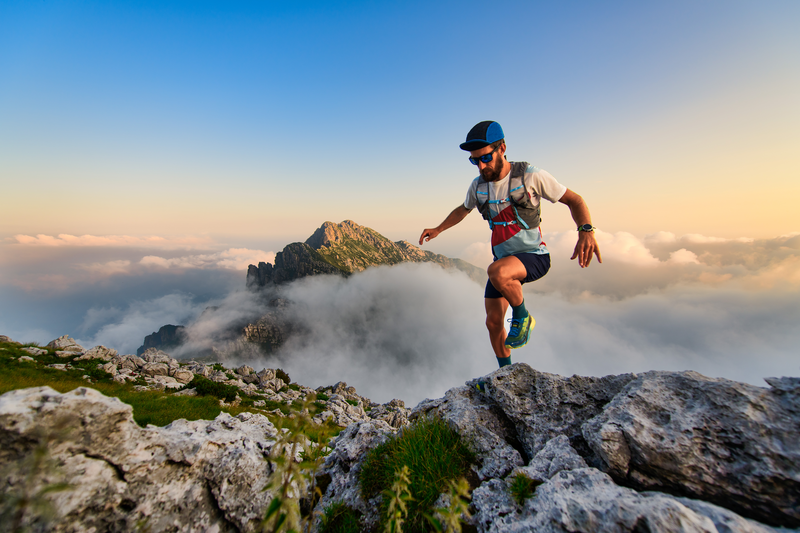
94% of researchers rate our articles as excellent or good
Learn more about the work of our research integrity team to safeguard the quality of each article we publish.
Find out more
REVIEW article
Front. Med. , 29 June 2022
Sec. Intensive Care Medicine and Anesthesiology
Volume 9 - 2022 | https://doi.org/10.3389/fmed.2022.910388
This article is part of the Research Topic Intensive Management of Cardiovascular Disease Patients with Confirmed or Suspected COVID-19 in Area where Chagas Disease is Endemic View all 6 articles
Cardiovascular diseases (CVD) are the most important cause of morbidity and mortality in the general population. Because the high prevalence of COVID-19 and chronic Chagas disease (CCD) where the latter is endemic, all such diseases will likely be observed in the same patient. While COVID-19 can provoke generalized endotheliitis, which can lead to a cytokine storm and a hyper-coagulable state culminating into in-site and at a distance thrombosis. Therefore, small-vessel coronary artery disease (CAD), cerebrovascular disease, thromboembolism, and arrhythmias are prominent findings in COVID-19. In CCD, small-vessel CAD, cardioembolic stroke, pulmonary embolism, heart failure and arrhythmias are frequently observed as a result of a similar but less intense mechanism. Consequently, the association of CCD and COVID-19 will likely increase the incidence of CVD. Thus, doctors on the frontline should be on the alert for this diagnostic possibility so that the proper treatment can be given without any delay.
At the time of this writing, the COVID-19 pandemic has affected more than 312 million people, and ceased life of 5.5 million people worldwide, with a mortality rate approaching 1.76% (1). COVID-19 is caused by SARS-CoV-2, and can unmask, aggravate, or provoke cardiovascular disease (CVD), including acute coronary syndrome, cerebrovascular disease (CEVD), acute heart failure (AHF) [acute decompensating of chronic heart failure (CHF) or de novo heart failure], thromboembolism, arrhythmias, and sudden cardiac death (SCD).
CVD are the main cause of morbidity, mortality, and disability-adjusted life years (DALY) globally. The economic burden of CVD will be USD 1,044 billion by 2030. Furthermore, loss of productivity due to morbidity and mortality accounts for 11 and 15%, respectively, of total healthcare costs. Finally, the prevalence of DALY associated with CVD is 4,530/100,000 inhabitants (2).
Chagas disease is caused by the protozoan Trypanosoma cruzi, which is transmitted in the majority of cases by a kissing bug. After the initial infection, which terminates spontaneously in the overwhelming majority of cases, approximately 60% of patients are asymptomatic, showing only a positive serology. They are said to be in the indeterminate stage of the disease. However, about 20–30% of patients can develop chronic Chagas heart disease (CCHD) up to 20 years after initial infection (3).
CCHD manifests as AHF or CHF, thromboembolic phenomena, cardiac arrhythmias (3), and obstructive or non-obstructive coronary artery disease (CAD) (4). Therefore, the management of patients with CVD with superimposed COVID-19 in an area where Chagas disease is endemic is a colossal task because all diseases may manifest at the same time in the same patient. The potential cardiovascular complications for which we should be on the alert in the association of CCHD and COVID-19 are highlighted in Figure 1. On the other hand, Table 1 compares some particularities between these manifestations.
The aim of this paper is to provide suggestions for the management of CVD in patients with COVID-19 in areas where Chagas disease is endemic because coinfection—Chagas disease and SARS-CoV-2- may further aggravate morbidity, mortality, and DALY of patients with CVD.
The incidence of CAD is 176.3/100,000 inhabitants, whereas the prevalence of this disease is 2,270/100,000 inhabitants (2). CAD is the most important cause of death in patients with CVD (5), accounting for 38% of overall deaths (2). In addition, the DALY associated with CAD approaches 54% of the total DALYS caused by CVD (2). The economic burden of CAD is substantial, as it is associated with 27% of the total costs of CVD (2).
CAD is initially caused by endothelial dysfunction secondary to several comorbidities, including dyslipidemia, diabetes mellitus, systemic arterial hypertension, and smoking. At first, the low-density lipoprotein cholesterol fraction penetrates the intima of the dysfunctional endothelium; inflammatory cells are recruited to the vascular intima, and ingest low-density lipoprotein cholesterol fraction, thus forming the foam cells and inducing the proliferation of vascular smooth cells. The latter forms a fibrous cap and, ultimately, an established atherosclerotic plaque ensues. The narrowing luminal provoked by plaque or plaque rupture-associated thrombus superposition are the main mechanisms behind the clinical manifestations of CAD (5). However, thrombus and coronary spasm even in the angiographically proven normal epicardial coronary arteries are well other recognized causes of CAD.
Clinically, CAD manifests by stable angina pectoris or acute coronary syndrome—unstable angina pectoris, and acute myocardial infarction with elevated ST-segment (STEMI) or non-elevated ST-segment (non-STEMI). Nonetheless, many patients are found to have silent CAD, and they are at risk of death as well.
In patients with COVID-19, STEMI incidence may have increased by 35%, suggesting that SARS-CoV-2 infection itself might have caused such abnormality (6). In fact, SARS-CoV-2 recruits macrophages, which secrete collagenases in the endothelium, thus destroying the fibrous cap and leading to plaque rupture and intraluminal thrombosis (7). Therefore, SARS-CoV-2 infection may induce episodes of acute coronary syndrome in patients with CAD. In addition, a case of severe vasospasm has also been detected in a patient with COVID-19 (8). Furthermore, in patients with COVID-19 and STEMI, as compared with patients with STEMI without COVID-19, a higher proportion of multiple culprit thrombotic lesions, stent thrombosis, higher thrombus grade, and more importantly, lower myocardial blush grade following procedural intervention compatible with microthrombi have been observed (9).
Overall, angiographic studies show that about one-third of patients with STEMI and COVID-19 do not have underlying obstructive CAD. There are parallel autopsy studies in which cardiac thrombosis is found in 79% of patients with myocardial necrosis. However, underlying epicardial coronary thrombosis is detected in only 14% of patients; the remaining individuals are found to have microthrombi in myocardial capillaries, arterioles, and small muscular arteries (6). Collectively, such findings suggest that in patients with COVID-19 the mechanism behind CAD clinical manifestations may be different from that seen in non-COVID-19 patients, suggesting a major hypercoagulation state. This can be found in patients with chronic Chagas disease (CCD) as well.
Chest pain—atypical or typical—can be found in up to 15% of patients with CCD (10). Megaoesophagus has been associated with chest pain in patients with this condition (11). Nonetheless, in the absence of concomitant megaoesophagus, microvascular CAD seems to underlie chest pain in patients with CCHD (12), as obstructive epicardial CAD is rarely seen in patients with this condition (13, 14). Moreover, abnormalities in the tonus of epicardial coronary arteries have also been observed in patients with CCHD (15).
The presence of microvascular CAD with or without precordial chest pain can impact on the clinical course of patients with CCHD. In fact, acute coronary syndrome can be associated with this abnormality (10), and atypical chest pain can herald the appearance of acute myocardial infarction with non-obstructive coronary arteries in patients with such a condition (4). Moreover, microvascular disease-induced ischemia can progress to myocardial fibrosis and ventricular remodeling in patients with CCHD (16).
The pathophysiology of microvascular CAD in patients with CCD is not fully understood at this time; however, abnormalities in the microcirculation caused by vasospasm and platelet aggregation associated with inflammation and autonomic dysfunction have been postulated (17, 18). In this regard, a 3-month treatment with verapamil (80 mg/bid) and acetylsalicylic acid (100 mg/once a day) reduced the burden of ischemic episodes in patients with CCHD with microvascular CAD (19).
In patients with COVID-19 and CCHD, the potential for the occurrence of abnormalities in the epicardial and small coronary arteries does exist. Such abnormalities, according to all that have previously been mentioned, will more likely be manifested by acute coronary syndromes, either in patients with previously known CAD or in patients with unknown CAD. Therefore, such patients should undergo coronary angiography without any delay; if obstructive CAD is found, patients should be treated according to guidelines. However, if non-obstructive CAD is the diagnosis, calcium inhibitors and acetylsalicylic acid should be considered. In the case of chronic chest pain, myocardium scintigraphy or stress echocardiography should be performed. If ischemia is detected, patients should undergo coronary angiography; the absence of CAD prompts the diagnosis of microvascular angina, and calcium inhibitors and acetylsalicylic acid should be considered. If necessary, beta-blockers can be added to the treatment (20).
The incidence of stroke is about 143/100,000 inhabitants, whereas the prevalence of CEVD is 1,276/100,000 inhabitants. Stroke is the second most important cause of death in patients with CEVD, being responsible for 26% of all CVD deaths. CEVD is the second leading cause of disability, accounting for 27% of all DALYs associated with CVD. Moreover, CEVD impacts 20% on the overall costs on CVD (2).
Overall, about 38% of CEVD is associated with COVID-19 at the present time (6). In patients with COVID-19, the incidence of CEVD can be as high as 4.5% of the affected population (21–23). The prevalence of ischemic stroke (1.1%) is by far more common than haemorrhagic stroke (0.2%) in patients with COVID-19. A neuroimaging study shows that about 60% of cases of haemorrhagic stroke are not associated with systemic arterial hypertension (6). The risk of both ischemic stroke and cryptogenic stroke in COVID-19 patients is four times higher than that observed in non-COVID-19 patients.
The treatment of COVID-19-associated CEVD has become cumbersome during the pandemic. As observed in other diseases, the fear of exposure to COVID-19 in hospital settings probably explains the reduced 31% of reperfusion, and 22% of mechanical thrombectomies in patients with stroke (6). In-hospital mortality is higher (33 vs. 13%) in patients with COVID-19 associated stroke than in patients with stroke not associated with COVID-19 (24). This in-hospital mortality is three times higher than that seen in the pre-pandemic era despite similar door-to-needle time, time to thrombolysis with alteplase, door-to-reperfusion, and time of successful recanalization (25). Collectively, these findings suggest a more aggressive pathophysiological mechanism in patients with CEVD with COVID-19 than in those without. Therefore, the intensive management of patients with CEVD in the setting of COVID-19 is much necessary.
The prevalence of CEVD is 2% in patients with CCD (26). However, CEVD can affect about 11% of patients with CCHD (27), and up to 20% of a referral population of patients with this condition (28). In patients with CCD, CEVD manifests by cardioembolic stroke in up to 76% of cases; nonetheless, 12% of such patients are found to have occlusion of a major cerebral vessel, whereas 6% had occlusion of a small cerebral artery. Haemorrhagic stroke was not found. Risk factors for cardioembolic stroke are diabetes mellitus, young age, female sex, previous stroke, and a Souza score ≥ 3, whereas systemic arterial hypertension and dyslipidaemia are risk factors for non-cardioembolic stroke (29, 30).
CEVD may be the first clinical manifestation of CCHD in 3% to 33% of cases, and stroke recurrence can be found from 3 to 20% of patients (31). CHF, mural thrombus, left ventricular apical aneurysm, and cardiac arrhythmias [particularly atrial fibrillation] are independent predictors of CEVD in patients with CCD (32). In patients with mild to moderate CHF due to CCHD, left ventricular apical aneurysm and left ventricular thrombus are independent predictors of CEVD (28). Stroke is more frequently found in patients with CCHD with CHF than in those without, and the diagnosis of CCD is an independent predictor of stroke in patients with CHF (32).
Such clinical data are supported by a landmark morphological study carried out in 1,345 autopsied patients, which revealed cardiac thrombosis in 370 (28%) and thromboembolism in 638 (57%) patients. In that study, a cardiac thrombus was detected in the left ventricle in 121 (33%) patients; cardioembolic stroke occurred in 21% of patients (33).
Collectively, such data suggest that patients with COVID-19, CCD, and CEVD must be screened for cardioembolic stroke with echocardiography without any delay, and routine anticoagulation should be promptly administered if the diagnosis of cardioembolic stroke is made. In those patients in which cardioembolic stroke has been discarded or is a less likely diagnosis, the protocol for treating non-cardioembolic stroke should be immediately given.
The prevalence of CHF is approximately 1–2% of the general population. The incidence of CHF approaches 5/1,000 patients-year in an adult population (34). CHF may affect about 3% of patients with COVID-19 (35). Approximately 23% of hospitalized patients with COVID-19 develop AHF; the prevalence is still higher (49%) in those who died (36). In non-Chagas disease patients with cardiovascular risk factors and no previous history of CHF, a condition similar to that seen in patients in the indeterminate stage of CCD, AHF appears in about 20% of patients with superimposed COVID-19 (37).
Coinfection with SARS-CoV-2 has been associated with death in patients in the indeterminate phase of CCD (38). Therefore, in patients in the indeterminate stage of CCD who develop AHF the diagnosis of COVID-19 should be considered. In this regard, even patients with mild symptoms should be suspected of having COVID-19 coinfection because the SARS-CoV-2 strain that is grassing at the time of this writing (Omicron strain) can manifest a clinical profile different from that seen with the other SARS-CoV-2 strains (39).
AHF in patients with Chagas disease should promptly be diagnosed because the very poor prognosis associated with this condition. A study that included 767 patients, one in five with CCHD, showed that the proportion of cardiogenic shock at hospital admission was higher in Chagas disease than in non-Chagas disease patients. In addition, Chagas disease patients were more frequently in need of inotropic support or intra-aortic balloon pump, and had larger hearts than non-Chagas disease patients. Moreover, prognosis at 6 months were poorer in Chagas disease than in non-Chagas disease patients (40).
Non-Chagas disease patients with previous compensated CHF coinfected with SARS-CoV-2 are at higher risk (3 times more) of developing AHF than patients without; the mortality rate is 48% in patients with CHF and 19% in those without. De novo AHF can be detected in about 1.5% of patients with COVID-19. The withdrawal of beta-blockers, angiotensin converting enzyme inhibitors/angiotensin receptors blocks, and mineralocorticoid antagonists are the powerful independent predictors of mortality in such patients (41).
In non-Chagas disease patients with advanced CHF, Bocchi et al. reported that infection with SARS-CoV-2 worsens clinical status, as shown by the increased need for inotropic support or the introduction of a new inotropic drug for cardiac compensation. However, and not unexpectedly, mortality was dramatically increased at the 6-month follow-up. Interestingly, patients with advanced CHF with clinical deterioration following SARS-CoV-2 infection do not necessarily develop fever or other signs consistent with acute infection syndrome, thus suggesting that acute decompensation of CHF can be caused by COVID-19 in a region with high transmissibility of SARS-CoV-2 (42).
CHF can be found in about 14% of patients with CCD in a population-based cohort (43), and in up to 46% of patients in a referral center population (44). CHF is the main clinical manifestation of CCHD and the leading cause of CHF in endemic areas (45). The outcome of CHF secondary to CCHD is relentless, with an annual mortality of up to 20% (46). This high overall mortality may account, at least in part, for the poorer outcome observed in CHF due to CCHD in comparison with other causes of CHF (47–49). The reason for this dismal outcome may be ascribed to the aggressive ventricular remodeling observed in patients with this condition i.e., the association of mononuclear cell infiltrate and reparative fibrosis throughout the myocardium (3).
CHF secondary to CCHD is characterized by reduced left ventricular ejection fraction (LVEF); no cases of CHF due to left ventricular diastolic dysfunction have been observed. Therefore, the treatment of CHF secondary to CCHD is similar to that given to patients with non-Chagas disease heart failure, consisting of beta-blockers, angiotensin converting enzyme inhibitors/angiotensin receptor blocks, and mineralocorticoid antagonists (50). Ivabradine can also be of value to some Chagas disease patients with CHF (51). Reversion of the ventricular remodeling can occur in patients with CHF secondary to CCHD, but its significance on the prognosis of such patients is unknown (52). About 39% of patients with this condition do not tolerate the association of beta-blockers with angiotensin converting enzyme inhibitors/angiotensin receptor blocks at targeted doses because of symptomatic systemic arterial hypotension (53). This fact can also account for the unfavorable outcome of such patients.
Molina et al. screened 7,018 hospitalized patients with COVID-19 from a Brazilian Registry; 31 (0.4%) of them had CCD, and 124 patients with COVID-19 alone (matched by sex, age, systemic arterial hypertension, diabetes mellitus) served as controls. Compared with controls, patients with CCD and COVID-19 had a higher prevalence of AHF and atrial fibrillation, as well as lower levels of C-reactive protein. However, the proportion of patients who progressed to critical COVID-19 and overall mortality were similar in both groups (54). Although the small sample size precludes a firm conclusion regarding the impact of COVID-19 on patients with CCHD, it appears that coinfection—Chagas disease and COVID-19 can precipitate AHF in such patients. Furthermore, coinfection with SARS-CoV-2 can be associated with death in patients with CCHD (38).
This might be the consequence of viral infection itself, which could induce more myocardial inflammation, myocardial necrosis, and myocardial fibrosis. In fact, patients with Chagas cardiomyopathy show an increase in angiotensin converting enzyme 2 serum levels, which are associated with cardiac damage, ventricular remodeling, and unfavorable outcome in patients with CCD (55). Theoretically, therefore, infection with T. cruzi might facilitate SARS-CoV-2 binding to angiotensin converting enzyme 2 and start a vicious cycle. Another pathogenetic mechanism could be the overexpression of proinflammatory cytokines, especially interferon-37, which plays a central role in the pathogenesis of CCD (3). Heart endothelial inflammatory dysfunction, a condition observed in both diseases (56, 57), could also play a role in the aggravation of heart disease by provoking microvascular coronary disease, microvascular thrombosis, chronic ischemia, and ventricular remodeling.
In essence, patients in the indeterminate stage of CCD and compensated CHF due to CCHD should follow all restrictive measures in an attempt to avoid the infection of COVID-19 (58). In addition, those with compensated CHF should closely be followed-up to monitor adherence to guideline-recommended treatment because of the negative impact of the non-adherence to treatment on the prognosis of patients with this condition.
The epidemiology of pulmonary embolism (PE) is scanty. The prevalence of PE is estimated to be 60/100,000 inhabitants, while the prevalence of deep vein thrombosis, the major risk factor for PE, is 124/100,000 persons. The incidence of PE is estimated to approach 1/1,000 inhabitants yearly. PE is considered to be the third leading cause of CVD around the western world (59).
The prevalence of PE in patients with COVID-19 can vary from 8 to 16% (60, 61). The prevalence of PE is higher in patients aged < 65 years (20%) than in older patients (14%) (62). C-reactive protein, male gender, and time from symptom onset to hospitalization were positively associated with PE on multivariate analysis (60). Therefore, PE in patients with COVID-19 has been associated with coagulopathy and inflammation rather than with traditional deep-vein thromboembolism; this means that traditional anticoagulant risk factors may not be major determinants of PE in patients with COVID-19 (60).
Clinically, the clinical picture of PE in hospitalized patients with COVID-19 is characterized by the persistence or deterioration of respiratory symptoms, increased oxygen requirement, and a rapid increase in D-dimer levels. Approximately 28% of patients had deep vein thrombosis of the lower limbs, and 43% were on heparin prophylaxis. Chest computed tomography reveals massive pulmonary embolism or thrombosis of pulmonary arteries with segmental of subsegmental pattern; in some patients, subsegmental thrombosis of pulmonary arteries within lung opacities suggested local mechanisms rather than embolism at a distance. Overall mortality is around 1% (63).
Autopsy studies have shown that PE in patients with COVID-19 is associated with pulmonary intravascular coagulopathy leading to intrapulmonary thrombosis (64). In an African autopsy series, PE has been observed in 76% of patients who died suddenly in the community, but only 14% were found to have concomitant venous thrombosis (64). Therefore, a high level of suspicion is necessary to make the diagnosis of PE in patients with COVID-19.
Prophylaxis with anticoagulants in patients with COVID-19 is still evolving. In patients with COVID-19 admitted to the critical care unit, a randomized trial showed that the impact of standard-dose prophylactic anticoagulation with enoxaparin on vascular thrombosis, treatment with extracorporeal membrane oxygenation, or mortality was similar to that observed with intermediate- dose prophylactic anticoagulation. Therefore, intermediate-dose prophylactic anticoagulation appears not to be indicated to treat patients with severe COVID-19 (65). However, in non-critically hospitalized patients with COVID-19, treatment with a therapeutic dose of enoxaparin improved outcome (survival to hospital discharge, reduced need of respiratory or cardiovascular support at discharge) in comparison with usual prophylactic anticoagulation (66). In cases of PE presenting with cardiogenic shock, however, catheter-directed thrombolysis should be performed promptly, as it may be lifesaving (67).
A landmark autopsy study has revealed that right-sided cardiac thrombus can be detected in the right atrium in about 20% of patients with CCHD. PE can be diagnosed in 27% patients; a concomitant right-sided cardiac thrombus was found in 33% of such patients. This suggests that a substantial number of right-sided thrombosis progressed to PE (33).
Clinically, the diagnosis of Chagas disease is an independent predictor of PE (68), and right-sided cardiac thrombosis can be detected in about 58% of patients with Chagas disease heart failure (69). Nevertheless, right-sided cardiac thrombosis can be found in patients with right ventricular dysfunction without overt CHF (70). Therefore, the high frequency of PE in patients with CCHD appears to be the consequence of a high prevalence of right-sided cardiac thrombosis-induced PE rather than in situ thrombosis of the affected areas.
The mechanism behind cardiac thrombosis in patients with CCHD is obscure. Cardiac thrombosis might be the consequence of generalized vasculitis as part of a generalized chronic inflammation (71), culminating in platelet hyper aggregation (72), which has been detected even in patients in the indeterminate stage of chronic Chagas disease (73). Some variants of the human platelet polymorphism may be associated with CCHD severity, thus lending credence to the involvement of platelets on the thrombotic process in patients with CCD (74).
The SARS-CoV-2 binds to the angiotensin converting enzyme 2 receptor of vascular endothelial cells, including those placed in the heart microvasculature, thus provoking endotheliitis (36). Endothelial activation releases several proinflammatory cytokines, chemokines, von Willebrand and factor VIII, which lead to platelet aggregation and superimposed thrombosis (75). Placing patients with CCD into perspective with the aforementioned hypercoagulation condition system, the association of both states of hypercoagulability can pose all patients with CCD at risk of cardiac thrombosis and PE. Therefore, it would be desirable that all coinfected and symptomatic patients require close medical attention irrespective of the severity of the diseases.
Anticoagulation is the cornerstone of the treatment of patients with CCHD at high-risk for thromboembolism: those with atrial fibrillation, cardiac thrombosis, previous thromboembolism, apical left ventricular aneurysm, or a risk score > 4 points (76). When coinfected with SARS-CoV-2, non-etheless, current data do not support the widespread use of anticoagulation in the outpatient setting to reduce the major adverse cardiovascular or pulmonary consequences associated with symptomatic but clinically stable conditions in patients without the abnormalities mentioned earlier, even in individuals with high-levels of D-dimer.
In a general population aged 60 years or older, the prevalence of premature ventricular contractions, supraventricular premature contractions, and supraventricular tachycardia is 8.4, 7.7, and 9.0%, respectively (77). However, in a non-elderly population, the prevalence of premature ventricular contractions and supraventricular premature contractions is 0.22 and 0.44%, respectively (78). The incidence of such arrhythmias is about 1% yearly (77). The prevalence of atrial fibrillation in a general population is 4.1% (77), whereas the incidence of atrial fibrillation is 0.75/100,000 inhabitants (2) or approximately 1% yearly (77).
The prevalence of sustained ventricular tachycardia (SVT) is low, usually affecting about 0.2% of the general population (79), whereas the incidence of SVT is about 13 cases/100,000 inhabitants (80). The prevalence of ventricular fibrillation can be surmised to be 6,3 cases/100,000 inhabitants (81). The incidence of ventricular fibrillation is about 1.83 per 10,000 inhabitants (82).
Palpitations can be observed in about 7% of hospitalized patients with COVID-19 (83). The overall prevalence of arrhythmias in hospitalized patients with COVID-19 seems to be 17%, which is much higher in critical patients than in non-critical patients (44 vs. 7%) (84). Several abnormalities underlie the appearance of arrhythmias in hospitalized patients with COVID-19, such as hypoxia, acid-base imbalance, electrolyte disturbance, and myocardial ischemia.
In a cohort of 140 patients with COVID-19 that underwent telemetry monitoring during hospitalization, seven (5%) developed ventricular tachycardia/ventricular fibrillation; all of these patients had metabolic, hypoxemic, or high vasopressor need (85). Elevated troponin serum levels may also be a harbinger of malignant arrhythmias in patients with this condition (83). Therefore, malignant arrhythmia per se does not seem to be the etiology of death in patients with critical or severe COVID-19.
On the other hand, palpitations can be the reason by which patients with COVID-19 search for medical assistance in the absence of any other symptoms (86). Therefore, arrhythmias can appear not only in hospitalized patients, but also early in the course of COVID-19. The prevalence, incidence, and clinical characteristics of arrhythmias in the setting of ambulatory patients or in those with long COVID-19 are not known at the present.
Premature ventricular contractions can be found in the resting electrocardiogram of about 7% of a population-based cohort of patients with CCD, whereas complex premature ventricular contractions (Lown classification grade III or higher) can be observed in 2% of them (43). In contrast, 43% of patients with CCD with CHF are found to have premature ventricular contractions (47). At 24h-Holter monitoring, non-sustained ventricular tachycardia affects approximately 21% of patients with CCHD and approximately 50% of patients with reduced LVEF (87), which appears to be 30 times more frequent than in those with non-Chagas disease heart failure (88).
The importance of detecting non-sustained ventricular tachycardia is that about 10% of patients with CCHD with this arrythmia subsequently present SVT (89), which shows a typical electrocardiographic pattern (90) and may degenerate into ventricular fibrillation in 24% of cases (91). Although the severity of premature ventricular contractions depends on the degree of ventricular function in patients with this condition, SVT or ventricular fibrillation can be observed in approximately 14% of patients with preserved LVEF who have recovered from cardiac arrest (91).
The association of complex premature ventricular contractions and moderately reduced LVEF overshadows the prognosis of patients with CCHD; the same, however, does not occur when the LVEF is severely reduced (< 30%) or normal (92). In a population-based cohort, the presence of complex premature ventricular contractions reduced survival to virtually zero percent at a 10-year follow up and was an independent predictor of mortality in patients with this condition (43).
SCD is a continuous threat for patients with CCD. In a general population of patients with CCD, the prevalence of SCD is about 29%, being the second leading cause of death following death from irreversible heart failure (93). In patients with CCHD, about 46% can experience SCD (43); in those with reduced LVEF, SCD is the mode of death more frequently found in patients with mild to moderate CHF than in those with advanced stages of the syndrome (94).
The annual incidence of SCD in patients with CCHD disease can be roughly estimated from 5 to 6% in a general population (27, 94) to 22% in patients from a referral center (43). A morphological study carried out in the first decade of this century showed that CCD is still the cause of SCD in approximately 5% of cases (95). SVT degenerating into ventricular fibrillation seems to be the main cause of SCD (91). Although advanced atrioventricular block was an important cause of SCD in patients with CCD before the era of pacemaker implantation, advanced atrioventricular block can rarely cause SCD nowadays (96).
Macro reentry at the His-Purkinje system can be the cause of SCD in patients with CCHD, inasmuch as there has been an association between left ventricular apical aneurysm and left anterior fascicular block in patients with this condition (97). However, the peculiarities of the underlying microscopic lesions—extensive myocardial inflammation intermingled with reparative fibrosis throughout the myocardium—form the environment to multiple sites of micro re-entry to cause SCD in patients with CCHD (96). The scar-associated reentry phenomenon observed in patients with SVT who underwent endocardial mapping for potential SVT treatment (98) lends support to this assumption.
In fact, when there is no myocardial fibrosis on cardiac magnetic resonance imaging, there is no aborted SCD or shock therapy with implantable cardioverter-defibrillator in patients with CCHD (99). In contrast, the presence of scar on magnetic resonance imaging predicted cardiovascular death or the appearance of SVT in a 34-median follow-up of patients with this condition (100). Furthermore, the amount of myocardial fibrosis on magnetic resonance imaging is higher in patients with malignant ventricular arrhythmia than in those without (101). In addition, the presence of two or more segments of delayed enhancement further stratifies the risk of SVT occurrence in patients with CCHD and myocardial fibrosis at magnetic resonance imaging (102). Therefore, myocardial fibrosis appears to play a central role in the pathogenesis of SCD in patients with CCHD.
Based on the clinical characteristics and the pathophysiological mechanism of arrhythmias mentioned earlier, it is plausible to consider that the association of COVID-19 and CCHD can predispose patients to developing malignant ventricular arrhythmias. Therefore, such patients must be telemonitored continuously when hospitalized, and metabolic abnormalities must be promptly corrected. Furthermore, high-risk outpatients with coinfection should be considered for screening of non-sustained ventricular tachycardia at 24-h Holter monitoring and 2-D echocardiography to assess LVEF, whenever possible. When they have long COVID-19, these patients should also be considered for magnetic resonance imaging examination to detect potential myocardial fibrosis, which must be treated according to guidelines.
The association of COVID-19 and CCHD will probably overshadow the outcome of patients with CVD, i.e., those with CAD, CEVD, AHF/CHF, PE, and SCD. Despite the lack of guidelines on this subject, our suggestions may be of value to draw attention to doctors on the frontline of this deleterious association and to treat patients accordingly without any delay.
RBB, EB, and MN drafted the manuscript. RB, VI, and RF-D researched and discussed the manuscript. RBB and MN revised the final version. All authors have approved the manuscript in its final format.
This work was supported by the University of Ribeirão Preto.
The authors declare that the research was conducted in the absence of any commercial or financial relationships that could be construed as a potential conflict of interest.
All claims expressed in this article are solely those of the authors and do not necessarily represent those of their affiliated organizations, or those of the publisher, the editors and the reviewers. Any product that may be evaluated in this article, or claim that may be made by its manufacturer, is not guaranteed or endorsed by the publisher.
AHF, Acute heart failure; CAD, Coronary artery disease; CHF, Chronic heart failure; CCD, Chronic Chagas disease; CCHD, Chronic Chagas heart disease; CEVD, Cerebrovascular disease; CVD, Cardiovascular diseases; COVID-19, Coronavirus disease 2019; DALY, Disability-adjusted life years; LVEF, Left ventricular ejection fraction; PE, Pulmonary embolism; SARS-CoV-2, Severe acute respiratory syndrome coronavirus 2; SCD, Sudden cardiac death; STEMI, Acute myocardial infarction with elevated ST-segment; SVT, Sustained ventricular tachycardia.
2. Timmis A, Townsend N, Gale CP, Torbica A, Lettino M, Petersen SE, et al. European society of cardiology: cardiovascular disease statistics 2019. Eur Heart J. (2020) 41:12–85. doi: 10.1093/eurheartj/ehz859
3. Bocchi EA, Bestetti RB, Scanavacca MI, Cunha-Neto E, Issa VS. Chronic Chagas heart disease management: from etiology to cardiomyopathy treatment. J Am Coll Cardiol. (2017) 70:1510–24. doi: 10.1016/j.jacc.2017.08.004
4. Bestetti RB, Ariolli MT, do Carmo JL, Passos AD, Santos CR, Machado-Jr O, et al. Clinical characteristics of acute myocardial infarction in patients with Chagas disease. Int J Cardiol. (1992) 35:371–6. doi: 10.1016/0167-5273(92)90236-V
5. Dawson LP, Lum M, Nerleker N, Nicholls SJ, Layland J. Coronary atherosclerotic plaque regression. JACC. (2022) 79:66–82. doi: 10.1016/j.jacc.2021.10.035
6. Greenberg A, Pemmasani G. Cardiovascular and cerebrovascular complications with COVID-19. Cardiol Rev. (2021) 29:143–9. doi: 10.1097/CRD.0000000000000385
7. Shafeghat M, Aminorroaya A, Rezaei N. How stable ischemic heart disease leads to acute coronary syndrome in COVID-19? Acta Biom. (2021) 92:e2021512. doi: 10.23750/abm.v92i5.12013
8. Rivero F, Antuña P, Cuesta J, Alfonso F. Severe coronary spasm in a COVID-19 patient. Catheter Cardiovasc Interv. (2021) 97:e670–2. doi: 10.1002/ccd.29056
9. Choudry F, Hamshere SM, Rathod KS, Akhtar MM, Archbold RA, Gutmman OP, et al. High thrombus burden in patients with COVD-19 presenting with ST-segment elevation myocardial infarction. J Am Coll Cardiol. (2020) 76:1168–76. doi: 10.1016/j.jacc.2020.07.022
10. Bestetti RB, Restini CBA. Precordial chest pain in patients with chronic Chagas disease. Int J Cardiol. (2014) 176:309–14. doi: 10.1016/j.ijcard.2014.07.112
11. Lopes ER. Esophageal etiology of precordial pain in chagasic patients. Arq Bras Cardiol. (1995) 64:153–4.
12. Marin-Neto JA, Marzullo P, Marcassa C, Gallo-Jr L, Maciel BC, Bellina CR, et al. Myocardial perfusion abnormalities in chronic Chagas disease as detected by thallium-201 scintigraphy. Am J Cardiol. (1992) 69:780–4. doi: 10.1016/0002-9149(92)90505-S
13. Oliveira JSM, dos Santos JC, Muccillo G, Ferreira AL. Increased capacity of the coronary arteries in chronic Chagas heart disease: further support for the neurogenic pathogenesis concept. Am Heart J. (1985) 109:304–8. doi: 10.1016/0002-8703(85)90598-8
14. Carvalho G, Rassi S, Bastos JMDA, Câmara SSP. Asymptomatic coronary artery disease in chagasic patients with heart failure: prevalence and risks factor. Arq Bras Cardio. (2011) 97:408–12. doi: 10.1590/S0066-782X2011005000103
15. Torres FW, Acquatella H, Condado JA, Dinsmore R, Palacios I. Coronary vascular activity is abnormal in patients with chronic Chagas heart disease. Am Heart J. (1995) 129:995–1001. doi: 10.1016/0002-8703(95)90122-1
16. Hiss FC, Lascala TF, Maciel BC, Marin-Neto JA, Simões MV. Changes in myocardial perfusion correlate with deterioration of left ventricular systolic function in chronic Chagas cardiomyopathy. JACC Img. (2009) 2:164–72. doi: 10.1016/j.jcmg.2008.09.012
17. Rossi MA. Microvascular changes as a cause of chronic cardiomyopathy in Chagas disease. Am Heart J. (1990) 120:233–6. doi: 10.1016/0002-8703(90)90191-Y
18. Bestetti RB. Role of parasites in the pathogenesis of Chagas cardiomyopathy. Lancet. (1996) 347:913–4. doi: 10.1016/S0140-6736(96)91403-8
19. Pavão RB, Moreira HT, Pintya AO, Haddad JL, Badran AV, Lima-Filho MO, et al. Aspirin plus verapamil relieves angina and perfusion abnormalities in patients with coronary microvascular dysfunction and Chagas disease: a pilot non-randomized study. Rev Soc Med Trop. (2021) 54:e0181. doi: 10.1590/0037-8682-0181-2021
20. Bestetti RB, Sales-Neto VN, Pinto LZ, Soares EG, Muccillo G, Oliveira JSM. Effects of long-term metoprolol administration on the electrocardiogram of rats infected with T. cruzi. Cardiovasc Res. (1990) 24:521–7. doi: 10.1093/cvr/24.7.521
21. Harapan BN, Yoo HJ. Neurological symptoms, manifestations, and complications associated with severe acute respiratory syndrome coronavirus 2 (SARS-CoV-2) and coronavirus disease 19 (COVID-19). J Neurol. (2021) 268:3059–71. doi: 10.1007/s00415-021-10406-y
22. Siepmman T, Sedghi A, Simon E, Winzer S, Barlinn J, de With K, et al. Increased risk of acute stroke among patients with severe COVID-19: a multicentre study and meta-analysis. Eur J Neurol. (2021) 28:238–47. doi: 10.1111/ene.14535
23. Mendes A, Herrmann FR, Genton L, Serratrice C, Carrera E, Vargas MI, et al. Incidence, characteristics, and clinical relevance of acute stroke in old patients hospitalized with COVID-19. BMC Geriatr. (2021) 21:52. doi: 10.1186/s12877-021-02006-2
24. Dhamoon MS, Thaler A, Gururangan K, Kohli K, Sisniega D, Wheelwright D, et al. Acute cerebrovascular events with COVID-19 infection. Stroke. (2021) 52:48–56. doi: 10.1161/STROKEAHA.120.031668
25. Agarwal S, Scher E, Raghunath NR, Marolia D, Butnar M, Torres J, et al. Acute stroke care in a New York city comprehensive stoke center during the COVID-19 pandemic. J Stroke Cerebrovasc Dis. (2020) 29:105068. doi: 10.1016/j.jstrokecerebrovasdis.2020.105068
26. Bestetti R. Stroke in a hospital-derived cohort of patients with chronic Chagas disease. Acta Cardiol. (2000) 55:33–8. doi: 10.2143/AC.55.1.2005715
27. Espinosa R, Carrasco HA, Belandria F, Fuenmayor AM, Molina C, Gonzáles R, et al. Life expectancy analysis in patients with Chagas disease; prognostic after on decade (1973-1983). Int J Cardiol. (1985) 8:45–56. doi: 10.1016/0167-5273(85)90262-1
28. Nunes MCP, Kreuser LJ, Ribeiro AL, Souza GR, Costa HS, Botoni FA, et al. Prevalence and risk factors for embolic cerebrovascular events associated with Chagas heart disease. Glob Heart. (2015) 10:151–7. doi: 10.1016/j.gheart.2015.07.006
29. Bezerra RP, Alves MAM, Conforto AB, Rodrigues DLG, Silva GS. Etiological classification of stroke in patients with Chagas disease using TOAST, causative classification system TOAST, and ASCOD phenotyping. J Stroke Cerebrovasc Dis. (2017) 26:2864–9. doi: 10.1016/j.jstrokecerebrovasdis.2017.07.007
30. Montanaro VVA, Hora TF, Guerra AA, Silva GS, Bezerra RP, Oliveira-Filho J, et al. Artificial inteligence based- decision for the prediction of cardioembolism in patients with Chagas disease and ischemic stroke. J Stroke Cerebrovasc Dis. (2021) 30:106034. doi: 10.1016/j.jstrokecerebrovasdis.2021.106034
31. Carod-Artal J. Chagas disease and stroke. Lancet Neurol. (2010) 9:533–42. doi: 10.1016/S1474-4422(10)70042-9
32. Silva TC, Gonçalves BM, Pereira CB, Porto LM, Marques ME, Santos LB, et al. Chagas disease is an independent predictor of stroke and death in a cohort of heart failure patients. Int J Stroke. (2022) 17:180–8. doi: 10.1177/17474930211006284
33. Oliveira JSM, Correia de Araujo RL, Navarro MA, Muccillo G. Cardiac thrombosis and thromboembolism in chronic Chagas heart disease. Am J Cardiol. (1983) 52:147–51. doi: 10.1016/0002-9149(83)90085-1
34. McDonagh TA, Metra M, Adammo M, Gardner RS, Baumbach A, Böhm M, et al. 2021 ESC guidelines for the diagnosis and treatment of acute and chronic heart failure of the European Society of Cardiology. Eur Heart J. (2021) 42:3599–726. doi: 10.1093/eurheartj/ehab368
35. Escher F, Pietsch H, Aleshcheva G, Bock T, Baumeir C, Elsaesser A, et al. Detection of viral SASRS-CoV-2 genomes and histopathological changes in endomyocardial biopsies. ESC Heart Fail. (2020) 7:2440–7. doi: 10.1002/ehf2.12805
36. Liu F, Liu F, Wang L. COVID-19 and cardiovascular diseases. J Mol Cell Biol. (2021) 13:161–7. doi: 10.1093/jmcb/mjaa064
37. Sokoslki M, Trenson S, Sokolska JM, D'Amario D, Meyer P, Poku NK, et al. Heart failure in COVID-19: the multicentre, multinational PCHF-COVICAV registry. ESC Heart Fail. (2021) 8:4955–67. doi: 10.1002/ehf2.13549
38. Alberca RW, Yendo TM, Ramos YAl, Fernandes IG, Oliveira LM, Teixeira FME, et al. Case report: COVID-19 and Chagas disease in two coinfected patients. Am J Trop Med. (2020) 103:2353–6. doi: 10.4269/ajtmh.20-1185
39. Maslo C, Friedland R, Toubkin M, Laubscher A, Akaloo T, Kama BK. Characteristics and outcomes of hospitalized patients in South Africa during the COVID-19 omicron wave compared with previous waves. JAMA. (2022) 327:583–4. doi: 10.1001/jama.2021.24868
40. Issa VS, Ayoub-Ferreira SH, Schoroyens M, Chizzola P, Soares PR, Lage SHG, et al. The course of patients with Chagas heart disease during episodes of decompensated heart failure. ESC Heart Fail. (2021) 8:1460–71. doi: 10.1002/ehf2.13232
41. Rey JR, Codon JC, Rosillo SR, Iniesta AM, Castrejon SC, Clement IM, et al. Heart failure in COVID-19 patients: prevalence, incidence, and prognostic implications. Eur J Heart Fail. (2020) 22:2205–15. doi: 10.1002/ejhf.1990
42. Bocchi EA, Lima IGCV, Biselli B, Salemi VMC, Ayoub-Ferreira SM, Chizzolla PR, et al. Worsening of heart failure by coronavirus disease 2019 is associated with high mortality. ESC Heart Fail. (2021) 8:943–52. doi: 10.1002/ehf2.13199
43. Gonçalves JGF, Silva VJD, Borges MCC, Prata A, Correia D. Mortality indicators among chronic Chagas patients living in an endemic area. Int J Cardiol. (2010) 143:235–42. doi: 10.1016/j.ijcard.2009.02.011
44. Bestetti RB, Dalbo CMR, Arruda CA, Correia-Filho D, Freitas OC. Predictors of sudden cardiac death for patients with Chagas' disease: a hospital-derived cohort study. Cardiology. (1996) 87:481–7. doi: 10.1159/000177142
45. Nakazone MA, Otaviano AP, Machado MN, Bestetti RB. The use of the CALL risk score for predicting mortality in Brazilian heart failure patients. ESC Heart Fail. (2020) 7:2331–9. doi: 10.1002/ehf2.12770
46. Theodoropoulos TA, Bestetti RB, Otaviano AP, Cordeiro JA, Rodrigues VC, Silva AC. Predictors of all-cause mortality in chronic Chagas heart in the current era of heart failure therapy. Int J Cardiol. (2008) 128:22–9. doi: 10.1016/j.ijcard.2007.11.057
47. Bertolino ND, Villafanha DF, Cardinalli-Neto A, Cordeiro JÁ, Arcanjo MA, Theodoropoulos TAD, et al. Prognostic impact of Chagas disease in patients awaiting heart transplantation. J Heart Lung Transplant. (2010) 29:449–53. doi: 10.1016/j.healun.2009.10.014
48. Barbosa AP, Cardinalli-Neto A, Otaviano AP, Rocha BF, Bestetti RB. Comparison of outcome between Chagas cardiomyopathy and Idiopathic Dilated Cardiomyopathy. Arq Bras Cardiol. (2011) 97:517–25. doi: 10.1590/S0066-782X2011005000112
49. Shen L, Ramires F, Martinez F, Bodanese LC, Echeverria LE, Gomez EA, et al. Contemporary characteristics and outcomes in Chagasic heart failure compared with other nonischemic and ischemic cardiomyopathy. Circ Heart Fail. (2017) 10:e004361. doi: 10.1161/CIRCHEARTFAILURE.117.004361
50. Rhode LEP, Montera MW, Bocchi EA, Clausell NO, Albuquerque DC, Rassi S, et al. Diretriz Brasileira de Insuficiência Cardíaca Crônica e Aguda. Arq Bras Cardiol. (2018) 111:436–539. doi: 10.5935/abc.20180190
51. Bocchi EA, Rassi S, Guimarães GV. Safety profile and efficacy of ivabradine in heart failure due to Chagas heart disease: a post-hoc analysis of the SHIFT trial. ESC Heart Fail. (2018) 5:1072–3. doi: 10.1002/ehf2.12344
52. Nakazone MA, Otaviano AP, Machado MN, Bestetti RB. Does left ventricular reverse remodelling influence long-term outcomes in patients with Chagas cardiomyopathy? Cardiol J. (2022) 29:44–52. doi: 10.5603/CJ.a2020.0038
53. Bestetti RB, Furlan-Daniel RA. The treatment of chronic heart failure secondary to Chagas cardiomyopathy in the contemporary era. Int Cardiovasc Forum J. (2016) 7:19–25. doi: 10.17987/icfj.v7i0.217
54. Molina I, Marcolino MS, Pires MC, Ramos LEF, Silva RT, Guimarães MH Jr, et al. Chagas disease and SARS-CoV-2 coinfection does not lead to worse in-hospital outcomes. Sci Rep. (2021) 11:20289. doi: 10.1038/s41598-021-96825-3
55. Wang Y, Moreira MCV, Walther SH, Ebermann L, Schulteiss HP, Wessel N, et al. Plasma ACE2 activity is an independent prognostic marker in Chagas disease and equally potent as BNP. J Cardiac Fail. (2010) 16:157–63. doi: 10.1016/j.cardfail.2009.09.005
56. Dellalibera-Joviliano R, Bestetti RB, Lopes GS, Furlan-Daniel R, Lopes KC, Faria-Jr M, et al. Kinins and nitric oxide in patients with chronic Chagas disease and systemic arterial hypertension. Cardiovasc Pathol. (2020) 49:107257. doi: 10.1016/j.carpath.2020.107257
57. Varga Z, Flammer AJ, Steiger P, Haberecker M, Andermatt R, Zinkernagel AS, et al. Endothelial cell infection and endotheliitis in COVID-19. Lancet. (2020) 395:1417–8. doi: 10.1016/S0140-6736(20)30937-5
58. Bestetti RB, Furlan-Daniel R, Couto LB. Nonpharmaceutical public health interventions to curb the COVID-19 pandemic: a narrative review. J Infect Dev Ctries. (2022) 16:583–91. doi: 10.3855/jidc.14580
59. Belohlávek J, Dytrych V, Linhart A. Pulmonary embolism, part I. Epidemiology, risk factors and risk stratification, pathophysiology, clinical presentation, diagnosis and nonthrombotic pulmonary embolism. Exp Clin Cardiol. (2013) 18:129–38.
60. Fauvel C, Weizman O, Trimaille A, Mika D, Pommier T, Pace N, et al. Pulmonary embolism in COVID-19 patients: a French multicentre cohort study. Eur Heart J. (2020) 41:3058–68. doi: 10.1093/eurheartj/ehaa500
61. Desai R, Gandhi Z, Singh M, Sachdeva S, Manaktala P, Savani S, et al. Prevalence of pulmonary embolism in COVID-19: a pooled analysis. SN Compr Clin Med. (2020) 2:2722–5. doi: 10.1007/s42399-020-00605-5
62. Faggiano P, Bonelli A, Paris S, Milesi G, Bisegna S, Bernardi S, et al. Acute pulmonary embolism in COVID-19 disease: preliminary report on seven patients. Int J Cardiol. (2020) 313:129–31. doi: 10.1016/j.ijcard.2020.04.028
63. Carsana L, Sonzogni A, Nasr A, Rossi R, Pellegrinelli A, Zerbi P, et al. Pulmonary post-mortem findings in a series of COVID-19 cases from Northern Italy: a two centre descriptive studies. Lancet Infect Dis. (2020) 20:1135–40. doi: 10.1016/S1473-3099(20)30434-5
64. Mucheleng'anga LA, Telendiy V, Hamukale A, Shibemba Al, Zumla A, Himwaze CM. COVID-10 and sudden community deaths in Lusaka, Zambia, Africa – A medico-legal whole-body autopsy case series. Int J Infect Dis. (2021) 109:160–7. doi: 10.1016/j.ijid.2021.07.001
65. Sadeghipour P, Talasaz AH, Rashidi F, Kashani BS, Beigmohammadi MT, Farrokhpour M, et al. Effect of intermediate-dose vs standard-dose prophylactic anticoagulation on thrombotic events, extracorporeal membrane oxygenation treatment, or mortality among patients with COVID-19 admitted to the intensive care unit. JAMA. (2021) 325:1620–30. doi: 10.1001/jama.2021.4152
66. Lawler PR, Goligher EC, Berger JS, Neal MD, McVerry BJ, Nicolau JC, et al. Therapeutic anticoagulation with heparin in noncritically ill patients with COVID-19. N Engl J Med. (2021) 385:790–802. doi: 10.1056/NEJMoa2105911
67. Pendower L, Benedetti G, Breen K, Karunanithy N. Catheter-directed thrombolysis to treat acute pulmonary thrombosis in a patient with COVID-19 pneumonia. BMJ Case rep. (2020) 13:e237046. doi: 10.1136/bcr-2020-237046
68. Macedo IS, Dinardi LFL, Pereira TV, Almeida LKR, Barbosa TS, Benvenuti LA, et al. Thromboembolic findings in patients with heart failure at autopsy. Cardiovasc Pathol. (2018) 35:23–8. doi: 10.1016/j.carpath.2018.04.004
69. Fernandez EA, Barretto ACP, Ianni BM et al. Cardiac thrombosis and embolism in patients having died of chronic Chagas cardiopathy. Arq Bras Cardiol. (1989) 52:189–92.
70. Bestetti RB, Corbucci HAR, Cardinalli-Neto Augusto. Massive right-sided cardiac thrombosis in Chagas heart disease without left ventricular dysfunction. Acta Cardiol. (2011) 66:67–9. doi: 10.1080/AC.66.1.2064969
71. Melo LMMP, Souza GEC, Valim LR, Moreira LFP, Damico EA, Rocha TRB, et al. Study of pro-thrombotic and pro-inflammatory factors in Chagas cardiomyopathy. Arq Bra Cardiol. (2010) 95:655–62. doi: 10.1590/S0066-782X2010005000146
72. Mayoral LPC, Huerta MTH, Garcia DP, Barritault D, Zenteno E, Navarro LMS, et al. Immunothrombotic dysregulation in Chagas disease and COVID-19: a comparative study of anticoagulation. Mol Cell Bioch. (2021) 476:3815–25. doi: 10.1007/s11010-021-04204-3
73. Castillejos FR, Mayoral LPC, Andrade GM, Huerta MTH, Canseco SP, Cruz RM, et al. A preliminary study of platelet hyperactivity in the chronic indeterminate phase of Chagas disease. Trop Biomed. (2018) 35:678–83.
74. Correa DEC, Ayo CM, Visentainer JEL, Albuquerque EPA, Reis PG, Mattos CCS et al. Human platelet antigen polymorphisms and the risk of chronic Chagas disease cardiomyopathy. Platelets. (2020) 31:272–5. doi: 10.1080/09537104.2019.1667496
75. Zhang J, Tecson KM, McCullough PA. Endothelial dysfunction contributes to COVID-19- associated vascular inflammation and coagulopathy. Rev Cardiovasc Med. (2020) 21:315–9. doi: 10.31083/j.rcm.2020.03.126
76. Dias JCP, Ramos-Jr AN, Gontijo ED, Luquetti A, Yasuda MAS, Coura JR, et al. Second Brazilian Consensus on Chagas disease. Epidemiol Serv Saúde. (2016) 25:7–86. doi: 10.5123/S1679-49742016000500002
77. Lindberg T, Wimo A, Elmsthäl S, Qiu C, Bohman D, Berglubnd JS. Prevalence and incidence of atrial fibrillation and other arrhythmias in the general older population: findings from the Swedish national study on aging and care. Gerontol Geriat Med. (2019) 5:1–8. doi: 10.1177/2333721419859687
78. Khurshid H, Choi SH, Weng LC, Wang EY, Trinquart L, Benjamin EJ, et al. Frequency of cardiac rhythm abnormalities inn a half million adults. Circ Arrhyth Electrophysiol. (2018) 11:e006273. doi: 10.1161/CIRCEP.118.006273
79. Solomon MD, Yang J, Hung J, Sung SH, Livingston ML, Sarlas G, et al. Incidence and timing of potentially high-risk arrhythmias detected through long-term continuous ambulatory electrocardiographic monitoring. BMC Cardiovasc Dis. (2016) 16:35. doi: 10.1186/s12872-016-0210-x
80. Sirichand S, Killu AK, Padmanabhan D, Hodge DO, Chamberlain AM, Brady PA, et al. Incidence of idiopathic ventricular arrhythmias: a population-based study. Circ Arrhythm Electrophysiol. (2017) 10:e004662. doi: 10.1161/CIRCEP.116.004662
81. Chugh SS, Reinier K, Teodorescu C, Evanado E, Kehr E, Al-Samara M, et al. Epidemiology of sudden cardiac death: clinical and research implications. Prog Cardiovasc Dis. (2008) 51:213–28. doi: 10.1016/j.pcad.2008.06.003
82. Tseng WC, Wu MH, Chen HC, Kao FY, Huang SK. Ventricular fibrillation in a general population. Circ J. (2016) 80:2310–6. doi: 10.1253/circj.CJ-16-0602
83. Liu K, Fang YY, Liu W, Wang MF, Ma JP, Xiao W, et al. Clinical characteristics of novel coronavirus cases in tertiary hospitals in Hubei province. Chin Med J. (2020) 133:1025–31. doi: 10.1097/CM9.0000000000000744
84. Wang D, Hu B, Hu C, Zhu F, Liu X, Zhang J, et al. Clinical characteristics of 138 hospitalized patients with 2019 novel coronavirus-infected pneumonia in Wuhan, China. JAMA. (2020) 323:1061–9. doi: 10.1001/jama.2020.1585
85. Turagam MK, Musikantow D, Goldman ME, Marcus AB, Chu E, Shivamurthy P, et al. Malignant arrhythmias in patients with COVID-19. Circ Arrhythm Electrophysiol. (2020) 13:e008920. doi: 10.1161/CIRCEP.120.008920
86. Zheng YY, Ma YT, Zhang JY, Xie X. COVID-19 and the cardiovascular system. Nat Rev Cardiol. (2020) 17:259–260. doi: 10.1038/s41569-020-0360-5
87. Carrasco HA, Guerrero L, Parada H, Molina C, Vegas E, Chuecos R. Ventricular arrhythmias and left ventricular myocardial function in chronic chagasic patients. Int J Cardiol. (1990) 28:35–41. doi: 10.1016/0167-5273(90)90006-Q
88. Rassi-Jr A, Rassi AG, Rassi SG, Rassi-Jr L, Rassi A. Arritmias ventriculares na doença de Chagas. Particularidades diagnósticas, prognósticas e terapêuticas. Arq Bras Cardiol. (1995) 65:377–87.
89. Silva RMFL, Tavora MZP, Gondin FAA, Mehta N, Hara VM, de Paola AAV. Predictive value of clinical and electrophysiological variables in patients with chronic chagasic cardiomyopathy and nonsustained ventricular tachycardia. Arq Bras Cardiol. (2000) 75:41–7. doi: 10.1590/S0066-782X2000000700004
90. Bestetti RB, Santos CR, Machado-Jr O, Ariolli MT, Carmo JL, Costa NK, et al. Clinical profile of patients with Chagas disease before and during sustained ventricular tachycardia. Int J Cardiol. (1990) 29:39–46. doi: 10.1016/0167-5273(90)90271-6
91. Cardinalli-Neto A, Grecco OT, Bestetti RB. Automatic implantable cardioverter-defibrillators in Chagas heart disease patients with malignant ventricular arrhythmias. PACE. (2006) 29:467–70. doi: 10.1111/j.1540-8159.2006.00377.x
92. Carrasco HA, Parada H, Guerrero L, Duque M, Duran D, Molina C. Prognostic implications of clinical, electrocardiographic and hemodynamic findings in chronic Chagas disease. Int J Cardiol. (1994) 43:27–38. doi: 10.1016/0167-5273(94)90087-6
93. Manzullo EC, Chuit R. Risk of death due to chronic chagasic cardiopathy. Mem Inst Oswaldo Cruz. (1999) 94 (Suppl 1):317–20. doi: 10.1590/S0074-02761999000700060
94. Ayoub-Ferreira Mangini S, Issa VS, Cruz FD, Bacal F, Guimarâes GV, et al. Mode of death of non-Chagas heart disease: comparison with other etiologies. A subanalysis of the REMADHE trial. PLoS Neglec Trop Dis. (2013) 7:e2176. doi: 10.1371/journal.pntd.0002176
95. Braggion-Santos MF, Volpe GJ, Pazin-Filho A, Maciel BC, Marin-Neto JA, Schimidt A. Sudden cardiac death in Brazil: a community-based autopsy series (2006-2010). Arq Bras Cardiol. (2015) 104:120–7. doi: 10.5935/abc.20140178
96. Bestetti RB, Cardinalli-Neto A. Sudden cardiac death in Chagas heart disease in the contemporary era. Int J Cardiol. (2008) 131:9–17. doi: 10.1016/j.ijcard.2008.05.024
97. de Paola AAV, Horowitz LN, Miyamoto F, Pinheiro P, Ferreira DF, Terzian N, et al. Angiographic and electrophysiologic substrates of ventricular tachycardia in chronic chagasic myocarditis. Am J Cardiol. (1990) 65:360–3. doi: 10.1016/0002-9149(90)90302-H
98. Sarabanda AVL, Sosa E, Simões MV, Figueiredo GL, Pyntia AO, Marin-Neto JA. Ventricular tachycardia in Chagas disease: a comparison of clinical, angiographic, electrophysiological and myocardial perfusion disturbances between patients presenting with either sustained or nonsustained forms. Int J Cardiol. (2005) 102:9–19. doi: 10.1016/j.ijcard.2004.03.087
99. Senra T, Ianni BM, Costa ACP, Mady C, Martinelli-Filho M, Kalil-Filho R, et al. Long-term prognostic value of myocardial fibrosis in patients with Chagas cardiomyopathy. JACC. (2018) 72:2577–87. doi: 10.1016/j.jacc.2018.08.2195
100. Volpe GJ, Moreira HT, Trad HS, Wu KC, Braggion-Santos MF, Santos MK, et al. Left ventricular scar and prognosis in chronic Chagas cardiomyopathy. JACC. (2018) 72:2567–76. doi: 10.1016/j.jacc.2018.09.035
101. Rochitte CE, Oliveira PF, Andrade JA, Ianni BM, Parga JR, Avila LF, et al. Myocardial delayed enhancement by magnetic resonance imaging in patients with Chagas disease: a marker of disease severity. JACC. (2005) 46:1553–8. doi: 10.1016/j.jacc.2005.06.067
Keywords: COVID-19, Chagas disease, cardiovascular disease, SARS-CoV-2, Trypanosoma cruzi
Citation: Bestetti RB, Bocchi EA, Bestetti R, Issa VS, Furlan-Daniel RA and Nakazone MA (2022) Management of Cardiovascular Disease in Patients With COVID-19 and Chronic Chagas Disease: Implications to Prevent a Scourge Still Larger. Front. Med. 9:910388. doi: 10.3389/fmed.2022.910388
Received: 01 April 2022; Accepted: 03 June 2022;
Published: 29 June 2022.
Edited by:
Sumera Zaib, University of Central Punjab, PakistanReviewed by:
Julio A. Urbina, Instituto Venezolano de Investigaciones Cientificas, VenezuelaCopyright © 2022 Bestetti, Bocchi, Bestetti, Issa, Furlan-Daniel and Nakazone. This is an open-access article distributed under the terms of the Creative Commons Attribution License (CC BY). The use, distribution or reproduction in other forums is permitted, provided the original author(s) and the copyright owner(s) are credited and that the original publication in this journal is cited, in accordance with accepted academic practice. No use, distribution or reproduction is permitted which does not comply with these terms.
*Correspondence: Reinaldo Bulgarelli Bestetti, cmJlc3RldHRpNDRAZ21haWwuY29t
Disclaimer: All claims expressed in this article are solely those of the authors and do not necessarily represent those of their affiliated organizations, or those of the publisher, the editors and the reviewers. Any product that may be evaluated in this article or claim that may be made by its manufacturer is not guaranteed or endorsed by the publisher.
Research integrity at Frontiers
Learn more about the work of our research integrity team to safeguard the quality of each article we publish.