- 1Centro de Investigación Médica Pacifica Salud-INDICASAT AIP, Pacifica Salud, Hospital Punta Pacicifica, Panama City, Panama
- 2Centro de Biología Celular y Molecular de Enfermedades-Instituto de Investigaciones Científicas y Servicios de Alta Tecnología (INDICASAT-AIP), City of Knowledge, Panama City, Panama
- 3Hospital Rafael Estévez, Caja de Seguro Social, Aguadulce, Panama
- 4Department of Health Sciences, College of Medicine, University of Leicester, Leicester, United Kingdom
Objective: The aim of this study was to assess the accuracy of prenatal imaging for the diagnosis of congenital Zika syndrome.
Data sources: Medline (via Pubmed), PubMed, Scopus, Web of Science, and Google Scholar from inception to March 2022. Two researchers independently screened study titles and abstracts for eligibility.
Study eligibility criteria: Observational studies with Zika virus-infected pregnant women were included. The index tests included ultrasound and/or magnetic resonance imaging. The reference standard included (1) Zika infection-related perinatal death, stillbirth, and neonatal death within the first 48 h of birth, (2) neonatal intensive care unit admission, and (3) clinically defined adverse perinatal outcomes.
Synthesis methods: We extracted 2 × 2 contingency tables. Pooled sensitivity and specificity were estimated using the random-effects bivariate model and assessed the summary receiver operating characteristic (ROC) curve. Risk of bias was assessed using QUADAS 2 tool. The certainty of the evidence was evaluated with grading of recommendations.
Results: We screened 1,459 references and included 18 studies (2359 pregnant women, 347 fetuses with confirmed Zika virus infection). Twelve studies (67%) were prospective cohorts/case series, and six (37%) were retrospective cohort/case series investigations. Fourteen studies (78%) were performed in endemic regions. Ten studies (56%) used prenatal ultrasound only, six (33%) employed ultrasound and fetal MRI, and two studies (11%) used prenatal ultrasound and postnatal fetal MRI. A total of six studies (ultrasound only) encompassing 780 pregnant women (122 fetuses with confirmed Zika virus infection) reported relevant data for meta-analysis (gestation age at which ultrasound imagining was captured ranged from 16 to 34 weeks). There was large heterogeneity across studies regarding sensitivity (range: 12 to 100%) and specificity (range: 50 to 100%). Under a random-effects model, the summary sensitivity of ultrasound was 82% (95% CI, 19 to 99%), and the summary specificity was 97% (71 to 100%). The area under the ROC curve was 97% (95% CI, 72 to 100%), and the summary diagnostic odds ratio was 140 (95% CI, 3 to 7564, P < 0.001). The overall certainty of the evidence was “very low”.
Conclusion: Ultrasound may be useful in improving the diagnostic accuracy of Zika virus infection in pregnancy. However, the evidence is still substantially uncertain due to the methodological limitations of the available studies. Larger, properly conducted diagnostic accuracy studies of prenatal imaging for the diagnosis of congenital Zika syndrome are warranted.
Systematic review registration: Identifier [CRD42020162914].
Introduction
Zika virus (ZIKV) infection is an emerging public health problem in several regions worldwide. Zika virus (ZIKV) was first isolated in 1947 from a sentinel monkey in the Zika forest in Uganda, Africa (1). Following an outbreak in the Americas in 2015, the virus spread across 59 countries, and transmission still persists in many countries with more than 500,00 suspected cases reported (2–6).
The rate of maternal–fetal transmission ranges from 7 to 18% in confirmed or probable cases (7). A recent study in Brazil reported a rate as high as 35% (8). ZIKV perinatal infection causes neurological malformations that characterize congenital Zika syndrome (CZS) (3–5). The ZIKV strains, belonging to the Asian lineage, replicate in the placenta and fetal brain (9–11). In the brain, the virus infects neuronal progenitor cells, resulting in the inhibition of cell proliferation and differentiation, as well as neuronal apoptosis (12). CZS includes few developmental and morphological hallmarks, including but not limited to microcephaly, agenesia/hypoplasia of the corpus callosum, parenchymal calcifications, brain stem hypoplasia, and ocular injury (4, 9, 11, 13). Neonatal findings range from cognitive, sensory, and motor disabilities to macular scarring and focal pigmentary retinal mottling; marked early hypertonia and extrapyramidal symptoms may also be present (3–7).
ZIKV infection diagnosis is marked by unique challenges that affect accuracy. According to the Center for Disease Control (CDC) guidelines, ZIKV RNA amplification by reverse transcription-polymerase chain reaction (RT-PCR) should be performed in serum, blood, or urine as early as possible and up to 12 weeks after symptom onset (CDC guidelines). Diagnosing ZIKV infection is complicated for several reasons: the mother is usually asymptomatic or shows non-specific symptoms (7). ZIKV has a narrow window of viremia and viruria, making RT-PCR detection difficult, and diagnostic tests can show cross-reactivity between ZIKV and dengue virus (14, 15). A recent systematic review indicated that ZIKV RNA could be cleared from amniotic fluid during pregnancy. Therefore, a negative ZIKV test result from amniocentesis did not provide adequate reassurance that the fetus was unaffected (16). The optimal timing for performing amniocentesis and the positive predictive value of amniocentesis for congenital ZIKV syndrome have not yet been established (16, 17).
The CDC, Society of Maternal-Fetal Medicine, the American College of Obstetricians and Gynecologists (ACOG), and International Society of Ultrasound in Obstetrics and Gynecology (ISUOG) all recommend ultrasound (US) and neurosonology as the preferred tool to evaluate the fetal brain and screen for other fetal anomalies (12–15, 17–20).
Fetal magnetic resonance imaging (MRI) has increased the positive predictive value of fetal brain abnormalities; however, it has a low negative predictive value and is associated with a greater number of false positives (21).
In a recent study investigating the rate of adverse outcomes in proven infected fetuses/newborns, 45% of the cases presented no signs of complications. Also, 20% had mild/moderate signs potentially correlated with congenital ZIKV (cZIKV) infection, 21% had severe complications, and 14% resulted in fetal loss (7).
The objective of this systematic review was to identify the diagnostic accuracy of prenatal imaging studies for perinatal outcomes of fetuses exposed to maternal ZIKV infection.
Methods
This systematic review was conducted following the “Synthetizing Evidence from Diagnostic Accuracy Test (SEDATE) guidelines” (Supplementary Table 1) (22) and reported in agreement with the “Preferred Reporting Items for Systematic Reviews and Meta-Analyses (PRISMA-DTA statement guidelines)” (Supplementary Table 2) (23).
The study protocol was prospectively registered at the International Prospective Register PROSPERO (number: CRD42018097200) for quality control (24).
Study selection process
We included observational cohort studies (retrospective and prospective) of pregnant women with ZIKV infection in any trimester of pregnancy whose fetuses developed CZS and underwent prenatal imaging studies (USG and/or MRI) and clinical follow-up of the neonate. We selected studies that determined ZIKV infection through real-time PCR when possible, immunoglobulin M (IgM) serological tests, and/or plaque neutralization reduction tests (PRNT). Exclusion criteria included case series with less than four cases, pediatric series on newborns and children from which maternal and pregnancy information could not be retrieved. We also excluded studies with insufficient outcome data.
Index test
We considered ultrasound and fetal magnetic resonance as index tests, either alone or in combination.
Reference standard test
The lack of a gold standard reference test is an acknowledged problem in studies of CZS. Reference tests for the diagnosis of CZS have important limitations (25).
For the present review, infants with a positive Zika RT-PCR from at least one fetal or neonatal sample (cerebrospinal fluid, amniotic fluid, placental tissue, or fetal tissue) were defined as confirmed CZS cases. Infants with Zika virus IgM detected in umbilical cord/neonatal blood or in cerebrospinal fluid were also considered confirmed cases. When performed, amniocentesis was used to rule outinfections such as toxoplasmosis, syphilis, rubella, cytomegalovirus and herpes simplex or genetic causes for congenital anomalies. We also considered as reference standard clinical examinations performed at birth by neonatologists, pediatric infectious disease specialists, and geneticists. A fetus or neonate with severe microcephaly, cortical hypoplasia with abnormal gyral patterns, intracranial calcifications located between cortex or subcortex, congenital contractures, or macular scarring was considered as confirmed CZS case. We also considered as confirmed CZS cases perinatal death, including stillbirth and neonatal death within the first 48 h after birth; admission to the neonatal intensive care unit (NICU); composite adverse perinatal outcomes as defined by each individual study; and abnormal clinical follow-up, which included seizures, eye abnormalities, hearing abnormalities, and abnormal neurological examinations that included postnatal imaging, such as transcranial ultrasound and computerized tomography scan.
In this study, the reference standard is composed of
1. Perinatal death, including stillbirth and neonatal death within the first 48 h after birth;
2. Admission to neonatal intensive care unit (NICU);
3. Composite adverse perinatal outcomes according to the definition of each individual study, which commonly includes any adverse outcomes occurring in the studied population; and/or
4. Abnormal clinical follow-up included seizures, eye abnormalities, hearing abnormalities, and abnormal neurological examinations.
Data extraction
We piloted the screening strategy and the data extraction sheet. Two investigators (TH and AG) extracted data from eligible studies independently. We used a modified extraction template obtained from the Cochrane community web site (26) including the following study characteristics: authors, year of publication, settings, country, study design, period when the study was conducted, inclusion criteria, sample size, patient characteristics (inclusion and exclusion criteria, demographics), how the test was performed (gestational age at testing), and the type of reference standard test. We carefully cross-checked references for overlapping studies and solved all discrepancies between assessors via consensus, or consultation with a third investigator (ICB).
Assessment of risk of bias
Assessments were conducted by a pair of reviewers (TH and AG) working independently. Discrepancies were resolved by consensus or discussion with a third investigator. We used the QUADAS 2 tool, which evaluates the risk of bias and applicability of the tests. QUADAs 2 has four domains: patient selection, index test, reference standard, and flow and timing (27). Each domain had signaling questions that were categorized as low, high, or unclear risk of bias (Supplementary Table S3). We used the risk of bias assessment to judge the certainty of the overall evidence using the Grading of Recommendations Assessments, Development and Evaluation (GRADE) system (28).
Strategy for data synthesis and statistical analysis
A template for 2 × 2 tables describing the true positive (TP), false positive (FP), false negative (FN), and true negative (TN) results for each included study was constructed. We performed a meta-analysis assuming a random-effect model to obtain summary diagnostic accuracy estimates (and their 95% CIs). We used both bivariate and hierarchical summary receiver operating characteristic (HSROC) meta-analysis models (29–31). Summary HSROC curves with 95% confidence and 95% prediction regions were presented. The latter reflects the between-study heterogeneity, indicating the approximate predictive distribution of accuracy measures to be estimated in a future diagnostic accuracy study. The random-effects summary diagnostic odds ratio (DOR) was also calculated as a single metric that combines sensitivity and specificity (29, 31). A DOR > 1 captures how many times the chance of a positive US finding is higher among ZIKV-infected pregnant women than among pregnant women without Zika infection.
The statistical heterogeneity across sensitivity and specificity measures was assessed by Cochran’s Q-test and quantified with the I2 statistic. For Cochran’s Q-test, a p-value < 0.10 was considered evidence of variability across studies larger than expected by chance (31). For the I2 statistic, values > 50% were considered evidence of substantial statistical heterogeneity (30). Deek’s test was used to evaluate funnel plot asymmetry (31). We also plotted the log(DOR) against the inverse squared root of the effective sample size for visual inspection of funnel plot asymmetry. A p-value < 0.10 was deemed evidence of funnel plot asymmetry. All analyses were conducted in Stata (version 14, College Station, TX, United States).
Results
Study selection and study characteristics
Figure 1 shows the study selection process. We identified 1459 citations. After removing duplicates, we assessed 91 full-length studies, of which 18 were included in our systematic review. Supplementary Table S4 summarizes the list of excluded studies with reasons for their exclusion.
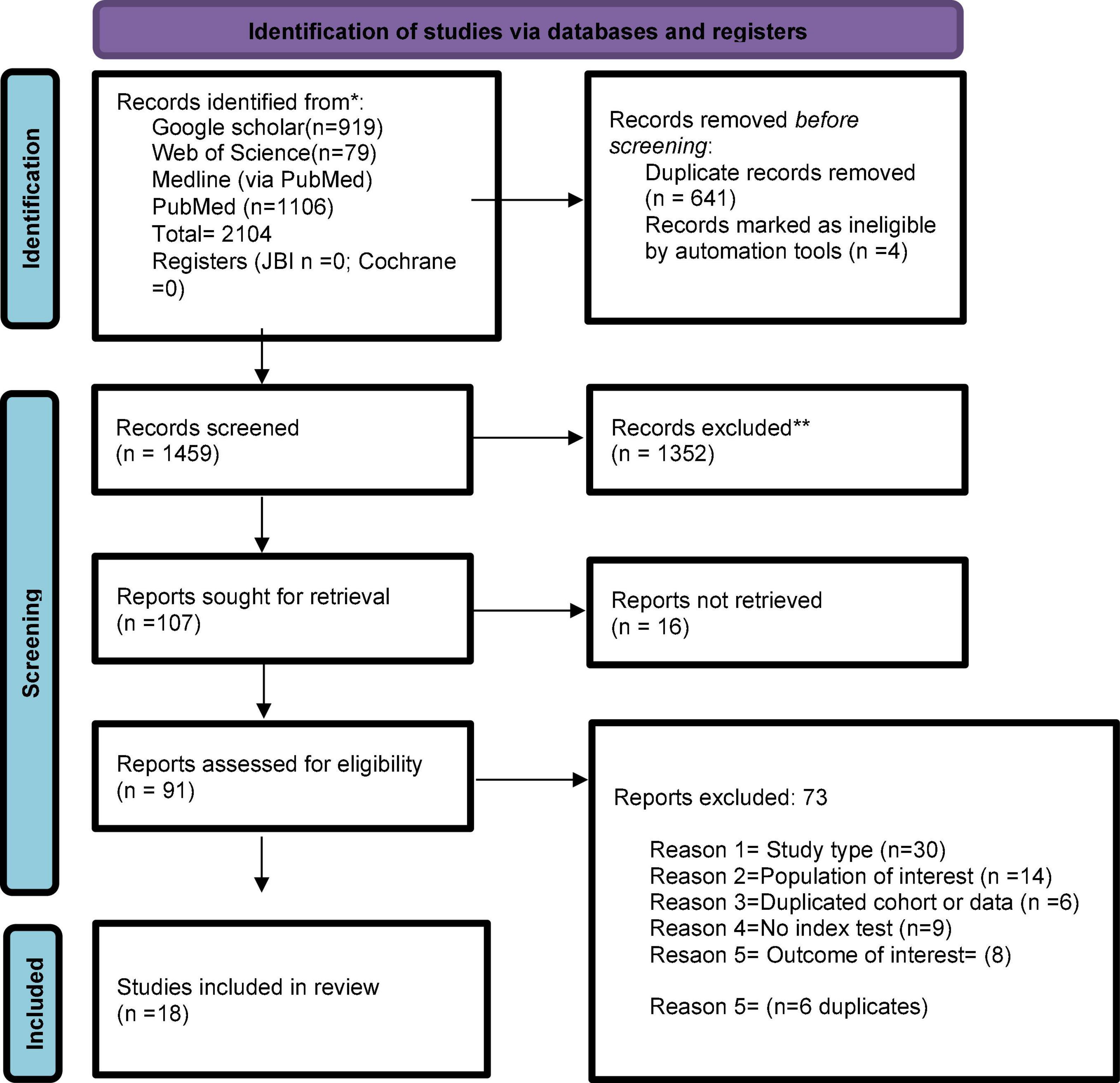
Figure 1. Flowchart summarizing inclusion in systematic review of studies. *Consider, if feasible to do so, reporting the number of records identified from each database or register searched (rather than the total number across all databases/registers). **If automation tools were used, indicate how many records were excluded by a human and how many were excluded by automation tools. From: Page MJ, McKenzie JE, Bossuyt PM, Boutron I, Hoffmann TC, Mulrow CD, et al. The PRISMA 2020 statement: an updated guideline for reporting systematic reviews. BMJ 2021;372:n71. doi: 10.1136/bmj.n71. For more information, visit: http://www.prisma-statement.org/.
A total of 347 fetuses with confirmed Zika Virus infection were investigated across the 18 included studies. The pregnancy termination rate after fetal diagnosis of CZS was low, with only 36 (1.5%) women choosing to have an abortion. Across studies, the median (interquartile range) sample size of was 89 (22 to 209) participants. Of the included 18 studies, 12 (67%) had a prospective temporality. Fourteen studies (78%) were performed in endemic regions: Brazil (n = 7), Colombia (n = 3), French Polynesia (n = 1), French Guinea (n = 1), Martinique (n = 1) and Trinidad and Tobago (n = 1). The main characteristics of the included studies are displayed in Table 1.
The studies were published between 2016 and 2021, 6 were retrospective and 12 prospective studies. Among the eligible studies, there were a total of 2,359 fetuses; however, 36 (1.5%) women opted for termination of pregnancy.
Geographical distribution was as follows: studies in endemic settings: seven studies were performed in Brazil (29–35), three in Colombia (4, 36, 37), one in French Polynesia (38), one in French Guinea (39), one in Martinique (1), and one in Trinidad and Tobago (40). Two studies were performed in Barcelona, a non-endemic setting (41, 42). Two other studies were from the United States (43, 44). The variation in the number of pregnant women ranged from 8 (31) to 511 (35).
Overview of diagnostic criteria used in the included studies
Brazil (endemic region)
Six out of the seven (86%) studies conducted in Brazil used definitions of presumed Zika infection, characterized by symptoms and/or serological Zika IgG/IgM in the absence of dengue and chikungunya antibodies. Confirmed Zika maternal infection was defined according to The Brazilian Ministry of Health and WHO guidelines. Two studies (32, 33) defined inclusion criteria as fetal microcephaly with a molecular or epidemiological link to ZIKV without other conditions known to cause microcephaly. One study included mothers with symptoms of ZIKV infection, whose fetuses had brain abnormalities (34).
Colombia (endemic region)
Two of the three (67%) studies conducted in Colombia were performed in the same city (Barranquilla). One of these two studies recruited participants from a clinical research facility with pregnant mothers with symptoms that met the guidelines from the CDC with or without confirmatory laboratory testing for ZIKV. The other study from Barranquilla included mothers with symptoms of infection or if their partners had symptoms, and anti-ZIKV IgM positive or ZIKV RT-PCR was required (4). The third study, conducted in Santander, used inclusion criteria similar to those used in above studies, enrolling women with symptoms of infection or if their partners had symptoms, and anti-ZIKV IgM positive or ZIKV RT-PCR was required.
Other endemic regions
Studies conducted in Trinidad and Tobago and French territories used similar inclusion criteria: pregnant women with positive serology for Zika virus antibodies, confirmation by RT-PCR ZIKV in at least one of the following biological materials: fetal/neonatal sample amniotic fluid, fetal blood, placenta, amnion, cerebrospinal fluid or brain tissue after delivery. Besides checking for antibodies of Dengue and Chikungunya, one study ruled out those infections with RT-PCR (35).
Europe (non-endemic region)
In two studies carried out in Spain.
Non-endemic region
In European studies, an inclusion criterion (41, 42) was a history of travel to an endemic area during pregnancy or 2 months before pregnancy. If the sexual partner had traveled to affected countries in the previous 6 months to the pregnancy, it was considered potential exposure to ZIKV. A positive RT-PCR test of ZIKV in serum and/or urine samples and/or a pregnant woman with a positive anti-ZIKV IgM antibodies, with a negative anti-dengue virus IgM antibodies and an anti-ZIKV neutralization titer ≥ 1/32 was a confirmation of infection.
Two retrospective cohorts were from the United States, one from New York and the other from Miami (43, 44). No informed consent was required in either. The definition of exposure to ZIKV infection was made following CDC guidelines. A confirmed cZIKV was reported with any positive nucleic acid test from a serum, urine, or cerebrospinal fluid sample. A positive or equivocal anti-ZIKV IgM antibody from an infant’s blood sample with a negative nucleic acid test was considered probable ZIKV infection.
Index test
Of the 18 included studies, 10 (56%) used prenatal ultrasound only, six studies (33%) evaluated fetuses with a combination of prenatal ultrasound and fetal MRI, and two studies (11%) added a postnatal MRI evaluation in addition to the prenatal ultrasound. Prenatal ultrasound included fetal biometry, detailed anatomy assessment and/or neuro-sonography performed by experienced sonographers, radiologists, or maternal fetal medicine specialists. Fetal MRI was performed in a total of six studies; two (4, 36) used 1.5T scanners and one (34) included a 3T MRI evaluation. Standard acquisition protocols were reported in the above studies, without use of maternal or fetal sedation. The MRI findings were interpreted by neuroradiologists and fetal radiologists. The remaining three studies did not report on the fetal MRI procedures or results.
In six studies the image test was performed between 16 and 25 weeks (1, 34–36, 38, 40) while four performed the test between 26 and 32.3 weeks (4, 31, 41, 42), and seven studies reported monthly scans (37, 38, 43–47). In the remaining study (39), the index test was performed between 18 and 33–34 weeks and stratified by trimester of gestation. More information on scans and definitions of anomalies is provided in the supplementary material (Tables SA1, SA2 Appendix 2 in Supplementary files).
Reference standard
A total of 10 studies reported that children underwent complete clinical examination and additional tests like cranial US, computed tomography, and ocular examination (4, 31, 33, 35, 37, 39–41, 43). Two studies reported just birth outcomes (gestational age at delivery, cesarean section delivery, and Apgar scores). One study reported a composite neonatal outcome, defined as perinatal death (stillbirth or death within 28 days of life), an abnormal finding on neonatal examination, or an abnormal finding in postnatal neuroimage (42). One study reported the outcome as a categorization of brain abnormalities (43). Four studies reported adverse perinatal outcomes, brain abnormalities and postnatal neurological assessment (38, 40, 45, 47). More information on definitions of reference standard is provided in Table SA2 Appendix 2 in Supplementary files).
Risk of bias
Figure 2 summarizes the risk of bias assessment and applicability concerns in the 18 included studies. Only three (17%) of the 18 studies were considered at low risk of bias for all risk domains – including applicability concerns. Overall, a low risk of patient selection was found in 10 (56%) studies, a low risk of bias of index test in 9 (50%) studies, a low risk of bias of reference test in 7 (39%) and a low risk of bias in flow and timing in 6 (33%) studies.
Characteristics of the included studies in the meta-analysis
Sufficient outcome data were available for six studies involving 763 pregnant women. Five studies (83%) had a prospective temporality. The median sample was 82 participants (interquartile range: 64 to 226). Among the 763 participants, there were 168 (22%) cases identified with laboratory confirmed ZIKV.
Of the six studies with sufficient information for meta-analysis, one did not report the gestation age at which ultrasound imagining was captured. One study performed ultrasound monthly, and the four remaining studies performed ultrasound at a median (interquartile range) of 25.7 (22.6 to 27.4) weeks of gestational age (min = 20, max = 28.4). Fetal MRI was performed in four studies (6 with a total of 136 fetuses, in three studies, the median time for performing the fetal MRI was 28.1 (27.1 to 29.6) weeks of gestational age (Supplementary Table SA2).
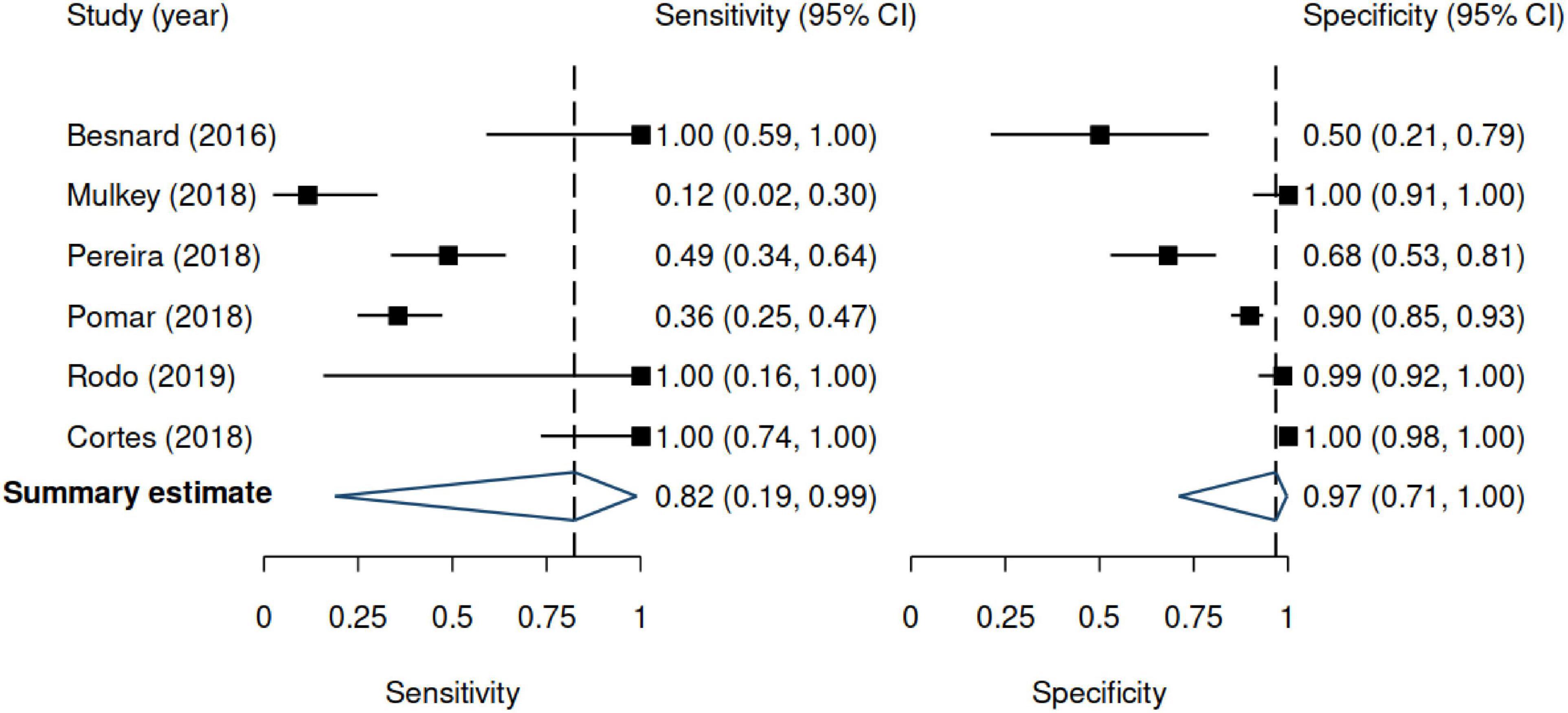
Figure 3. Forest plots for sensitivity and specificity. Results are based on the random-effects model. 95% CI denotes 95% confidence interval. The positive likelihood ratio was 24.9 (95% CI 2.11–293.2), and the negative likelihood ratio was 0.19 (95% CI 0.02–2.05).
Diagnostic accuracy of ultrasound
Across studies, the sensitivity of US ranged from 12 to 100%, whereas the specificity ranged from 50 to 100%. Figure 3 shows the random-effects model summary estimates for sensitivity and specificity. There was evidence of statistical heterogeneity, indicating that the estimates varied more than expected by chance. The I2 was 87.6% for sensitivity and 92.1% for specificity (Cochran’s Q-test, p < 0.001 for both), indicating substantial heterogeneity. Deek’s test indicated no evidence of funnel plot asymmetry (p = 0.33) (Figure 4).
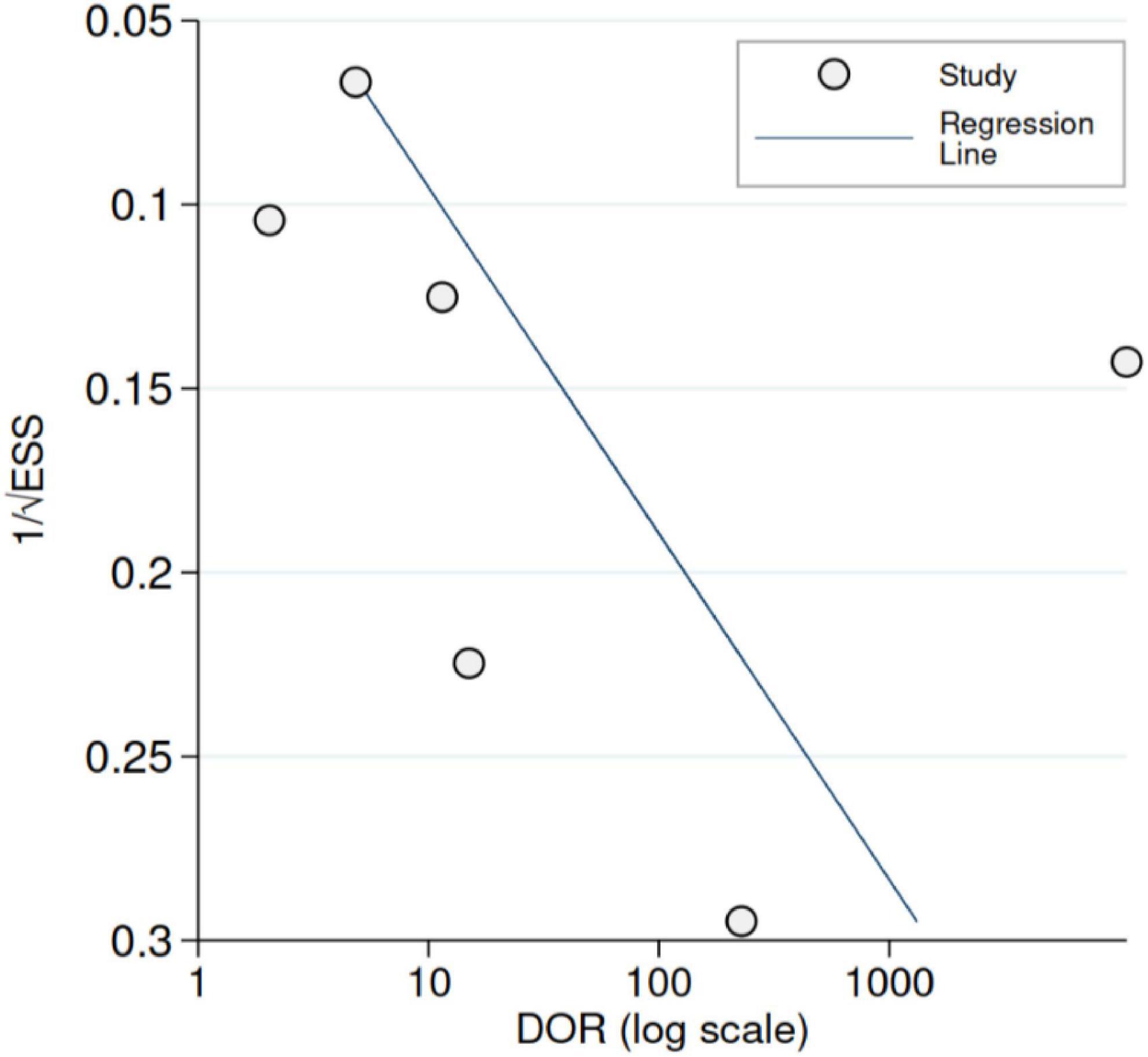
Figure 4. Funnel plot with Deek’s regression line [predicted diagnostic odds ratio (DOR)]. ESS denotes effective sample size. Deek’s test (p = 0.33).
Figure 5 shows the HSROC. The area under the HSROC was 0.97 (95% CI 0.72–1.00) and the summary DOR was 140 (95% CI 3.0–7564, p < 0.001). However, the predictive region showed large uncertainty regarding true accuracy to be estimated in a new study.
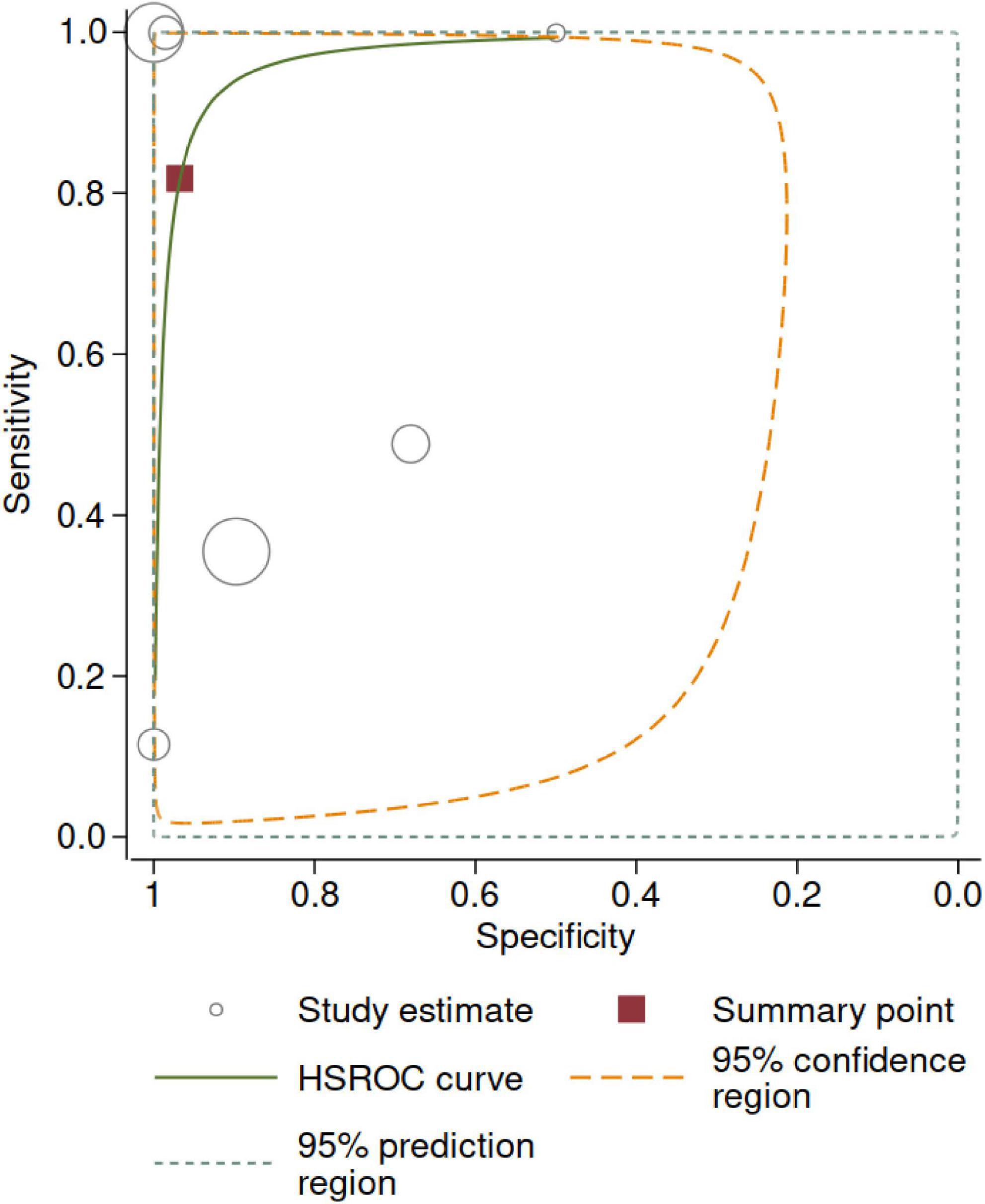
Figure 5. Hierarchical summary receiver operating curve (HSROC). The size of the circles is proportional to the number of participants in each study. The 95% confidence region represents the uncertainty around the summary point. The 95% prediction region represents the uncertainty regarding the estimate to be obtained in a new, well-conducted study—given the observed data (i.e., current levels of statistical heterogeneity).
Certainty of the evidence (GRADE)
Table 2 summarizes the GRADE assessment based on the meta-analysis of 6 studies. Overall, the certainty of the evidence was “very low”, primarily because of the risk of bias, inconsistency, and imprecision.
Summary of evidence
Table 2 provides a summary of the main findings. Incorporated in the table are the explanations for grading of the evidence. We presented hypothetical testing outcomes for 1,000.
Discussion
In this systematic review and meta-analysis, existing evidence on the diagnostic accuracy of prenatal image for congenital Zika syndrome was characterized by (1) substantial heterogeneity; (2) studies clustered in Brazil show that approximately half of the fetuses born out of ZIKV-infected mothers have abnormalities in the prenatal US imaging; (3) studies conducted around the world show no or minimal association between fetus prenatal imaging and the CZS hallmarks; (4) despite their heterogeneity, all studies confirmed the appearance of major anomalies of the central nervous system, including ventriculomegaly, cortical atrophy, calcifications, and anomalies of the corpus callosum; (5) less commonly reported abnormalities include intracranial calcifications at the gray matter–white matter junction, basal ganglia, and/or thalamus; cortical migrational abnormalities; skull with a collapsed appearance with overlapping sutures and redundant skin fold; intracranial herniation of orbital fat; and clot in the confluence of sinuses.
Studies clustered in Brazil show higher proportion of abnormalities related to CZS. The study by Sarno, Pires, Pereira, Carvalhlo, Brasil, and colleagues clearly states the proportion of CZS abnormalities ranging from a quarter up to 80% in some cases (32, 33, 41, 42). Of highlight is the report by Pomar et al. that one-third of cases show severe manifestation of CZS at birth or fetal loss. The remaining studies from Spain, France, Guyana, and Trinidad and Tobago describe lower proportion of abnormalities observed in prenatal imaging related to maternal ZIKV infection. These studies show fetus abnormalities in < 20% of cases.
Strengths and limitations
This review has several strengths. The search strategies were sensitive, extensive, updated, and peer-reviewed by an information specialist. Then, the study protocol was prospectively designed and registered with PROSPERO in order to reduce bias.
This systematic review, like similar studies, has some limitations. During the meta-analysis, we compared pooled estimates between different study populations. None of the studies that were included provided head-to-head comparison between MRI and US.
Interpretation of results
Caution in the interpretation of the results is warranted. In this study, there was a high proportion of studies at high risk of bias, and with high concern regarding applicability across all domains. These findings reduce the validity and applicability of the findings. The lack of standardization of definitions of brain abnormalities and lack of similar time follow-up are the limitations of the study. A plausible solution is conducting long-term follow-up studies to accurately assign newborns and infants suffering from CZS. The use of various instruments and algorithms to perform US or MRI definitively could affect the diagnosis of the cases.
Future research
Long-term studies are currently warranted to clarify the clinical and developmental relevance of any CZS abnormality findings. Such studies should first clarify the role of imaging abnormalities in predicting CZS, as well as differentiating it from other congenital infections. This can be accomplished by elaborating diagnostic accuracy studies: performance of the index test in a standardized and blinded manner and ensuring that the reference standard is performed on all participants.
One plausible approach includes the use of artificial intelligence to accurately identify additional abnormalities that could suggest CZS. Also, long-term studies looking at postnatal neuroimaging in infants who had normal prenatal imaging could reveal new mild abnormalities. Perhaps, the term congenital Zika syndrome is preferable to refer to cases that include microcephaly and other clinical signs of this congenital malformation disorder. Such endeavor will help in defining combined findings from clinical, laboratory, imaging, and pathological examinations that provide a more complete picture of the severe damage and developmental abnormalities caused by ZIKV infection.
Conclusion
The evidence supporting the accuracy of prenatal image for early diagnosis of congenital Zika syndrome remains low. The available evidence is in urgent need of development of standardized algorithms that could identify the whole spectrum of the disease. For now, we recommend the integration of prenatal and postnatal imaging and blood biomarkers to fully evaluate the risk assessment of fetus born to maternal infected women.
Data availability statement
All coded data will be available upon reasonable request to corresponding author, provided the citation of this study. The protocol can be accessed at doi: 10.1097/MD9.0000000000000183.
Author contributions
TH: conceptualization. TH, IC-B, AG, and TVP: data curation. TH, IC-B, and TVP: formal analysis. TH, IC-B, and AG: investigation and software. TH and TVP: methodology. TH: writing the original draft. IC-B, AG, and TVP: writing – review, and editing. All authors contributed to the article and approved the submitted version.
Funding
This research was funded by the Secretaria Nacional de Ciencia, Tecnología e Innovación (SENACYT) Sistema Nacional de Investigación de Panamá grant nos. 216-2017, 220-2019, and 22-2020 and the APC was funded by Sistema Nacional de Investigación. TVP was funded by the Chevening Scholarship Programme (Foreign and Commonwealth Office, United Kingdom).
Acknowledgments
The authors thank Deirdre Beecher, Senior Metadata Specialist at Cochrane, for her valuable help in verifying the search strategy for this work.
Conflict of interest
The authors declare that the research was conducted in the absence of any commercial or financial relationships that could be construed as a potential conflict of interest.
Publisher’s note
All claims expressed in this article are solely those of the authors and do not necessarily represent those of their affiliated organizations, or those of the publisher, the editors and the reviewers. Any product that may be evaluated in this article, or claim that may be made by its manufacturer, is not guaranteed or endorsed by the publisher.
Supplementary material
The Supplementary Material for this article can be found online at: https://www.frontiersin.org/articles/10.3389/fmed.2022.962765/full#supplementary-material
References
1. Schaub B, Gueneret M, Jolivet E, Decatrelle V, Yazza S, Gueye H., et al. Ultrasound imaging for identification of cerebral damage in congenital ZIKV syndrome: a case series. Lancet Child Adolsc Health. (2017) 1:45–55. doi: 10.1016/S2352-4642(17)30001-9
2. Relich RF, Loeffelholz M. Zika Virus. Clin Lab Med. (2017) 37:253–67. doi: 10.1016/j.cll.2017.01.002
3. Conners EE, Lee EH, Thompson CN, McGibbon E, Rakeman JL, Iwamoto M, et al. Zika virus infection among pregnant women and their neonates in New York Ci(y, January 2016-June 2017. Obstet Gynecol. (2018) 132:487. doi: 10.1097/AOG.0000000000002737
4. Sanz Cortes M, Rivera AM, Yepez M, Guimaraes CV, Yunes ID, Bs AZ, et al. Clinical assessment and brain findings in a cohort of mothers, fetuses and infants infected with ZIKA virus. Am J Obstet Gynecol. (2018) 218:440. doi: 10.1016/j.ajog.2018.01.012
5. C Lage ML, Carvalho AL, Ventura PA, Taguchi TB, Fernandes AS, Pinho SF, et al. Clinical, neuroimaging, and neurophysiological findings in children with microcephaly related to congenital zika virus infection. Int J Environ Res Public Health. (2019) 16:309. doi: 10.3390/ijerph16030309
6. Musso D, Ko AI, Baud D. Zika Virus Infection - After the Pandemic. N Engl J Med. (2019) 381:1444–57. doi: 10.1056/NEJMra1808246
7. Wheeler AC. Development of infants with congenital zika syndrome: what do we know and what can we expect? Pediatrics. (2018) 141:S154–60. doi: 10.1542/peds.2017-2038D
8. Saad T, PennaeCosta AA, de Góes FV, de Freitas M, de Almeida JV, de Santa Ignêz LJ, et al. Neurological manifestations of congenital ZIKV infection. Childs Nerv Syst. (2018) 34:73–8. doi: 10.1007/s00381-017-3634-4
9. Citil Dogan A, Wayne S, Bauer S, Ogunyemi D, Kulkharni SK, Maulik D, et al. The ZIKV and pregnancy: evidence, management, and prevention. J Matern Fetal Neonatal Med. (2017) 30:386–96. doi: 10.3109/14767058.2016.1174210
10. Guibaud L, Lacalm A. Diagnostic imaging tools to elucidate decreased cephalic biometry and fetal microcephaly: a systematic analysis of the central nervous system. Ultrasound Obstet Gynecol. (2016) 48:16–25. doi: 10.1002/uog.15926
11. Eppes C, Rac M, Dunn J, Versalovic J, Murray KO, Suter MA, et al. Testing for ZIKV infection in pregnancy: key concepts to deal with an emerging epidemic. Am J Obstet Gynecol. (2017) 216:209–25. doi: 10.1016/j.ajog.2017.01.020
12. Society for Maternal Fetal Medicine statement. Ultrasound Screening for Fetal Microcephaly Following ZIKV Exposure. Washington, DC: Society for Maternal Fetal Medicine statement (2019).
13. Papageorghiou AT, Thilaganathan B, Bilardo CM, Ngu A, Malinger G, Herrera M, et al. ISUOG Interim Guidance on ultrasound for ZIKV infection in pregnancy: information for healthcare professionals. Ultrasound Obstet Gynecol. (2016) 47:530–2. doi: 10.1002/uog.15896
14. Khalil A, Sotiriadis A, Chaoui R, da Silva Costa F, D’Antonio F, Heath PT, et al. ISUOG practice guidelines: role of ultrasound in congenital infection. Ultrasound Obstet Gynecol. (2020) 56:128–51. doi: 10.1002/uog.21991
15. Meaney-Delman D, Rasmussen SA, Staples JE, Oduyebo T, Ellington SR, Petersen EE, et al. Zika virus and pregnancy: what obstetric health care providers need to know. Obstet Gynecol. (2016) 127:642–8. doi: 10.1097/AOG.0000000000001378
16. Viens LJ, Fleck-Derderian S, Baez-Santiago MA, Oduyebo T, Broussard CS, Khan S, et al. Role of prenatal ultrasonography and amniocentesis in the diagnosis of congenital zika syndrome: a systematic review. Obstet Gynecol. (2020) 135:1185–97. doi: 10.1097/AOG.0000000000003829
17. Wu S, Zeng Y, Lerner A, Gao B, Law M. Nervous system injury and neuroimaging of zika virus infection. Front Neurol. (2018) 9:227. doi: 10.3389/fneur.2018.00227
18. Radaelli G, Lahorgue Nunes M, Bernardi Soder R, Monteiro de Oliveira J, Thays Konat Bruzzo F, Kalil Neto F, et al. Review of neuroimaging findings in congenital ZIKV syndrome and its relation to the time of infection. Neuroradiol J. (2020) 33:152–7. doi: 10.1177/1971400919896264
19. Walker CL, Little ME, Roby JA, Armistead B, Gale M Jr., Rajagopal L, et al. ZIKV and the nonmicrocephalic fetus: why we should still worry. Am J Obstet Gynecol. (2019) 220:45–56. doi: 10.1016/j.ajog.2018.08.035
20. Adhikari EH, Nelson DB, Johnson KA, Jacobs S, Rogers VL, Roberts SW, et al. Infant outcomes among women with ZIKV infection during pregnancy: results of a large prenatal Zika screening program. Am J Obstet Gynecol. (2017) 216:292. doi: 10.1016/j.ajog.2017.01.018
21. Pool KL, Adachi K, Karnezis S, Salamon N, Romero T, Nielsen-Saines K, et al. Association Between Neonatal Neuroimaging and Clinical Outcomes in Zika-Exposed Infants From Rio de Janeiro, Brazil. JAMA Netw Open. (2019) 2:e198124. doi: 10.1001/jamanetworkopen.2019.8124
22. Sotiriadis A, Papatheodorou SI, Martins WP. Synthesizing evidence from diagnostic accuracy tests: the SEDATE guideline. Ultrasound Obstet. Gynecol. (2016) 47:386–95. doi: 10.1002/uog.15762
23. McInnes MDF, Moher D, Thombs BD, McGrath TA, Bossuyt PM Prisma-Dta Group. Preferred reporting items for a systematic review and meta-analysis of diagnostic test accuracy studies: The PRISMA-DTA Statement. JAMA. (2018) 319:388–96. doi: 10.1001/jama.2017.19163
24. Herrera T, Cubilla-Batista I, Goodridge A. Prenatal imaging for the diagnosis of congenital Zika syndrome. Med Case Reports Study Protoc [Internet]. (2022) 3:e0183. Available online at: https://journals.lww.com/md-cases/Fulltext/2022/02000/Prenatal_imaging_for_the_diagnosis_of_congenital.4.aspx
25. Ciapponi A, Matthews S, Cafferata ML, Comandé D, Gibbons L, Núñez-González S, et al. Laboratory tests for diagnosis of congenital Zika virus in fetuses and neonates. Cochrane Database Syst Rev [Internet]. (2020).
26. COCHRANE. Data extraction forms | Cochrane Developmental, Psychosocial and Learning Problems [Internet]. (2021) Available online at: https://dplp.cochrane.org/data-extraction-forms
27. Whiting PF, Rutjes AWS, Westwood ME, Mallett S, Deeks JJ, Reitsma JB, et al. Quadas-2: A revised tool for the quality assessment of diagnostic accuracy studies. Ann Intern Med. (2011) 155:529–36.
28. GRADEpro GDT: GRADEpro Guideline Development Tool [Software]. McMaster University and Evidence Prime (2022). Available online at: https://gradepro.org/gradepro.org
29. Rutter CM, Gatsonis CA A hierarchical regression approach to meta-analysis of diagnostic test accuracy evaluations. Stat Med Stat Med. (2001) 20:2865–84.
30. Glas AS, Lijmer JG, Prins MH, Bonsel GJ, Bossuyt PMM The diagnostic odds ratio: A single indicator of test performance. J Clin Epidemiol. (2003) 56:1129–35.
31. Pereira TV, Patsopoulos NA, Salanti G, Ioannidis JPA Critical interpretation of Cochran’s Q test depends on power and prior assumptions about heterogeneity. Res Synth Methods [Internet]. (2010) 1:149–61.
32. Carvalho FHC, Cordeiro KM, Peixoto AB, Tonni G, Moron AF, Feitosa FEL, et al. Associated ultrasonographic findings in fetuses with microcephaly because of suspected Zika virus (ZIKV) infection during pregnancy. Prenat Diagn [Internet]. (2016) 36:882–7.
33. Sarno M, Aquino M, Pimentel K, Cabral R, Costa G, Bastos F, et al. Progressive lesions of central nervous system in microcephalic fetuses with suspected congenital Zika virus syndrome. Ultrasound Obstet Gynecol. (2017) 50:717–22.
34. De Oliveira Melo AS, Aguiar RS, Amorim MMR, Arruda MB, De Oliveira Melo F, Ribeiro STC, et al. Congenital zika virus infection: Beyond neonatal microcephaly. JAMA Neurol [Internet]. (2016) 73:1407–16. Available online at: https://jamanetwork.com/journals/jamaneurology/fullarticle/2557231
35. Sohan K, Cyrus CA Ultrasonographic observations of the fetal brain in the first 100 pregnant women with Zika virus infection in Trinidad and Tobago. Int J Gynecol Obstet [Internet]. (2017) 139:278–83. Available online at: https://onlinelibrary.wiley.com/doi/full/10.1002/ijgo.12313
36. Rodó C, Suy A, Sulleiro E, Soriano-Arandes A, Maiz N, García-Ruiz I, et al. Pregnancy outcomes after maternal Zika virus infection in a non-endemic region: prospective cohort study. Clin Microbiol Infect [Internet]. (2019) 25:633.e5–633.e9. Available online at: http://www.clinicalmicrobiologyandinfection.com/article/S1198743X19300540/fulltext
37. Marbán-Castro E, Goncé A, Fumadó V, Martínez MJ, López M, García-Otero L, et al. Zika virus infection in pregnant travellers and impact on childhood neurodevelopment in the first two years of life: A prospective observational study. Travel Med Infect Dis. (2021) 40:101985.
38. Walker CL, Ehinger N, Mason B, Oler E, rèse Little M-TE, Ohuma EO, et al. Ultrasound prediction of Zika virus-associated congenital injury using the profile of fetal growth. doi: 10.1371/journal.pone.0233023
39. Walker CL, Merriam AA, Ohuma EO, Dighe MK, Gale M, Rajagopal L, et al. Femur-sparing pattern of abnormal fetal growth in pregnant women from New York city after maternal zika virus infection. Am J Obstet Gynecol [Internet]. 219:187.e1.
40. Mulkey SB, Bulas DI, Vezina G, Fourzali Y, Morales A, Arroyave-Wessel M, et al. Sequential neuroimaging of the fetus and newborn with in utero zika virus exposure. JAMA Pediatr [Internet]. (2019) 173:52.
41. Pires P, Jungmann P, Galvão JM, Hazin A, Menezes L, Ximenes R, et al. Neuroimaging findings associated with congenital Zika virus syndrome: case series at the time of first epidemic outbreak in Pernambuco State, Brazil. Child’s Nerv Syst. (2017) 34:957-63. doi: 10.1007/s00381-017-3682-9
42. Pereira JP, Nielsen-Saines K, Sperling J, Maykin MM, Damasceno L, Cardozo RF, et al. Association of prenatal ultrasonographic findings with adverse neonatal outcomes among pregnant women with zika virus infection in Brazil. JAMA Netw Open [Internet]. (2018) 1:e186529–e186529.
43. Besnard M, Eyrolle-Guignot D, Guillemette-Artur P, Lastère S, Bost-Bezeaud F, Marcelis L, et al. Congenital cerebral malformations and dysfunction in fetuses and newborns following the 2013 to 2014 Zika virus epidemic in French Polynesia. Eurosurveillance [Internet]. (2016) 21:30181. doi: 10.2807/1560-7917.ES.2016.21.13.30181
44. Nogueira M, Nery Júnior N, Estofolete C, Bernardes Terzian A, Guimar G, Zini N, et al. Adverse birth outcomes associated with Zika virus exposure during pregnancy in São Jose do Rio Preto, Brazil. Clin Microbiol Infect [Internet]. (2018) 24:646. doi: 10.1016/j.cmi.2017.11.004
45. Coutinho CM, Negrini SFBM, Araujo DCA, Teixeira SR, Amaral FR, Moro MCR, et al. Early maternal Zika infection predicts severe neonatal neurological damage: results from the prospective Natural History of Zika Virus Infection in Gestation cohort study. BJOG An Int J Obstet Gynaecol [Internet]. (2021) 128:317–26. doi: 10.1111/1471-0528.16490
46. Gutiérrez-Sánchez LÁ, Becerra-Mojica CH, Rojas MA, Díaz-Martínez LA, Pérez Vera LA, Contreras García GA, et al. Fetal central nervous system anomalies according to RT-PCR and trimester of maternal infection with Zika virus: A prospective cohort study. Acta Obstet Gynecol Scand [Internet]. (2022) 101:221–31. doi: 10.1111/aogs.14301
47. Pomar L, Vouga M, Lambert V, Pomar C, Hcini N, Jolivet A, et al. Maternal-fetal transmission and adverse perinatal outcomes in pregnant women infected with Zika virus: prospective cohort study in French Guiana. BMJ [Internet]. (2018) 363:4431. Available online at: https://www.bmj.com/content/363/bmj.k4431
Keywords: congenital Zika syndrome (CZS), Zika virus infection, pregnancy, neurosonology, ventriculomegaly, ZIKV, microcephaly
Citation: Herrera TT, Cubilla-Batista I, Goodridge A and Pereira TV (2022) Diagnostic accuracy of prenatal imaging for the diagnosis of congenital Zika syndrome: Systematic review and meta-analysis. Front. Med. 9:962765. doi: 10.3389/fmed.2022.962765
Received: 06 June 2022; Accepted: 29 July 2022;
Published: 29 September 2022.
Edited by:
Raigam Jafet Martinez-Portilla, Instituto Nacional de Perinatología (INPER), MexicoReviewed by:
Feymi Areli Gutiérrez López, Instituto Nacional de Perinatología (INPER), MexicoJohnatan Torres, Instituto Nacional de Perinatología (INPER), Mexico
Copyright © 2022 Herrera, Cubilla-Batista, Goodridge and Pereira. This is an open-access article distributed under the terms of the Creative Commons Attribution License (CC BY). The use, distribution or reproduction in other forums is permitted, provided the original author(s) and the copyright owner(s) are credited and that the original publication in this journal is cited, in accordance with accepted academic practice. No use, distribution or reproduction is permitted which does not comply with these terms.
*Correspondence: Tania T. Herrera, dGFuaWFoMzBAZ21haWwuY29t
†These authors have contributed equally to this work