- 1School of Medicine, Western Sydney University, Campbelltown, NSW, Australia
- 2Translational Health Research Institute, Western Sydney University, Campbelltown, NSW, Australia
Decreased light transmittance through the ocular lens, termed cataract, is a leading cause of low vision and blindness worldwide. Cataract causes significantly decreased quality of life, particularly in the elderly. Environmental risk factors, including aging, UV exposure, diabetes, smoking and some prescription drugs, are all contributors to cataract formation. In particular, drug-induced cataract represents a poorly-addressed source of cataract. To better understand the potential impact of prescription drugs on cataract, we analyzed publicly-available drug prescriptions data from the Australian Pharmaceutical Benefits Scheme. The data was analyzed for the 5-year period from July 2014 to June 2019. Analyses included the number of prescriptions for each drug, as well as the associated government and total prescription costs. The drugs chosen for analysis belonged to any of four broad categories—those with known, probable, possible or uncertain association with cataract in patients. The analyses revealed high prescription rates and costs for drugs in the Known category (e.g., steroids) and Possible category (e.g., psychotropic drugs). Collectively, these data provide valuable insights into specific prescription drugs that likely contribute to the increasing annual burden of new cataract cases. These data highlight the need—as well as new, stem cell-based opportunities—to elucidate molecular mechanisms of drug-induced cataract formation.
Introduction
Cataracts disrupt light transmission through the lens of the eye. Excluding uncorrected refractive errors, cataracts are the leading cause of blindness and low vision worldwide—with over 65 million (M) patients affected in 2015 (1). Current cataract treatment involves removal of the cataractous lens tissue and replacement of lens function with a synthetic intra-ocular lens. Where access to treatment is available, cataract surgery is a relatively simple and effective approach to restoring vision. However, despite continued advances in cataract surgery, the number of patients affected by cataract continues to increase (1).
Over 70 drugs have been associated with a known or suspected increased risk of cataract formation (2). These drugs can be grouped into four distinct categories based on the evidence underpinning their association with cataract formation: (i) Known category drugs are known to increase the risk of cataract in patients; (ii) Probable category drugs are likely to cause increased risk of cataracts; (iii) Possible category drugs, and (iv) Uncertain category drugs may increase cataract risk but the data is inconclusive (Supplementary Table 1). A collective analysis of the prescribing rates for the cataract-associated drugs in these four categories is yet to be performed.
In Australia, the Federal Government manages two programs that provide universal healthcare to citizens, permanent residents, and some international travelers. The Medicare scheme provides access to health and hospital services, including cataract surgery, at low or no cost. The Pharmaceutical Benefits Scheme (PBS) subsidizes the cost of prescription medicines approved for inclusion in the scheme. Among the PBS-listed medicines are drugs known or suspected to increase the risk of cataract. To investigate the number of prescriptions and associated costs for these drugs in Australia, we examined publicly available, PBS drug prescriptions data for the 5-year period from July 2014 to June 2019.
Results
Known category drugs dominated by high and increasing glucocorticoid prescriptions
Of the 31 drugs known to increase the risk of cataract (Supplementary Table 1), analysis of the PBS data revealed 17 were prescribed over the 5-year period analyzed (Table 1). The top 3 most prescribed Known category drugs averaged more than 1 M prescriptions per year, and cost from $18 M to $53 M a year each (Table 1).
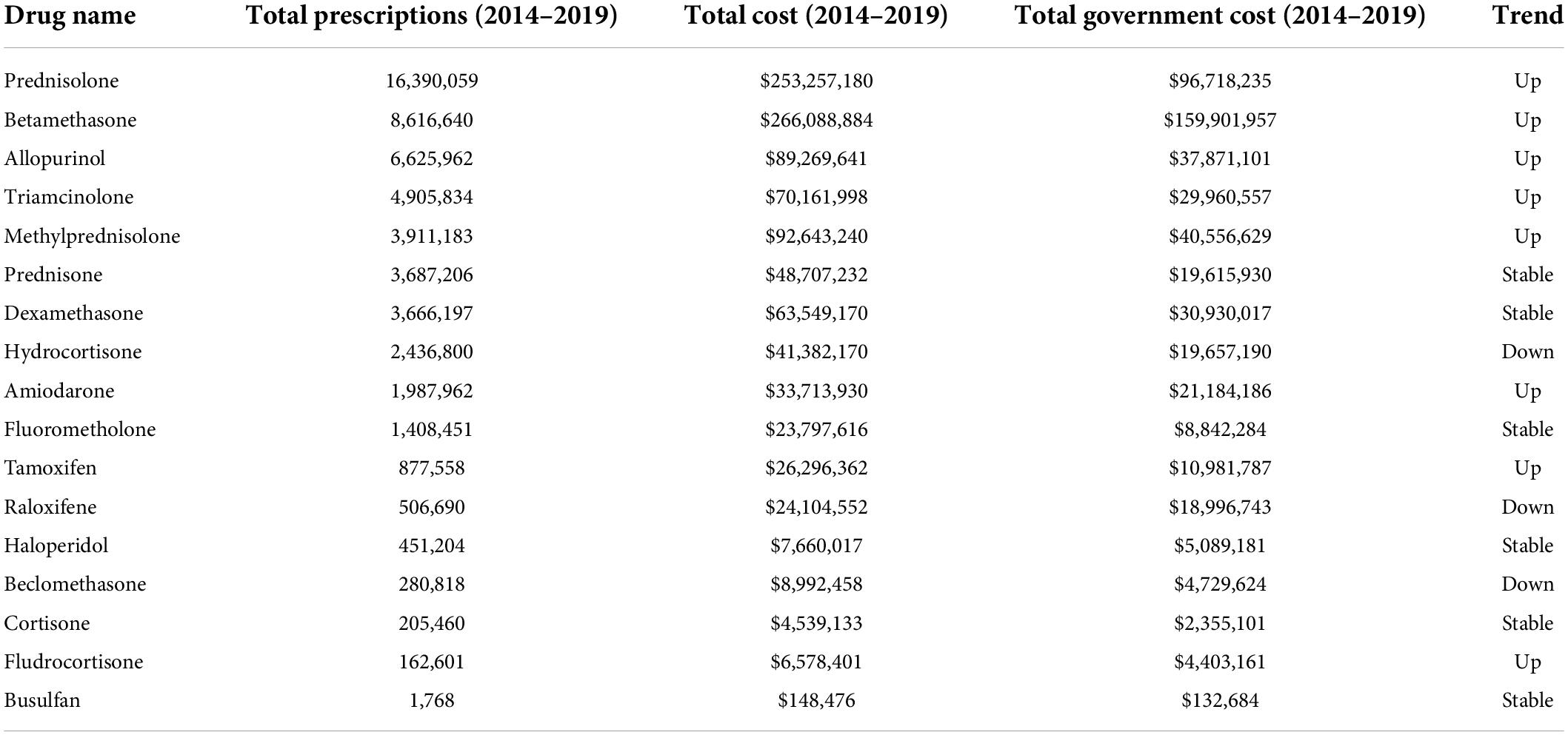
Table 1. PBS data (2014–2019) showing prescription numbers and costs for members of the known category of drugs associated with cataract (gray background = steroids).
Most notable among the Known category drugs prescribed over the 5 years were 11 steroids, of which 8 were in the top 10 most prescribed Known drugs (Table 1). On average, each year over 9 M total steroid prescriptions were supplied at a combined average annual cost of > $175 M (Figure 1). Prednisolone (>3 M prescriptions and > $50 M per year) and betamethasone (>1.7 M prescriptions and > $53 M per year) were the most prescribed and also the most costly drugs in the Known category.
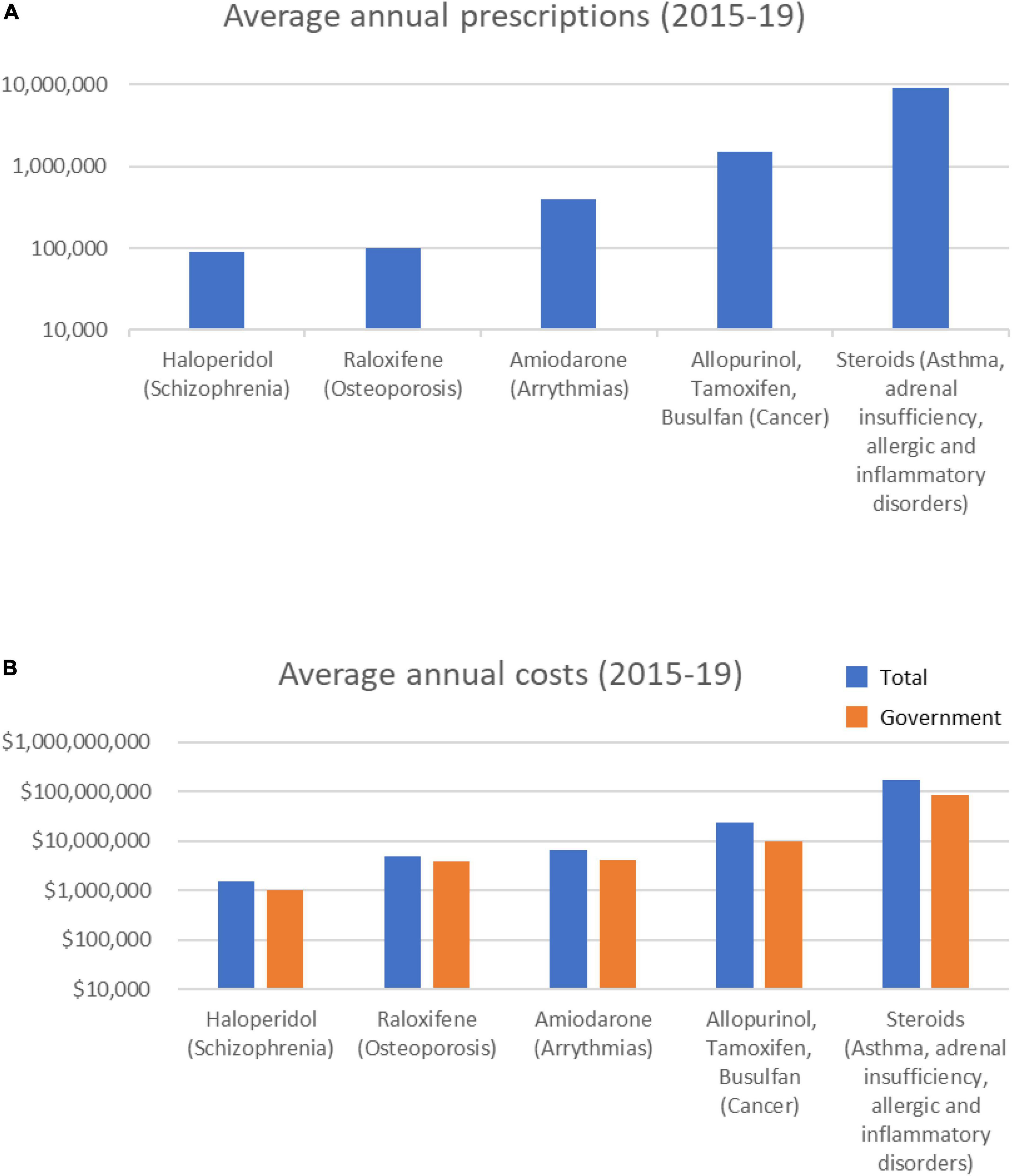
Figure 1. Average annual prescription numbers (A) and costs (B) for members of the Known category of drugs associated with cataract, together with typical conditions for which they are prescribed.
Over the 5-year period, 8 Known category drugs showed increasing annual prescriptions (5 steroids) and 9 showed increasing annual costs (Supplementary Figure 1). The total number of annual steroid prescriptions increased 7.7% over the 5 years (9,031,988 in 2014/15 to 9,725,044 in 2018/19), and the associated costs increased 42.9% $142M in 2014/15 to $203M in 2018/19).
Overall, these data indicate that numerous Known cataract-inducing drugs are being increasingly used. At a combined average annual cost of >$212 M per year, of which the direct government cost is > $102 M, it is clear a large investment is being made to treat patients using drugs known to increase the rate of cataract formation.
Probable cataract-inducing drugs largely involves methotrexate
Of the 9 drugs classified as having a probable association with cataract formation (Supplementary Table 1), only two were prescribed over the 5-year period analyzed–methotrexate and pilocarpine (Table 2). These drugs are prescribed for conditions including cancer and rheumatoid arthritis (methotrexate), or glaucoma (pilocarpine). Methotrexate averaged > 330,000 annual prescriptions (Figure 2), with prescriptions increasing ∼28% over the 5 years (from 298,589 in 2014/15 to 398,799 in 2018/19). Average methotrexate costs were > $11 M/yr (Figure 2), having increased 49% over the 5 years ($9.9M to $14.7M; Supplementary Figure 2). In contrast, pilocarpine, averaged > 40,000 prescriptions/year at an average annual cost of $677,000/year (Figure 2). The prescribing rate for pilocarpine steadily decreased over the 5 years (Supplementary Figure 2).

Table 2. PBS data (2014–2019) showing prescription numbers and costs for members of the probable category of drugs associated with cataract.
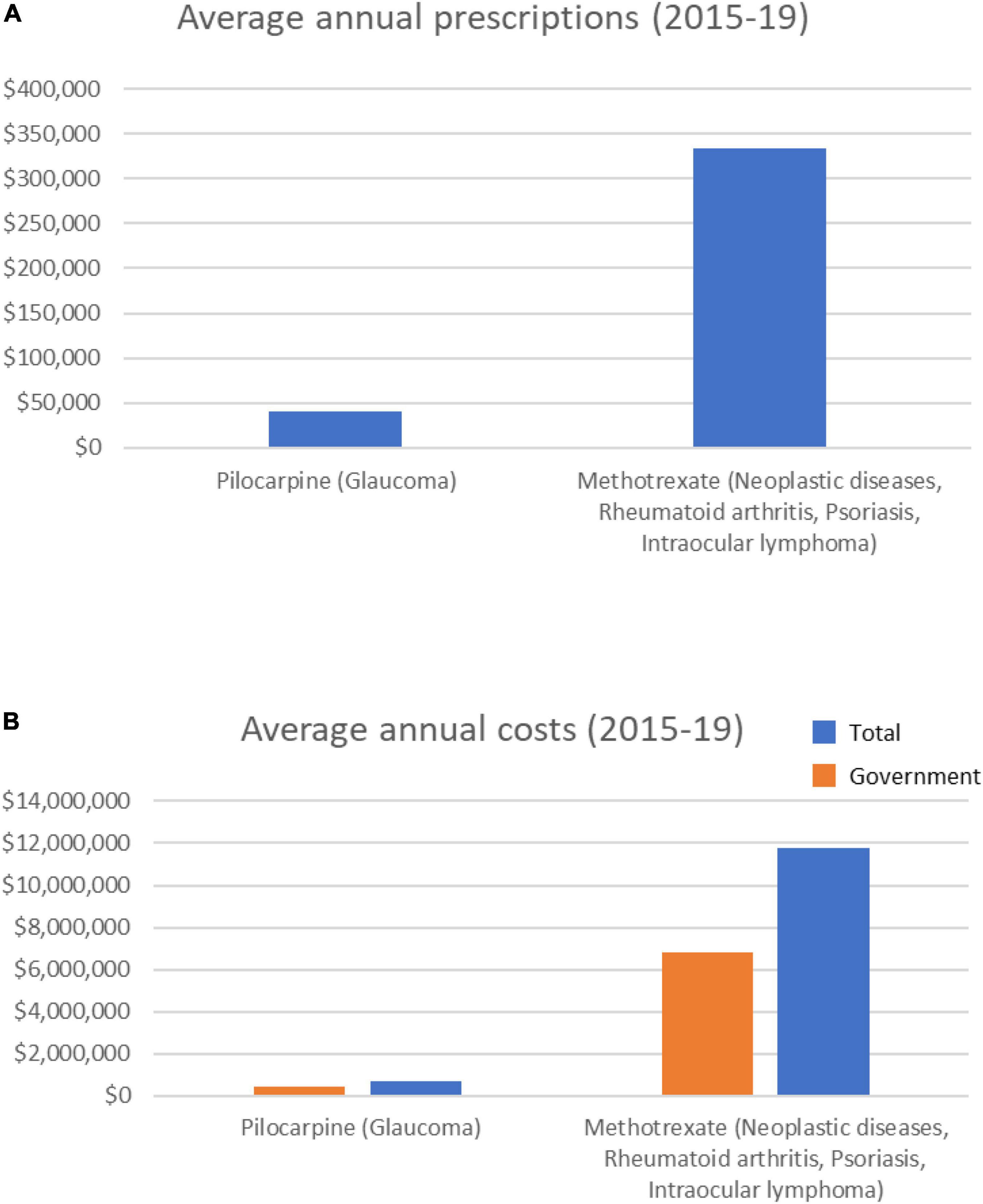
Figure 2. Average annual prescription numbers (A) and costs (B) for members of the probable category of drugs associated with cataract, together with typical conditions for which they are prescribed.
Possible cataract-inducing drugs dominated by psychotropic drugs
Of the 23 drugs having a possible association with increased risk of cataract (Supplementary Table 1), 14 were prescribed over the 5 years (Table 3). These drugs are typically prescribed for conditions from depression to diabetes mellitus. Notably, 8 psychotropic drugs were in the top 10 most prescribed drugs in this category (Table 3). The top 6 most prescribed Possible category drugs averaged more than 1 M prescriptions per year and cost from $16 M to $53 M annually (Figure 3). Psychotropic drug prescriptions averaged > 14 M annually, at a combined average annual cost of >$237 M. Escitalopram (>3.9 M prescriptions and > $53 M per year) and sertraline (>3.9 M prescriptions and >$50 M per year) were the most prescribed and most costly drugs.
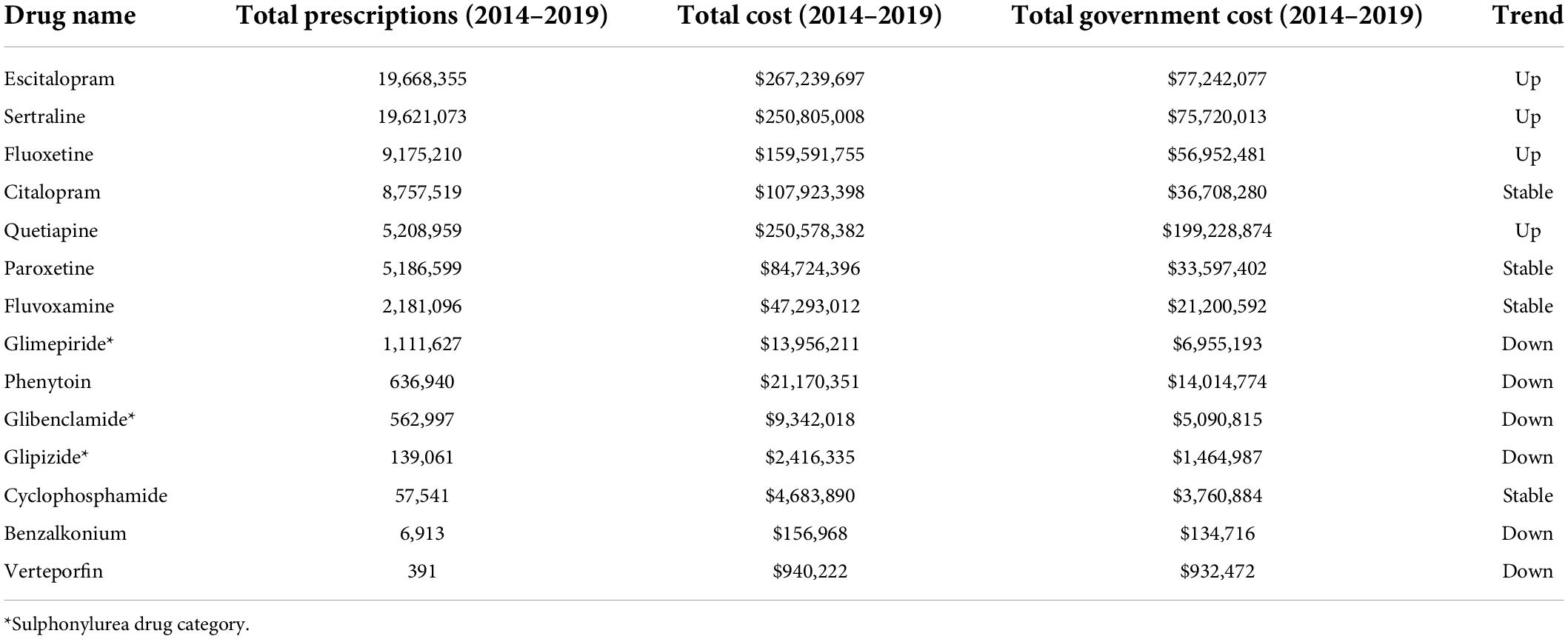
Table 3. PBS data (2014–2019) showing prescription and cost data for drugs that have a probable association with cataract; drugs with gray background are psychotropic drugs (gray background = psychotropic drugs).
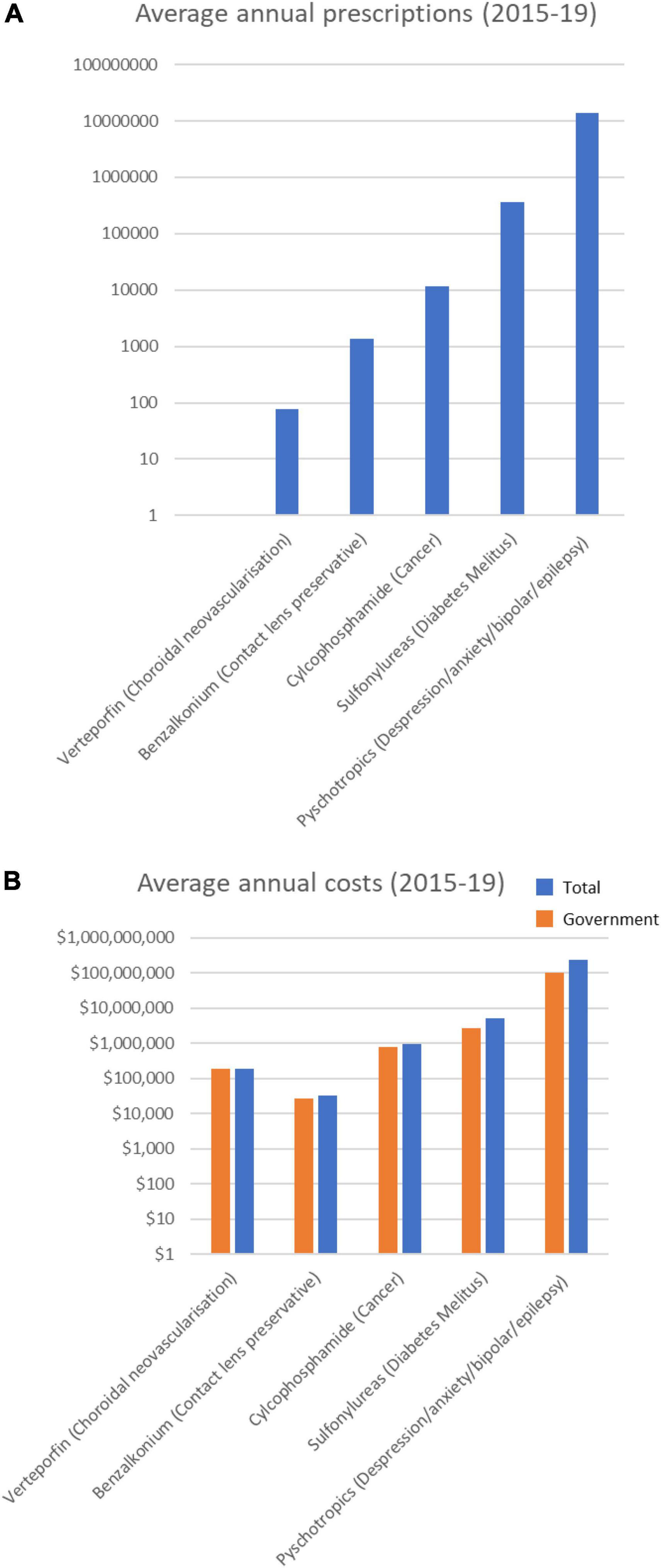
Figure 3. Average annual prescription numbers (A) and costs (B) for members of the Possible category of drugs associated with cataract, together with typical conditions for which they are prescribed.
Of the 14 drugs in the Possible Category, 4 (all psychotropic drugs) increased in annual prescriptions, government cost and total cost, including 4 of the top 5 drugs (Supplementary Figure 3). The other 4 psychotropic drugs showed relatively stable prescribing rates over the past 5 years. Overall, the number of annual psychotropic prescriptions increased by 19.7% over the 5 years (from 12.8 M in 2014/15 to 15.4 M in 2018/19), with an associated increase in costs 7% (from $230 M in 2014/15 to $246 M in 2018/19).
The other drugs having a Possible association with cataracts were the sulfonylureas (Table 3) typically used to treat diabetes mellitus. Sulfonylureas were prescribed at an annual rate of > 360,000 prescriptions a year (Figure 3), costing ∼$5 M/year, and with a steadily decreasing prescribing rate over the 5 years (Supplementary Table 2).
Statins dominate the drugs with uncertain effects on cataract: Statins
Of the 15 drugs in the Uncertain category (Supplementary Table 1), the PBS data revealed 11 were prescribed over the 5-year period (Table 4). These drugs are prescribed for conditions ranging from hypercholesterolemia to endometriosis. The top three most prescribed averaged > 3 M annual prescriptions, and cost from $107 M to $260 M a year each (Figure 4). Most notable among these Uncertain category drugs were 5 statins, 3 of which were in the top five most prescribed (Table 4). Atorvastatin (> 12 M prescriptions and > $224 M per year) and rosuvastatin (>10 M prescriptions and > $260 M per year) were the most prescribed and most costly over the 5-year period. On average each year > 27 M statin prescriptions were filled in total at a combined average annual cost of over $604 M.
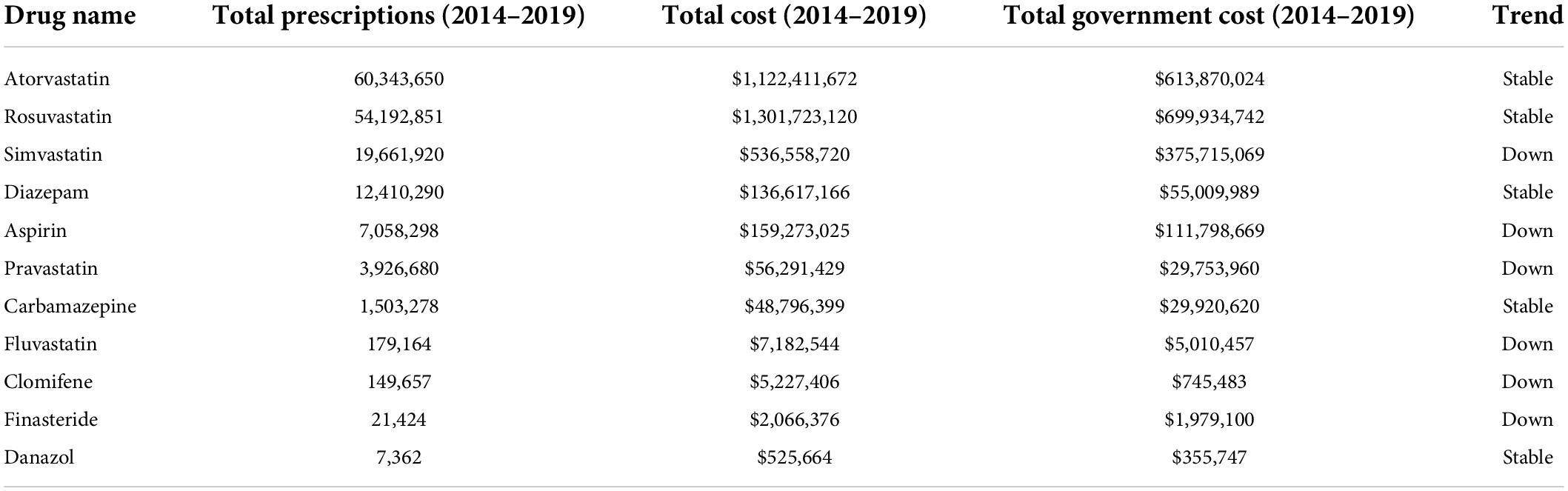
Table 4. PBS data (2014–2019) showing prescription numbers and costs for members of the uncertain category of drugs associated with cataract (gray background = statins).
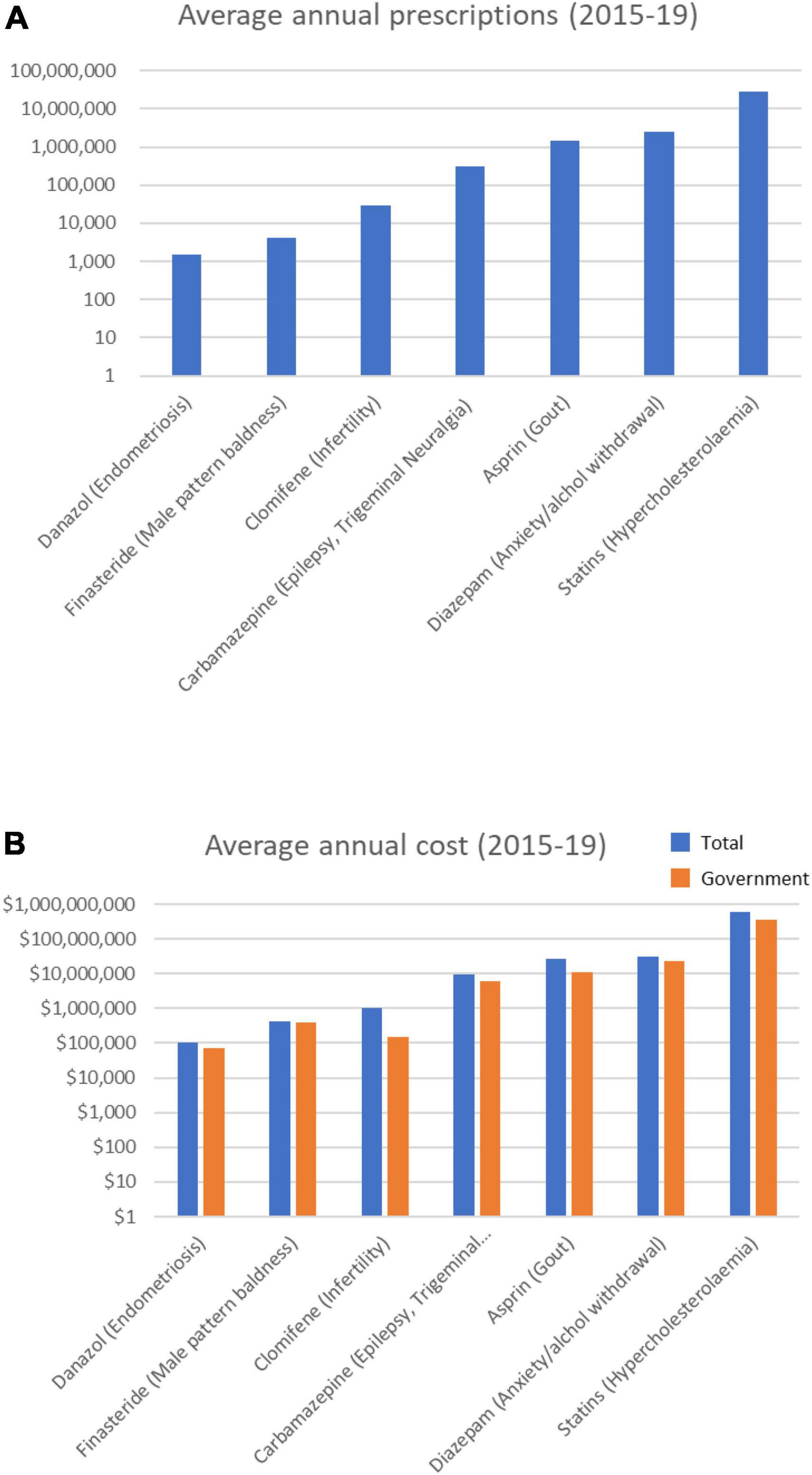
Figure 4. Average annual prescription numbers (A) and costs (B) for members of the Uncertain category of drugs associated with cataract, together with typical conditions for which they are prescribed.
The prescribing rates of statins were mixed, with some trending up and others trending down over the 5 years (Supplementary Figure 4). Statins were the highest prescribed and most costly drugs in this study (>138 M prescriptions and > $3 billion over 5 years). Overall, the number of annual statin prescriptions increased by 11.8% over the 5 years (from 26,064,117 in 2014/15 to 29,127,562 in 2018/19), though the total costs decreased by –33% (from $775M in 2014/15 to $516M in 2018/19). The annual governments costs decreased by 51% (from ∼$510M in 2014/15 to ∼$249M in 2018/19).
Discussion
The analysis of PBS data presented here shows tens-of- millions of prescriptions are filled every year in Australia for drugs known to or suspected of inducing cataract. The most prescribed and costly of these drugs are glucocorticoids, psychotropic drugs, and statins.
Glucocorticoids
Glucocorticoids—a class of drug known to cause posterior subcapsular cataract, particularly in people above the age of 40 (3)—are prescribed for a variety of diseases including ocular conditions (e.g., macula edema), asthma, arthritis and inflammatory bowel disease (4, 5). The data here showed an average of ∼9 M annual steroid prescriptions in Australian over the 5 years from 2014 to 2019. Over that period, the annual number of steroid prescriptions increased 7.7% to 9,725,044 in 2018/19, and the associated costs increased by 42.9% to $203M. A UK trial found, on average, patients require 6.5 glucocorticoid prescriptions (6), and approximately 50% of these patients were 45 years and above (7). Using this as a guide, this equates to ∼1.5 M Australians using prescription steroids in 2018/19 with approximately 750,000 patients 45 years or older. In Australia, the increased risk of PSC due to glucocorticoids is OR 2.5 for inhaled and OR 4.1 for oral corticosteroids. Glucocorticoids were also associated with an increase in nuclear cataracts, with an OR of 2.0 for inhaled and 3.5 for oral corticosteroids (mean age 63 years) (3).
As discussed below, minimizing drug-induced cataract could help offset the burden of cataract in Australia and elsewhere. The large number and cost of cataract surgeries likely arising from prescription steroids provides a strong argument for better understanding the molecular mechanisms of steroid-induced cataract. Application of such molecular knowledge could lead to early identification of at-risk patients, and potentially to development of alternative treatment strategies. For example, folate is recommended as a co-therapy with methotrexate prescribed for rheumatoid arthritis, to mitigate risks associated with methotrexate-induced folate deficiency (8).
Clinically, the cataracts caused by glucocorticoid steroids are centrally-located, posterior subscapular cataracts with vacuoles (9, 10)—suggesting they relate to aberrant migration of lens epithelial cells (LECs) along the posterior capsule. At present, there is little data describing the molecular mechanisms of steroid-induced cataracts in human lenses. While steroids can bind to lens proteins, this is generally discounted as a mechanism for cataract formation as they do so with lower affinity than other proteins that do not induce cataract (11).
Primary human LECs transfected with firefly luciferase (controlled by glucocorticoid response elements) showed increased luciferase activity when exposed to dexamethasone (12)—demonstrating the ability of primary human LECs to activate the glucocorticoid receptor. In the same study, microarray analysis of the immortalized human LEC line, HLE B-3, after dexamethasone treatment revealed altered expression of various genes (136 and 86 genes after 4 and 16 h of treatment, respectively).
Analysis of primary human LECs from patients with steroid-induced cataract showed a small increase in mRNA expression and protein activity for the matrix metalloproteinases, MMP2 and MMP-9 (13). Additional studies using primary and immortalized human LECs showed dexamethasone treatment led to phosphorylation of the glucocorticoid receptor, altered expression of MAPK and PI3K/AKT regulators, decreased phosphorylation of MAPK- and AKT-related proteins (14), and altered expression of cell adhesion molecules (15). No detectable effect of dexamethasone on proliferation or apoptosis of the human LEC line (HLE B-3) was observed, though some dexamethasone-induced apoptosis in human lens cells has been reported elsewhere (16, 17).
Overall, these studies provide initial insights into steroid-induced effects in human lens cells. However, their clinical relevance remains unclear due to differences (abnormalities) in behavior of immortalized lens cells compared to normal primary human LECs, and also the short timeframes being analyzed (i.e., typically < 24 h). Notably, none of these in vitro studies involving human LECs or lens cell lines were able to assess the effects of dexamethasone on critical lens functional properties of transparency or focusing.
Psychotropic drugs
In Australia, one in five people experienced a mental disorder in a 12-month period (18), with many of them prescribed psychotropic drugs. The PBS data show eight psychotropic drugs from the possible category were prescribed in Australia, with most averaging > 1 M prescriptions a year. The average annual number of psychotropic drug prescriptions was > 14 M, with an associated cost of > $237 M per year. Annual prescriptions increased 19.7% over the 5 years to 15.4 M in 2018/19, at a cost of $246 M (a 7% increase over the 5 years).
Published health record analyses have shown significant positive associations between risk of cataract formation and use of psychotropic drugs, including: citalopram (OR = 1.53, 95% CI, 1.33–1.77; P < 0.001) and fluvoxamine (RR, 1.39; 95% CI, 1.07–1.80) (19, 20); fluoxetine (AOR: 1.21; 95% CI, 1.01–1.46, p = 0.042) (21), fluvoxamine (AOR: 1.47; 95% CI: 1.01–2.12, p = 0.043) and sertraline (AOR: 1.29; 95% CI, 1.12–1.48, p < 0.001); sertraline (AOR: 1.29; 95% CI, 1.12–1.48, p < 0.001) and fluvoxamine (AOR: 1.37; 95% CI, 1.07–1.76, p = 0.012) (21). A recent meta-analysis also identified an association between cataract and use of fluoxetine (RR 1.08, 95% CI, 1.03–1.12) and fluvoxamine (RR 1.22, 95% CI, 1.06–1.40) (22).
These studies also showed many working-age patients (e.g., 50–64) who have taken these psychotropic drugs have required cataract surgery, with direct implications for labor force productivity (discussed below) as well as direct medical costs. In some studies, the average time to cataract diagnosis while on SSRI (Selective Serotonin Reuptake Inhibitor) therapy was relatively short, ∼656 days (20). The large annual number of psychotropic prescriptions in Australia may lead to sizeable numbers of drug-induced cataract.
At present, it is not clear how psychotropic drugs could lead to cataract formation in patients. It is possible selective serotonin reuptake inhibitors (such as citalopram, escitalopram, fluoxetine, fluvoxamine and sertraline all prescribed in Australia) could lead to elevated levels of serotonin, as detected in the aqueous humor of patients having undergone cataract surgery (23). In rats, application of serotonin via injection or eyedrops led to rapid development of dense cataracts thought to be related to reduced aqueous (24). These findings suggest an indirect mechanism for cataract formation via selective serotonin reuptake inhibitors. However, rabbit lenses express serotonin receptors (e.g., 5-HT1A and 5-HT7) (25) and exposure of rabbit LECs to serotonin led to phosphoinositide turnover (26). A more comprehensive analysis of the effects of psychotropic drugs on human cataract formation is needed.
Statins
Statins are prescribed to reduce blood cholesterol levels in order to reduce the risk of cardiovascular disease. Statins are effective inhibitors of 3-hydroxy-3-methyl-glutaryl-CoA reductase, a key enzyme in cholesterol biosynthesis. Statins can also increase expression of low density lipoprotein (LDL) receptors, enabling liver cells to capture cholesterol-containing LDL particles from the blood (27). The PBS data revealed the average annual number of statin prescriptions in Australia is > 27 M, with an average annual cost of > $604 M. Approximately 44% of Australians were prescribed and used statins in 2016 (28). Statins are listed in the Uncertain category of cataract-inducing drugs. In the lens, it appears cholesterol levels need to be maintained within a relatively narrow range to avoid cataract formation (29). Increased cholesterol levels in the lens–for example, 25-hydroxycholesterol–have been associated with cataract (30). Conversely, the cholesterol-lowering drug, triparanol, also causes irreversible cataract (31). Triparanol inhibits cholesterol synthesis downstream of lanosterol production, leading to accumulation of lanosterol in lenses (32, 33). While recent reports suggest cataracts can be dissolved with intermediates of cholesterol biosynthesis–lanosterol or 25-hydroxycholesterol (34, 35) (that increase the chaperone activity of α-crystallin)–other studies have failed to replicate these effects anti-cataract effects (30, 36, 37). Perhaps unsurprisingly, the evidence relating to statins and cataract formation has been contradictory. Outcomes from systematic reviews have been mixed–with positive, negative and no associations all identified (38–41). A recent meta-analysis encompassing 313,200 patients found no association with cataract. However, given the heterogeneity in the studies underpinning the meta-analysis, it was recommended that large, multicenter, pragmatic, prospective observational studies or registries be performed to assess the risk of cataracts arising from statin use (41).
Elucidating mechanisms of drug-induced cataracts in humans
Worldwide, cataracts are a large and increasing cause of blindness. The number of people with low vision or blindness due to cataracts increased from 50.5 M in 1990 to 65.2 M in 2015 (1), because of the increasing size and age of populations worldwide. The PBS data analysis presented here indicates prescription drugs could be a significant source of cataracts in Australia. In the United States, outpatient services are used annually by 1.6 M cataract patients aged 40-64 years, and 8.9 M aged 65 and older (42). Direct medical costs attributed to these two groups are $2.14 billion/year and $4.66 billion/year, respectively. US cataract patients also contributed to $11.2 billion in other annual direct costs (e.g., care programs); and $8 billion in annual productivity losses (e.g., lower participation and lower wages). Worldwide, the economic, employment and social consequences of cataracts cost $tens-of-billions annually. It is possible a significant proportion of annual cataract cases arise due to prescription drug use in both working-aged people and retirees. However, cataractous human lens tissue is difficult to obtain, and transparent/light-focusing human lens tissue is essentially impossible to reliably obtain in meaningful amounts for research during the early stages of cataract formation.
Human pluripotent stem cells offer the ability to generate large numbers of human LECs and light-focusing micro-lenses (43, 44). These stem cell-derived human LECs share morphological, transcriptional and proteomic profiles similar to fetal human LECs (43, 44). Light-focusing micro-lenses derived from these human LECs share similar anatomical and molecular characteristics with human lenses, including expression of a broad range of crystallin proteins associated with the focusing ability of primary human lenses (43, 44). Notably, exposing human stem cell-derived micro-lenses to a cystic fibrosis drug suspected of causing cataract in human patients (43, 44), or to dexamethasone (45), resulted in decreased light focusing in the treated micro-lenses. Together, these data suggest human stem cell-derived micro-lenses may provide a useful new tool for investigating the initiating molecular mechanisms of drug-induced cataract. Consistent with this, the human micro-lens system is amenable to detailed analyses including imaging (light, confocal and electron microscopy), transcriptomics and proteomics. Thus, the human micro-lens system provides a novel and potentially powerful approach to time-course cataract studies in vitro, with lens transparency and light-focusing as functional end-points. This includes new opportunities to elucidate the molecular mechanisms through which prescription drugs cause cataracts. Such new knowledge could provide opportunities to decrease the annual global burden of cataract through improved identification of at-risk patients, prescription of co-therapies, or identification of candidate anti-cataract drugs. Such studies would address the large, unmet need for a reduction in the amount of drug-induced cataract that currently occurs worldwide. Use of human stem cell-derived micro-lenses could also provide a functional human lens system to reduce reliance on animal-based lens models for investigating molecular mechanisms of cataract formation (46, 47).
Materials and methods
Public PBS drug prescription data from Australia was analyzed for the period 2014 to 2019, to identify the prescribing rates for drugs associated with cataract formation. Categories of drugs that have different associations with cataract formation were obtained from Drug-Induced Ocular Side Effects, 7th Edition. Australian PBS data supply records were downloaded from PBS and RPBS Section 85 Date of Supply Data (48). This data was divided into financial years from July to June based on the month of supply on the PBS per item. Item codes for drugs of interest were matched with the item codes in the PBS prescription record data. Total frequency of prescriptions, cost and government cost for the July–June financial years from 2014 to 2019. The frequency of total prescriptions, average annual prescription rates, total cost, average annual cost and total government cost were calculated for each potential cataract causing agent.
Data availability statement
Publicly available datasets were analyzed in this study. This data can be found here: Health AGD of Pharmaceutical Benefits Scheme (PBS) and PBS and RPBS Section 85 Date of Supply Data.
Author contributions
MO’C: conceptualization, resources, project administration, and funding acquisition. MO’C and KM: methodology and supervision. JC and MO’C: validation and visualization. JC: formal analysis, investigation, and writing—original draft preparation. JC, MO’C, and KM: writing—review and editing. All authors have read and agreed to the published version of the manuscript.
Conflict of interest
The authors declare that the research was conducted in the absence of any commercial or financial relationships that could be construed as a potential conflict of interest.
Publisher’s note
All claims expressed in this article are solely those of the authors and do not necessarily represent those of their affiliated organizations, or those of the publisher, the editors and the reviewers. Any product that may be evaluated in this article, or claim that may be made by its manufacturer, is not guaranteed or endorsed by the publisher.
Supplementary material
The Supplementary Material for this article can be found online at: https://www.frontiersin.org/articles/10.3389/fmed.2022.947659/full#supplementary-material
Abbreviations
AOR, adjusted odds ratio; CI, confidence interval; LEC, lens epithelial cell; M, million; PBS, Pharmaceutical Benefits Scheme; RR, relative risk.
References
1. Flaxman SR, Bourne RRA, Resnikoff S, Ackland P, Braithwaite T, Cicinelli MV, et al. Global causes of blindness and distance vision impairment 1990-2020: a systematic review and meta-analysis. Lancet Glob Health. (2017) 5:e1221–34.
2. Fraunfelder FFT, Fraunfelder FRW, Chambers WA. Drug-Induced Ocular Side Effects. Amsterdam: Elsevier (2014). p. 47–343. doi: 10.1016/B978-0-323-31984-3.00005-2
3. Wang JJ, Rochtchina E, Tan AG, Cumming RG, Leeder SR, Mitchell P, et al. Use of inhaled and oral corticosteroids and the long-term risk of cataract. Ophthalmology. (2009) 116:652–7. doi: 10.1016/j.ophtha.2008.12.001
4. Chawan-Saad J, Wu M, Wu A, Wu L. Corticosteroids for diabetic macular edema. Taiwan J Ophthalmol. (2019) 9:233–42. doi: 10.4103/tjo.tjo_68_19
5. Imam L, Haboubi HN. G-Eye: ocular manifestations of gastrointestinal disease. Frontline Gastroenterol. (2020) 11:162–7. doi: 10.1136/flgastro-2018-101083
6. van Staa TP, Leufkens HG, Abenhaim L, Begaud B, Zhang B, Cooper C, et al. Use of oral corticosteroids in the United Kingdom. QJM. (2000) 93:105–11. doi: 10.1093/qjmed/93.2.105
7. Waljee AK, Rogers MAM, Lin P, Singal AG, Stein JD, Marks RM, et al. Short term use of oral corticosteroids and related harms among adults in the United States: population based cohort study. BMJ. (2017) 357:j1415. doi: 10.1136/bmj.j1415
8. Whittle SL, Hughes RA. Folate supplementation and methotrexate treatment in rheumatoid arthritis: a review. Rheumatology (Oxford). (2004) 43:267–71. doi: 10.1093/rheumatology/keh088
9. James ER. The etiology of steroid cataract. J Ocul Pharmacol Ther. (2007) 23:403–20. doi: 10.1089/jop.2006.0067
10. Eshagian J. Human posterior subcapsular cataracts. Trans Ophthalmol Soc U K. (1982) 102(Pt 3):364–8.
11. Dickerson JE, Dotzel E, Clark AF. Steroid-induced cataract: new perspective from in vitro and lens culture studies. Exp Eye Res. (1997) 65:507–16. doi: 10.1006/exer.1997.0359
12. Gupta V, Galante A, Soteropoulos P, Guo S, Wagner BJ. Global gene profiling reveals novel glucocorticoid induced changes in gene expression of human lens epithelial cells. Mol Vis. (2005) 11:1018–40.
13. Alapure BV, Praveen MR, Gajjar DU. Matrix metalloproteinase–2 and –9 activities in the human lens epithelial cells and serum of steroid induced posterior subcapsular cataracts. Mol Vis. (2012) 18:64–73.
14. Gupta V, Awasthi N, Wagner BJ. Specific activation of the glucocorticoid receptor and modulation of signal transduction pathways in human lens epithelial cells. Invest Ophthalmol Vis Sci. (2007) 48:1724–34. doi: 10.1167/iovs.06-0889
15. Celojevic D, Carlsson T, Johansson B, Nannmark U, Petersen A. Cell adhesion molecule expression in human lens epithelial cells after corticosteroid exposure. Open Ophthalmol J. (2012) 6:42–8. doi: 10.2174/1874364101206010042
16. Wang L, Zhao W, Leng F, Ge J, Bu Z, Zhang Y, et al. Glucocorticoid receptors take part in the apoptotic process of human lens epithelial cells, but the glucocorticoid receptor antagonist RU486 does not rescue the cells fully. Mol Biosyst. (2011) 7:1926–37. doi: 10.1039/c1mb05045a
17. Petersen A, Carlsson T, Karlsson J-O, Jonhede S, Zetterberg M. Effects of dexamethasone on human lens epithelial cells in culture. Mol Vis. (2008) 14:1344–52.
18. Mental Health. Australian Institute of Health and Welfare. (2021). Available online at: https://www.aihw.gov.au/reports/australias-health/mental-health (accessed May 15, 2021).
19. Erie JC, Brue SM, Chamberlain AM, Hodge DO. Selective serotonin reuptake inhibitor use and increased risk of cataract surgery: a population-based, case-control study. Am J Ophthalmol. (2014) 158:192–7.e1. doi: 10.1016/j.ajo.2014.03.006
20. Etminan M, Mikelberg FS, Brophy JM. Selective serotonin reuptake inhibitors and the risk of cataracts: a nested case-control study. Ophthalmology. (2010) 117:1251–5. doi: 10.1016/j.ophtha.2009.11.042
21. Chou P-H, Chu C-S, Chen Y-H, Hsu MY, Huang MW, Lan TH, et al. Antidepressants and risk of cataract development: a population-based, nested case-control study. J Affect Disord. (2017) 215:237–44. doi: 10.1016/j.jad.2017.03.044
22. Fu Y, Dai Q, Zhu L, Wu S. Antidepressants use and risk of cataract development: a systematic review and meta-analysis. BMC Ophthalmol. (2018) 18:31. doi: 10.1186/s12886-018-0699-0
23. Trope GE, Sole M, Aedy L, Madapallimattam A. Levels of norepinephrine, epinephrine, dopamine, serotonin and N-acetylserotonin in aqueous humour. Can J Ophthalmol. (1987) 22:152–4.
24. Boerrigter RM, Siertsema JV, Kema IP. Serotonin (5-HT) and the rat’s eye. Some pilot studies. Doc Ophthalmol. (1992) 82:141–50. doi: 10.1007/BF00157004
25. Chidlow G, Le Corre S, Osborne NN. Localization of 5-hydroxytryptamine1A and 5-hydroxytryptamine7 receptors in rabbit ocular and brain tissues. Neuroscience. (1998) 87:675–89. doi: 10.1016/s0306-4522(98)00181-x
26. Vivekanandan S, Lou MF. Evidence for the presence of phosphoinositide cycle and its involvement in cellular signal transduction in the rabbit lens. Curr Eye Res. (1989) 8:101–11. doi: 10.3109/02713688909013899
27. Goldstein JL, Brown MS. A century of cholesterol and coronaries: from plaques to genes to statins. Cell. (2015) 161:161–72. doi: 10.1016/j.cell.2015.01.036
28. Ofori-Asenso R, Ilomäki J, Zomer E, Curtis AJ, Zoungas S, Liew D, et al. A 10-Year trend in statin use among older adults in australia: an analysis using national pharmacy claims data. Cardiovasc Drugs Ther. (2018) 32:265–72. doi: 10.1007/s10557-018-6794-x
29. Widomska J, Subczynski WK. Why is very high cholesterol content beneficial for the eye lens but negative for other organs? Nutrients. (2019) 11:E1083. doi: 10.3390/nu11051083
30. Girão H, Mota MC, Ramalho J, Pereira P. Cholesterol oxides accumulate in human cataracts. Exp Eye Res. (1998) 66:645–52. doi: 10.1006/exer.1998.0465
31. Kirby TJ. Cataracts produced by triparanol. (MER-29). Trans Am Ophthalmol Soc. (1967) 65:494–543.
32. Popják G, Meenan A, Parish EJ, Nes WD. Inhibition of cholesterol synthesis and cell growth by 24(R,S),25-iminolanosterol and triparanol in cultured rat hepatoma cells. J Biol Chem. (1989) 264:6230–8. doi: 10.1016/S0021-9258(18)83338-5
33. Seo S, Tonda K, Uomori A, Takeda K, Hirata M. Effect of sterol biosynthesis inhibitor, SSF-109, on cholesterol synthesis in isolated rat hepatocytes. Steroids. (1993) 58:74–8. doi: 10.1016/0039-128x(93)90056-s
34. Zhao L, Chen X-J, Zhu J, Xi YB, Yang X, Hu LD, et al. Lanosterol reverses protein aggregation in cataracts. Nature. (2015) 523:607–11. doi: 10.1038/nature14650
35. Makley LN, McMenimen KA, DeVree BT, Goldman JW, McGlasson BN, Rajagopal P, et al. Pharmacological chaperone for α-crystallin partially restores transparency in cataract models. Science. (2015) 350:674–7. doi: 10.1126/science.aac9145
36. Shanmugam PM, Barigali A, Kadaskar J, Borgohain S, Mishra DKC, Ramanjulu R, et al. Effect of lanosterol on human cataract nucleus. Indian J Ophthalmol. (2015) 63:888–90. doi: 10.4103/0301-4738.176040
37. Daszynski DM, Santhoshkumar P, Phadte AS, Sharma KK, Zhong HA, Lou MF, et al. Failure of oxysterols such as lanosterol to restore lens clarity from cataracts. Sci Rep. (2019) 9:8459. doi: 10.1038/s41598-019-44676-4
38. Alves C, Mendes D, Batel Marques F. Statins and risk of cataracts: a systematic review and meta-analysis of observational studies. Cardiovasc Ther. (2018) 36:e12480. doi: 10.1111/1755-5922.12480
39. Kostis JB, Dobrzynski JM. Prevention of cataracts by statins: a meta-analysis. J Cardiovasc Pharmacol Ther. (2014) 19:191–200. doi: 10.1177/1074248413511690
40. Laties AM, Shear CL, Lippa EA, Gould AL, Taylor HR, Hurley DP, et al. Expanded clinical evaluation of lovastatin (EXCEL) study results. II. Assessment of the human lens after 48 weeks of treatment with lovastatin. Am J Cardiol. (1991) 67:447–53. doi: 10.1016/0002-9149(91)90002-3
41. Yu S, Chu Y, Li G, Ren L, Zhang Q, Wu L, et al. Statin use and the risk of cataracts: a systematic review and meta-analysis. J Am Heart Assoc. (2017) 6:e004180. doi: 10.1161/JAHA.116.004180
42. Prevent Blindness America [PBA]. The Economic Impact of Vision Problems. (2017). Available online at: https://preventblindness.org/wp-content/uploads/2020/04/Impact_of_Vision_Problems.pdf (accessed February 5, 2021).
43. Murphy P, Kabir MH, Srivastava T, Mason ME, Dewi CU, Lim S, et al. Light-focusing human micro-lenses generated from pluripotent stem cells model lens development and drug-induced cataract in vitro. Development. (2018) 145:dev155838. doi: 10.1242/dev.155838
44. Dewi CU, Mason M, Cohen-Hyams T, Killingsworth MC, Harman DG, Gnanasambandapillai V, et al. A simplified method for producing human lens epithelial cells and light-focusing micro-lenses from pluripotent stem cells. Exp Eye Res. (2021) 202:108317. doi: 10.1016/j.exer.2020.108317
45. Dewi CU, O’Connor MD. Use of human pluripotent stem cells to define initiating molecular mechanisms of cataract for anti-cataract drug discovery. Cells. (2019) 8:1269. doi: 10.3390/cells8101269
46. Miyashita T, Senshu M, Ibi K, Yamanaka H, Nejishima H, Fukami T, et al. Evaluation of lens opacity due to inhibition of cholesterol biosynthesis using rat lens explant cultures. Toxicology. (2022) 465:153064. doi: 10.1016/j.tox.2021.153064
47. O’Connor MD. The 3R principle: advancing clinical application of human pluripotent stem cells. Stem Cell Res Ther. (2013) 4:21. doi: 10.1186/scrt169
48. Pharmaceutical Benefits Scheme [PBS]. PBS and RPBS Section 85 Date of Supply Data. (2021). Available online at: https://www.pbs.gov.au/info/statistics/dos-and-dop/dos-and-dop (accessed May 15, 2021).
Keywords: prescription drug, dexamethasone, human pluripotent stem cell, micro-lens, cataracts, bioinformatics, lens
Citation: Carlson J, McBride K and O’Connor M (2022) Drugs associated with cataract formation represent an unmet need in cataract research. Front. Med. 9:947659. doi: 10.3389/fmed.2022.947659
Received: 19 May 2022; Accepted: 13 July 2022;
Published: 15 August 2022.
Edited by:
Rita Mencucci, University of Florence, ItalyReviewed by:
Alberto Chiarugi, University of Florence, ItalyRoberto Vignapiano, University of Florence, Italy
Copyright © 2022 Carlson, McBride and O’Connor. This is an open-access article distributed under the terms of the Creative Commons Attribution License (CC BY). The use, distribution or reproduction in other forums is permitted, provided the original author(s) and the copyright owner(s) are credited and that the original publication in this journal is cited, in accordance with accepted academic practice. No use, distribution or reproduction is permitted which does not comply with these terms.
*Correspondence: Michael O’Connor, m.oconnor@westernsydney.edu.au