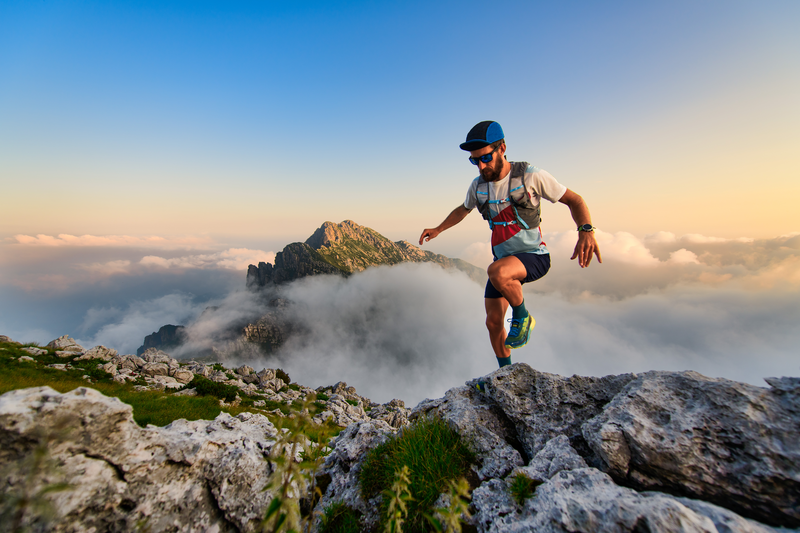
94% of researchers rate our articles as excellent or good
Learn more about the work of our research integrity team to safeguard the quality of each article we publish.
Find out more
ORIGINAL RESEARCH article
Front. Med. , 01 July 2022
Sec. Ophthalmology
Volume 9 - 2022 | https://doi.org/10.3389/fmed.2022.956339
This article is part of the Research Topic New Perspectives in Glaucoma Pathophysiology, Diagnosis, and Treatment View all 15 articles
Background: Glaucoma is hypothesized to originate in the brain but manifests as an eye disease as it possesses the common features of neurodegeneration diseases. But there is no evidence to demonstrate the primary brain changes in glaucoma patients. In the present study, we have used Mendelian randomization (MR) to understand the causal effect of brain alterations on glaucoma.
Methods: Our MR study was carried out using summary statistics from genome-wide associations for 110 diffusion tensor imaging (DTI) measurements of white matter (WM) tracts (17,706 individuals), 101 brain region-of-interest (ROI) volumes (19,629 individuals), and glaucoma (8,591 cases, 210,201 control subjects). The causal relationship was evaluated by multiplicative random effects inverse variance weighted (IVW) method and verified by two other MR methods, including MR Egger, weighted median, and extensive sensitivity analyses.
Results: Genetic liability to fornix fractional anisotropy (FX.FA) (OR = 0.71, 95%CI = 0.56–0.88, P = 2.44 × 10–3), and uncinate fasciculus UNC.FA (OR = 0.65, 95%CI = 0.48–0.88, P = 5.57 × 10–3) was associated with a low risk of glaucoma. Besides, the right ventral diencephalon (OR = 1.72, 95%CI = 1.17–2.52, P = 5.64 × 10–3) and brain stem (OR = 1.35, 95%CI = 1.08–1.69, P = 8.94 × 10–3) were associated with the increased risk of glaucoma. No heterogeneity and pleiotropy were detected.
Conclusion: Our study suggests that the fornix and uncinate fasciculus degenerations and injures of the right ventral diencephalon and brain stem potentially increase the occurrence of glaucoma and reveal the existence of the brain-eye axis.
Glaucoma is one of the common blinding disorders characterized by progressive damage to the retinal ganglion cells (RGCs) along with visual field loss (1). Many elements are considered major risk factors, including intraocular pressure (IOP), genes, family factors, vascular factors, and high myopia (2, 3). However, elevated IOP is the only modifiable risk factor; thus, IOP reduction is considered a helpful treatment until now (4, 5). However, whereas many normal IOP individuals suffer from the disorder, IOP reduction could not completely stem the glaucoma progression (6). This suggests that it is necessary to find other effective ways to alleviate glaucomatous neurodegeneration.
The eye is the extension of the brain and has many embryological, functional, and developmental similarities with the central nervous system (7). As the hallmark of glaucoma, the primary cells to die in glaucoma are RGCs, which are typical neurons (8). Although the cell bodies of RGCs lie in the retina, most portions of axons lie outside the ocular area, which forms the optic nerve, optic chiasm, and optic tract (8). Besides, many observational studies have shown that glaucoma patients had cognition and memory decline (9, 10). In addition, motor coordinating impairment and psychological disorders such as depression and anxiety were prevalent among the glaucoma patients (11, 12). Based on these theories, various scholars hypothesize that brain tissues are associated with glaucoma pathogenesis (13, 14).
Magnetic resonance imaging (MRI) studies are an important tool as a non-invasive method to evaluate the structure, function, and neurochemistry of the brain (15). Some researchers investigated the structural and functional brain changes in patients with glaucoma using MRI. They reported white matter structural abnormalities in different parts of the visual pathway and altered brain connectivity beyond the visual system (16, 17). These observations led to the question of whether brain changes precede or follow the RGCs degeneration. Although an anterograde tans-synaptic degeneration might be responsible for some visual pathway damage, the brain structures outside the visual pathway generally reflect a primary neuropathological process. Besides, due to the high cost and limited accessibility to MRI facilities, it is hard to complete randomized, controlled trials to explore the primary brain changes in glaucoma patients.
Mendelian randomization (MR) uses a genetic variation to evaluate the inter-causality of disease risk factors, which is not affected by most acquired confounding factors (such as environment) (18). Thus far, MR has been used to derive the causal relationship between kidney damage and brain cortical structure, brain structures, and neuropsychiatric disorders, including Alzheimer’s disease (AD) (19, 20). Recently, a GWAS study reported an association of single nucleotide polymorphisms (SNPs) of brain structural measurements with glaucoma (21–24). These studies bought insight into finding the causal relationship between glaucoma and brain structures. Thus, we aimed to identify the causal relationship between white matter (WM) structures [diffusion tensor imaging (DTI), brain subregion volumes region of interest (ROI)], and glaucoma by using the two-sample MR analysis. Our results indicate the potential risks of glaucoma in the brain and provide new insight into the possible existence of the brain-eye axis.
The valid genetic instrumental variables (IVs) in the MR study must fulfill the 3 assumptions (Figure 1): (1) The IVs must be associated with DTI parameters of WM tracts and ROI volumes. (2) The IVs must not be associated with factors that confound the relationship between DTI parameters of WM tracts and glaucoma, ROI volumes, and glaucoma. (3) The IVs are only associated with glaucoma through DTI parameters of WM tracts and ROI volumes.
Figure 1. Mendelian Randomization Analysis Overview. Overview of Mendelian randomization analyses and major assumptions. DTI, indicates diffusion tensor imaging; WM, white matter; ROI, region of interest; FA, fractional anisotropy; MD, mean diffusivity; AD, axial diffusivity; RD, radial diffusivity; MO, mode of anisotropy.
The genetic variants of DTI parameters of WM traces were taken from the genome-wide association studies (GWAS) of Zhao et al. (21). This GWAS study consisted of a total of 110 DTI parameters from 17,706 subjects of European ancestry, including fractional anisotropy (FA), mean diffusivity (MD), axial diffusivity (AD), radial diffusivity (RD), mode of anisotropy (MO), and the average values of 21 WM tracts. The 21 WM tracts were labeled through the Enhancing NeuroImaging Genetics through Meta-Analysis (ENIGMA) DTI pipeline (22, 23), and the full names of the tracts are shown in Supplementary Table 1.
The genetic variants of the ROI volumes came from the genome-wide association studies (GWAS) study of Zhao et al. (24). This GWAS study analyzed MRI data from 19,629 European participants through consistent procedures via normalization tools (ANTs)1 and used Mindboggle-101 atlas for labeling.
The single nucleotide polymorphisms (SNPs) related to DTI and ROI were screened to obtain the required IVs. First, the SNPs associated with DTI or ROI exhibited genome-wide significance (P < 5 × 10–8). Also, SNPs with linkage disequilibrium (LD) were removed (R2 < 0.001) in the LD data from the 1,000 Genomes Project (25). In addition, we removed the SNPs with the presence of palindromes. Finally, to clarify the direction of causality, all SNPs were examined using MR Steiger (26), and the SNPs that could lead to causality were removed.
To avoid the overlapping between DTI and ROI-related and glaucoma-related data sources as much as possible, the association of glaucoma relative SNPs was obtained from the FinnGen consortium (Release 5, May 11, 2021, comprising 218,792 individuals). This study defines glaucoma by H40-H42 of the International Classification of Disease-10 (ICD-10). Genotype data from the FinnGen research project included 8,591 glaucoma patients of Finnish origin and 210,201 control subjects.
To test the robustness of the MR estimates, we applied sensitivity analysis. First, MR Egger’s intercept values were used to assess the multiplicity of SNPs. The closer the intercept to 0, the lower the multiplicity was considered. Meanwhile, MR-Pleiotropy RESidual Sum and Outlier (PRESSO) (27) was used to evaluate further the SNPs pleiotropy that has the potential causality, and the SNPs with abnormalities were removed. Meanwhile, Cochran’s Q test was used for the assessment of heterogeneity. Finally, to ensure the robustness of the results, the analysis was carried out using the leave-one-out test.
“Two Sample MR” packages were used to estimate the MR results using the R software (version 1.4.1717). The Wald ratio was used to assess the causal effect of individual SNPs. Provided that sensitivity analysis was passed, inverse variance weighting (IVW) was used as the main method to assess MR effects. The weighted median (28) and MR-Egger regression (27) were used as additional methods to determine the MR results validity further. P < 0.05 was considered to have potential causality.
A total of 394 SNPs were selected to predict 81 DTI parameters of WM traces genetically, and 389 SNPs were used to predict 61 ROI volumes (Supplementary Tables 2, 3). An overview of the flow charts is shown in Figure 1.
We evaluated the DTI-Glaucoma association by Mendelian randomization analysis, and the specific statistical results are shown in Figure 2 and Supplementary Table 4. There were two FA parameters of the white matter that reached statistical significance: fornix fractional anisotropy (FX.FA) and uncinate fasciculus fractional anisotropy (UNC.FA) (Table 1). Then, IVW was used to confirm that FX.FA (OR = 0.71, 95%CI = 0.56–0.88, P = 2.44 × 10–3), UNC.FA (OR = 0.65, 95%CI = 0.48–0.88, P = 5.57 × 10–3) was associated with a low risk of glaucoma. Additionally, we further validated the results of IVW by weighted median (FX.FA: P = 1.03 × 10–2; UNC.FA: P = 2.42 × 10–2) and MR-egger (FX.FA: P = 0.614, UNC.FA: P = 0.735) (Table 1).
Figure 2. MR results for DTI-Glaucoma associations. Each radius represents OR of one DTI measurement (red dot: MR-IVW; yellow dot: orange dot; blue dot: MR-Egger). From outer to inner layer: p-value for MR-IVW; OR for MR-IVW; p-value for MR-WM; OR for MR-WM; p-value for MR Egger; OR for MR-Egger. The dotted gray line indicated OR = 1.
MR genetic instruments should only impact the outcome via exposure and not any other pathway (horizontal pleiotropy and heterogeneity). Therefore, we confirmed our results by applying sensitivity tests (Supplementary Tables 5, 6). The result showed little pleiotropy (FX.FA: P = 0.91 and UNC.FA: P = 0.67) and heterogeneity [FX.FA (IVW: P = 0.65; MR Egger: P = 0.76) and UNC.FA (IVW: P = 0.53; MR Egger: P = 0.70)] (Table 2). Further, MR-PRESSO demonstrated no significant horizontal pleiotropy of FX.FA (P = 0.762). The MR-PRESSO test could not be performed due to insufficient SNPs in UNC.FA. Additionally, the leave-one-out method confirmed the robustness of the causal association in FX.FA-Glaucoma and UNC.FA-Glaucoma pairs (Supplementary Figures 1A,B). Considering no heterogeneity or horizontal pleiotropy was present in the selected SNPs after sensitivity testing, the results of IVW were more reliable. Therefore, these results demonstrated that reducing fornix and uncinate fasciculus fractional anisotropy made a causal contribution to glaucoma.
By Mendelian randomization analysis, we found that the volumes of the right ventral diencephalon (right.Ventral.DC) and brain stem (Brain.stem) had a significant causal relationship with glaucoma (Table 3). The statistical results for the other regions are shown in Figure 3 and Supplementary Table 7. The results of IVW confirmed the volume of the right.ventral.DC (OR = 1.72, 95%CI = 1.17–2.52, P = 5.64 × 10–3) and Brain.stem (OR = 1.35, 95%CI = 1.08–1.69, P = 8.94 × 10–3) were positively associated with glaucoma risk. Meanwhile, weighted median (right.ventral.DC: P = 1.10 × 10–3; Brain.stem: P = 5.06 × 10–3) and MR-Egger (right.ventral.DC: P = 0.578; Brain.stem: P = 0.994). were used to verify the findings further.
Figure 3. MR results for ROI-Glaucoma associations. Each radius represents one ROI measurement. From outer to inner layer: MR results analyzed with IVW; MR results analyzed with WM; MR results analyzed with MR-Egger; heterogeneity of each ROI measure. The red dot represents the ROI-Glaucoma relation. The X-axis shows the beta value, and Y-axis shows the p-value. The dotted gray line indicated the nominal p threshold (0.05).
We performed pleiotropic analysis (Supplementary Table 8) and heterogeneity tests (Supplementary Table 9). By calculating the MR-Egger intercept, no significant horizontal pleiotropy was observed in the right.ventral.DC (intercept = 0.003, P = 0.95) and Brain.stem (intercept = 0.018, P = 0.62) (Table 4). MR-PRESSO further validated the absence of significant horizontal pleiotropy (right.ventral.DC: P = 0.430; Brain.stem: P = 0.126). Besides, the results of heterogeneity tests showed that the right.ventral.DC (IVW: Q = 5.67, P = 0.22; MR-Egger: Q = 5.66, P = 0.13) and Brain.stem (IVW:Q = 17.92, P = 0.12); MR-Egger: Q = 17.51, P = 0.09) associated SNPs that were not significantly heterogeneous (Table 4). Meanwhile, the leave-one-out test confirmed the causal association in the right.ventral.DC-Glaucoma and Brain.stem-Glaucoma pairs (Supplementary Figures 1C,D). Therefore, the results of IVW were more reliable as no heterogeneity or horizontal pleiotropy was present in the selected SNPs after sensitivity testing. Thus the inferred causality between right.ventral.DC-glaucoma and Brain.stem-Glaucoma pairs were plausible.
To the best of our knowledge, this is the first MR analysis that leveraged population-scale human genetics to understand the causal relationship between neuroimaging polymorphisms and glaucoma in an unbiased manner. The imaging changes in the brain could not be directly associated as a cause of glaucoma in many current neuroimaging studies. However, our results show that the changes in the microstructures of the white matter and the volume of specific brain regions can affect the onset of glaucoma and reveal the existence of the brain-eye axis.
Large cohort studies have confirmed that patients with glaucoma have shown progressive vision loss even with a significant reduction in IOP (6). This finding indicates that IOP-independent mechanisms contribute to the etiopathogenesis of this disorder. Recently, there has been growing attention about the brain changes in glaucoma. In particular, numerous MRI studies performed on glaucoma patients have shown brain structural differences. Hernowo et al. (29) found decreased volume along the full length of the visual pathway for both gray and white matter in a follow-up study of glaucoma patients. Moreover, changes in the brain beyond the primary visual pathways, including the middle occipital gyrus, inferior temporal gyrus, the anterior thalamic radiation, corticospinal tract, superior temporal gyrus, and occipital lobe white matter, were also reported (30–32). However, the distinction between these brain changes as cause or consequence remains difficult as nearly all current literatures are cross-sectional with limited population and testing methods. Thus, understanding the brain-eye interactions across disease severity could provide valuable insights into the pathogenesis of glaucoma and lead to new treatment modalities.
MRI provides a non-invasive method to evaluate structural, metabolic, and functional changes in the brain. Diffusion-weighted imaging measures the 3-dimensional displacement of water molecules and provides important information about changes in the microstructures of the white matter (33). Many studies have used DTI to describe the white matter integrity in glaucoma. Hui et al. (34) reported decreased FA and increased MD at the optic nerve for the first time in a rat model of glaucoma. Decreased FA at the proximal portion of the optic nerve and chiasm were also detected in glaucoma patients (35, 36). Meanwhile, recent DTI studies demonstrated that glaucoma patients had a lower FA within the visual white matter pathway, negatively correlated with glaucoma severity (37). Additionally, the decreased FA in the inferior fronto-occipital fasciculus, the longitudinal and inferior frontal fasciculi, the putamen, the caudate nucleus, the anterior and posterior thalamic radiations, and the anterior and posterior limbs of the internal capsule were reported in glaucoma (38). In our results, decreased FX.FA and UNC.FA was a novel risk factor for glaucoma. Lower FA values indicate the presence of axonal disruption and reduced structural integrity. The fornix carries the axons projecting from the hippocampus, and fornix FA reduces in subjects with hippocampal atrophy, correlating with memory function and emotion regulation (39). Uncinate fascicles are the connecting fibers of the medial and lateral orbitofrontal gyrus, anterior cingulate gyrus, and anterior temporal lobe, including the amygdala and hippocampus. Lower FA in the uncinate fasciculus is involved in emotional regulation, including bipolar disorder and late-life depression (40, 41). Of note, glaucoma patients were associated with a reduction in cognition, memory, and psychological disorders such as depression and anxiety (12). Moreover, the hippocampal shrinks in glaucoma patients were associated with disease severity (42). Given this evidence, we speculate that the FA reduction in the fornix and uncinate fasciculus may precede glaucoma’s hippocampal atrophy and clinical symptoms.
Using a similar methodology, we detected the increased volumes of the right.ventral.DC and Brain. stem are both risk factors for glaucoma. Brain stem is involved in many of the basic functions of the encephalon, such as motor control and sensory analysis. Consistent with our results, Williams et al. (31). reported the volume of brain stem was larger in the early stage of glaucoma group and the volume decline later stage of the disease. The ventral diencephalon (VDC) is a group of structures including the hypothalamus, papillary body, subthalamic nucleus, substantia nigra, red nucleus, lateral geniculate nucleus (LGN), and medial geniculate nucleus (MGN) that cannot be normally distinguished by standard MRI images. Several primate studies have reported LGN input with additional modulatory input from various brain regions (including the striate cortex, the brain stem, and the thalamic reticular nucleus) (43). Moreover, recent observations with experimentally induced glaucoma have confirmed the loss of LGN neurons in the layers associated with the eye (44). Increased volume is likely a result of inflammatory processes and neuronal injuries, such as cellular swelling or microglial activation (31). Therefore, the injuries of right ventral diencephalon and stem brain may induce irreversible damage to the optic nerve in glaucoma.
Many research hypotheses that glaucoma originates in the brain but manifest as an eye disease. One of these studies indicated that glaucoma should be seen as part of a neurological disorder. They found that trans-lamina cribrosa pressure difference (TLCPD), calculated as the IOP minus the cerebrospinal fluid pressure, had a better association with glaucoma presence than IOP alone (45). Other studies have revealed that a higher proportion of AD patients had a probable diagnosis of glaucoma than controls, while the primary open-angle glaucoma (POAG) patients had a higher risk of developing AD (46, 47). RGCs are located in the retinal ganglion cell layer, and the axons of RGCs constitute the optic nerve and reach up to the LGN. Retrograde trans-synaptic degeneration is currently responsible for RGC loss in various neurodegeneration diseases, including Alzheimer’s (AD), and Parkinson’s (PD) disease (48). Meanwhile, various neuroimaging research converges evidence that glaucoma is associated with central nervous system changes and shares commonality with neurodegeneration diseases, including loss of RGCs and the deposition of abnormal proteins in specific anatomical areas like the hippocampus. The primary brain alterations could induce the RGCs death through retrograde trans-synaptic degeneration. Besides, recent studies also considered that glaucoma shares the common pathogenic mechanisms (oxidative stress, mitochondrial dysfunction, inflammation, and apoptosis) with neurodegeneration diseases (49). Our study conducted hypothesis-free, data-driven MR analyses and identified FA reduction in the fornix and uncinate fasciculus and increased volume in right.ventral.DC and brain stem are the primary causes of glaucoma. Therefore, combined with previous results, our data suggest that brain damage is an important factor in the onset of glaucoma. Future molecular and clinical research is required to improve our understanding of the underlying mechanisms. These potential pathogenic mechanisms linking brain alterations to retinal physiology will probably lead to early diagnosis of glaucoma with more appropriate and accurate approaches for its treatment.
Although more reliable conclusions can be obtained using large-scale GWAS studies, some limitations should be considered for the present study. First, data used in the current study were derived from the European population; therefore, the causal relationship between brain structural alteration and glaucoma in other populations such as Asia is unknown. Therefore, further research on different ethnicities should be conducted to explore the variation. Second, although we assessed the associations between brain alteration and glaucoma, it is expected that more MRI metrics of larger sample sizes can be used in future MR studies to find out the precise lesions in the brain of glaucoma patients. Third, the brain alteration in different types of glaucoma might be different, and further studies considering the glaucoma group are required. So forth, it was difficult to achieve a full non-overlap between exposure (DIT and ROI) and outcome (Glaucoma), even though we chose the data from publicly available summary data.
In summary, FA reduction in the fornix and uncinate fasciculus and increased volume of the right ventral diencephalon and brain stem might be the primary causes of glaucoma. Furthermore, our study illustrates that the fornix and uncinate fasciculus degenerations and injuries of the right ventral diencephalon and stem brain potentially increase the risk of glaucoma onset. These novel findings support the hypothesis that brain alteration can trigger glaucoma, and can be utilized for the advancement of diagnostics and therapies for glaucoma in the future.
The datasets presented in this study can be found in online repositories. The names of the repository/repositories and accession number(s) can be found in the article/Supplementary Material.
Ethical review and approval was not required for the study on human participants in accordance with the local legislation and institutional requirements. Written informed consent for participation was not required for this study in accordance with the national legislation and the institutional requirements.
JZ: conceptualization and writing—review and editing. PW: methodology. KL: investigation and writing—original draft. BC: resources. RY and YC: supervision. All authors contributed to the article and approved the submitted version.
This study was supported by a grant from the National Natural Science Foundation of China (No. 81600713) to JZ.
The authors declare that the research was conducted in the absence of any commercial or financial relationships that could be construed as a potential conflict of interest.
All claims expressed in this article are solely those of the authors and do not necessarily represent those of their affiliated organizations, or those of the publisher, the editors and the reviewers. Any product that may be evaluated in this article, or claim that may be made by its manufacturer, is not guaranteed or endorsed by the publisher.
We thank the participants and investigators of the FinnGen study for their GWAS data.
The Supplementary Material for this article can be found online at: https://www.frontiersin.org/articles/10.3389/fmed.2022.956339/full#supplementary-material
Supplementary Figure 1 | Leave-one-out permutation analysis for the fornix fractional anisotropy (A), uncinate fasciculus fractional anisotropy (B), and the volume of right ventral diencephalon (C) and brain stem (D).
1. Tham YC, Li X, Wong TY, Quigley HA, Aung T, Cheng CY. Global prevalence of glaucoma and projections of glaucoma burden through 2040: a systematic review and meta-analysis. Ophthalmology. (2014) 121:2081–90. doi: 10.1016/j.ophtha.2014.05.013
2. McMonnies CW. Glaucoma history and risk factors. J Optom. (2017) 10:71–8. doi: 10.1016/j.optom.2016.02.003
3. Schuster AK, Wagner FM, Pfeiffer N, Hoffmann EM. Risk factors for open-angle glaucoma and recommendations for glaucoma screening. Ophthalmologe. (2021) 118:145–52. doi: 10.1007/s00347-021-01378-5
4. Lichter PR, Musch DC, Gillespie BW, Guire KE, Janz NK, Wren PA, et al. Interim clinical outcomes in the collaborative initial glaucoma treatment study comparing initial treatment randomized to medications or surgery. Ophthalmology. (2001) 108:1943–53. doi: 10.1016/s0161-6420(01)00873-9
5. Musch DC, Gillespie BW, Lichter PR, Niziol LM, Janz NK. Visual field progression in the collaborative initial glaucoma treatment study the impact of treatment and other baseline factors. Ophthalmology. (2009) 116:200–7. doi: 10.1016/j.ophtha.2008.08.051
6. Susanna R Jr., De Moraes CG, Cioffi GA, Ritch R. Why do people (Still) go blind from glaucoma? Transl Vis Sci Technol. (2015) 4:1. doi: 10.1167/tvst.4.2.1
7. Lamb TD, Collin SP, Pugh EN Jr. Evolution of the vertebrate eye: opsins, photoreceptors, retina and eye cup. Nat Rev Neurosci. (2007) 8:960–76. doi: 10.1038/nrn2283
8. Sanes JR, Masland RH. The types of retinal ganglion cells: current status and implications for neuronal classification. Annu Rev Neurosci. (2015) 38:221–46. doi: 10.1146/annurev-neuro-071714-034120
9. Lee SH, Han JW, Lee EJ, Kim TW, Kim H, Kim KW. Cognitive impairment and lamina cribrosa thickness in primary open-angle glaucoma. Transl Vis Sci Technol. (2020) 9:17. doi: 10.1167/tvst.9.7.17
10. Shang X, Zhu Z, Wang W, He M. Associations of vision impairment and eye diseases with memory decline over 4 years in china and the United States. Am J Ophthalmol. (2021) 228:16–26. doi: 10.1016/j.ajo.2021.03.021
11. Zwierko T, Jedziniak W, Lesiakowski P, Śliwiak M, Kirkiewicz M, Lubiński W. Eye-hand coordination impairment in glaucoma patients. Int J Environ Res Public Health. (2019) 16:4332. doi: 10.3390/ijerph16224332
12. Rezapour J, Nickels S, Schuster AK, Michal M, Münzel T, Wild PS, et al. Prevalence of depression and anxiety among participants with glaucoma in a population-based cohort study: the Gutenberg health study. BMC Ophthalmol. (2018) 18:157. doi: 10.1186/s12886-018-0831-1
13. Martucci A, Cesareo M, Toschi N, Garaci F, Bagetta G, Nucci C. Brain networks reorganization and functional disability in glaucoma. Prog Brain Res. (2020) 257:65–76. doi: 10.1016/bs.pbr.2020.07.007
14. Jindal V. Interconnection between brain and retinal neurodegenerations. Mol Neurobiol. (2015) 51:885–92. doi: 10.1007/s12035-014-8733-6
15. Yousaf T, Dervenoulas G, Politis M. Advances in MRI methodology. Int Rev Neurobiol. (2018) 141:31–76. doi: 10.1016/bs.irn.2018.08.008
16. Wang R, Tang Z, Sun X, Wu L, Wang J, Zhong Y, et al. White matter abnormalities and correlation with severity in normal tension glaucoma: a whole brain atlas-based diffusion tensor study. Invest Ophthalmol Vis Sci. (2018) 59:1313–22. doi: 10.1167/iovs.17-23597
17. Haykal S, Curcic-Blake B, Jansonius NM, Cornelissen FW. Fixel-based analysis of visual pathway white matter in primary open-angle glaucoma. Invest Ophthalmol Vis Sci. (2019) 60:3803–12. doi: 10.1167/iovs.19-27447
18. Sekula P, Del Greco MF, Pattaro C, Köttgen A. Mendelian randomization as an approach to assess causality using observational data. J Am Soc Nephrol. (2016) 27:3253–65. doi: 10.1681/asn.2016010098
19. Song W, Qian W, Wang W, Yu S, Lin GN. Mendelian randomization studies of brain MRI yield insights into the pathogenesis of neuropsychiatric disorders. BMC Genet. (2021) 22:342. doi: 10.1186/s12864-021-07661-8
20. Chen X, Kong J, Pan J, Huang K, Zhou W, Diao X, et al. Kidney damage causally affects the brain cortical structure: a Mendelian randomization study. EBioMedicine. (2021) 72:103592. doi: 10.1016/j.ebiom.2021.103592
21. Zhao B, Zhang J, Ibrahim JG, Luo T, Santelli RC, Li Y, et al. Large-scale gwas reveals genetic architecture of brain white matter microstructure and genetic overlap with cognitive and mental health traits (N=17,706). Mol Psychiatry. (2021) 26:3943–55. doi: 10.1038/s41380-019-0569-z
22. Jahanshad N, Kochunov PV, Sprooten E, Mandl RC, Nichols TE, Almasy L, et al. Multi-site genetic analysis of diffusion images and voxelwise heritability analysis: a pilot project of the enigma-DTI working group. Neuroimage. (2013) 81:455–69. doi: 10.1016/j.neuroimage.2013.04.061
23. Kochunov P, Jahanshad N, Sprooten E, Nichols TE, Mandl RC, Almasy L, et al. Multi-site study of additive genetic effects on fractional anisotropy of cerebral white matter: comparing meta and megaanalytical approaches for data pooling. Neuroimage. (2014) 95:136–50. doi: 10.1016/j.neuroimage.2014.03.033
24. Zhao B, Luo T, Li T, Li Y, Zhang J, Shan Y, et al. Genome-wide association analysis of 19,629 individuals identifies variants influencing regional brain volumes and refines their genetic co-architecture with cognitive and mental health traits. Nat Genet. (2019) 51:1637–44. doi: 10.1038/s41588-019-0516-6
25. Auton A, Brooks LD, Durbin RM, Garrison EP, Kang HM, Korbel JO, et al. A global reference for human genetic variation. Nature. (2015) 526:68–74. doi: 10.1038/nature15393
26. Hemani G, Tilling K, Davey Smith G. Orienting the causal relationship between imprecisely measured traits using gwas summary data. PLoS Genet. (2017) 13:e1007081. doi: 10.1371/journal.pgen.1007081
27. Verbanck M, Chen CY, Neale B, Do R. Detection of widespread horizontal pleiotropy in causal relationships inferred from Mendelian randomization between complex traits and diseases. Nat Genet. (2018) 50:693–8. doi: 10.1038/s41588-018-0099-7
28. Bowden J, Davey Smith G, Haycock PC, Burgess S. Consistent estimation in Mendelian randomization with some invalid instruments using a weighted median estimator. Genet Epidemiol. (2016) 40:304–14. doi: 10.1002/gepi.21965
29. Hernowo AT, Boucard CC, Jansonius NM, Hooymans JM, Cornelissen FW. Automated morphometry of the visual pathway in primary open-angle glaucoma. Invest Ophthalmol Vis Sci. (2011) 52:2758–66. doi: 10.1167/iovs.10-5682
30. Frezzotti P, Giorgio A, Toto F, De Leucio A, De Stefano N. Early changes of brain connectivity in primary open angle glaucoma. Hum Brain Mapp. (2016) 37:4581–96. doi: 10.1002/hbm.23330
31. Williams AL, Lackey J, Wizov SS, Chia TM, Gatla S, Moster ML, et al. Evidence for widespread structural brain changes in glaucoma: a preliminary voxel-based MRI study. Invest Ophthalmol Vis Sci. (2013) 54:5880–7. doi: 10.1167/iovs.13-11776
32. Jiang F, Zeng FF, Yu C, Ye YQ, Zeng XJ. Altered whole-brain gray matter volume in primary angle closure glaucoma patients: a voxel-based morphometry study. Neuroreport. (2018) 29:1405–12. doi: 10.1097/wnr.0000000000001126
33. Jones DK, Knösche TR, Turner R. White matter integrity, fiber count, and other fallacies: the do’s and don’ts of diffusion MRI. Neuroimage. (2013) 73:239–54. doi: 10.1016/j.neuroimage.2012.06.081
34. Hui ES, Fu QL, So KF, Wu EX. Diffusion tensor MR study of optic nerve degeneration in glaucoma. Conf Proc IEEE Eng Med Biolsoc. (2007) 2007:4312–5. doi: 10.1109/iembs.2007.4353290
35. Chang ST, Xu J, Trinkaus K, Pekmezci M, Arthur SN, Song SK, et al. Optic nerve diffusion tensor imaging parameters and their correlation with optic disc topography and disease severity in adult glaucoma patients and controls. J Glaucoma. (2014) 23:513–20. doi: 10.1097/IJG.0b013e318294861d
36. Zhang YQ, Li J, Xu L, Zhang L, Wang ZC, Yang H, et al. Anterior visual pathway assessment by magnetic resonance imaging in normal-pressure glaucoma. Acta Ophthalmol. (2012) 90:e295–302. doi: 10.1111/j.1755-3768.2011.02346.x
37. Dai H, Yin D, Hu C, Morelli JN, Hu S, Yan X, et al. Whole-brain voxel-based analysis of diffusion tensor MRI parameters in patients with primary open angle glaucoma and correlation with clinical glaucoma stage. Neuroradiology. (2013) 55:233–43. doi: 10.1007/s00234-012-1122-9
38. Zikou AK, Kitsos G, Tzarouchi LC, Astrakas L, Alexiou GA, Argyropoulou MI. Voxel-based morphometry and diffusion tensor imaging of the optic pathway in primary open-angle glaucoma: a preliminary study. AJNR Am J Neuroradiol. (2012) 33:128–34. doi: 10.3174/ajnr.A2714
39. Kantarci K. Fractional anisotropy of the fornix and hippocampal atrophy in Alzheimer’s disease. Front Aging Neurosci. (2014) 6:316. doi: 10.3389/fnagi.2014.00316
40. Kim YK, Han KM. Neural substrates for late-life depression: a selective review of structural neuroimaging studies. Prog Neuropsychopharmacol Biol Psychiatry. (2021) 104:110010. doi: 10.1016/j.pnpbp.2020.110010
41. Kurumaji A, Itasaka M, Uezato A, Takiguchi K, Jitoku D, Hobo M, et al. A Distinctive abnormality of diffusion tensor imaging parameters in the fornix of patients with bipolar Ii disorder. Psychiatry Res Neuroimaging. (2017) 266:66–72. doi: 10.1016/j.pscychresns.2017.06.005
42. Wang Y, Wang X, Zhou J, Qiu J, Yan T, Xie Y, et al. Brain morphological alterations of cerebral cortex and subcortical nuclei in high-tension glaucoma brain and its associations with intraocular pressure. Neuroradiology. (2020) 62:495–502. doi: 10.1007/s00234-019-02347-1
43. McAlonan K, Cavanaugh J, Wurtz RH. Guarding the gateway to cortex with attention in visual thalamus. Nature. (2008) 456:391–4. doi: 10.1038/nature07382
44. Yücel YH, Zhang Q, Gupta N, Kaufman PL, Weinreb RN. Loss of neurons in magnocellular and parvocellular layers of the lateral geniculate nucleus in glaucoma. Arch Ophthalmol. (2000) 118:378–84. doi: 10.1001/archopht.118.3.378
45. Jonas JB, Wang NL, Wang YX, You QS, Xie XB, Yang DY, et al. Estimated trans-lamina cribrosa pressure difference versus intraocular pressure as biomarker for open-angle glaucoma. The Beijing eye study 2011. Acta Ophthalmol. (2015) 93:e7–13. doi: 10.1111/aos.12480
46. Tamura H, Kawakami H, Kanamoto T, Kato T, Yokoyama T, Sasaki K, et al. High frequency of open-angle glaucoma in Japanese patients with Alzheimer’s disease. J Neurol Sci. (2006) 246:79–83. doi: 10.1016/j.jns.2006.02.009
47. Bayer AU, Ferrari F, Erb C. High occurrence rate of glaucoma among patients with Alzheimer’s disease. Eur Neurol. (2002) 47:165–8. doi: 10.1159/000047976
48. Davis BM, Crawley L, Pahlitzsch M, Javaid F, Cordeiro MF. Glaucoma: the retina and beyond. Acta Neuropathol. (2016) 132:807–26. doi: 10.1007/s00401-016-1609-2
Keywords: diffusion tensor imaging, white matter, region-of-interest, glaucoma, Mendelian randomization
Citation: Liu K, Wu P, Chen B, Cai Y, Yuan R and Zou J (2022) Implicating Causal Brain Magnetic Resonance Imaging in Glaucoma Using Mendelian Randomization. Front. Med. 9:956339. doi: 10.3389/fmed.2022.956339
Received: 30 May 2022; Accepted: 13 June 2022;
Published: 01 July 2022.
Edited by:
Alessio Martucci, University of Rome Tor Vergata, ItalyReviewed by:
Valeria Albano, Azienda Ospedaliero Universitaria Consorziale Policlinico di Bari, ItalyCopyright © 2022 Liu, Wu, Chen, Cai, Yuan and Zou. This is an open-access article distributed under the terms of the Creative Commons Attribution License (CC BY). The use, distribution or reproduction in other forums is permitted, provided the original author(s) and the copyright owner(s) are credited and that the original publication in this journal is cited, in accordance with accepted academic practice. No use, distribution or reproduction is permitted which does not comply with these terms.
*Correspondence: Jing Zou, anpvdTMwQGNzdS5lZHUuY24=
Disclaimer: All claims expressed in this article are solely those of the authors and do not necessarily represent those of their affiliated organizations, or those of the publisher, the editors and the reviewers. Any product that may be evaluated in this article or claim that may be made by its manufacturer is not guaranteed or endorsed by the publisher.
Research integrity at Frontiers
Learn more about the work of our research integrity team to safeguard the quality of each article we publish.