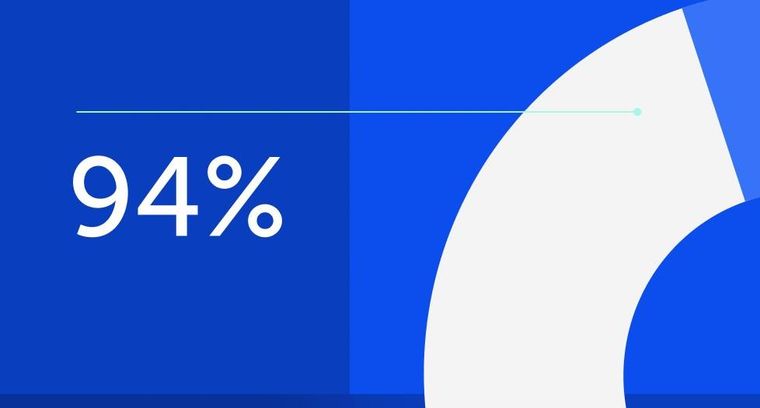
94% of researchers rate our articles as excellent or good
Learn more about the work of our research integrity team to safeguard the quality of each article we publish.
Find out more
ORIGINAL RESEARCH article
Front. Med., 20 October 2022
Sec. Nephrology
Volume 9 - 2022 | https://doi.org/10.3389/fmed.2022.953643
This article is part of the Research TopicGenetics And Epigenetics of Chronic Kidney DiseaseView all 17 articles
X-linked Alport syndrome (AS) caused by hemizygous disease-causing variants in COL4A5 primarily affects males. Females with a heterozygous state show a diverse phenotypic spectrum ranging from microscopic hematuria to end-stage kidney disease (ESKD) and extrarenal manifestations. In other X-linked diseases, skewed X-inactivation leads to preferential silencing of one X-chromosome and thus can determine the phenotype in females. We aimed to show a correlation between X-inactivation in blood and urine-derived renal cells and clinical phenotype of females with a heterozygous disease-causing variant in COL4A5 compared to healthy controls. A total of 56 females with a heterozygous disease-causing COL4A5 variant and a mean age of 31.6 ± 18.3 SD years were included in this study. A total of 94% had hematuria, 62% proteinuria >200 mg/day, yet only 7% had decreased eGFR. Using human androgen receptor assay X-inactivation was examined in blood cells of all 56 individuals, in urine-derived cells of 27 of these individuals and in all healthy controls. X-inactivation did not correlate with age of first manifestation, proteinuria or eGFR neither in blood, nor in urine. The degree of X-inactivation showed a moderate association with age, especially in urine-derived cells of the patient cohort (rho = 0.403, p = 0.037). Determination of X-inactivation allelity revealed a shift of X-inactivation toward the COL4A5 variant bearing allele. This is the first study examining X-inactivation of urine-derived cells from female individuals with AS. A correlation between phenotype and X-inactivation could not be observed suspecting other genetic modifiers shaping the phenotype in female individuals with AS. The association of X-inactivation with age in urine-derived cells suggests an escape-mechanism inactivating the COL4A5 variant carrying allele in female individuals with AS.
Alport syndrome (AS) is a hereditary nephropathy characterized by (microscopic) hematuria, proteinuria, chronic kidney disease potentially progressing to end-stage kidney disease (ESKD), hearing loss, and typical ocular changes (1, 2). The syndrome is caused by disease-causing [(likely) pathogenic] variants in genes encoding collagen type IV leading to an altered glomerular basement membrane (GBM). AS can be inherited in an X-linked form due to disease-causing variants in COL4A5 or by disease-causing variants in COL4A3 or COL4A4 comparable with an autosomal inheritance (3–9).
Males with X-linked AS show a distinct genotype-phenotype correlation, illustrated by more severe symptoms in individuals with loss-of-function variants and less severe symptoms in individuals with missense variants or in-frame deletions. Additionally, variants located at the 5′ end have been described to present with earlier onset of ESKD and higher risk of extrarenal manifestations (10, 11).
Female individuals with heterozygous disease-causing variants in COL4A5 have traditionally been described as healthy carriers even though the penetrance is variable both intrafamilial and interfamilial, showing a broad spectrum of clinical symptoms, varying from mild isolated microscopic hematuria to severe AS (12–15). By age 60 years, 15–30% of these female individuals develop ESKD. Furthermore, extrarenal manifestation like eye abnormalities and hearing impairment have been described and can affect up to 30% of female individuals (13). Until now, the cause for the phenotypic variability in female individuals with a heterozygous disease-causing variant in COL4A5 is unclear. In contrast to male individuals, genotype-phenotype correlation is less-well described in females so far but severe variants (e.g., loss-of-function variants) appear to result in a more severe phenotype–similarly to affected males (13, 16).
Interindividual variability in females with a heterozygous disease-causing COL4A5 variant might be explained by skewed, i.e., preferential X-inactivation of one X chromosome, as already discussed in the literature (12, 17, 18). X-inactivation results in transcriptional silencing of one X chromosome in females to attain gene dosage parity between XX female and XY male mammals (19). Either the maternal or the paternal X chromosome is randomly silenced through a complex cellular process resulting in a female being a mosaic of cells with either an active maternal or paternal X chromosome (17). X-inactivation has long been thought to be an irreversible occurrence. In recent years, there is increasing evidence, that in cells with rapid turnover, such as peripheral blood cells and buccal cells, skewing of X-inactivation increases with older age (20–23). However, an age-dependent mechanism in other tissues and their possible implications have not been shown yet.
So far, only insufficient molecular genetic investigations can be found focusing on X-inactivation in AS. Vetrie et al. tried to detect a correlation between the X-inactivation allelity of female carriers and the severity of their disease, but in DNA derived from peripheral blood lymphocytes no correlation was found (17). In contrast, Rheault et al. could show that X-inactivation is a major modifier of the female carrier phenotype in murine X-linked AS (24).
In this study, the relationship of X-inactivation of female individuals with a heterozygous disease-causing COL4A5 variant with phenotypic characteristics in blood as well as urine-derived cells consisting of podocytes and tubular cells was investigated. Furthermore, these X-inactivation data were compared with those from healthy controls.
Inclusion criteria of the study were proof of a heterozygous disease-causing COL4A5 variant and biologically female gender. Individuals of all ages were allowed to be included in the study (age range of included individuals: 5–79 years). There were no comorbidities observed in the included individuals. Exclusion criteria were missing written informed consent and absence of above-mentioned inclusion criteria. Recruitment was primarily achieved by acquiring relatives of known male AS individuals with disease-causing COL4A5 variants. Altogether 60 female individuals were recruited fulfilling the inclusion criteria. Four individuals had to be excluded due to indistinguishable X-inactivation analysis (see subsection X-inactivation analysis), leading to 56 available individuals from 43 unrelated families. In 49 of those individuals, a blood sample from an AS-affected relative was available needed for determining the potential skewing.
A total of 43/56 patients were recruited as part of the NephroGen cohort of the Institute of Human Genetics at the Klinikum rechts der Isar of the Technical University of Munich, and 2/56 at the Department of Pediatrics I of the University Children’s Hospital in Heidelberg. The remaining 11 patients were recruited at the Department of Pediatric Nephrology of the University Hospital in Essen, Germany. The study was approved by the respective local ethics committee of each contributing center and performed in accordance to the standards of the 2013 Helsinki Declaration. All individuals or their legal guardians gave written and informed consent.
Phenotypic information was gathered by studying medical records and interviews. Age of first manifestation was considered as time point of first proof of an abnormal renal phenotype (either microscopic hematuria, macroscopic hematuria or proteinuria >200 mg/day) or diagnosis of an extrarenal manifestation. When 24-h urine collection was not available, urinary protein/creatinine ratio of more than 200 mg/g creatinine was considered as proteinuria. Semi-quantitative urine dipstick measurements with the result “negative” were considered as proteinuria less than 200 mg/day. Serum creatinine values were determined by the treating physician. eGFR was calculated for individuals >14 years with CKD-EPI formula (25), for individuals ≤14 years with the “Bedside Schwartz formula” (26) considering the height and serum creatinine of the individual.
Healthy female control individuals without history of AS in their families were recruited and blood was drawn as well as spontaneous urine collected. Out of 40 healthy control individuals, five were homozygous for the AR locus and therefore not useable for the control cohort of this study. X-inactivation data of the remaining 35 female healthy controls were available for the study.
Supplementary Table 1 displays COL4A5 variants and the clinical phenotype of the affected individuals. Variants were adjusted to the RefSeq Sequence NM_033380.3. Frameshift, non-sense and canonical splice site variants as well as a large deletion (70 kb, exons 38–51) were considered loss-of-function variants. For statistical calculation, two variants with small in-frame deletions were grouped with missense variants (c.2048_2050delCTG, p.Pro683_Gly684delinsArg, and c.1751_1756delCAGGGC, p.Pro584_Gly585del). Additionally, they were counted as glycine-affecting variants.
The severity of variants was calculated based on a classification of variants according to their position in the transcript. Variants were not located in the signal peptide and NC2 domain. Variants were classified according to their domain and the proximity to the 5′-end. Variants in the 5′-end near collagenous domain (c.124-c.2246) were considered “severe,” variants in the 3′-end near collagenous domain (c.2247-c.4386) were considered “moderate” and variants in the NC1 domain (c.4399-c.5073; 3′-end part of the protein) were considered “mild” (Supplementary Table 2) (10).
DNA was automatically extracted from EDTA blood samples with “Chemagic DNA Blood 5 k Kit” using a Chemagen 360 (PerkinElmer, Waltham, MA, USA) according to the manufacturer’s instructions.
Kidney tissue from biopsy samples is the gold standard to obtain renal-derived cells but was seldom available for analysis. Therefore, we compared X-inactivation in tissue-derived and urine-derived cells from individuals without renal disease and achieved comparable (less than 20% difference) results in 75% of individuals.
In addition, urine-derived cells were analyzed in five individuals with AS of the study cohort regarding the presence of podocytes and tubular cells. Therefore, podocytes and tubular cells were isolated from urine samples with magnetic cell separation using the “CD10 Microbead Kit human” (Miltenyi Biotec B.V. & Co.KG, Bergisch Gladbach, Germany). Subsequent FACS analysis confirmed the enrichment of CD10 positive cells. DNA was then extracted from these cells with Qiagen’s “DNeasy Blood and Tissue Kit 250” (Qiagen GmbH, Hilden, Germany).
The expression of CD10 in podocytes and tubular cells has been demonstrated in immunohistochemical stainings (Supplementary Figure 1). In 80% of analyses both cell types were detected within the urinary sediment. For these analyses first morning urine was collected and urine samples were immediately centrifuged for 10 min at 14,000 rpm at 4°C. Afterward the supernatant was discarded and urine cells were isolated right away or immediately frozen and stored at −80°C (for DNA isolation).
The X-inactivation status was measured by assessing the DNA methylation based on the difference between the number of trinucleotide repeats at the human androgen receptor locus (AR, Xq11-12; HUMARA-Assay) (21).
Due to the correlation of X-inactivation with hypermethylation, digestion with methylation sensitive restriction enzymes (Hpall) can differentiate between active and inactive alleles. A male sample was enclosed in each assay in order to control sufficient Hpall digestion. Subsequent to digestion and amplification of the samples with fluorescence-tagged PCR primers, fragment length analysis of the PCR products was run on an ABI 3130XL genetic analyzer and the GeneMarker software (Softgenetics, PA, USA). If amplified DNA fragments exhibited the same size on both alleles, X-inactivation could not be determined.
X-inactivation data was generated and is displayed in two different ways depending on the availability of an affected male relative. In a first step, skewness of X-inactivation was measured with the ratio of inactivation between the two alleles without knowledge which of the alleles carried the COL4A5 variant (e.g., 80:20 or 72:28). These data are referred to as “X-inactivation without allelity” and are displayed in a range from theoretically 50% (not skewed, both alleles with same X-inactivation percentage) to 100% (completely skewed).
In families with an available affected male, segregation analysis of the male individual (0% X-inactivation, COL4A5 variant bearing allele not inactivated at all) allowed determining which of the two alleles in a female individual with a heterozygous disease-causing variant was the one bearing the COL4A5 variant (27). Thus X-inactivation data could be displayed in a range from theoretically 0% (COL4A5 variant bearing allele not inactivated at all) to 100% (COL4A5 variant bearing allele completely inactivated). This dataset is referred to as “X-inactivation with allelity.”
Parametric data are presented as mean ± standard deviation and non-parametric data as median [Interquartile range, IQR]. For correlation analyses Spearman’s rho was calculated (Figures 2, 3 and Table 1). When comparing two groups (Table 2 and Supplementary Table 3) we used Mann–Whitney U-test for non-parametric variables, Student’s t-test for parametric variables and Chi square test for categorical variables. For comparison of more than 2 groups Kruskal–Wallis test was applied due to the non-normal distribution of the dependent parameters (Supplementary Table 2). SPSS® Statistics, version 26 (IBM, Armonk, NY, USA), was used for all statistical tests.
Table 1. Correlation coefficients of X-inactivation in blood and urine with phenotypic markers in female individuals with a heterozygous variant in COL4A5.
In total, 56 resp. 27 female individuals with a disease-causing variant in COL4A5 with X-inactivation data in peripheral blood cells resp. in urine-derived cells were available for analysis (Figure 1). In 49 of the 56 blood X-inactivation datasets, the COL4A5-dependent allelity of X-inactivation was revealed by analyzing affected male relatives. For the urine-derived samples, COL4A5-dependent X-inactivation allelity could be determined in 26 out of 27 samples (Figure 1).
Figure 1. Flowchart illustrating the recruitment process of participants. Figure shows recruitment procedure for individuals with a heterozygous disease-causing COL4A5 variant (A) and healthy controls (B).
The mean age of the included individuals was 31.6 ± 18.3 years (mean ± SD; Table 3). Almost every female individual with a heterozygous disease-causing COL4A5 variant had a renal manifestation (94.6%) with first symptoms/abnormal urine analysis detected at a median age of 9.5 [IQR 5.3; 19.7] years. Most common manifestation was hematuria (93.7%; microscopic or macroscopic) followed by proteinuria >200 mg/day (62.3%), whereas mean eGFR was normal. Four of the 56 individuals progressed to ESKD with a mean age of onset at 32.8 ± 5.3 years (mean ± SD). Extrarenal manifestation defined as hearing impairment (5.4%) or ocular manifestation (7.1%) were detected only in a few individuals.
Loss-of-function variants were present in 42.9% of cases, mostly represented by frameshift and canonical splice site variants (Table 3). Individuals with missense variants had primarily glycine-variants except for 3 out of 32 individuals. Most variants were located in the collagenous domain of the COL4A5 gene (87.5%).
Comparing the total cohort of 56 individuals with the 27 individuals with additional urine samples, the subcohort of individuals with X-inactivation data from urine-derived cells were slightly older, had less proteinuria, slightly decreased eGFR and did not include individuals with ESKD due to loss of remaining urine excretion or history of kidney transplantation (Table 3).
Healthy controls (n = 35) had no history of AS in the family, were all female and had a mean age of 37.1 ± 19.1 years (mean ± SD).
Median age of first manifestation was significantly lower in individuals with loss-of-function variants compared to individuals with missense variants (Table 2) (6.6 vs. 12.4 years, p = 0.011). Individuals with ocular manifestations or hearing impairment predominately carried loss-of-function variants.
After classification of variants according to their expected severity (10), most variants were located in the collagenous domain, which can be divided in the more severe 5′-end near part and the less severe 3′-end near part (Supplementary Table 2). Individuals with variants near the 5′-end showed earlier first manifestation, higher percentage of proteinuria >200 mg/day, ocular manifestation and ESKD, yet without significance. The variants of the four individuals who progressed to ESKD were three missense and one splice site variant.
Median X-inactivation of cases without considering allelity was 65% [IQR 58; 72] in blood cells and 59% [IQR 53; 69] in urine-derived cells (data not shown). Analysis of COL4A5-dependent X-inactivation of blood and urine samples showed a tendency toward inactivation of the variant bearing allele (55% and 56%). For the controls, the degree of X-inactivation amounted to median 65% [IQR 59; 79] for blood cells and 66% [IQR 59; 70] for urine-derived cells.
X-inactivation measured without allelity showed a medium to strong association between blood and urine-derived cells in affected female individuals and healthy controls (rho = 0.348, p = 0.075 and rho = 0.551, p = 0.001; Figures 2A,B), however the association was not significant in affected individuals. Regarding the COL4A5-dependent X-inactivation allelity, the association between blood and urine-derived cells was even higher (rho = 0.591, p = 0.001; Figure 2C).
Figure 2. Correlation between X-inactivation in blood and urine cells. Scatter-dot plots of the relationship of X-inactivation between blood and urine cells for individuals with a heterozygous disease-causing COL4A5 variant (A,C) and healthy controls (B). Spearman’s rho was calculated and is displayed in the boxes. Linear regression lines were plotted for illustration purposes.
Correlating age with X-inactivation (without allelity) in urine-derived cells of the affected individuals, a significant association with a Spearman’s rho of 0.403 (p = 0.037, Figure 3A) was seen. Considering X-inactivation with COL4A5-dependent allelity, the association with age was similar (rho = 0.348, Supplementary Figure 2A), however not significant. In comparison, X-inactivation in blood cells of affected individuals showed weak, non-significant correlations with age, with or without considering the COL4A5-dependent allelity (Figure 3B and Supplementary Figure 2B).
Figure 3. Correlation between age of individuals and X-inactivation in blood and urine cells. Scatter-dot plots of the association of participant age and X-inactivation in blood and urine cells for individuals with a heterozygous disease-causing COL4A5 variant (A,B) and healthy controls (C,D). Spearman’s rho was calculated and is displayed in the boxes. Linear regression lines were plotted for illustration purposes.
Comparing the individuals to healthy controls, the latter showed only a weak association of X-inactivation with age for both, blood and urinary cells (Figures 3C,D; rho = 0.237; p = 0.171, and rho = 0.281; p = 0.101; Supplementary Figure 3).
Correlation analyses of phenotypic characteristics and X-inactivation could not demonstrate a significant association for age of first manifestation, eGFR or proteinuria (Table 1). There was a trend toward decreased eGFR with enhanced X-inactivation, however eGFR and age naturally showed a strong dependency in our cohort (rho = −0.547, p < 0.001; data not shown).
Further analysis of blood of individuals with ESKD (n = 4) revealed increased X-inactivation without allelity (77% in ESKD vs. 64% in non-ESKD) as well as increased X-inactivation with COL4A5-dependent allelity (72% in ESKD vs. 55% in non-ESKD, Supplementary Table 3).
Individuals with ocular manifestation or hearing impairment showed increased X-inactivation in urine-derived cells. X-inactivation with and without allelity was 71% in individuals with ocular manifestations vs. 53–58% in individuals without ocular manifestations. Regarding hearing impairment, the results were similar (69–73% in individuals affected by hearing impairment vs. 54–58% without hearing impairment; Supplementary Table 3). However, blood cells did not show differences in X-inactivation when comparing individuals with and without extrarenal manifestations. Of the 5 individuals presenting with extrarenal manifestations, everyone had normal eGFR, but four individuals exhibited proteinuria >200 mg/day with one individual presenting with proteinuria of 1.42 g/day. However, statistical analysis was not possible due to the small number of individuals with ESKD or extrarenal manifestations.
This is the first study investigating X-inactivation in urine-derived cells of female individuals with X-linked AS. Additionally, the COL4A5-dependent allelity of X-inactivation depending on the COL4A5 variant bearing allele was included in the analysis as a novelty in a human AS cohort.
Unlike the hypothesis, X-inactivation was not relevantly associated with phenotypic characteristics like age of first manifestation, proteinuria or decreased eGFR. However, we were able to demonstrate a statistically significant correlation of age with skewed X-inactivation in urine-derived cells of female individuals with AS. This correlation was also found in peripheral blood cells of the individuals as well as in urine-derived and blood cells of the healthy controls, yet only in a weak, not statistically significant fashion.
The variability of X-inactivation and its association with age has been proven in cross-sectional and recently in longitudinal studies enrolling healthy controls (22, 28, 29). The mechanisms for this association are unclear, but intrinsic as well as environmental influences have been suggested (28). So far, correlation of X-inactivation with age has only been demonstrated for rapidly proliferating tissues like blood granulocytes or buccal epithelium (21, 23). A recent study including over 300 healthy females could not show an age-dependent effect in slowly proliferating cells like fat or skin tissue, but it did show an effect in lymphocyte-derived cells (22). The only study investigating cells isolated from urine of approximately 40 healthy controls was not able to show an age-dependent effect of X-inactivation (21). Our results in healthy controls show only a weak, non-significant correlation of age with X-inactivation in urine-derived cells, which underlines the observation for slowly proliferating cells in the existing literature.
In contrast, urine-derived cells from our individuals with AS exhibited a moderate and statistically significant correlation of age with X-inactivation. Older individuals with AS were also more likely to present with increased X-inactivation of the variant allele (rho = 0.348, p = 0.076) and the degree of X-inactivation of the disease-causing allele amounted to 55% in blood cells and 56% in urine-derived cells. This suggests that X-inactivation could serve as an escape-mechanism protecting individuals from the development of severe complications of AS as they get older. Involvement of X-inactivation has been described for heterozygous carriers in X-linked diseases like severe combined immunodeficiency (30) and X-linked intellectual disability (31). In these individuals the allelity of X-inactivation also led to a more inactive disease variant bearing allele in heterozygous carriers. Thus, the gene dosage is adjusted in favor of the wild-type allele by silencing the mutant allele. The proposed mechanism for this phenomenon is a survival selection of cells which have inactive disease-causing alleles. Even though the data might suggest that this is also the case in individuals with a heterozygous disease-causing variant in COL4A5, the size of the present study cohort is not sufficient to definitely draw this conclusion. Further studies including more individuals with a severe phenotype would be needed to elucidate these mechanisms.
Regarding the relationship between the tissues, the data showed a moderate to high and significant correlation between X-inactivation in blood and urine-derived cells (rho = 0.551, p = 0.001 for controls; and rho = 0.591, p = 0.001 for cases considering COL4A5-dependent X-inactivation allelity). These associations were stronger than described in the literature for cells from other slowly proliferating tissues (like fat and skin) (22). As blood cells are known to have the highest degrees of X-inactivation skewness in healthy subjects compared to other tissues (22, 23), urine cells should be considered when analyzing X-inactivation in certain cases.
Nonetheless, the hypothesized X-inactivation-phenotype relationship could not be shown in blood or urine-derived cells. This is in line with the previous observation in 30 female individuals with a heterozygous disease-causing variant in COL4A5 in blood cells only (17). Murine data, however, point to a role for X-inactivation in phenotype development in an AS mouse model (24). X-inactivation of the mutant allele was correlated with less proteinuria and plasma urea nitrogen, demonstrated in cells from whole murine kidneys. Yet one has to keep in mind that X-inactivation is measured differently in mice, and the effect of age, which evidently plays an important role in human X-inactivation, is not accounted for. Additionally, a standardized inbred murine model cannot be compared with humans, especially as more evidence emerges demonstrating a role for other genetic modifiers influencing the phenotype like FMN1, which has been described to be associated with albuminuria in COL4A5-deficient mice (32). In general, the genotype-phenotype correlation in female individuals with a heterozygous disease-causing COL4A5 variant is weak compared to descriptions in male individuals with AS (16). A slight, but significant difference in age of first manifestation between loss-of-function and missense variants could be seen, however, no difference regarding renal parameters relevant for prognosis were found (proteinuria, eGFR). As X-inactivation does not seem to play a big role in female individuals with AS apart from age, other genetic modifiers like disease-causing variants or single nucleotide polymorphisms in other genes like autosomal COL4A3, COL4A4 or recently described FMN1 become increasingly important and future studies should be dedicated to these (32).
One limitation of the present study is the relatively young age of included individuals (31.6 years), which also led to decreased numbers of individuals with ESKD and extrarenal manifestations. This bias is based on the recruitment process, which mostly included sisters or mothers of pediatric patients. The degree of skewness in blood cells evidently accelerates above the age of 60 years (22). Thus, associations of X-inactivation with phenotypic characteristics could have been detected with an older study population. Additionally, our study population primarily included individuals of Caucasian descent and therefore the results might not be applicable for all ethnicities. Another limitation of the study applies to the method of X-inactivation measurement. Indistinguishable PCR fragments due to same-sized trinucleotide repeats can make an analysis impossible as it was the case in nine participants of this study. Moreover, collection of urine-derived cells in individuals with ESKD is difficult due to loss of urine production or kidney transplantation, which often excludes severely affected individuals from X-inactivation analysis of urine-derived cells. Renal cell isolation and transportation (dry ice) is also more complex than drawing EDTA-blood resulting in a markedly reduced number of urine X-inactivation data.
In conclusion, our data show an association of X-inactivation and age in urine-derived cells of female individuals with AS, which could present an escape-mechanism to avoid expression of the COL4A5 variant as individuals get older. A relationship between X-inactivation and phenotypic characteristics was not present in blood as well as urine cells. Future studies about X-inactivation in female individuals should focus on older cohorts.
The datasets presented in this study can be found in online repositories. The names of the repository/repositories and accession number(s) can be found in the article/Supplementary material.
This study was approved by the respective local ethics committee of each contributing center. Written informed consent to participate in this study was provided by the participants’ legal guardian/next of kin. Written informed consent was obtained from the individual(s), and minor(s)’ legal guardian/next of kin, for the publication of any potentially identifiable images or data included in this article.
RG, LK, SJ, BL-D, CH, KR, JC, and KB performed the analysis. RS, MB, BT, VT, NA-E, VN-S, NG, AM, LP, MK, OG, AL, SW, LR, UH, TM, and AB cared for the patients and acquired and provided the clinical data. RG, AB, and JH were responsible for writing and revision of the manuscript. All authors contributed to the article and approved the submitted version.
This study was supported by the German Pediatric Nephrology Association and the Technical University of Munich (TUM) in the framework of the Open Access Publishing Program.
We thank the individuals and their families for participation in this study. Especially, we also thank “Alport Selbsthilfegruppe e.V.,” a non-profit organization consisting of many Alport syndrome patients.
The authors declare that the research was conducted in the absence of any commercial or financial relationships that could be construed as a potential conflict of interest.
All claims expressed in this article are solely those of the authors and do not necessarily represent those of their affiliated organizations, or those of the publisher, the editors and the reviewers. Any product that may be evaluated in this article, or claim that may be made by its manufacturer, is not guaranteed or endorsed by the publisher.
The Supplementary Material for this article can be found online at: https://www.frontiersin.org/articles/10.3389/fmed.2022.953643/full#supplementary-material
1. Gross O, Netzer KO, Lambrecht R, Seibold S, Weber M. Meta-analysis of genotype-phenotype correlation in X-linked Alport syndrome: impact on clinical counselling. Nephrol Dial Transplant. (2002) 17:1218–27. doi: 10.1093/ndt/17.7.1218
2. Williamson DA. Alport’s syndrome of hereditary nephritis with deafness. Lancet. (1961) 2:1321–3. doi: 10.1016/S0140-6736(61)90899-6
3. Weber S, Strasser K, Rath S, Kittke A, Beicht S, Alberer M, et al. Identification of 47 novel mutations in patients with Alport syndrome and thin basement membrane nephropathy. Pediatr Nephrol. (2016) 31:941–55. doi: 10.1007/s00467-015-3302-4
4. Funk SD, Lin MH, Miner JH. Alport syndrome and Pierson syndrome: diseases of the glomerular basement membrane. Matrix Biol. (2018) 7:250–61. doi: 10.1016/j.matbio.2018.04.008
5. Abou Tayoun AN, Pesaran T, DiStefano MT, Oza A, Rehm HL, Biesecker LG, et al. Recommendations for interpreting the loss of function PVS1 ACMG/AMP variant criterion. Hum Mutat. (2018) 39:1517–24. doi: 10.1002/humu.23626
6. Kearney HM, Thorland EC, Brown KK, Quintero-Rivera F, South ST, Working Group of the American College of Medical Genetics Laboratory Quality Assurance Committee. American college of medical genetics standards and guidelines for interpretation and reporting of postnatal constitutional copy number variants. Genet Med. (2011) 13:680–5. doi: 10.1097/GIM.0b013e3182217a3a
7. Richards S, Aziz N, Bale S, Bick D, Das S, Gastier-Foster J, et al. Standards and guidelines for the interpretation of sequence variants: a joint consensus recommendation of the American college of medical genetics and genomics and the association for molecular pathology. Genet Med. (2015) 17:405–24. doi: 10.1038/gim.2015.30
8. Ellard S, Baple EL, Berry I, Forrester N, Turnbull C, Owens M, et al. ACGS Best Practice Guidelines for Variant Classification 2019. (2019). Available online at: https://www.acgs.uk.com/media/11631/uk-practice-guidelines-for-variant-classification-v4-01-2020.pdf (accessed May 25, 2022).
9. Gibson J, Fieldhouse R, Chan MMY, Sadeghi-Alavijeh O, Burnett L, Izzi V, et al. Prevalence estimates of predicted pathogenic COL4A3-COL4A5 variants in a population sequencing database and their implications for Alport syndrome. J Am Soc Nephrol. (2021) 32:2273–90. doi: 10.1681/ASN.2020071065
10. Bekheirnia MR, Reed B, Gregory MC, McFann K, Shamshirsaz AA, Masoumi A, et al. Genotype-phenotype correlation in X-linked Alport syndrome. J Am Soc Nephrol. (2010) 21:876–83. doi: 10.1681/ASN.2009070784
11. Jais JP, Knebelmann B, Giatras I, Marchi M, Rizzoni G, Renieri A, et al. X-linked Alport syndrome: natural history in 195 families and genotype- phenotype correlations in males. J Am Soc Nephrol. (2000) 11:649–57. doi: 10.1681/ASN.V114649
12. Rheault MN. Women and Alport syndrome. Pediatr Nephrol. (2012) 27:41–6. doi: 10.1007/s00467-011-1836-7
13. Savige J, Colville D, Rheault M, Gear S, Lennon R, Lagas S, et al. Alport syndrome in women and girls. Clin J Am Soc Nephrol. (2016) 11:1713–20. doi: 10.2215/CJN.00580116
14. Yamamura T, Nozu K, Fu XJ, Nozu Y, Ye MJ, Shono A, et al. Natural history and genotype-phenotype correlation in female X-linked Alport syndrome. Kidney Int Rep. (2017) 2:850–5. doi: 10.1016/j.ekir.2017.04.011
15. Kashtan CE, Ding J, Garosi G, Heidet L, Massella L, Nakanishi K, et al. Alport syndrome: a unified classification of genetic disorders of collagen IV alpha345: a position paper of the Alport syndrome classification working group. Kidney Int. (2018) 93:1045–51. doi: 10.1016/j.kint.2017.12.018
16. Jais JP, Knebelmann B, Giatras I, De Marchi M, Rizzoni G, Renieri A, et al. X-linked Alport syndrome: natural history and genotype-phenotype correlations in girls and women belonging to 195 families: a “European community Alport syndrome concerted action” study. J Am Soc Nephrol. (2003) 14:2603–10. doi: 10.1097/01.ASN.0000090034.71205.74
17. Vetrie D, Flinter F, Bobrow M, Harris A. X inactivation patterns in females with Alport’s syndrome: a means of selecting against a deleterious gene? J Med Genet. (1992) 29:663–6. doi: 10.1136/jmg.29.9.663
18. Shimizu Y, Nagata M, Usui J, Hirayama K, Yoh K, Yamagata K, et al. Tissue-specific distribution of an alternatively spliced COL4A5 isoform and non-random X chromosome inactivation reflect phenotypic variation in heterozygous X-linked Alport syndrome. Nephrol Dial Transplant. (2006) 21:1582–7. doi: 10.1093/ndt/gfl051
19. Deakin JE, Chaumeil J, Hore TA, Marshall Graves JA. Unravelling the evolutionary origins of X chromosome inactivation in mammals: insights from marsupials and monotremes. Chromosome. (2009) 17:671–85. doi: 10.1007/s10577-009-9058-6
20. Bolduc V, Chagnon P, Provost S, Dubé MP, Belisle C, Gingras M, et al. No evidence that skewing of X chromosome inactivation patterns is transmitted to offspring in humans. J Clin Invest. (2008) 118:333–41. doi: 10.1172/JCI33166
21. Sharp A, Robinson D, Jacobs P. Age- and tissue-specific variation of X chromosome inactivation ratios in normal women. Hum Genet. (2000) 107:343–9. doi: 10.1007/s004390000382
22. Zito A, Davies MN, Tsai PC, Roberts S, Andres-Ejarque R, Nardone S, et al. Heritability of skewed X-inactivation in female twins is tissue-specific and associated with age. Nat Commun. (2019) 10:5339. doi: 10.1038/s41467-019-13340-w
23. Knudsen GP, Pedersen J, Klingenberg O, Lygren I, Orstavik KH. Increased skewing of X chromosome inactivation with age in both blood and buccal cells. Cytogenet Genome Res. (2007) 116:24–8. doi: 10.1159/000097414
24. Rheault MN, Kren SM, Hartich LA, Wall M, Thomas W, Mesa HA, et al. X-inactivation modifies disease severity in female carriers of murine X-linked Alport syndrome. Nephrol Dial Transplant. (2010) 25:764–9. doi: 10.1093/ndt/gfp551
25. Levey AS, Stevens LA, Schmid CH, Zhang YL, Castro AF III, Feldman HI, et al. A new equation to estimate glomerular filtration rate. Ann Intern Med. (2009) 150:604–12. doi: 10.7326/0003-4819-150-9-200905050-00006
26. Schwartz GJ, Muñoz A, Schneider MF, Mak RH, Kaskel F, Warady BA, et al. New equations to estimate GFR in children with CKD. J Am Soc Nephrol. (2009) 20:629–37. doi: 10.1681/ASN.2008030287
27. Archer H, Evans J, Leonard H, Colvin L, Ravine D, Christodoulou J, et al. Correlation between clinical severity in patients with Rett syndrome with a p.R168X or p.T158M MECP2 mutation, and the direction and degree of skewing of X-chromosome inactivation. J Med Genet. (2007) 44:148–52. doi: 10.1136/jmg.2006.045260
28. Mengel-From J, Lindahl-Jacobsen R, Nygaard M, Soerensen M, Ørstavik KH, Hertz JM, et al. Skewness of X-chromosome inactivation increases with age and varies across birth cohorts in elderly Danish women. Sci Rep. (2021) 11:4326. doi: 10.1038/s41598-021-83702-2
29. Hatakeyama C, Anderson CL, Beever CL, Penaherrera MS, Brown CJ, Robinson WP. The dynamics of X-inactivation skewing as women age. Clin Genet. (2004) 66:327–32. doi: 10.1111/j.1399-0004.2004.00310.x
30. Puck JM, Nussbaum RL, Conley ME. Carrier detection in X-linked severe combined immunodeficiency based on patterns of X chromosome inactivation. J Clin Invest. (1987) 79:1395–400. doi: 10.1172/JCI112967
31. Plenge RM, Stevenson RA, Lubs HA, Schwartz CE, Willard HF. Skewed X-chromosome inactivation is a common feature of X-linked mental retardation disorders. Am J Hum Genet. (2002) 71:168–73. doi: 10.1086/341123
Keywords: Alport syndrome, X-inactivation, COL4A5, urine-derived cells, microscopic hematuria, proteinuria, end-stage kidney disease
Citation: Günthner R, Knipping L, Jeruschke S, Satanoskij R, Lorenz-Depiereux B, Hemmer C, Braunisch MC, Riedhammer KM, Ćomić J, Tönshoff B, Tasic V, Abazi-Emini N, Nushi-Stavileci V, Buiting K, Gjorgjievski N, Momirovska A, Patzer L, Kirschstein M, Gross O, Lungu A, Weber S, Renders L, Heemann U, Meitinger T, Büscher AK and Hoefele J (2022) Renal X-inactivation in female individuals with X-linked Alport syndrome primarily determined by age. Front. Med. 9:953643. doi: 10.3389/fmed.2022.953643
Received: 26 May 2022; Accepted: 21 September 2022;
Published: 20 October 2022.
Edited by:
Chunlin Gao, Nanjing General Hospital of Nanjing Military Command, ChinaReviewed by:
Moshe Levi, Georgetown University, United StatesCopyright © 2022 Günthner, Knipping, Jeruschke, Satanoskij, Lorenz-Depiereux, Hemmer, Braunisch, Riedhammer, Ćomić, Tönshoff, Tasic, Abazi-Emini, Nushi-Stavileci, Buiting, Gjorgjievski, Momirovska, Patzer, Kirschstein, Gross, Lungu, Weber, Renders, Heemann, Meitinger, Büscher and Hoefele. This is an open-access article distributed under the terms of the Creative Commons Attribution License (CC BY). The use, distribution or reproduction in other forums is permitted, provided the original author(s) and the copyright owner(s) are credited and that the original publication in this journal is cited, in accordance with accepted academic practice. No use, distribution or reproduction is permitted which does not comply with these terms.
*Correspondence: Julia Hoefele, anVsaWEuaG9lZmVsZUB0dW0uZGU=
†These authors have contributed equally to this work
‡ORCID: Korbinian M. Riedhammer, orcid.org/0000-0002-7503-5801
Disclaimer: All claims expressed in this article are solely those of the authors and do not necessarily represent those of their affiliated organizations, or those of the publisher, the editors and the reviewers. Any product that may be evaluated in this article or claim that may be made by its manufacturer is not guaranteed or endorsed by the publisher.
Research integrity at Frontiers
Learn more about the work of our research integrity team to safeguard the quality of each article we publish.