Leppkes M, Knopf J, Naschberger E, Lindemann A, Singh J, Herrmann I, et al. Vascular occlusion by neutrophil extracellular traps in COVID-19. EBioMedicine. (2020) 58:102925. doi: 10.1016/j.ebiom.2020.102925
25. Gu SX, Tyagi T, Jain K, Gu VW, Lee SH, Hwa JM, et al. Thrombocytopathy and endotheliopathy: crucial contributors to COVID-19 thromboinflammation. Nat Rev Cardiol. (2021) 18:194–209. doi: 10.1038/s41569-020-00469-1
26. Bikdeli B, Madhavan MV, Gupta A, Jimenez D, Burton JR, Der Nigoghossian C, et al. Pharmacological agents targeting thromboinflammation in COVID-19: review and implications for future research. Thromb Haemost. (2020) 120:1004–24. doi: 10.1055/s-0040-1713152
27. Gurbel PA, Bliden KP, Schrör K. Can an old ally defeat a new enemy? Circulation. (2020) 142:315–7. doi: 10.1161/CIRCULATIONAHA.120.047830
28. Tantry US, Bliden KP, Gurbel PA. Further evidence for the use of aspirin in COVID-19. Int J Cardiol. (2022) 346:107–8. doi: 10.1016/j.ijcard.2021.11.021
29. Goligher EC, Bradbury CA, Mcverry BJ, Lawler PR, Berger JS, Gong MN, et al. Therapeutic anticoagulation with heparin in critically ill patients with covid-19. N Engl J Med. (2021) 385:777–89. doi: 10.1056/NEJMoa2103417
30. Lawler PR, Goligher EC, Berger JS, Neal MD, Mcverry BJ, Nicolau JC, et al. Therapeutic anticoagulation with heparin in noncritically ill patients with covid-19. N Engl J Med. (2021) 385:790–802. doi: 10.1056/NEJMoa2105911
31. Denorme F, Ajanel A, Campbell RA. Shining a light on platelet activation in COVID-19. J Thromb Haemost. (2022) 20:1286–9. doi: 10.1111/jth.15678
32. Kow CS, Hasan SS. Use of antiplatelet drugs and the risk of mortality in patients with COVID-19: a meta-analysis. J Thromb Thrombolysis. (2021) 52:124–9. doi: 10.1007/s11239-021-02436-0
33. Martha JW, Pranata R, Lim MA, Wibowo A, Akbar MR. Active prescription of low-dose aspirin during or prior to hospitalization and mortality in COVID-19: a systematic review and meta-analysis of adjusted effect estimates. Int J Infect Dis. (2021) 108:6–12. doi: 10.1016/j.ijid.2021.05.016
34. Srivastava R, Kumar A. Use of aspirin in reduction of mortality of COVID-19 patients: a meta-analysis. Int J Clin Pract. (2021) 75:e14515. doi: 10.1111/ijcp.14515
35. Bun R-S, Scheer J, Guillo S, Tubach F, Dechartres A. Meta-analyses frequently pooled different study types together: a meta-epidemiological study. J Clin Epidemiol. (2020) 118:18–28. doi: 10.1016/j.jclinepi.2019.10.013
36. Khaw KT, Day N, Bingham S, Wareham N. Observational versus randomised trial evidence. Lancet. (2004) 364:753–4. doi: 10.1016/S0140-6736(04)16924-9
37. Moher D, Liberati A, Tetzlaff J, Altman DG, Group P. Preferred reporting items for systematic reviews and meta-analyses: the PRISMA statement. BMJ. (2009) 339:b2535. doi: 10.1136/bmj.b2535
38. Higgins JP, Thompson SG, Deeks JJ, Altman DG. Measuring inconsistency in meta-analyses. BMJ. (2003) 327:557–60. doi: 10.1136/bmj.327.7414.557
39. Deeks JJ, Higgins JPT, Altman DG. Analysing Data and Undertaking Meta-analyses Cochrane Handbook for Systematic Reviews of Interventions. Chichester: John Wiley & Sons, Ltd (2019). p. 241–84. doi: 10.1002/9781119536604.ch10
40. Wells G, Shea B, O'connell J. The Newcastle-Ottawa Scale (NOS) for Assessing The Quality of Nonrandomised Studies in Meta-analyses. Ottawa, ON: Ottawa Health Research Institute (2014). p. 7.
41. Aydinyilmaz F, Aksakal E, Pamukcu HE, Aydemir S, Dogan R, Saraç I, et al. Significance of MPV, RDW and PDW with the severity and mortality of COVID-19 and effects of acetylsalicylic acid use. Clin Appl Thromb Hemost. (2021) 27:10760296211048808. doi: 10.1177/10760296211048808
42. Chow JH, Yin Y, Yamane DP, Davison D, Keneally RJ, Hawkins K, et al. Association of prehospital antiplatelet therapy with survival in patients hospitalized with COVID-19: a propensity score-matched analysis. J Thromb Haemost. (2021) 19:2814–24. doi: 10.1111/jth.15517
43. Corrochano M, Acosta-Isaac R, Mojal S, Miqueleiz S, Rodriguez D, Quijada-Manuitt M, et al. Impact of pre-admission antithrombotic therapy on disease severity and mortality in patients hospitalized for COVID-19. J Thromb Thrombolysis. (2021) 2:1–7. doi: 10.1007/s11239-021-02507-2
44. Fröhlich GM, Jeschke E, Eichler U, Thiele H, Alhariri L, Reinthaler M, et al. Impact of oral anticoagulation on clinical outcomes of COVID-19: a nationwide cohort study of hospitalized patients in Germany. Clin Res Cardiol. (2021) 110:1041–50. doi: 10.1007/s00392-020-01783-x
45. Gupta A, Madhavan MV, Poterucha TJ, Defilippis EM, Hennessey JA, Redfors B, et al. Association between antecedent statin use and decreased mortality in hospitalized patients with COVID-19. Nat Commun. (2021) 12:1325. doi: 10.1038/s41467-021-21553-1
46. Haji Aghajani M, Moradi O, Amini H, Azhdari Tehrani H, Pourheidar E, Rabiei MM, et al. Decreased in-hospital mortality associated with aspirin administration in hospitalized patients due to severe COVID-19. J Med Virol. (2021) 93:5390–5. doi: 10.1002/jmv.27053
47. Ho G, Dusendang JR, Schmittdiel J, Kavecansky J, Tavakoli J, Pai A. Association of chronic anticoagulant and antiplatelet use on disease severity in SARS-CoV-2 infected patients. J Thromb Thrombolysis. (2021) 52:476–81. doi: 10.1007/s11239-021-02383-w
48. Izzi-Engbeaya C, Distaso W, Amin A, Yang W, Idowu O, Kenkre JS, et al. Adverse outcomes in COVID-19 and diabetes: a retrospective cohort study from three London teaching hospitals. BMJ Open Diabetes Res Care. (2021) 9:1858. doi: 10.1136/bmjdrc-2020-001858
49. Liu Q, Huang N, Li A, Zhou Y, Liang L, Song X, et al. Effect of low-dose aspirin on mortality and viral duration of the hospitalized adults with COVID-19. Medicine. (2021) 100:e24544. doi: 10.1097/MD.0000000000024544
50. Matli K, Chamoun N, Fares A, Zibara V, Al-Osta S, Nasrallah R, et al. Combined anticoagulant and antiplatelet therapy is associated with an improved outcome in hospitalised patients with COVID-19: a propensity matched cohort study. Open Heart. (2021) 8:1785. doi: 10.1136/openhrt-2021-001785
51. Meizlish ML, Goshua G, Liu Y, Fine R, Amin K, Chang E, et al. Intermediate-dose anticoagulation, aspirin, and in-hospital mortality in COVID-19: a propensity score-matched analysis. Am J Hematol. (2021) 96:471–9. doi: 10.1002/ajh.26102
52. Merzon E, Green I, Vinker S, Golan-Cohen A, Gorohovski A, Avramovich E, et al. The use of aspirin for primary prevention of cardiovascular disease is associated with a lower likelihood of COVID-19 infection. FEBS J. (2021) 288:5179–89. doi: 10.1111/febs.15784
53. Mura C, Preissner S, Nahles S, Heiland M, Bourne PE, Preissner R. Real-world evidence for improved outcomes with histamine antagonists and aspirin in 22,560 COVID-19 patients. Signal Transduct Target Ther. (2021) 6:267. doi: 10.1038/s41392-021-00689-y
54. Osborne TF, Veigulis ZP, Arreola DM, Mahajan SM, Roosli E, Curtin CM. Association of mortality and aspirin prescription for COVID-19 patients at the Veterans Health Administration. PLoS ONE. (2021) 16:246825. doi: 10.1371/journal.pone.0246825
55. Pan D, Ip A, Zhan S, Wasserman I, Snyder DJ, Agathis AZ, et al. Pre-hospital antiplatelet medication use on COVID-19 disease severity. Heart Lung. (2021) 50:618–21. doi: 10.1016/j.hrtlng.2021.04.010
56. Russo V, Di Maio M, Attena E, Silverio A, Scudiero F, Celentani D, et al. Clinical impact of pre-admission antithrombotic therapy in hospitalized patients with COVID-19: a multicenter observational study. Pharmacol Res. (2020) 159:104965. doi: 10.1016/j.phrs.2020.104965
57. Sahai A, Bhandari R, Godwin M, Mcintyre T, Chung MK, Iskandar JP, et al. Effect of aspirin on short-term outcomes in hospitalized patients with COVID-19. Vasc Med. (2021) 2021:1358863x211012754. doi: 10.1177/1358863X211012754
58. Santoro F, Nuñez-Gil IJ, Vitale E, Viana-Llamas MC, Reche-Martinez B, Romero-Pareja R, et al. Antiplatelet therapy and outcome in COVID-19: the Health Outcome Predictive Evaluation Registry. Heart. (2021) 2021:319552. doi: 10.1136/heartjnl-2021-319552
59. Sisinni A, Rossi L, Battista A, Poletti E, Battista F, Battista RA, et al. Pre-admission acetylsalicylic acid therapy and impact on in-hospital outcome in COVID-19 patients: the ASA-CARE study. Int J Cardiol. (2021) 344:240–5. doi: 10.1016/j.ijcard.2021.09.058
60. Soldevila L, Valerio-Sallent L, Roure S, Pérez-Quílez O, Mas M, Miralles R, et al. Drug exposure may have a substantial influence on COVID-19 prognosis among residents of long-term care facilities: an exploratory analysis. Int J Infect Dis. (2021) 109:192–4. doi: 10.1016/j.ijid.2021.07.007
61. Terlecki M, Wojciechowska W, Klocek M, Olszanecka A, Stolarz-Skrzypek K, Grodzicki T, et al. Association between cardiovascular disease, cardiovascular drug therapy, and in-hospital outcomes in patients with COVID-19: data from a large single-center registry in Poland. Kardiol Pol. (2021) 79:773–80. doi: 10.33963/KP.15990
62. Tremblay D, Van Gerwen M, Alsen M, Thibaud S, Kessler A, Venugopal S, et al. Impact of anticoagulation prior to COVID-19 infection: a propensity score-matched cohort study. Blood. (2020) 136:144–7. doi: 10.1182/blood.2020006941
63. Zhao X, Gao C, Dai F, Treggiari MM, Deshpande R, Meng L. Treatments associated with lower mortality among critically ill COVID-19 patients. Anesthesiology. (2021) 2021:3999. doi: 10.1097/ALN.0000000000003999
64. Connors JM, Brooks MM, Sciurba FC, Krishnan JA, Bledsoe JR, Kindzelski A, et al. Effect of antithrombotic therapy on clinical outcomes in outpatients with clinically stable symptomatic COVID-19: the ACTIV-4B randomized clinical trial. J Am Med Assoc. (2021) 326:1703–12. doi: 10.1001/jama.2021.17272
65. Abani O, Abbas A, Abbas F, Abbas M, Abbasi S, Abbass H, et al. Aspirin in patients admitted to hospital with COVID-19 (RECOVERY): a randomised, controlled, open-label, platform trial. Lancet. (2022) 399:143–51. doi: 10.1016/S0140-6736(21)01825-0
66. Berger JS, Kornblith LZ, Gong MN, Reynolds HR, Cushman M, Cheng Y, et al. Effect of P2Y12 inhibitors on survival free of organ support among non-critically ill hospitalized patients with COVID-19: a randomized clinical trial. J Am Med Assoc. (2022) 327:227–36. doi: 10.1001/jama.2021.23605
67. Investigators R-CWCFTR-C, Bradbury CA, Lawler PR, Stanworth SJ, Mcverry BJ, Mcquilten Z, et al. Effect of antiplatelet therapy on survival and organ support-free days in critically ill patients with COVID-19: a randomized clinical trial. J Am Med Assoc. (2022) 327:1247–59. doi: 10.1001/jama.2022.2910
68. Anglemyer A, Horvath HT, Bero L. Healthcare outcomes assessed with observational study designs compared with those assessed in randomized trials. Cochr Database Systemat Rev. (2014) 2014:MR000034. doi: 10.1002/14651858.MR000034.pub2
69. Sedgwick P. Retrospective cohort studies: advantages and disadvantages. BMJ. (2014) 348:g1072. doi: 10.1136/bmj.g1072
70. Rossaint J, Thomas K, Mersmann S, Skupski J, Margraf A, Tekath T, et al. Platelets orchestrate the resolution of pulmonary inflammation in mice by T reg cell repositioning and macrophage education. J Exp Med. (2021) 218:jem.20201353. doi: 10.1084/jem.20201353
71. Ortiz-Muñoz G, Mallavia B, Bins A, Headley M, Krummel MF, Looney MR. Aspirin-triggered 15-epi-lipoxin A4 regulates neutrophil-platelet aggregation and attenuates acute lung injury in mice. Blood. (2014) 124:2625–34. doi: 10.1182/blood-2014-03-562876
72. Middleton EA, Weyrich AS, Zimmerman GA. Platelets in pulmonary immune responses and inflammatory lung diseases. Physiol Rev. (2016) 96:1211–59. doi: 10.1152/physrev.00038.2015
73. Amison RT, O'shaughnessy BG, Arnold S, Cleary SJ, Nandi M, Pitchford SC, et al. Platelet depletion impairs host defense to pulmonary infection with Pseudomonas aeruginosa in mice. Am J Respir Cell Mol Biol. (2018) 58:331–40. doi: 10.1165/rcmb.2017-0083OC
74. Fiolet T, Kherabi Y, Macdonald CJ, Ghosn J, Peiffer-Smadja N. Comparing COVID-19 vaccines for their characteristics, efficacy and effectiveness against SARS-CoV-2 and variants of concern: a narrative review. Clin Microbiol Infect. (2022) 28:202–21. doi: 10.1016/j.cmi.2021.10.005
75. Tregoning JS, Flight KE, Higham SL, Wang Z, Pierce BF. Progress of the COVID-19 vaccine effort: viruses, vaccines and variants versus efficacy, effectiveness and escape. Nat Rev Immunol. (2021) 21:626–36. doi: 10.1038/s41577-021-00592-1
76. National Institutes of Health. COVID-19 Treatment Guidelines Panel. Coronavirus Disease 2019 (COVID-19) Treatment Guidelines. (2019). National Institutes of Health. Available online at: https://www.covid19treatmentguidelines.nih.gov/ (accessed May 25, 2020).
77. National Institutes of Health. Outpatient Liquid Aspirin (OLA) (OLA COVID). (2022). Available online at: https://www.clinicaltrials.gov/ct2/show/NCT04937088 (accessed May 25, 2022).
78. De Candia E, De Cristofaro R, Landolfi R. Thrombin-induced platelet activation is inhibited by high- and low-molecular-weight heparin. Circulation. (1999) 99:3308–14. doi: 10.1161/01.CIR.99.25.3308
79. Hottz ED, Azevedo-Quintanilha IG, Palhinha L, Teixeira L, Barreto EA, Pão CRR, et al. Platelet activation and platelet-monocyte aggregate formation trigger tissue factor expression in patients with severe COVID-19. Blood. (2020) 136:1330–41. doi: 10.1182/blood.2020007252
80. Puhm F, Allaeys I, Lacasse E, Dubuc I, Galipeau Y, Zaid Y, et al. Platelet activation by SARS-CoV-2 implicates the release of active tissue factor by infected cells. Blood Adv. (2022) 6:3593–605. doi: 10.1182/bloodadvances.2022007444
81. Eikelboom JW, Hirsh J. Combined antiplatelet and anticoagulant therapy: clinical benefits and risks. J Thromb Haemost. (2007) 5(Suppl.1):255–63. doi: 10.1111/j.1538-7836.2007.02499.x
82. Ataga KI, Kutlar A, Kanter J, Liles D, Cancado R, Friedrisch J, et al. Crizanlizumab for the prevention of pain crises in sickle cell disease. N Engl J Med. (2017) 376:429–39. doi: 10.1056/NEJMoa1611770
83. Man Y, Goreke U, Kucukal E, Hill A, An R, Liu S, et al. Leukocyte adhesion to P-selectin and the inhibitory role of Crizanlizumab in sickle cell disease: a standardized microfluidic assessment. Blood Cells Mol Dis. (2020) 83:102424. doi: 10.1016/j.bcmd.2020.102424
84. Kohlmorgen C, Gerfer S, Feldmann K, Twarock S, Hartwig S, Lehr S, et al. Dapagliflozin reduces thrombin generation and platelet activation: implications for cardiovascular risk reduction in type 2 diabetes mellitus. Diabetologia. (2021) 64:1834–49. doi: 10.1007/s00125-021-05498-0
Keywords: coronavirus disease 2019 (COVID-19), thromboembolism, antiplatelet therapy, aspirin, clopidogrel, systematic review, meta-analysis
Citation: Zong X, Wang X, Liu Y, Li Z, Wang W, Wei D and Chen Z (2022) Antiplatelet therapy for patients with COVID-19: Systematic review and meta-analysis of observational studies and randomized controlled trials. Front. Med. 9:965790. doi: 10.3389/fmed.2022.965790
Received: 10 June 2022; Accepted: 19 August 2022;
Published: 07 September 2022.
Edited by:
Luis Garcia De Guadiana-Romualdo, Santa Lucía University General Hospital, SpainReviewed by:
Ivan Castellví, Hospital Universitari de la Santa Creu i Sant Pau, SpainFumihiro Ogawa, Yokohama City University, Japan
Copyright © 2022 Zong, Wang, Liu, Li, Wang, Wei and Chen. This is an open-access article distributed under the terms of the Creative Commons Attribution License (CC BY). The use, distribution or reproduction in other forums is permitted, provided the original author(s) and the copyright owner(s) are credited and that the original publication in this journal is cited, in accordance with accepted academic practice. No use, distribution or reproduction is permitted which does not comply with these terms.
*Correspondence: Dianjun Wei, d2VpZGlhbmp1bjAxJiN4MDAwNDA7MTYzLmNvbQ==; Zhuqing Chen, MzM1OTk4NjA0JiN4MDAwNDA7cXEuY29t