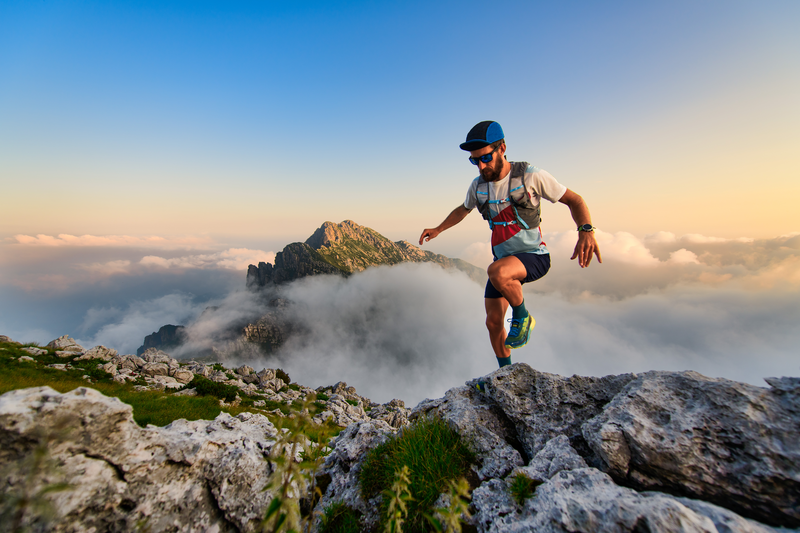
94% of researchers rate our articles as excellent or good
Learn more about the work of our research integrity team to safeguard the quality of each article we publish.
Find out more
ORIGINAL RESEARCH article
Front. Med. , 08 August 2022
Sec. Nephrology
Volume 9 - 2022 | https://doi.org/10.3389/fmed.2022.964101
This article is part of the Research Topic Enlightening the Renal Pathophysiology: New Biomarkers and Clinical Approaches View all 7 articles
Background: Hyperhomocysteinemia is an important factor for endothelial cell damage and a risk factor for cardiovascular diseases. Chronic kidney disease (CKD) is recognized as a leading burden in Taiwan’s healthcare system. This study aimed to investigate the association between homocysteine levels and CKD in middle-aged and elderly adults from a community in northern Taiwan.
Methods: A total of 396 middle-aged and elderly Taiwanese adults were enrolled and completed the health survey. We divided participants according to tertiles of homocysteine levels as first group (homocysteine level ≤ 11.1 μmol/L), second group (homocysteine level 11.2∼14.3 μmol/L), and third group (homocysteine level > 14.3 μmol/L). CKD was defined as estimated glomerular filtration rate (eGFR) < 60 (mL/min/1.73 m2) or urine albumin to creatinine ratio > 30 (mg/g). Pearson correlation was calculated between eGFR and other related risk factors after adjustment for age. The risk of CKD in the second and third groups compared to that in the first group was assessed by multivariate logistic regression after adjustment for age, sex, smoking, hypertension (HTN), diabetes mellitus (DM), body mass index (BMI), dyslipidemia, and uric acid. The Youden index and receiver operating characteristic (ROC) curve were calculated for the optimized cutoff value.
Results: Elevated plasma homocysteine levels were more likely to lower the eGFR and increase the prevalence of CKD. Pearson correlation showed a positive correlation between eGFR and high-density lipoprotein cholesterol, while a negative correlation was observed between homocysteine levels, waist circumference, systolic blood pressure, uric acid levels and BMI (all p < 0.05). In the logistic regression analysis, the prevalence of CKD increased, as well as the homocysteine level. The odds ratio of CKD under 95% confidence interval was 2.655 (1.284–5.490) for the third group compared with the first group after adjusting for age, sex, smoking, DM, HTN, dyslipidemia, uric acid, and BMI (p = 0.008). The area under the ROC curve was 0.662, and a cutoff value of 15.15 μmol/L for the homocysteine level was obtained for detecting subjects with CKD.
Conclusion: Our study findings revealed that elevated homocysteine levels were significantly associated with CKD and could be used as an indicator of CKD among the middle-aged and elderly populations in Taiwan.
Elevated serum homocysteine levels have been considered an important risk factor for atherosclerosis (1). Many studies have also indicated that hyperhomocysteinemia could be a predictor of peripheral arterial disease (2) and coronary artery disease (3). There are many possible mechanisms underlying the relationship between homocysteine levels and atherosclerosis. These mechanisms include reduction in endothelial cell relaxation (4), decreased synthesis of high-density lipoprotein (HDL; 5), and reduction in endothelial cell growth (6). The study has shown that the elevated serum homocysteine level can compromise the peripheral microvascular endothelial function (7) and homocysteine also plays a crucial role in coronary microvascular endothelial dysfunction (8). Further research also revealed that high homocysteine levels indicated the poor prognosis for patients with stable coronary disease (9). Moreover, elevated homocysteine not only has adverse effects on cardiovascular system but also causes cerebrovascular accidents, retinopathy, nephropathy, and other chronic diseases (10). That evidence implied that the microvascular structure is vulnerable under high homocysteine levels. Due to the microvascular structure of the glomerulus, we suspect that homocysteine level also has relationship with chronic kidney disease (CKD). Recent studies have shed light on the relationship between homocysteine levels and CKD (11). According to recent research, homocysteine also impacts endothelial cells in the glomerulus (12). The accumulation of homocysteine eventually damages the glomerular cells and causes glomerular sclerosis (13).
Kidney also plays an important role in plasma amino acid clearance and metabolism (14). The renal function impairment may compromise the clearance and metabolism of plasma homocysteine, although the detailed mechanism is not entirely understood (15). On the other hand, there are many risk factors like BMI that can also impact the serum homocysteine level and those risk factors should be considered during the investigation of homocysteine (16). Our study discloses the relationship between homocysteine and renal function under meticulous study which involved the related risk factors of high homocysteine level.
This cross-sectional study aimed to further explore the association between serum homocysteine levels and CKD in Taiwan. Adequate participants were included and many related risk factors were collected for adjustment. We also propose the optional cutoff point of homocysteine for predicting CKD in the middle-aged and elderly populations in Taiwan. The result of our study provided substantial evidence for medical practices in screening CKD. By treating CKD in advance, patients can have better life quality and the burden of healthcare can be alleviated.
The participants in this cross-sectional and community-based study were recruited from a health survey project conducted in 2019 in northern Taiwan. The inclusion criteria were as follows: (1) age between 50 and 85 years, (2) ability to complete a questionnaire, (3) living in the community and ability to walk to the clinic, and (4) completed all examinations. The exclusion criteria were as follows: (1) cardiovascular disease in recent 3 months and (2) missing or incomplete data. Finally, 396 subjects were enrolled in this study and were eligible for the analysis. The sample size was determined by the G*power 3.1 software. The sample of this study included 396 subjects, which implied sufficient statistical power. All participants completed a questionnaire that included their medical history and personal data during the health survey. Blood and urine tests were performed for each participant to obtain biochemical data. A total of 396 participants were divided into three groups based on the homocysteine levels. This study was approved by the Institutional Review Board of Linkou Chang Gung Memorial Hospital, and all participants were informed and provided their consent before enrollment.
The content of the questionnaire included sex, alcohol drinking status, and current smoking status. Data on hypertension (HTN), diabetes mellitus (DM), CKD, and dyslipidemia. Resting systolic blood pressure (SBP, mm Hg) and diastolic blood pressure (DBP, mm Hg) were measured at least twice. Waist circumference (WC, cm) was measured at the midpoint between the inferior margin of the last rib and the iliac crest in a horizontal plane while the participant was in a standing position. Body mass index (BMI) was calculated as the person’s weight in kilograms divided by the square of height in meters. The following biomedical laboratory parameters were analyzed at the Roche® model lab at Taiwan E&Q Clinical Laboratory: fasting blood sugar (AC sugar) and homocysteine, low-density lipoprotein (LDL-C, mg/dL), HDL-cholesterol (HDL-C; mg/dL), creatinine (mg/dL), triglyceride (TG, mg/dL), alanine transaminase (ALT, U/L), and uric acid (mg/dL) levels. A standardized kit (cobas™ Homocysteine Enzymatic Assay 100 tests) manufactured by Roche Diagnostics GmbH was used for the determination of homocysteine level in our research. Urine albumin to creatinine ratio (urine ACR, mg/g) was calculated as microalbumin (mg/dL) divided by creatinine (mg/dL). Estimated glomerular filtration rate (eGFR) was calculated using the following equation: 186 × (creatinine/88.4) –1.154 × (age) –0.203 × (0.742 if female) using the modification of diet in renal disease equation.
Chronic kidney disease was defined as the presence of kidney damage (urine ACR ≥ 30 mg/g) or decreased renal function with an eGFR < 60 mL/min/1.73 m2 (31). DM was defined as a fasting plasma glucose level ≥ 126 mg/dL or the use of oral antidiabetic drugs or insulin therapy. HTN was defined as SBP ≥ 140 mm Hg, DBP ≥ 90 mm Hg, or the use of medication treatment for HTN. Dyslipidemia was defined as LDL-C level ≥ 130 mg/dL, HDL-C level < 40 mg/dL in men or <50 mg/dL in women, TG level ≥150 mg/dL, TC level ≥200 mg/dL, or the use of lipid-lowering medication. Drinking status was defined as alcohol drinking frequency ≥ 3 days/week.
Participants were divided into three groups according to the homocysteine level: first group (homocysteine level ≤ 11.1 μmol/L), second group (homocysteine level 11.2∼14.3 μmol/L), and third group (homocysteine level > 14.3 μmol/L). Then, the laboratory and clinical data were analyzed within each group. We checked the normality of the continuous variables by using Shapiro–Wilk Normality Test. We presented data as mean ± [SD] if our data is consistent with a normal distribution variables, and median [Q1, Q3] if the variables (AC sugar, TG level, HDL-C level, ALT level, Creatinine level, and Urine ACR) significantly deviated from a normal distribution in Table 1. P-value were derived from one-way ANOVA for data consistent with a normal distribution and Kruskal–Wallis ANOVA for data consistent with non-normal distribution. The categorical variables were analyzed by the chi-square test and expressed as n (%). The correlation between eGFR and age, AC sugar, homocysteine, TG, HDL-C, and LDL-C level, WC, SBP, BMI, and uric acid level were analyzed using Pearson’s correlation coefficient, which was also adjusted for age. A scatterplot is also displayed. The prevalence of CKD in each group is expressed as a bar chart. Furthermore, multiple logistic regression was performed to evaluate the association between groups and CKD after adjusting for age, sex, BMI, smoking, HTN, DM, dyslipidemia, and uric acid level in each group. Throughout our study, p < 0.05 was considered statistically significant. Finally, the Youden index and receiver operating characteristic (ROC) curves were used to determine the optimized homocysteine cutoff value to determine CKD risk. All statistical analyses were performed using SPSS for Windows (version 25.0, released 2011, IBM SPSS Statistics, IBM Corp., Armonk, NY, United States).
Table 1. General characteristics of the study population according to tertiles of homocysteine levels.
A total of 396 middle-aged and elderly individuals from communities in northern Taiwan were enrolled in this study. The participants included 164 men (41.4%) and 232 women (58.6%), with a mean age of 63.72 ± 8.76 years. Table 1 summarizes the demographic and clinical characteristics of the study participants. The enrolled participants were categorized into three subgroups according to their homocysteine levels: first group (homocysteine level ≤ 11.1 μmol/L), second group (homocysteine level 11.2∼14.3 μmol/L), and third group (homocysteine level > 14.3 μmol/L). There were no statistically significant differences in age (p = 0.551), AC sugar (p = 0.115), TG level (p = 0.201), LDL-C level (p = 0.332), ALT levels (p = 0.081), dyslipidemia (p = 0.105), and alcohol consumption (p = 0.789) between the first, second, and third groups. The participants in the high homocysteine level group tended to be male (p < 0.001) and had higher uric acid level (p < 0.001), creatinine level (p < 0.001), urine ACR (p = 0.007), CKD prevalence (p < 0.001), WC (p < 0.001), SBP (p = 0.019), DBP (p = 0.002), BMI (p < 0.001), smoking rate (p < 0.001), HTN prevalence (p = 0.003), and DM prevalence (p = 0.002) than those in the low homocysteine level group. Conversely, the participants in the high homocysteine level group had lower HDL-C level (p < 0.001) and eGFR (p < 0.001) than those in the low homocysteine level group.
Table 2 shows the correlation between eGFR levels and various cardiovascular risk factors. eGFR was positively correlated with HDL-C level (r = 0.107, p = 0.033) and was negatively correlated with age (r = –0.387, p < 0.001), homocysteine level (r = –0.369, p < 0.001), WC (r = –0.136, p = 0.007), SBP (r = –0.165, p < 0.001), BMI (r = –0.115, p = 0.023), and uric acid level (r = –0.346, p < 0.001). Even after adjusting for age, homocysteine level (r = –0.363, p < 0.001), WC (r = –0.125, p = 0.013), SBP (r = –0.090, p = 0.074), BMI (r = –0.149, p = 0.003), and uric acid level (r = –0.347, p < 0.001) still remained statistically significant, except for SBP. Meanwhile, eGFR still remained positive in correlation with HDL-C (r = 0.131, p = 0.009) after adjusting for age. Figure 1 shows a scatterplot of the eGFR with homocysteine level. Pearson’s correlation was –0.369 with p < 0.001.
Figure 1. The correlation between the homocysteine level and eGFR. Abbreviations: eGFR, estimated glomerular filtration rate.
The association between CKD and homocysteine levels is discussed further. The bar chart in Figure 2 reveals that the third group had 40.3% CKD prevalence, second group had 20.8% CKD prevalence, and first group had 14.4% CKD prevalence. This result showed that high homocysteine level group had a higher CKD prevalence than the low homocysteine level group with statistical significance (p < 0.001). In Table 3, a multiple logistic regression model was used to calculate the odds ratio (OR) of homocysteine levels with CKD after adjusting for other risk factors. The third and second groups were compared with the first group in all three models. Model 1 was unadjusted. Model 2 was adjusted for age and sex. Model 3 was adjusted for age, sex, BMI, smoking, HTN, DM, dyslipidemia, and uric acid levels. All ORs decreased and p-values increased after adjusting for more factors in the logistic regression model. The variables of third group remained statistically significant after adjustment in model 1 (OR 4.014, 95% CI 2.212–7.286, p < 0.001), model 2 (adjusted OR 4.476, 95% CI 2.307–8.687, p < 0.001), and even model 3 (adjusted OR 2.655, 95% CI 1.284–5.490, p = 0.008). Figure 3 shows the ROC curve for homocysteine levels as a biomarker of CKD. The area under the ROC curve (AUC) was 0.67; the homocysteine level cutoff value was 15.15 μmol/L with a sensitivity of 0.470 and specificity of 0.801 (Table 4).
Figure 2. Prevalence of CKD based on the homocysteine level. A linear increasing trend across homocysteine tertiles. Abbreviations: CKD, chronic kidney disease.
Table 3. Multivariate logistic regression analysis of the association between the homocysteine level and CKD according the tertiles of the homocysteine level.
Figure 3. ROC curve for homocysteine level as a biomarker to detect subjects with CKD. Abbreviation: CKD, chronic kidney disease; ROC, receiver operating characteristic.
Table 4. The areas under ROC curve, sensitivity, and specificity of the optimized cutoff points for homocysteine level as a biomarker of CKD.
Chronic kidney disease is a heavy burden on the elderly and the society worldwide, and more than 750 million people suffer from kidney disease (17). In our community-based research, we investigated the association between homocysteine levels and CKD prevalence in middle-aged and elderly individuals in northern Taiwan. In Table 1, the participants in the high homocysteine level group tended to be male and have a higher uric acid level, creatinine level, urine ACR level, CKD prevalence, WC, SBP, DBP, BMI, smoking rate, DM prevalence, and HTN prevalence than those in the low homocysteine level group, but participants in the high homocysteine level group tended to have lower HDL-C level and eGFR. People with higher homocysteine level were considered to have a higher CVD risk (18), which was predicted by the Framingham Risk Score. According to the Framingham Risk Score, male sex, HTN, smoking status, and SBP were risk factors for CVD, but HDL level was a protective factor against CVD (19). Our results not only corresponded to the relationship between CVD risk factors and the Framingham Risk Score, but were also supported by many previous studies, which indicated a positive relationship between homocysteine level and male sex, HTN, smoking status, and SBP (20–23). The HDL-C level had an inverse relationship with the homocysteine level, which was also noted in a previous study (24). Meanwhile, our research revealed a negative relationship between homocysteine levels and kidney function. In the high homocysteine level group, the uric acid level, creatinine level, urine ACR, and CKD prevalence were all increased, but eGFR was decreased. These findings led us to speculate the relationship between kidney function and homocysteine levels.
In Table 2, we found a negative relationship between eGFR and homocysteine levels, along with other traditional CVD risk factors such as SBP and BMI. After adjusting for age, the negative correlation between homocysteine levels and eGFR still reached statistical significance. Pearson’s correlation between the homocysteine level and eGFR was –0.363 with p < 0.001 after adjusting for age, which corresponded to the results of previous studies showing that the homocysteine level was negatively correlated to eGFR and positively correlated to CKD (25, 26). Previous studies and results raise the question of whether the homocysteine level can be an important risk factor for CVD; therefore, we wanted to determine whether a high homocysteine level can be an independent risk factor for CKD.
As shown in Figure 2, the bar chart showed that the high homocysteine level groups tended to have a high CKD prevalence (p < 0.001). Furthermore, we analyzed the association between CKD and homocysteine levels using logistic regression with adjustment for other important parameters. In Table 3, the CKD in the second and third groups was compared with the CKD in the first group. The OR for CKD increased in the third group, which always had a greater OR for CKD with statistical significance compared to that in the first group. Even after adjusting for age, sex, BMI, smoking, HTN, DM, dyslipidemia, and uric acid level, the OR for CKD and 95% confidence interval for the third group in comparison with the first group were 2.655 and 1.284 to 5.490, respectively, with p = 0.008. This result confirmed that a high homocysteine level (>14.3 μmol/L) was an independent risk factor for CKD. Finally, we used the ROC curve to measure the diagnostic accuracy of the homocysteine level and determine the optimal cutoff value of the homocysteine level for CKD. Figure 3 shows the ROC curve for homocysteine levels as a biomarker to predict CKD. The AUC was 0.662; the homocysteine cutoff value was 15.15 μmol/L with a sensitivity of 0.470 and specificity of 0.801 (Table 4).
Hyperhomocysteinemia is an important biomedical culprit in CKD and is a serious risk factor that ultimately leads to renal failure. Homocysteine is synthesized from methionine as an intermediate product in the methionine cycle, and homocysteine can revert to methionine or cysteine, which plays an important role in cellular redox by modulating the level of glutathione. Homocysteine, cysteine, and glutathione form an interchain or intrachain with hydrogen sulfide between those residues in protein. Hence, homocysteine affects cellular oxidative stress, protein regulation, and cell signaling (27). There are many hypotheses regarding hyperhomocysteinemia-related renal injury.
Homocysteine-induced oxidation causes the primary pathogenesis of hyperhomocysteinemia (28–30). Homocysteine, cysteine, and glutathione are major thiol-containing amino acids in the human body. Thiols are easily catabolized into thiol-thial radicals (RS.), which interact with other thiols to produce disulfide anions (RSSR-), and other oxygen species (ROS), including superoxide anion radicals (O2.-) and hydrogen peroxide (H2O2; 31). Homocysteine can also produce ROS by activating the NADPH oxidase (32, 33). These ROS interrupt normal cellular function and lead to cardiovascular (34) and kidney disease (35). Indeed, studies have shown that hyperhomocysteinemia compromises the microvascular function (7) in many organs with the microvascular system including the kidney (10).
S-adenosyl-L-methionine-dependent methyltransferase (SAM) derived from methionine is catalyzed by SAM-dependent methyltransferases before donating its methyl group. SAM accounts for >90% methylation of nucleic acids, proteins, and lipids. S-adenosylhomocysteine (SAH), which inhibits the methyltransferase reaction, is also a product of SAM-dependent methyl transfer (36). Hyperhomocysteinemia increases intracellular SAH (37) and inhibits SAM-dependent methyl transfer. DNA methyltransferases are vulnerable to high SAH levels. The demethylation of certain genes, including the hTERT gene, affects kidney function (38, 39).
The kidney is the major site of homocysteine metabolism. Most (80%) of the homocysteine binds to plasma proteins, and only the unbound form is subject to glomerular filtration and tubular reabsorption (40, 41). Hyperhomocysteinemia damages renal function as previously mentioned; in return, decreasing renal function leads to further accumulation of homocysteine in CKD (42, 43). In previous studies, they pointed out the positive relationship between homocysteine levels and CKD, but most of them did not consider the influence of other factors and cutoff points for CKD survey. In our study, we considered other traditional CKD factors for adjustment, and the results indicated that a high homocysteine level could be an independent predictor of CKD. In addition to other traditional CKD parameters, homocysteine should be considered an important biomedical marker in healthy screens.
There are several strengths in our study. First, our study has a clear design, sufficient sample size, comprehensive and relevant confounders, and appropriate data analysis. Second, the novelty of our study was discovering the strong relationship between homocysteine and CKD from a community-dwelling population. Third, there was no similar research that investigates this topic in the middle-aged and older populations from the community in Taiwan.
However, there are several limitations to our study. First, the participants in our study were mainly recruited from northern Taiwan. The characteristics of our study subjects may differ from the general population, so the result cannot be generalized to other populations, Second, the number of participants in this study was relatively small, so future studies using a bigger population and random sampling of the community would make the study more convincing. Third, although our study indicated a strong relationship between homocysteine and CKD, this study was a cross-sectional the study, thus, the causal relationship between homocysteine and CKD could not be evaluated and determined. The mechanism of this relationship needs more exploration in the future.
Our study indicated that a high homocysteine level can be an independent risk factor for CKD in the middle-aged and elderly populations in Taiwan. Further prospective studies should examine and determine if lower homocysteine levels reduce the likelihood of CKD.
The raw data supporting the conclusions of this article will be made available by the authors, without undue reservation.
The studies involving human participants were reviewed and approved by Institutional Review Board of Linkou Chang Gung Memorial Hospital. The patients/participants provided their written informed consent to participate in this study.
Y-LS and J-YC composed and conducted the study. C-CS helped data collection. J-YC provided instruction and consultation, project administration, and supervision. All authors contributed to the article and approved the submitted version.
This study was supported by United Safety Medical Group (USGN2019001).
We would like to thank Editage (http://online.editage.jp) for English language editing.
The authors declare that the research was conducted in the absence of any commercial or financial relationships that could be construed as a potential conflict of interest.
All claims expressed in this article are solely those of the authors and do not necessarily represent those of their affiliated organizations, or those of the publisher, the editors and the reviewers. Any product that may be evaluated in this article, or claim that may be made by its manufacturer, is not guaranteed or endorsed by the publisher.
1. Temple ME, Luzier AB, Kazierad DJ. Homocysteine as a risk factor for atherosclerosis. Ann Pharmacother. (2000) 34:57–65. doi: 10.1345/aph.18457
2. Kazemi MB, Eshraghian K, Omrani GR, Lankarani KB, Hosseini E. Homocysteine level and coronary artery disease. Angiology. (2006) 57:9–14. doi: 10.1177/000331970605700102
3. Katsiki N, Perez-Martinez P, Mikhailidis DP. Homocysteine and non-cardiac vascular disease. Curr Pharma Design. (2017) 23:3224–32. doi: 10.2174/1381612823666170317124913
4. Zhao J, Chen H, Liu N, Chen J, Gu Y, Chen J, et al. Role of hyperhomocysteinemia and hyperuricemia in pathogenesis of atherosclerosis. J Stroke Cerebrovasc Dis. (2017) 26:2695–9. doi: 10.1016/j.jstrokecerebrovasdis.2016.10.012
5. Liao D, Tan H, Hui R, Li Z, Jiang X, Gaubatz J, et al. Hyperhomocysteinemia decreases circulating high-density lipoprotein by inhibiting apolipoprotein AI Protein synthesis and enhancing HDL cholesterol clearance. Circ Res. (2006) 99:598–606. doi: 10.1161/01.RES.0000242559.42077.22
6. Jamaluddin MDS, Chen I, Yang F, Jiang X, Jan M, Liu X, et al. Homocysteine inhibits endothelial cell growth via DNA hypomethylation of the cyclin a gene. Blood. (2007) 110:3648–55. doi: 10.1182/blood-2007-06-096701
7. Toya T, Sara JD, Lerman B, Ahmad A, Taher R, Godo S, et al. Elevated plasma homocysteine levels are associated with impaired peripheral microvascular vasomotor response. IJC Heart Vasc. (2020) 28:100515. doi: 10.1016/j.ijcha.2020.100515
8. Ahmad A, Corban MT, Toya T, Sara JD, Lerman B, Park JY, et al. Coronary microvascular endothelial dysfunction in patients with angina and nonobstructive coronary artery disease is associated with elevated serum homocysteine levels. J Am Heart Assoc. (2020) 9:e017746. doi: 10.1161/JAHA.120.017746
9. Rallidis LS, Kosmas N, Rallidi T, Pavlakis G, Kiouri E, Zolindaki M. Homocysteine is an independent predictor of long-term cardiac mortality in patients with stable coronary artery disease in the era of statins. Coron Artery Dis. (2020) 31:152–6. doi: 10.1097/MCA.0000000000000800
10. Corban MT, Lerman LO, Lerman A. Ubiquitous yet unseen: Microvascular endothelial dysfunction beyond the heart. Eur Heart J. (2018) 39:4098–100. doi: 10.1093/eurheartj/ehy576
11. Perna AF, Ingrosso D. Homocysteine and chronic kidney disease: An ongoing narrative. J Nephrol. (2019) 32:673–5. doi: 10.1007/s40620-019-00622-1
12. Yi F, Jin S, Zhang F, Xia M, Bao JX, Hu J, et al. Formation of lipid raft redox signalling platforms in glomerular endothelial cells: An early event of homocysteine-induced glomerular injury. J Cell Mol Med. (2009) 13:3303–14. doi: 10.1111/j.1582-4934.2009.00743.x
13. Yi F, Li PL. Mechanisms of homocysteine-induced glomerular injury and sclerosis. Am J Nephrol. (2008) 28:254–64. doi: 10.1159/000110876
14. Garibotto G, Sofia A, Saffioti S, Bonanni A, Mannucci I, Verzola D. Amino acid and protein metabolism in the human kidney and in patients with chronic kidney disease. Clin Nutr. (2010) 29:424–33. doi: 10.1016/j.clnu.2010.02.005
15. Friedman AN, Bostom AG, Selhub J, Levey AS, Rosenberg IH. The kidney and homocysteine metabolism. J Am Soc Nephrol. (2001) 12:2181–9. doi: 10.1681/ASN.V12102181
16. Zaric BL, Obradovic M, Bajic V, Haidara MA, Jovanovic M, Isenovic ER. Homocysteine and hyperhomocysteinaemia. Curr Med Chem. (2019) 26:2948–61. doi: 10.2174/0929867325666180313105949
17. Crews DC, Bello AK, Gamal S. 2019 World kidney day editorial – Burden, access, and disparities in kidney disease. J Bras Nefrol. (2019) 41:1–9. doi: 10.1590/2175-8239-jbn-2018-0224
18. Chrysant SG, Chrysant GS. The current status of homocysteine as a risk factor for cardiovascular disease: A mini review. Expert Rev Cardiovasc Ther. (2018) 16:559–65. doi: 10.1080/14779072.2018.1497974
19. Selvarajah S, Kaur G, Haniff J, Cheong KC, Hiong TG, van der Graaf Y, et al. Comparison of the framingham risk score, SCORE and WHO/ISH cardiovascular risk prediction models in an Asian population. Int J Cardiol. (2014) 176:211–8. doi: 10.1016/j.ijcard.2014.07.066
20. Cohen E, Margalit I, Shochat T, Goldberg E, Krause I. Gender differences in homocysteine concentrations, a population-based cross-sectional study. Nutr Metab Cardiovasc Dis. (2019) 29:9–14. doi: 10.1016/j.numecd.2018.09.003
21. Lin BY, Li P, Wu XD, Li H, Zeng ZY. The relationship between homocysteine, blood pressure variability, and left ventricular hypertrophy in patients with essential hypertension: An observational study. Adv Ther. (2019) 37:381–9. doi: 10.1007/s12325-019-01154-7
22. Awasthi M, Omoike OE, Paul TK, Ridner SL, Mamudu HM An association between smoking status and homocysteine levels and whether this association is modified by sex hormones and cholesterol. Johnson City, TN: Biomarkers (2019).
23. Weng H, Li Y, Fan F, Yang H, Zhou G, Sun P, et al. The association between total homocysteine and blood pressure in two independent Chinese populations. J Hum Hypertens. (2019) 34:657–65. doi: 10.1038/s41371-019-0288-6
24. Momin M, Jia J, Fan F, Li J, Dou J, Chen D, et al. Relationship between plasma homocysteine level and lipid profiles in a community-based Chinese population. Lipids Health Dis. (2017) 16:54. doi: 10.1186/s12944-017-0441-6
26. Chao MC, Hu SL, Hsu HS, Davidson LE, Lin CH, Li CI, et al. Serum homocysteine level is positively associated with chronic kidney disease in a Taiwan Chinese population. J Nephrol. (2014) 27:299–305.
27. Zhang C, Xia M, Boini KM, Li CX, Abais JM, Li XX, et al. Epithelial-to-mesenchymal transition in podocytes is mediated by the activation of NADPH oxidase in hyperhomocysteinemia. Pflugers Arch. (2011) 462:455–67. doi: 10.1007/s00424-011-0981-y
28. Herrmann W, Obeid R. Homocysteine: A biomarker in neurodegenerative diseases. Clin Chem Lab Med. (2011) 49:435–41. doi: 10.1515/CCLM.2011.084
29. Petras M, Tatarkova Z, Kovalska M, Mokra D, Dobrota D, Lehotsky J, et al. Hyperhomocysteinemia as a risk factor for the neuronal system disorders. J Physiol Pharmacol. (2014) 65:15–23.
30. Yi F, Zhang AY, Li N, Muh RW, Fillet M, Renert AF, et al. Inhibition of ceramide-redox signaling pathway blocks glomerular injury in hyperhomocysteinemic rats. Kidney Int. (2006) 70:88–96. doi: 10.1038/sj.ki.5001517
31. Jacobsen DW. Hyperhomocysteinemia and oxidative stress: Time for a reality check? Arterioscler Thromb Vasc Biol. (2000) 20:1182–4. doi: 10.1161/01.ATV.20.5.1182
32. Sipkens JA, Hahn N, van den Brand CS, Meischl C, Cillessen SA, Smith DE, et al. Homocysteine-induced apoptosis in endothelial cells coincides with nuclear NOX2 and nuclear NOX4 activity. Cell Biochem Biophys. (2013) 67:341–52. doi: 10.1007/s12013-011-9297-y
33. Ostrakhovitch EA, Tabibzadeh S. Homocysteine in chronic kidney disease. Adv Clin Chem. (2015) 72:77–106. doi: 10.1016/bs.acc.2015.07.002
34. Djuric D, Vladimir J, Vladimir Z, Ivan S. Homocysteine and homocysteine-related compounds: An overview of the roles in the pathology of the cardiovascular and nervous systems. Can J Physiol Pharmacol. (2018) 96:991–1003. doi: 10.1139/cjpp-2018-0112
35. Shah SV. The role of reactive oxygen metabolites in glomerular disease. Annu Rev Physiol. (1995) 57:245–62. doi: 10.1146/annurev.ph.57.030195.001333
36. Finkelstein JD. The metabolism of homocysteine: Pathways and regulation. Eur J Pediatr. (1998) 157:S40–4. doi: 10.1007/PL00014300
37. Perna AF, Ingrosso D, De Santo NG, Galletti P, Zappia V. Mechanism of erythrocyte accumulation of methylation inhibitor S-adenosylhomocysteine in uremia. Kidney Int. (1995) 47:247–53. doi: 10.1038/ki.1995.31
38. Zhang D, Sun X, Liu J, Xie X, Cui W, Zhu Y. Homocysteine accelerates senescence of endothelial cells via DNA hypomethylation of human telomerase reverse transcriptase. Arterioscler Thromb Vasc Biol. (2015) 35:71–8. doi: 10.1161/ATVBAHA.114.303899
39. Kloda K, Domanski L, Kwiatkowska E, Borowiecka E, Safranow K, Drozd A, et al. hTERT, BICD1, and chromosome 18 polymorphisms associated with telomere length affect kidney allograft function after transplantation. Kidney Blood Press Res. (2015) 40:111–20. doi: 10.1159/000368487
40. Selhub J. Homocysteine metabolism. Annu Rev Nutr. (1999) 19:217–46. doi: 10.1146/annurev.nutr.19.1.217
41. Dudman NP, Guo XW, Gordon RB, Dawson PA, Wilcken DE. Human homocysteine catabolism: Three major pathways and their relevance to the development of arterial occlusive disease. J Nutr. (1996) 126:1295S–300S. doi: 10.1093/jn/126.suppl_4.1295S
42. Rodriguez WE, Sen U, Tyagi N, Kumar M, Carneal G, Aggrawal D, et al. PPAR gamma agonist normalizes glomerular filtration rate, tissue levels of homocysteine, and attenuates endothelial-myocyte uncoupling in alloxan-induced diabetic mice. Int J Biol Sci. (2008) 4:236–44. doi: 10.7150/ijbs.4.236
Keywords: homocysteine, middle-age and elderly, chronic kidne disease, kidney function, biomarker
Citation: Shih Y-L, Shih C-C and Chen J-Y (2022) Elevated homocysteine level as an indicator for chronic kidney disease in community-dwelling middle-aged and elderly populations in Taiwan: A community-based cross-sectional study. Front. Med. 9:964101. doi: 10.3389/fmed.2022.964101
Received: 08 June 2022; Accepted: 18 July 2022;
Published: 08 August 2022.
Edited by:
Clara Barrios, Parc de Salut Mar, SpainReviewed by:
Ali Ahmad, Mayo Clinic, United StatesCopyright © 2022 Shih, Shih and Chen. This is an open-access article distributed under the terms of the Creative Commons Attribution License (CC BY). The use, distribution or reproduction in other forums is permitted, provided the original author(s) and the copyright owner(s) are credited and that the original publication in this journal is cited, in accordance with accepted academic practice. No use, distribution or reproduction is permitted which does not comply with these terms.
*Correspondence: Jau-Yuan Chen, d2VsaW5zQGNnbWgub3JnLnR3
†These authors have contributed equally to this work and share first authorship
Disclaimer: All claims expressed in this article are solely those of the authors and do not necessarily represent those of their affiliated organizations, or those of the publisher, the editors and the reviewers. Any product that may be evaluated in this article or claim that may be made by its manufacturer is not guaranteed or endorsed by the publisher.
Research integrity at Frontiers
Learn more about the work of our research integrity team to safeguard the quality of each article we publish.