- 1Department of Obstetrics, Ziekenhuis Oost Limburg, Genk, Belgium
- 2Department of Physiology, Hasselt University, Hasselt, Belgium
- 3Centre for Fetal Care, Queen Charlotte’s and Chelsea Hospital, Imperial College Healthcare NHS Trust, London, United Kingdom
- 4Department of Metabolism, Digestion and Reproduction, Institute for Reproductive and Developmental Biology, Imperial College London, London, United Kingdom
- 5Department of Development and Regeneration, KU Leuven, Leuven, Belgium
- 6Centre for Fetal Care, Queen Charlotte’s and Chelsea Hospital, London, United Kingdom
This narrative review summarizes current evidence on the association between maternal low volume circulation and poor fetal growth. Though much work has been devoted to the study of cardiac output and peripheral vascular resistance, a low intravascular volume may explain why high vascular resistance causes hypertension in women with preeclampsia (PE) that is associated with fetal growth restriction (FGR) and, at the same time, presents with normotension in FGR itself. Normotensive women with small for gestational age babies show normal gestational blood volume expansion superimposed upon a constitutionally low intravascular volume. Early onset preeclampsia (EPE; occurring before 32 weeks) is commonly associated with FGR, and poor plasma volume expandability may already be present before conception, thus preceding gestational volume expansion. Experimentally induced low plasma volume in rodents predisposes to poor fetal growth and interventions that enhance plasma volume expansion in FGR have shown beneficial effects on intrauterine fetal condition, prolongation of gestation and birth weight. This review makes the case for elevating the maternal intravascular volume with physical exercise with or without Nitric Oxide Donors in FGR and EPE, and evaluating its role as a potential target for prevention and/or management of these conditions.
Introduction
For the past 50 years, defective placentation and inadequate adaptation of uterine spiral arteries have been considered the key role players in the so-called obstetrical syndromes: preeclampsia (PE), fetal growth restriction (FGR), preterm labor, preterm premature rupture of the membranes, late spontaneous abortion, and abruptio placentae (1). Placental malperfusion and subsequent oxidative stress are associated with the impairment of both maternal and fetal systemic physiologic functions and, eventually, maternal disease, and/or fetal distress (2). In recent years, however, the focus of research into the origins of gestational hypertensive disorders with or without FGR has shifted from the placenta to maternal cardiovascular dysfunction, present before conception or developing during the earliest stages of placentation (3, 4). The interplay between maternal hemodynamics and the placentation process explains the two hemodynamic phenotypes of PE – one with high cardiac output (CO) and low vascular resistance and another with low CO and high vascular resistance – (5, 6), as well as FGR (7), and also links maternal low CO to fetal increased umbilical and reduced cerebral Doppler impedance (8). Next to this, suboptimal plasma volume expansion is considered an intrinsic pathophysiologic feature of PE with FGR (9), and maternal normotensive low volume circulation has been associated with neonatal birthweight of <10th percentile (10). The feasibility and clinical relevance of non-invasive assessment of maternal body water volumes, in association with cardiovascular assessments in both latent and symptomatic stages of PE and/or FGR, have been reported (11, 12), offering an opportunity for volume expansion strategies as potential management options in the prevention and treatment of poor fetal growth.
This narrative literature review aimed to summarize reported evidence on the association between poor fetal growth and low maternal circulating volume as a constitutional predisposing condition in normotensive FGR or as a consequence of suboptimal volume expansion in PE with FGR. The methodology was performed according to Sandra’s principles (13) using the following keywords (alone or in combination): FGR, intrauterine growth restriction, poor fetal growth, early onset preeclampsia (EPE), gestational hypertensive disorders, maternal hemodynamics, maternal cardiovascular function, CO, total vascular resistance (TVR), plasma volume, body water volume, maternal venous Doppler, fetal Doppler, venous hemodynamics, and volume regulation.
Maternal Hemodynamics and Fetal Growth
Birth weight relates to many variables, such as parental anthropometrics (height, weight, BMI), race, gender, gestational age, diet, drinking habits and substance (ab)use, and medical and obstetric history (14). Poor fetal growth and, in particular, FGR may relate to a chronic state of intrauterine hypoxia resulting from preplacental, placental (e.g., abruption), and postplacental causes (e.g., cord insertion, fetal genetics; 15). Known causes of preplacental hypoxia are not only pathologic conditions, such as chronic maternal cardiovascular, pulmonary or systemic disease, anemia, and infections, but also physiological determinants, such as high altitude and maternal CO.
Cardiac output is linked mathematically to mean arterial blood pressure (MAP) and TVR according to the hemodynamics’ variant of Ohm’s law
where CO is the product of stroke volume (SV) and heart rate (HR; 16). In the preconception period, low maternal CO, mostly in combination with increased TVR, predisposes pregnant women to gestational complications, such as PE with or without FGR (3), and the lowest CO values are observed in normotensive FGR (17). In an uncomplicated pregnancy, there is a positive correlation between CO change from preconception to mid-gestation and neonatal birth weight (18). During pregnancy from the first trimester onward, maternal CO is directly related to singleton birth weight (19–21), particularly at advanced maternal age (22), with parity (23), and multiple pregnancies (24), whereas there is an inverse relation with altitude (25) and gestational hypertensive disorders (26, 27). Figure 1 shows the gestational trends of CO and TVR as measured by the bioimpedance technology: contrary to pregnancies eventually developing PE, the evolution is similar in FGR and uncomplicated pregnancy, however, at lower CO and higher TVR (10, 11, 28). This observation has also been reported by others (29) and linked to a condition of low CO that is already present before conception (17). Low CO in FGR pregnancies mainly results from low SV (30, 31) and to a lesser degree from low HR (32, 33). Throughout an uncomplicated pregnancy, the fraction of CO deviated to the uterus doubles from 6 to 12% (34) and is achieved by an increase of (distal) internal iliac artery impedance in concert with a reduction of uterine artery impedance (35). Uterine artery blood volume flow positively correlates with birth weight (36, 37), reduces from maternal upright to the supine position with poor response to supine exercise (38), and is lower in FGR than in normal fetal growth (39). These observations all indicate that maternal cardiovascular function and uterine arterial blood supply strongly contribute to fetal growth and neonatal birth weight.
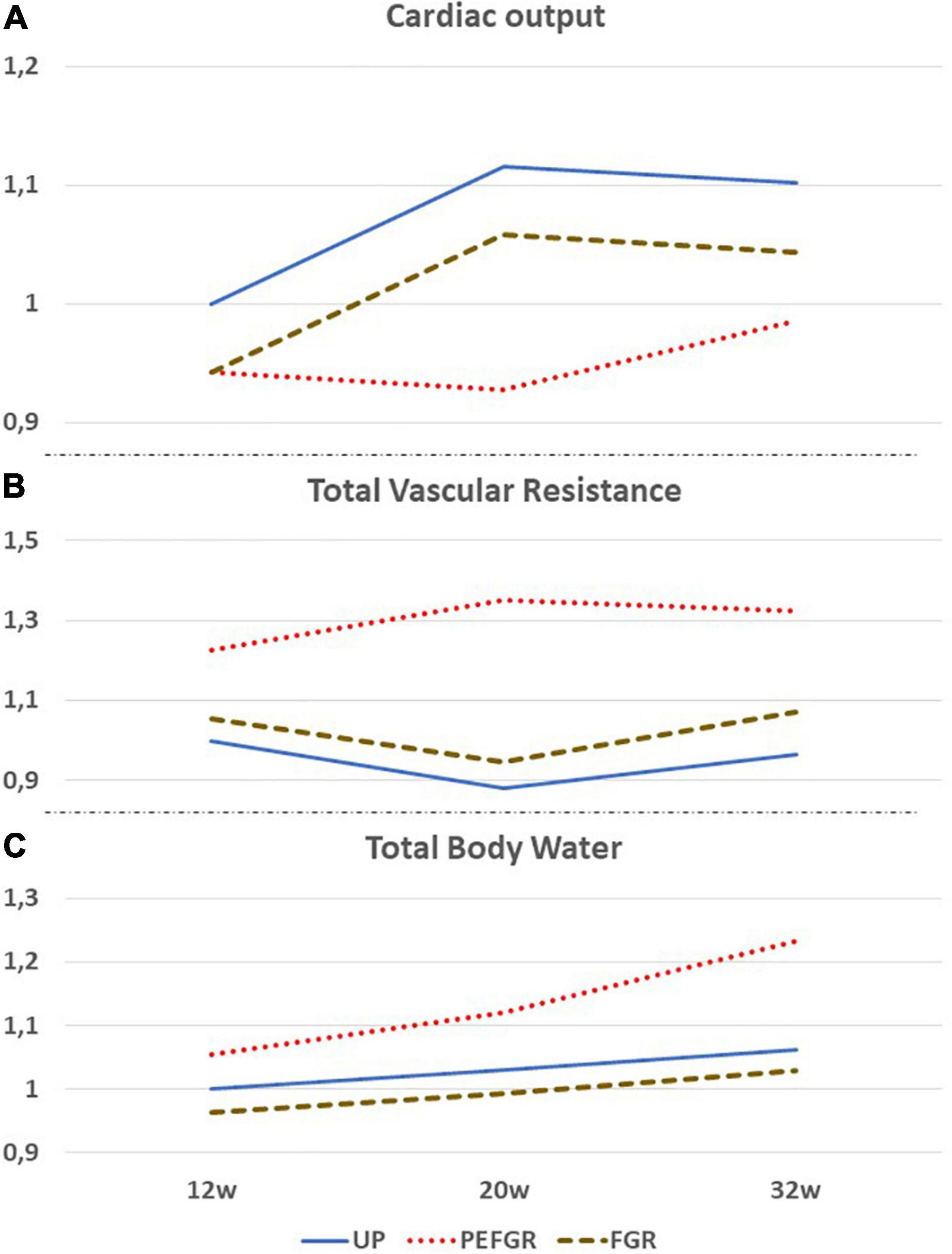
Figure 1. Comparative gestational evolution of maternal cardiac output (A), total vascular resistance (B), and total body water volume (C), as measured by the bioimpedance technology between uncomplicated pregnancies (UP), normotensive fetal growth restriction (FGR), and preeclampsia with FGR (PEFGR). Values are expressed as multiples of mean first trimester values in UP. Figures adapted from 10, 11, and 28.
Recently, it was shown that the venous compartment and body water volume load are also involved in the regulation of fetal growth (40). Next to its metabolic functions, the liver serves as a hemodynamic organ, where a large fraction of the unstressed blood volume is stored, as a reserve volume available for an instant increase of CO by sympathetic nervous-stimulated drainage of hepatic veins in the inferior vena cava. Inverse correlations have been reported between maternal CO and birth weight, on the one hand, and hepatic venous Doppler impedance index, on the other hand (40). Next to this, in normotensive women giving birth to neonates small for gestational age (SGA), low CO was associated with low body water volume and high Doppler impedance of uterine artery and hepatic veins, all of which are indicators of a low volume circulation (10; Figure 1). These observations are in line with reported impaired expansion of maternal plasma volume in FGR pregnancies in normal (41), small, and lean (42) women. Here, it is important to mention that the reduced plasma volume, as compared to normal pregnancy, precedes a suboptimal rise of the volume regulating hormones aldosterone, progesterone, and estrogens (43). The presence of a low intravascular volume before or during pregnancy can explain why low CO and high TVR can present with normotension and why a normal gestational plasma volume expansion fails to achieve normal values of maternal CO. Contrary to normotensive FGR, EPE – which mostly is associated with poor fetal growth – low CO and high TVR present with high body water volumes already from the first trimester onwards, suggesting a different pathophysiologic background mechanism (11; Figure 1).
Pathways of Maternal Low Volume Circulation in Early Onset Preeclampsia and Fetal Growth Restriction
In an uncomplicated pregnancy, maternal plasma volume expansion is triggered by a primary fall in systemic vascular resistance (44). The subsequent state of intravascular underfilling causes a reduction of cardiac afterload, which, in turn, is responsible for a rise in SV (45). Together with a rising HR, increased SV induces a 25% increase in CO at 6 weeks of gestation (17). Due to anatomic and physiologic properties of the high volume/low resistance of pulmonary circulation, increased CO results in a reduction of pulmonary vascular resistance via capillary recruitment and distention (46). In pregnant women, increased CO and the associated enlarged pulmonary capillary bed are responsible for a rise of intrathoracic water as measured by bioimpedance technology as early as 6–7 weeks of gestation (47, 48).
Meanwhile, SV and left atrial dimensions continue to rise in normal pregnancies; this is not true for pregnancies destined to develop severely impaired fetal growth (45) and/or EPE (33). As shown in Figure 1, CO is persistently lower than normal in normotensive FGR despite a normal gestational rise. In EPE, however, CO fails to rise after 8 weeks of gestation (17), and this is associated with the echocardiographic reduced left atrial area and fractional area change (33), as well as increased left ventricular end-systolic and end-diastolic volumes (49). This pathophysiologic condition is responsible for the increase of left atrial filling pressure, with the subsequent retrograde rise of pulmonary venous pressure and capillary hydrostatic pressure, resulting in exudation of intravascular fluids similar to the mechanisms observed in heart failure (50). A rise of pulmonary interstitial fluids before the clinical presentation of symptomatic pulmonary edema is a known phenomenon in chronic heart failure, and the detection of this condition by bioimpedance technology is useful to predict and timely counteract the severity of pulmonary edema (50, 51). As shown in Figure 1, the same mechanism is likely to occur in early preeclampsia (EPE): an asymptomatic exudation of intravascular fluids in the pulmonary interstitium can precede the development of edema elsewhere in the female body and explains the combination of increased total body water volume without the concomitant rise of CO in EPE. The constant exchange between intravascular and interstitial volumes is a normal physiologic function of the microcirculation and indicates that abnormal changes in plasma volume cannot be interpreted correctly without considering changes in other body volume compartments. This phenomenon is illustrated visually in Figure 2. The nature of this pathophysiologic pathway is in line with the reported increased serum concentrations of atrial natriuretic peptide (52) and copeptin/vasopressin in PE (53) and with the impaired expansion of maternal plasma volume in EPE (9). The early gestational onset of this phenomenon is also supported by the shallow, but significant, rise of serum hemoglobin concentrations and hematocrit in the first trimester of pregnancies destined to develop EPE (31, 54, 55) but not in those eventually leading to FGR (56). As explained above and illustrated in Figure 1, FGR pregnancies show a normal rise of plasma volume and total body water, superimposed upon constitutionally low body water already present before conception.
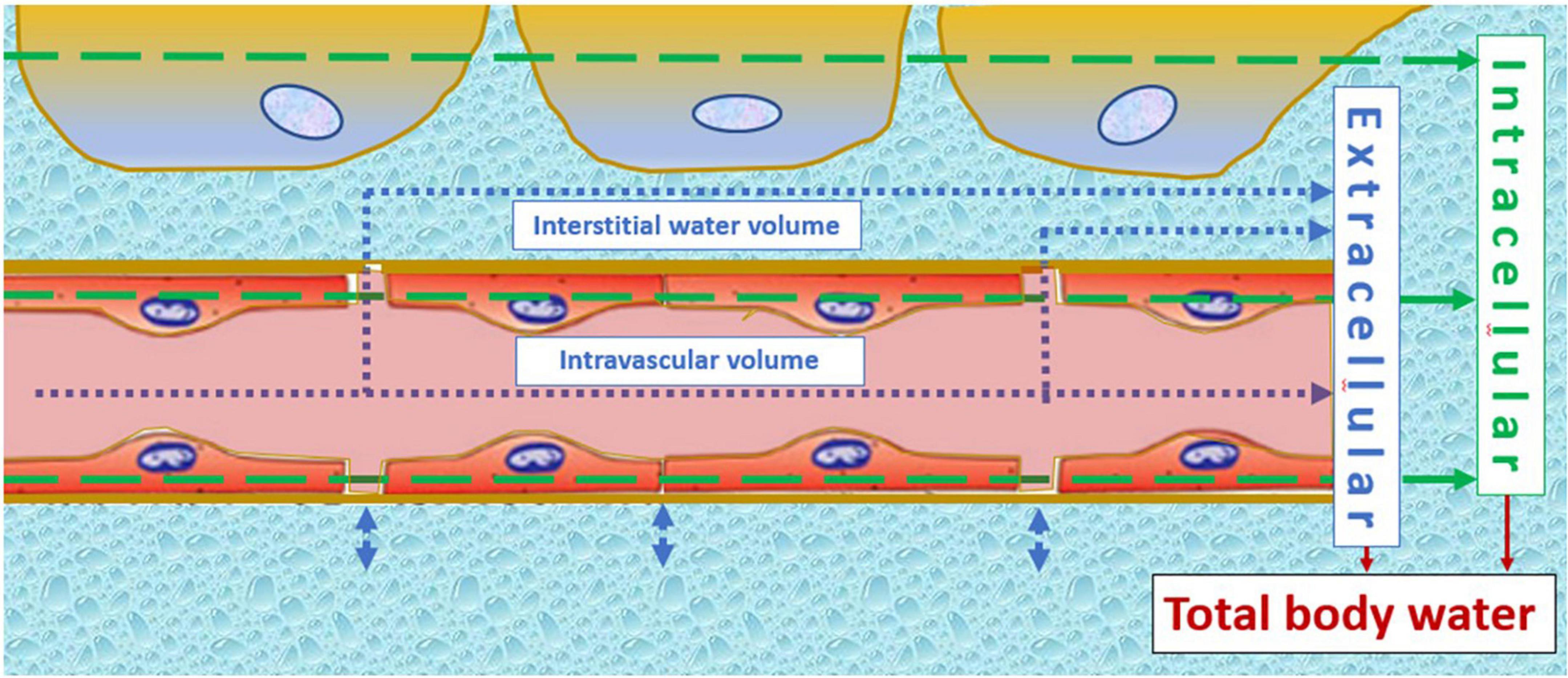
Figure 2. Illustration of the constant fluid exchange between intravascular and interstitial volumes at the level of the microcirculation. This interconnectivity indicates that plasma volume changes cannot be interpreted without knowledge of interstitial water volume, or indirectly via measurement of total body water volume. Normotensive fetal growth restriction relates to a constitutionally low intravascular volume, remaining lower in uncomplicated pregnancies despite normal gestational volume expansion. Early onset preeclampsia, on the other hand, is a state of increased adrenergic activation (155), shifting blood from the venous capacitance bed into the circulation and despite this, cardiac output fails to rise. This can only be explained by a shift from the intravascular compartment to the interstitium, which is in line with clinical signs, such as malleolar or pulmonary edema, in early onset preeclampsia. The arrows indicate the principle of volume estimation by bioimpedance spectrum analysis of intracellular (green) and extracellular (blue) compartments, the latter being the sum of intravascular and interstitial volumes.
Laboratory and Clinical Experiments Supporting Maternal Low Volume Circulation in Fetal Growth Restriction
A low sodium diet for pregnant rats prevents normal maternal plasma volume expansion and induces FGR and low placental weight (57). This association presents with poor dilatation of the uterine and radial arteries, together with reduced uterine blood volume flow and increased vascular resistance, and the activation of the renin-angiotensin-aldosterone system with reduced expression of placental Angiotensin II receptor subtype 1 (58) and with increased concentrations of placental markers of hypoxia (57, 58). Murine FGR presents with a reduced placental blood flow rate, demonstrable by both contrast-enhanced sonography and magnetic resonance imaging (MRI; 59).
Different types of MRI technology have been proven useful for non-invasive studies of placental perfusion in animals and human (60), allowing for the quantification of blood flow volume (61), and mapping and fractional differentiation of perfused and non-perfused areas (62, 63). As compared to normal pregnancies, FGR presents with a one-third decrease of placental perfusion fraction (64), strongly correlating with increased Doppler impedance measurements of uterine and umbilical arteries, particularly of the ductus venosus (DV; 64, 65). Blood flow velocity in the FGR placenta is reduced, is non-homogenous, and shows intermittent stops in severe cases with increased DV pulsatility index (65). Taken together, all MRI placenta perfusion studies show evidence for underfilling of the intervillous space, which is a trigger for both maternal and fetal reflex responses. Laboratory and animal models show that incomplete spiral artery trophoblast invasion results in an increase of oxygen tension (66) and perfusion pressure (67) in the intervillous space, with constriction of maternal chorionic plate venules (68), enhanced myogenic activity of uterine and radial arteries (69) despite an activated pathway of nitric oxide (NO)-dependent vasodilation (70), and altered placental cell populations and trophoblast differentiation (71).
Poor placenta perfusion in both PE and FGR is supported by histologic signs, such as accelerated villus branching, large and numerous syncytial knots, and small sclerotic villi, suggestive of placental hypoxia and/or oxidative stress (72).
Epidemiologic Data Supporting Maternal Low Volume Circulation in Fetal Growth Restriction
Epidemiologic studies have shown an intergenerational association of FGR: women who, themselves, were born with low birth weight are more likely to reproduce low birth weight offspring (73–75). Apart from genetic, familial, and socioeconomic predispositions, complex molecular processes, such as genetic imprinting, microchimerism, and epigenetic modifications, are involved (76, 77), and this results from early neonatal life onward in the disruption of endocrine and metabolic systems (78, 79) together with permanent dysfunctions of vital organ systems (80, 81), such as the kidneys (82, 83), the heart and blood circulation (76, 84, 85), the endothelium (86), and the immune system (80). In the long run, these systemic dysfunctions predispose to early onset adult disease (87). Body water volume homeostasis is another system that is dysfunctional in FGR, involving the renin-angiotensin system (88) and natriuretic peptides (89, 90). A particularly interesting observation is that FGR predisposes to low plasma volume in adult life (91). In former preeclamptic women, it was shown that preconceptional low plasma volume predisposes to recurrence of PE (92). Even more important is that, in nulliparous women, preconceptional vascular dysfunction (93) and angiotensinogen phenotype-dependent low plasma volume (94) predispose to abnormal perinatal outcomes (95). Low plasma volume coexists with poor venous reserves, resulting from abnormal venous capacitance and vascular compliance together with autonomic nervous dysfunction (96), and is associated with recurrent first-trimester pregnancy loss (97). As explained in the section, combined venous hemodynamic dysfunction in both mother and fetus in PE with FGR has also been observed in studies using Doppler-ECG ultrasonography.
Interactive Maternal-Fetal Hemodynamics in Fetal Growth Restriction
Mother-to-Fetus Circulatory Interactions in Fetal Growth Restriction
Placental angiogenesis and vasculogenesis involve cell-communicating factors including vascular endothelial growth factor (VEGF), placental growth factor (PlGF), and oxygen (98). Placental histology studies have shown that FGR is characterized by decreased branching angiogenesis with increased apoptosis, resulting in a reduced number of terminal villi and stromal capillaries, poor villi vascular density, fewer intervillous pores, increase of intervillous thrombi, villous infarctions, villitis, and thickening of the basal membrane (99, 100), finally resulting in overall reduced exchange surface area (101–103). This is associated with a lower and higher release of VEGF-A and PlGF, respectively, an unbalanced production of their cell receptors, and the release of anti-angiogenic factors, mechanisms supported by reduced oxygen tension and volume flow (39, 98). As a result, endothelial cells from the FGR placenta show dysregulated biochemical signaling with failed compensatory response to resist high blood flow (104), demonstrable by high Doppler impedance measurements at the level of uterine and umbilical arteries (15). Doppler sonography is a useful method to assess uterine and umbilical artery flow impedance. Increased uterine artery Doppler pulsatility index and resistivity index have long been considered a result of abnormal spiral artery adaptation at implantation, but, currently, increasing number of experimental, clinical, and epidemiological data are in favor of the opposite pathway, suggesting abnormal placentation merely as the result rather than the cause of abnormal uterine perfusion (105, 106). In this context, the inverse correlation between preconceptional uterine artery Doppler impedance measurements and subsequent birthweight in formerly preeclamptic women is illustrative (107).
With advancing gestation of FGR, the intrauterine environment becomes more and more hypoxic, to which the fetus responds by redirecting the blood supply, preferably to vital organs like the heart and the brain, at the expense of subdiaphragmatic organ perfusion (15). Fetal brain sparing can be documented by Doppler flow measurements at the level of umbilical and cerebral arteries with the calculation of relative impedance ratios (108–110). Associations have been reported between abnormal uterine-fetal Doppler measurements and maternal hemodynamic dysfunction (8) and between fetal cerebral Doppler changes and adverse outcomes (111).
On top of fetal arterial Doppler flow changes, DV Doppler flow patterns, shifting from biphasic to triphasic, offer additional information on deteriorating fetal condition (112–114; Figure 3). This evolution is very similar to the change of Doppler flow patterns in the maternal hepatic vein from early pregnancy to the clinical stage of EPE (115; Figure 3). The sequence of venous Doppler waveform changes, however, is different between the FGR fetus and the woman with EPE. In FGR, DV Doppler flow is secondary to altered cardiac function due to increased afterload for the right ventricle (RV) but not the left one, with subsequently reduced RV compliance and increased right atrium filling pressure, which reflects in the reversed DV Doppler A-wave (116, 117) and reduced CO to the placenta (118). In EPE, triphasic HV Doppler flow patterns are already present weeks before the clinical onset of disease (119), indicating the involvement of venous vascular wall activity (120, 121). Further research should elucidate whether this difference relates to a different intravascular filling state, which is low for the woman with PEFGR (Figure 1) and is linearly related to birth weight, irrespective of preceding intrauterine fetal condition (122, 123).
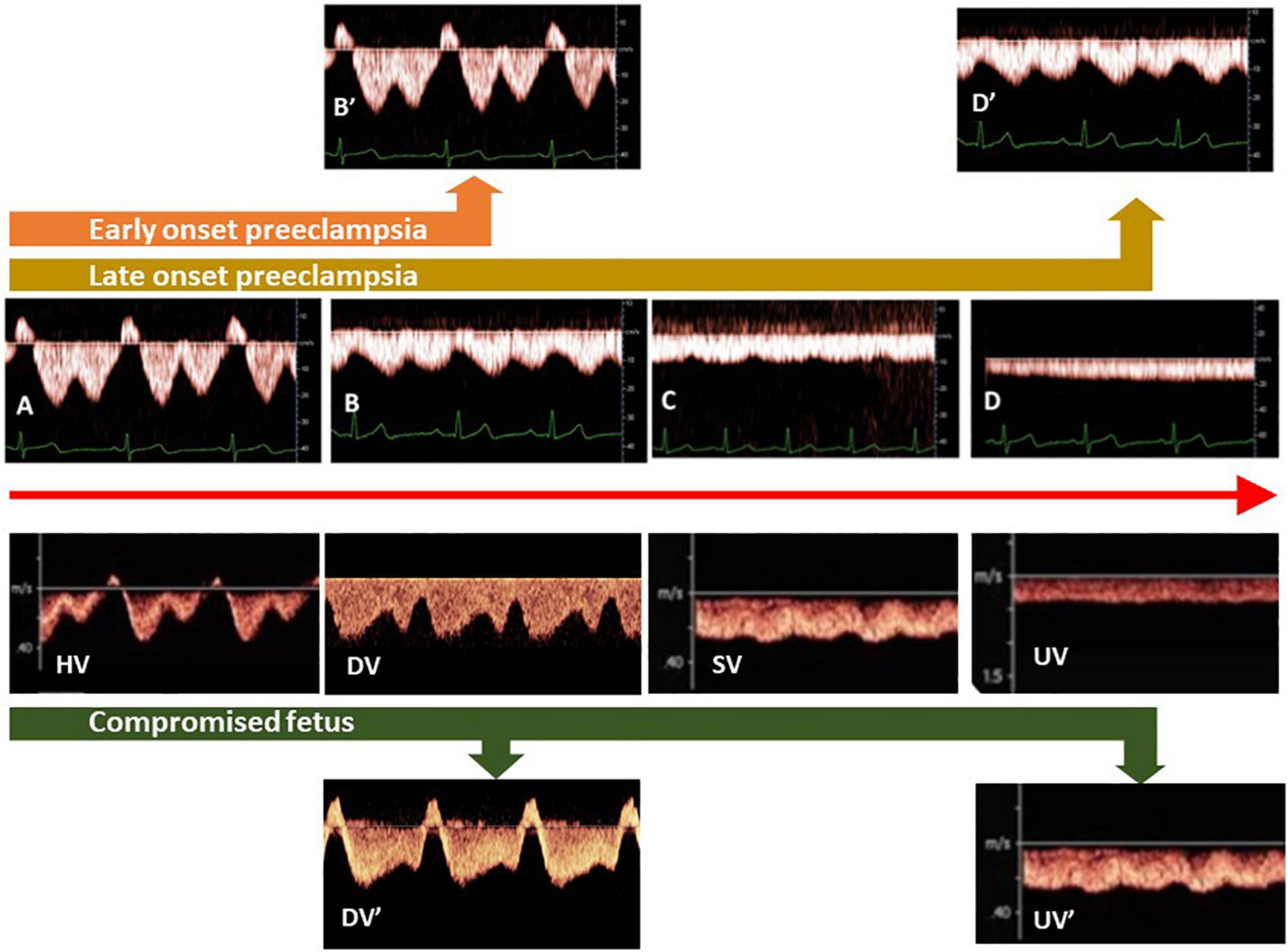
Figure 3. Different types of venous Doppler waveforms in maternal and fetal circulations, varying between triphasic (A), biphasic (B), and monophasic (C) to flat (D). In the fetus and non-pregnant adults, triphasic patterns are found close to the heart [hepatic veins (HV)], whereas flat patterns are present at distant locations [limbs, umbilical veins (UV)]. During uncomplicated pregnancy, HV patterns shift from (A–D). In the clinical stage of preeclampsia with FGR, biphasic HV patterns become triphasic (orange arrow), whereas in term preeclampsia, monophasic patterns evolve to biphasic (brown arrow). In ductus venosus (DV) of FGR fetuses, a shift from biphasic to triphasic patterns occurs simultaneously with deteriorating fetal condition (green arrow). (SV: fetal splenic vein).
Conceptus-to-Mother Circulatory Interactions in Fetal Growth Restriction
An important fetal-maternal communication system is the intravascular shedding of placental particles, varying in size and shape between multinucleated syncytial aggregates and subcellular nanovesicles, originating from not only apoptosis in a normal pregnancy but also necrosis in PE (124), and is associated with increased serum total cell-free DNA (125). These particles act via intravesical molecules and micro-RNA or circular RNA that, after phagocytosis by endothelium and immune cells, is capable of inducing sterile inflammation via increased surface expression of monocyte adhesion receptors, such as E-selectin, secretion of pro-inflammatory cytokines, namely interleukin 6 and transforming growth factor β (124). Downregulation of specific micro-RNAs has been reported in FGR, some of which are shared with PE (126). Next to this, the production of mediators of angiogenesis and vasoactivity is stimulated in FGR, such as soluble FMS-like tyrosine kinase and VEGF (127), whereas pregnancy-associated placental protein A (PAPP-A) is reduced (128). Many other vasoactive and immunologic mediators have also been studied in maternal serum concerning diagnosis or prediction of PE and/or FGR. The most-reported biomarkers studied for this purpose are summarized in Table 1. It has generally been accepted that the placenta is the primary source of these factors and, as such, is the primary driver of the global functioning of the maternal circulation. It should be emphasized, however, that abnormal serum concentrations of many of these factors have also been documented in non-pregnant individuals with (pre-clinical) chronic cardiovascular and/or renal disease (Table 1). This indicates that, apart from some placenta-specific products, it cannot be concluded indisputably whether the origin of the vasoactive and/or immunomodulatory serum substances associated with PE ± FGR is placental, maternal, or combined.
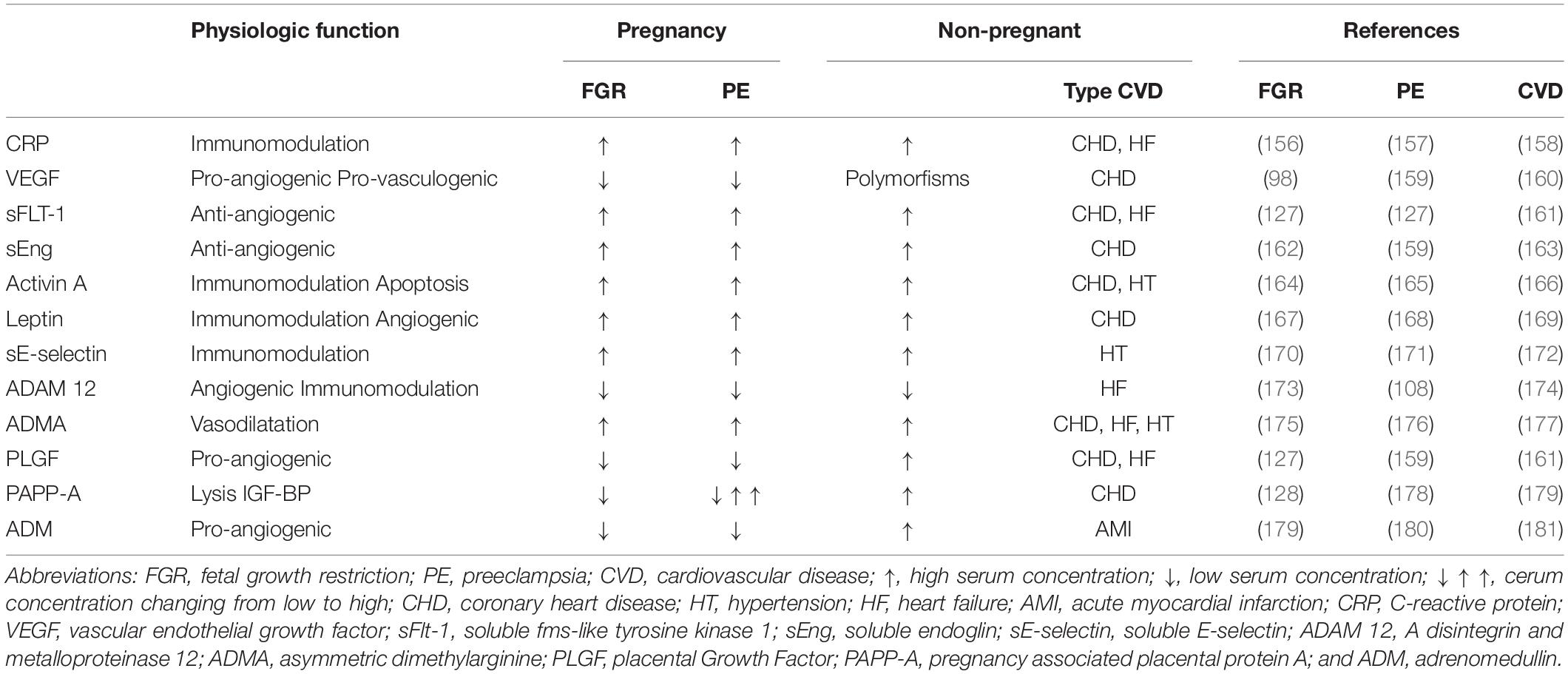
Table 1. Serum markers of fetal growth restriction (FGR), preeclampsia (PET), and/or cardiovascular disease (CVD).
Clinical Implications of Maternal Low Volume Circulation in Fetal Growth Restriction
The association between low maternal volume circulation and FGR has important clinical implications. First, maternal hemodynamics can offer additional information in unexplained cases of FGR, where all other known etiologic factors have been excluded (129). In recent times, non-invasive assessment of CO and peripheral resistance is feasible by different types of technologies (130), which, when used with appropriate reference ranges under standardized conditions (8, 131), can easily identify those women with a low output/high resistance circulation who are particularly at risk for FGR. This information is not only useful for the diagnosis of FGR but can also be of value from the first trimester onward before poor fetal growth is evident (29, 45, 132). This opens perspectives toward the implementation of maternal hemodynamics parameters into current screening programs for FGR (7, 133). Preliminary, though promising, data on the reduction of FGR have been reported on the supplementation of the screen positive high-risk group with antiplatelet therapy (134, 135) and/or the antioxidants lycopene or L-Arginin (136).
Secondly, maternal low volume circulation can be a target for the prevention of FGR pregnancies. Physical exercise is a well-known useful intervention for the improvement of cardiovascular functions (137). In formerly preeclamptic women included in a program of controlled physical exercise, an increase in stroke and plasma volume was observed (138) together with the improvement of venous reserves up to the pretraining levels of controls (139). In overweight and obese pregnant women, physical exercise throughout gestation was associated with a lower incidence of gestational diabetes and reduced third-trimester systolic blood pressure (140). Lower systolic blood pressure was also observed in trained versus non-trained normotensive pregnant women (141). Importantly, maternal physical training was shown to influence fetal cardiovascular functions by the increase of left ventricular output and aortic peak flow velocity (142) and by lower carotid artery wall thickness in offspring (143).
A third important implication for pregnancies complicated with both FGR and hypertension is the antihypertensive therapy of choice in addition to low dose aspirin (135) initiated before 16 weeks at ≥100 mg PD (134). Blood pressure-lowering pharmacologic mechanisms are different between beta-blockers, calcium blockers, and centrally active agents due to which the effects on neonatal birth weight are different. There is a growing body of evidence that adrenergic beta-blockers are associated with an increased birth rate of neonates SGA (144–148) with a mean effect estimated at ≤200 g at term (149). A similar but less pronounced effect has also been reported for Alfa-MethylDopa (145, 150) but not for calcium channel blockers (145). A possible explanation for this differential effect is that beta-blockers partially exert their effects via a reduction of CO (151), whereas calcium channel blockers mainly function via reduced peripheral resistance with a compensatory rise of CO (152). As such, from a theoretical perspective, calcium channel blockers might be a better choice than beta-blockers with respect to avoiding a negative pharmacologic impact on fetal growth. There, however, is an urgent need for more fundamental and clinical research into the differential mechanisms and outcomes of antihypertensive therapies in pregnant women.
An interesting clinical confirmation of the association between maternal intravascular volume and the gestational outcome has been reported in two studies by the Tor Vergata university of Rome’s research team (153, 154) using the potently vasodilating NO donors. As compared to a historical control group, a cohort of 26 FGR pregnancies treated with NO donors and plasma volume expansion showed an improvement in maternal CO and TVR and in higher birth weight after 2 weeks (153). Similarly, in 32 women with hypertension and FGR with absent end-diastolic umbilical Doppler flow, randomized between conventional management with or without NO donors and plasma volume expansion, the reappearance of diastolic umbilical blood flow and the prolongation of gestation were observed in the treated group (154).
Perspectives
This review summarizes evidence from clinical, experimental, and laboratory observations on the association between low volume maternal circulation and poor fetal growth. Conditions of low maternal CO can present before conception or develop during the earliest stages of pregnancy, in conditions of both normotension or hypertension relative to the balance between flow volume and vascular resistance. Intravascular volume is intimately related to CO, renal function, and through aldosterone and the renin-angiotensin system to peripheral vascular resistance and blood pressure. Hence, acknowledging that the association between maternal intravascular filling state and fetal wellbeing opens perspectives toward prevention, management, and reduction of intergenerational transfer of poor fetal growth. However, more in-depth exploration is needed on the role of normal or abnormal maternal cardiovascular function in obstetric and neonatal outcomes.
Author Contributions
Both authors listed have made a substantial, direct, and intellectual contribution to the work, and approved it for publication.
Funding
Publication fee will be paid by the research fund of WG (see further).
Conflict of Interest
The authors declare that the research was conducted in the absence of any commercial or financial relationships that could be construed as a potential conflict of interest.
Publisher’s Note
All claims expressed in this article are solely those of the authors and do not necessarily represent those of their affiliated organizations, or those of the publisher, the editors and the reviewers. Any product that may be evaluated in this article, or claim that may be made by its manufacturer, is not guaranteed or endorsed by the publisher.
Abbreviations
CO, cardiac output; DV, ductus venosus; EPE, early onset preeclampsia; FGR, fetal growth restriction; HR, heart rate; HV, hepatic veins; IL6, interleukin 6; MAP, mean arterial pressure; PAPP-A, pregnancy associated placental protein A; PE, preeclampsia; PlGF, placental growth factor; RV, right ventricle; S-FLT 1, soluble FMS-like tyrosine kinase 1; SGA, small for gestational age; SV, stroke volume; SV (Figure 3), splenic vein; TGF β, transforming growth factor β; TVR, total vascular resistance; UV, umbilical vein; VEGF, vascular endothelial growth factor.
References
1. Brosens I, Puttemans P, Benagiano G. Placental bed research: I. The placental bed: from spiral arteries remodeling to the great obstetrical syndromes. Am J Obstet Gynecol. (2019) 221:437–56. doi: 10.1016/j.ajog.2019.05.044
2. Burton GJ, Jauniaux E. Pathophysiology of placental-derived fetal growth restriction. Am J Obstet Gynecol. (2018) 218:S745–61. doi: 10.1016/j.ajog.2017.11.577
3. Foo FL, Mahendru AA, Masini G, Fraser A, Cacciatore S, MacIntyre DA, et al. Association between prepregnancy cardiovascular function and subsequent preeclampsia or fetal growth restriction. Hypertension. (2018) 72:442–50. doi: 10.1161/HYPERTENSIONAHA.118.11092
4. Gyselaers W. Preeclampsia is a syndrome with a cascade of pathophysiologic events. J Clin Med. (2020) 9:2245. doi: 10.3390/jcm9072245
5. Ferrazzi E, Stampalija T, Monasta L, Di Martino D, Vonck S, Gyselaers W. Maternal hemodynamics: a method to classify hypertensive disorders of pregnancy. Am J Obstet Gynecol. (2018) 218:124.e1–11. doi: 10.1016/j.ajog.2017.10.226
6. Tay J, Foo L, Masini G, Bennett PR, McEniery CM, Wilkinson IB, et al. Early and late preeclampsia are characterized by high cardiac output, but in the presence of fetal growth restriction, cardiac output is low: insights from a prospective study. Am J Obstet Gynecol. (2018) 218:517.e1–12. doi: 10.1016/j.ajog.2018.02.007
7. Mecacci F, Avagliano L, Lisi F, Clemenza S, Serena C, Vannuccini S, et al. Fetal Growth restriction: does an integrated maternal hemodynamic-placental model fit better? Reprod Sci. (2020) 28:2422–35. doi: 10.1007/s43032-020-00393-2
8. Masini G, Tay J, McEniery CM, Wilkinson IB, Valensise H, Tiralongo GM, et al. Maternal cardiovascular dysfunction is associated with hypoxic cerebral and umbilical Doppler changes. J Clin Med. (2020) 9:2891. doi: 10.3390/jcm9092891
9. de Haas S, Ghossein-Doha C, van Kuijk SM, van Drongelen J, Spaanderman ME. Physiological adaptation of maternal plasma volume during pregnancy: a systematic review and meta-analysis. Ultrasound Obstet Gynecol. (2017) 49:177–87. doi: 10.1002/uog.17360
10. Vonck S, Staelens AS, Lanssens D, Tomsin K, Oben J, Dreesen P, et al. Low volume circulation in normotensive women pregnant with neonates small for gestational age. Fetal Diagn Ther. (2019) 46:238–45. doi: 10.1159/000495507
11. Gyselaers W, Vonck S, Staelens AS, Lanssens D, Tomsin K, Oben J, et al. Body fluid volume homeostasis is abnormal in pregnancies complicated with hypertension and/or poor fetal growth. PLoS One. (2018) 13:e0206257. doi: 10.1371/journal.pone.0206257
12. Tiralongo GM, Lo Presti D, Pisani I, Gagliardi G, Scala RL, Novelli GP, et al. Assessment of total vascular resistance and total body water in normotensive women during the first trimester of pregnancy. A key for the prevention of preeclampsia. Pregnancy Hypertens. (2015) 5:193–7. doi: 10.1016/j.preghy.2015.02.001
13. Baethge C, Goldbeck-Wood S, Mertens S. SANRA-a scale for the quality assessment of narrative review articles. Res Integr Peer Rev. (2019) 4:5. doi: 10.1186/s41073-019-0064-8
14. Kramer MS. Determinants of low birth weight: methodological assessment and meta-analysis. Bull World Health Organ. (1987) 65:663–737.
15. Hutter D, Kingdom J, Jaeggi E. Causes and mechanisms of intrauterine hypoxia and its impact on the fetal cardiovascular system: a review. Int J Pediatr. (2010) 2010:401323. doi: 10.1155/2010/401323
16. Bertand ME, Widimsky J. Vascular resistance. Eur Heart J. (1985) 6(Suppl. C):19. doi: 10.1093/eurheartj/6.suppl_c.19
17. Rang S, van Montfrans GA, Wolf H. Serial hemodynamic measurement in normal pregnancy, preeclampsia, and intrauterine growth restriction. Am J Obstet Gynecol. (2008) 198:519.e1–9. doi: 10.1016/j.ajog.2007.11.014
18. Mahendru AA, Foo FL, McEniery CM, Everett TR, Wilkinson IB, Lees CC. Change in maternal cardiac output from preconception to mid-pregnancy is associated with birth weight in healthy pregnancies. Ultrasound Obstet Gynecol. (2017) 49:78–84. doi: 10.1002/uog.17368
19. Melchiorre K, Leslie K, Prefumo F, Bhide A, Thilaganathan B. First-trimester uterine artery Doppler indices in the prediction of small-for-gestational age pregnancy and intrauterine growth restriction. Ultrasound Obstet Gynecol. (2009) 33:524–9. doi: 10.1002/uog.6368
20. Roberts LA, Ling HZ, Poon LC, Nicolaides KH, Kametas NA. Maternal hemodynamics, fetal biometry and Doppler indices in pregnancies followed up for suspected fetal growth restriction. Ultrasound Obstet Gynecol. (2018) 52:507–14. doi: 10.1002/uog.19067
21. Stott D, Bolten M, Salman M, Paraschiv D, Clark K, Kametas NA. Maternal demographics and hemodynamics for the prediction of fetal growth restriction at booking, in pregnancies at high risk for placental insufficiency. Acta Obstet Gynecol Scand. (2016) 95:329–38. doi: 10.1111/aogs.12823
22. Yin O, Woods A, Koos B, DeVore G, Afshar Y. Central hemodynamics are associated with fetal outcomes in pregnancies of advanced maternal age. Pregnancy Hypertens. (2020) 19:67–73. doi: 10.1016/j.preghy.2019.12.009
23. Turan OM, De Paco C, Kametas N, Khaw A, Nicolaides KH. Effect of parity on maternal cardiac function during the first trimester of pregnancy. Ultrasound Obstet Gynecol. (2008) 32:849–54. doi: 10.1002/uog.5354
24. Kametas NA, McAuliffe F, Krampl E, Chambers J, Nicolaides KH. Maternal cardiac function in twin pregnancy. Obstet Gynecol. (2003) 102:806–15. doi: 10.1016/s0029-7844(03)00807-x
25. Kametas NA, McAuliffe F, Krampl E, Chambers J, Nicolaides KH. Maternal cardiac function during pregnancy at high altitude. BJOG. (2004) 111:1051–8. doi: 10.1111/j.1471-0528.2004.00246.x
26. Di Martino DD, Ferrazzi E, Garbin M, Fusè F, Izzo T, Duvekot J, et al. Multivariable evaluation of maternal hemodynamic profile in pregnancy complicated by fetal growth restriction: prospective study. Ultrasound Obstet Gynecol. (2019) 54:732–9. doi: 10.1002/uog.20118
27. Gyselaers W, Vonck S, Staelens AS, Lanssens D, Tomsin K, Oben J, et al. Gestational hypertensive disorders show unique patterns of circulatory deterioration with ongoing pregnancy. Am J Physiol Regul Integr Comp Physiol. (2019) 316:R210–21. doi: 10.1152/ajpregu.00075.2018
28. Tomsin K, Mesens T, Molenberghs G, Peeters L, Gyselaers W. Characteristics of heart, arteries, and veins in low and high cardiac output preeclampsia. Eur J Obstet Gynecol Reprod Biol. (2013) 169:218–22. doi: 10.1016/j.ejogrb.2013.03.016
29. Stott D, Papastefanou I, Paraschiv D, Clark K, Kametas NA. Longitudinal maternal hemodynamics in pregnancies affected by fetal growth restriction. Ultrasound Obstet Gynecol. (2017) 49:761–8. doi: 10.1002/uog.17340
30. Bamfo JE, Kametas NA, Turan O, Khaw A, Nicolaides KH. Maternal cardiac function in fetal growth restriction. BJOG. (2006) 113:784–91. doi: 10.1111/j.1471-0528.2006.00945.x
31. Di Pasquo E, Ghi T, Dall’Asta A, Angeli L, Ciavarella S, Armano G, et al. Hemodynamic findings in normotensive women with small for gestational age and growth restricted fetuses. Acta Obstet Gynecol Scand. (2020). doi: 10.1111/aogs.14026 [Epub ahead of print].
32. Odendaal H, Kieser E, Nel D, Brink L, du Plessis C, Groenewald C, et al. Effects of low maternal heart rate on fetal growth and birthweight. Int J Gynaecol Obstet. (2019) 146:250–6. doi: 10.1002/ijgo.12873
33. Valensise H, Vasapollo B, Gagliardi G, Novelli GP. Early and late preeclampsia: two different maternal hemodynamic states in the latent phase of the disease. Hypertension. (2008) 52:873–80. doi: 10.1161/HYPERTENSIONAHA.108.117358
34. Flo K, Wilsgaard T, Vårtun A, Acharya G. A longitudinal study of the relationship between maternal cardiac output measured by impedance cardiography and uterine artery blood flow in the second half of pregnancy. BJOG. (2010) 117:837–44. doi: 10.1111/j.1471-0528.2010.02548.x
35. Lang U, Baker RS, Braems G, Zygmunt M, Künzel W, Clark KE. Uterine blood flow–a determinant of fetal growth. Eur J Obstet Gynecol Reprod Biol. (2003) 110 (Suppl. 1):S55–61. doi: 10.1016/s0301-2115(03)00173-8
36. Guedes-Martins L, Cunha A, Saraiva J, Gaio R, Macedo F, Almeida H. Internal iliac and uterine arteries Doppler ultrasound in the assessment of normotensive and chronic hypertensive pregnant women. Sci Rep. (2014) 4:3785. doi: 10.1038/srep03785
37. McKelvey A, Pateman K, Balchin I, Peebles DM, Rodeck CH, David AL. Total uterine artery blood volume flow rate in nulliparous women is associated with birth weight and gestational age at delivery. Ultrasound Obstet Gynecol. (2017) 49:54–60. doi: 10.1002/uog.15917
38. Jeffreys RM, Stepanchak W, Lopez B, Hardis J, Clapp JF III. Uterine blood flow during supine rest and exercise after 28 weeks of gestation. BJOG. (2006) 113:1239–47. doi: 10.1111/j.1471-0528.2006.01056.x
39. Browne VA, Julian CG, Toledo-Jaldin L, Cioffi-Ragan D, Vargas E, Moore LG. Uterine artery blood flow, fetal hypoxia and fetal growth. Philos Trans R Soc Lond B Biol Sci. (2015) 370:20140068. doi: 10.1098/rstb.2014.0068
40. Vonck S, Staelens AS, Mesens T, Tomsin K, Gyselaers W. Hepatic hemodynamics and fetal growth: a relationship of interest for further research. PLoS One. (2014) 9:e115594. doi: 10.1371/journal.pone.0115594
41. Salas SP, Rosso P, Espinoza R, Robert JA, Valdés G, Donoso E. Maternal plasma volume expansion and hormonal changes in women with idiopathic fetal growth retardation. Obstet Gynecol. (1993) 81:1029–33.
42. Rosso P, Donoso E, Braun S, Espinoza R, Salas SP. Hemodynamic changes in underweight pregnant women. Obstet Gynecol. (1992) 79:908–12.
43. Salas SP, Marshall G, Gutiérrez BL, Rosso P. Time course of maternal plasma volume and hormonal changes in women with preeclampsia or fetal growth restriction. Hypertension. (2006) 47:203–8. doi: 10.1161/01.HYP.0000200042.64517.19
44. Duvekot JJ, Cheriex EC, Pieters FA, Menheere PP, Peeters LH. Early pregnancy changes in hemodynamics and volume homeostasis are consecutive adjustments triggered by a primary fall in systemic vascular tone. Am J Obstet Gynecol. (1993) 169:1382–92. doi: 10.1016/0002-9378(93)90405-8
45. Duvekot JJ, Cheriex EC, Pieters FA, Peeters LL. Severely impaired fetal growth is preceded by maternal hemodynamic maladaptation in very early pregnancy. Acta Obstet Gynecol Scand. (1995) 74:693–7. doi: 10.3109/00016349509021176
46. Rhoades R, Bell D. Pulmonary circulation and ventilation perfusion. 5th editon ed. In: R Rhoades, D Bell editors. Medical Physiology: Principles for Clinical Medicine. London: Wolters Kluwer. (2018) p. 398–411.
47. Lanssens Janssenssens D, Smeets CJP, Vandervoort P, Grieten L, Gyselaers W. Intrathoracic fluid changes from preconception to postpartum as measured by bio-impedance monitoring. J Matern Fetal Neonatal Med. (2020) 33:1625–7. doi: 10.1080/14767058.2018.1519797
48. Smeets CJ, Lanssens D, Gyselaers W, Bertrand PB, Grieten L, Vandervoort P. Detection of subclinical transient fluid accumulation during pregnancy in a patient with an implantable cardioverter defibrillator and OptiVol® fluid monitoring algorithm. Int J Cardiol. (2016) 214:163–5. doi: 10.1016/j.ijcard.2016.03.121
49. Cong J, Fan T, Yang X, Shen J, Cheng G, Zhang Z. Maternal cardiac remodeling and dysfunction in preeclampsia: a three-dimensional speckle-tracking echocardiography study. Int J Cardiovasc Imaging. (2015) 31:1361–8. doi: 10.1007/s10554-015-0694-y
50. Zeitler EP, Cooper LB, Clare RM, Chiswell K, Lowenstern A, Rogers JG, et al. OptiVol for volume assessment in patients with continuous flow left ventricular assist device. ASAIO J. (2021) 67:192–5. doi: 10.1097/MAT.0000000000001244
51. Massari F, Scicchitano P, Ciccone MM, Caldarola P, Aspromonte N, Iacoviello M, et al. Bioimpedance vector analysis predicts hospital length of stay in acute heart failure. Nutrition. (2019) 61:56–60. doi: 10.1016/j.nut.2018.10.028
52. Kumari M, Tang WH, Maroo AP. Natriuretic peptide testing in high-risk pregnancy: a preventive opportunity? Curr Heart Fail Rep. (2014) 11:471–6. doi: 10.1007/s11897-014-0228-2
53. Bellos I, Pergialiotis V, Papapanagiotou A, Loutradis D, Daskalakis G. Association between serum copeptin levels and preeclampsia risk: a meta-analysis. Eur J Obstet Gynecol Reprod Biol. (2020) 250:66–73. doi: 10.1016/j.ejogrb.2020.04.051
54. Golboni F, Heydarpour S, Taghizadeh Z, Kazemnezhad A. Predictive value of plasma haematocrit level in early diagnosis of pre-eclampsia. East Mediterr Health J. (2011) 17:744–8. doi: 10.26719/2011.17.10.744
55. Nasiri M, Faghihzadeh S, Alavi Majd H, Zayeri F, Kariman N, Safavi Ardebili N. Longitudinal discriminant analysis of hemoglobin level for predicting preeclampsia. Iran Red Crescent Med J. (2015) 17:e19489. doi: 10.5812/ircmj.19489
56. Jakó M, Surányi A, Kaizer L, Németh G, Bártfai G. Maternal hematological parameters and placental and umbilical cord histopathology in intrauterine growth restriction. Med Princ Pract. (2019) 28:101–8. doi: 10.1159/000497240
57. Bibeau K, Sicotte B, Béland M, Bhat M, Gaboury L, Couture R, et al. Placental underperfusion in a rat model of intrauterine growth restriction induced by a reduced plasma volume expansion. PLoS One. (2016) 11:e0145982. doi: 10.1371/journal.pone.0145982
58. Bédard S, Sicotte B, St-Louis J, Brochu M. Modulation of body fluids and angiotensin II receptors in a rat model of intra-uterine growth restriction. J Physiol. (2005) 562(Pt 3):937–50. doi: 10.1113/jphysiol.2004.064683
59. Arthuis CJ, Mendes V, Même S, Même W, Rousselot C, Winer N, et al. Comparative determination of placental perfusion by magnetic resonance imaging and contrast-enhanced ultrasound in a murine model of intrauterine growth restriction. Placenta. (2018) 69:74–81. doi: 10.1016/j.placenta.2018.07.009
60. Siauve N, Chalouhi GE, Deloison B, Alison M, Clement O, Ville Y, et al. Functional imaging of the human placenta with magnetic resonance. Am J Obstet Gynecol. (2015) 213(4 Suppl.):S103–14. doi: 10.1016/j.ajog.2015.06.045
61. Deloison B, Siauve N, Aimot S, Balvay D, Thiam R, Cuenod CA, et al. SPIO-enhanced magnetic resonance imaging study of placental perfusion in a rat model of intrauterine growth restriction. BJOG. (2012) 119:626–33. doi: 10.1111/j.1471-0528.2011.03251.x
62. Francis ST, Duncan KR, Moore RJ, Baker PN, Johnson IR, Gowland PA. Non-invasive mapping of placental perfusion. Lancet. (1998) 351:1397–9. doi: 10.1016/S0140-6736(97)07089-X
63. Javor D, Nasel C, Schweim T, Dekan S, Chalubinski K, Prayer D. In vivo assessment of putative functional placental tissue volume in placental intrauterine growth restriction (IUGR) in human fetuses using diffusion tensor magnetic resonance imaging. Placenta. (2013) 34:676–80. doi: 10.1016/j.placenta.2013.04.018
64. Sohlberg S, Mulic-Lutvica A, Olovsson M, Weis J, Axelsson O, Wikström J, et al. Magnetic resonance imaging-estimated placental perfusion in fetal growth assessment. Ultrasound Obstet Gynecol. (2015) 46:700–5. doi: 10.1002/uog.14786
65. Brunelli R, Masselli G, Parasassi T, De Spirito M, Papi M, Perrone G, et al. Intervillous circulation in intra-uterine growth restriction. Correlation to fetal well being. Placenta. (2010) 31:1051–6. doi: 10.1016/j.placenta.2010.09.004
66. Huppertz B, Weiss G, Moser G. Trophoblast invasion and oxygenation of the placenta: measurements versus presumptions. J Reprod Immunol. (2014) 10:74–9. doi: 10.1016/j.jri.2013.04.003
67. Roth CJ, Haeussner E, Ruebelmann T, Koch FV, Schmitz C, Frank HG, et al. Dynamic modeling of uteroplacental blood flow in IUGR indicates vortices and elevated pressure in the intervillous space – a pilot study. Sci Rep. (2017) 7:40771. doi: 10.1038/srep40771
68. Wareing M, Greenwood SL, Fyfe GK, Baker PN. Reactivity of human placental chorionic plate vessels from pregnancies complicated by intrauterine growth restriction (IUGR). Biol Reprod. (2006) 75:518–23. doi: 10.1095/biolreprod.106.051607
69. Reho JJ, Toot JD, Peck J, Novak J, Yun YH, Ramirez RJ. Increased myogenic reactivity of uterine arteries from pregnant rats with reduced uterine perfusion pressure. Pregnancy Hypertens. (2012) 2:106–14. doi: 10.1016/j.preghy.2011.11.005
70. Bigonnesse E, Sicotte B, Brochu M. Activated NO pathway in uterine arteries during pregnancy in an IUGR rat model. Am J Physiol Heart Circ Physiol. (2018) 315:H415–22. doi: 10.1152/ajpheart.00457.2017
71. Natale BV, Mehta P, Vu P, Schweitzer C, Gustin K, Kotadia R, et al. Reduced uteroplacental perfusion pressure (RUPP) causes altered trophoblast differentiation and pericyte reduction in the mouse placenta labyrinth. Sci Rep. (2018) 8:17162. doi: 10.1038/s41598-018-35606-x
72. Roberts JM, Escudero C. The placenta in preeclampsia. Pregnancy Hypertens. (2012) 2:72–83. doi: 10.1016/j.preghy.2012.01.001
73. Cheong JN, Wlodek ME, Moritz KM, Cuffe JS. Programming of maternal and offspring disease: impact of growth restriction, fetal sex and transmission across generations. J Physiol. (2016) 594:4727–40. doi: 10.1113/JP271745
74. Liu D, Lin G, Su D, Alexender JM, Sun X, Qu M. Intergenerational associations of adverse birth outcomes: a surveillance report. Prev Med Rep. (2020) 20:101226. doi: 10.1016/j.pmedr.2020.101226
75. Su D, Samson K, Garg A, Hanson C, Anderson Berry AL, Lin G, et al. Birth history as a predictor of adverse birth outcomes: evidence from state vital statistics data. Prev Med Rep. (2018) 11:63–8. doi: 10.1016/j.pmedr.2018.05.011
76. Langmia IM, Kräker K, Weiss SE, Haase N, Schütte T, Herse F, et al. Cardiovascular programming during and after diabetic pregnancy: role of placental dysfunction and IUGR. Front Endocrinol (Lausanne). (2019) 10:215. doi: 10.3389/fendo.2019.00215
77. Sailasree SP, Srivastava S, Mishra RK. The placental gateway of maternal transgenerational epigenetic inheritance. J Genet. (2017) 96:465–82. doi: 10.1007/s12041-017-0788-5
78. Baschat AA. Fetal responses to placental insufficiency: an update. BJOG. (2004) 111:1031–41. doi: 10.1111/j.1471-0528.2004.00273.x
79. Zadik Z. Maternal nutrition, fetal weight, body composition and disease in later life. J Endocrinol Invest. (2003) 26:941–5. doi: 10.1007/BF03345248
80. Colella M, Frérot A, Novais ARB, Baud O. Neonatal and long-term consequences of fetal growth restriction. Curr Pediatr Rev. (2018) 14:212–8. doi: 10.2174/1573396314666180712114531
81. Malhotra A, Allison BJ, Castillo-Melendez M, Jenkin G, Polglase GR, Miller SL. Neonatal morbidities of fetal growth restriction: pathophysiology and impact. Front Endocrinol (Lausanne). (2019) 10:55. doi: 10.3389/fendo.2019.00055
82. Nüsken E, Dötsch J, Weber LT, Nüsken KD. Developmental programming of renal function and re-programming approaches. Front Pediatr. (2018) 6:36. doi: 10.3389/fped.2018.00036
83. Paixão AD, Alexander BT. How the kidney is impacted by the perinatal maternal environment to develop hypertension. Biol Reprod. (2013) 89:144. doi: 10.1095/biolreprod.113.111823
84. Demicheva E, Crispi F. Long-term follow-up of intrauterine growth restriction: cardiovascular disorders. Fetal Diagn Ther. (2014) 36:143–53. doi: 10.1159/000353633
85. Menendez-Castro C, Rascher W, Hartner A. Intrauterine growth restriction - impact on cardiovascular diseases later in life. Mol Cell Pediatr. (2018) 5:4. doi: 10.1186/s40348-018-0082-5
86. Yzydorczyk C, Armengaud JB, Peyter AC, Chehade H, Cachat F, Juvet C, et al. Endothelial dysfunction in individuals born after fetal growth restriction: cardiovascular and renal consequences and preventive approaches. J Dev Orig Health Dis. (2017) 8:448–64. doi: 10.1017/S2040174417000265
87. Armengaud JB, Yzydorczyk C, Siddeek B, Peyter AC, Simeoni U. Intrauterine growth restriction: clinical consequences on health and disease at adulthood. Reprod Toxicol. (2020). doi: 10.1016/j.reprotox.2020.10.005 [Epub ahead of print].
88. Lumbers ER, Pringle KG, Wang Y, Gibson KJ. The renin-angiotensin system from conception to old age: the good, the bad and the ugly. Clin Exp Pharmacol Physiol. (2013) 40:743–52. doi: 10.1111/1440-1681.12098
89. Bahlmann F, Krummenauer F, Spahn S, Gallinat R, Kampmann C. Natriuretic peptide levels in intrauterine growth-restricted fetuses with absent and reversed end-diastolic flow of the umbilical artery in relation to ductus venosus flow velocities. J Perinat Med. (2011) 39:529–37. doi: 10.1515/jpm.2011.065
90. Capponi A, Rizzo G, De Angelis C, Arduini D, Romanini C. Atrial natriuretic peptide levels in fetal blood in relation to inferior vena cava velocity waveforms. Obstet Gynecol. (1997) 89:242–7. doi: 10.1016/S0029-7844(96)00432-2
91. Scholten RR, Oyen WJ, Van der Vlugt MJ, Van Dijk AP, Hopman MT, Lotgering FK, et al. Impaired fetal growth and low plasma volume in adult life. Obstet Gynecol. (2011) 118:1314–22. doi: 10.1097/AOG.0b013e3182383781
92. Scholten RR, Sep S, Peeters L, Hopman MT, Lotgering FK, Spaanderman ME. Prepregnancy low-plasma volume and predisposition to preeclampsia and fetal growth restriction. Obstet Gynecol. (2011) 117:1085–93. doi: 10.1097/AOG.0b013e318213cd31
93. Hale SA, Badger GJ, McBride C, Magness R, Bernstein IM. Prepregnancy vascular dysfunction in women who subsequently develop hypertension during pregnancy. Pregnancy Hypertens. (2013) 3:140–5. doi: 10.1016/j.preghy.2013.01.006
94. Bernstein IM, Ziegler W, Stirewalt WS, Brumsted J, Ward K. Angiotensinogen genotype and plasma volume in nulligravid women. Obstet Gynecol. (1998) 92:171–3. doi: 10.1016/s0029-7844(98)00206-3
95. Bernstein IM, Damron D, Schonberg AL, Sallam RM, Shapiro R. The relationship of plasma volume, sympathetic tone, and proinflammatory cytokines in young healthy nonpregnant women. Reprod Sci. 2009 Oct;16(10):980-5. doi: 10.1177/1933719109338876 Erratum Reprod Sci. (2014) 21:543.
96. Heidema WM, van Drongelen J, Spaanderman MEA, Scholten RR. Venous and autonomic function in formerly pre-eclamptic women and controls matched for body mass index. Ultrasound Obstet Gynecol. (2019) 53:376–82. doi: 10.1002/uog.19060
97. Donckers J, Scholten RR, Oyen WJ, Hopman MT, Lotgering FK, Spaanderman ME. Unexplained first trimester recurrent pregnancy loss and low venous reserves. Hum Reprod. (2012) 27:2613–8. doi: 10.1093/humrep/des245
98. Arroyo JA, Winn VD. Vasculogenesis and angiogenesis in the IUGR placenta. Semin Perinatol. (2008) 32:172–7. doi: 10.1053/j.semperi.2008.02.006
99. Silver RM. Examining the link between placental pathology, growth restriction, and stillbirth. Best Pract Res Clin Obstet Gynaecol. (2018) 49:89–102. doi: 10.1016/j.bpobgyn.2018.03.004
100. Vedmedovska N, Rezeberga D, Teibe U, Melderis I, Donders GG. Microscopic lesions of placenta and Doppler velocimetry related to fetal growth restriction. Arch Gynecol Obstet. (2011) 284:1087–93. doi: 10.1007/s00404-010-1781-x
101. Almasry SM, Eldomiaty MA, Elfayomy AK, Habib FA, Safwat MD. Structural analysis of human placental stem and terminal villi from normal and idiopathic growth restricted pregnancies. J Mol Histol. (2012) 43:263–71. doi: 10.1007/s10735-012-9405-3
102. Rainey A, Mayhew TM. Volumes and numbers of intervillous pores and villous domains in placentas associated with intrauterine growth restriction and/or pre-eclampsia. Placenta. (2010) 31:602–6. doi: 10.1016/j.placenta.2010.04.005
103. Scifres CM, Nelson DM. Intrauterine growth restriction, human placental development and trophoblast cell death. J Physiol. (2009) 587(Pt 14):3453–8. doi: 10.1113/jphysiol.2009.173252
104. Jones S, Bischof H, Lang I, Desoye G, Greenwood SL, Johnstone ED, et al. Dysregulated flow-mediated vasodilatation in the human placenta in fetal growth restriction. J Physiol. (2015) 593:3077–92. doi: 10.1113/JP270495
105. Gyselaers W, Lees C, Valensise H, Thilaganathan B. Preeclampsia: the role of persistent endothelial cells in uteroplacental arteries. Brosens I, Brosens JJ, Muter J, Puttemans P, Benagiano G. Am J Obstet Gynecol 2019;221:219-26. Am J Obstet Gynecol. (2020) 222:633. doi: 10.1016/j.ajog.2019.12.274
106. Ridder A, Giorgione V, Khalil A, Thilaganathan B. Preeclampsia: the relationship between uterine artery blood flow and trophoblast function. Int J Mol Sci. (2019) 20:3263. doi: 10.3390/ijms20133263
107. Spaanderman ME, Willekes C, Hoeks AP, Ekhart TH, Aardenburg R, Courtar DA, et al. Maternal nonpregnant vascular function correlates with subsequent fetal growth. Am J Obstet Gynecol. (2005) 192:504–12. doi: 10.1016/j.ajog.2004.08.035
108. El-Sherbiny W, Nasr A, Soliman A. Metalloprotease (ADAM12-S) as a predictor of preeclampsia: correlation with severity, maternal complications, fetal outcome, and Doppler parameters. Hypertens Pregnancy. (2012) 31:442–50.
109. Vollgraff Heidweiller-Schreurs CA, van Osch IR, Heymans MW, Ganzevoort W, Schoonmade LJ, Bax CJ, et al. Cerebroplacental ratio in predicting adverse perinatal outcome: a meta-analysis of individual participant data. BJOG. (2020). doi: 10.1111/1471-0528.16287 [Epub ahead of print].
110. Wolf H, Stampalija T, Monasta L, Lees CC. Ratio of umbilical and cerebral artery pulsatility indices in assessment of fetal risk: numerator and denominator matter. Ultrasound Obstet Gynecol. (2020) 56:163–5. doi: 10.1002/uog.22004
111. Stampalija T, Thornton J, Marlow N, Napolitano R, Bhide A, Pickles T, et al. Fetal cerebral Doppler changes and outcome in late preterm fetal growth restriction: prospective cohort study. Ultrasound Obstet Gynecol. (2020) 56:173–81. doi: 10.1002/uog.22125
112. Baschat AA, Gembruch U, Reiss I, Gortner L, Weiner CP, Harman CR. Relationship between arterial and venous Doppler and perinatal outcome in fetal growth restriction. Ultrasound Obstet Gynecol. (2000) 16:407–13. doi: 10.1046/j.1469-0705.2000.00284.x
113. Baschat AA, Gembruch U, Harman CR. The sequence of changes in Doppler and biophysical parameters as severe fetal growth restriction worsens. Ultrasound Obstet Gynecol. (2001) 18:571–7. doi: 10.1046/j.0960-7692.2001.00591.x
114. Müller T, Nanan R, Rehn M, Kristen P, Dietl J. Arterial and ductus venosus Doppler in fetuses with absent or reverse end-diastolic flow in the umbilical artery: longitudinal analysis. Fetal Diagn Ther. (2003) 18:163–9. doi: 10.1159/000069371
115. Gyselaers W. Hemodynamics of the maternal venous compartment: a new area to explore in obstetric ultrasound imaging. Ultrasound Obstet Gynecol. (2008) 32:716–7. doi: 10.1002/uog.6113
116. Seravalli V, Miller JL, Block-Abraham D, Baschat AA. Ductus venosus Doppler in the assessment of fetal cardiovascular health: an updated practical approach. Acta Obstet Gynecol Scand. (2016) 95:635–44. doi: 10.1111/aogs.12893
117. Severi FM, Rizzo G, Bocchi C, D’Antona D, Verzuri MS, Arduini D. Intrauterine growth retardation and fetal cardiac function. Fetal Diagn Ther. (2000) 15:8–19. doi: 10.1159/000020969
118. Rizzo G, Capponi A, Cavicchioni O, Vendola M, Arduini D. Low cardiac output to the placenta: an early hemodynamic adaptive mechanism in intrauterine growth restriction. Ultrasound Obstet Gynecol. (2008) 32:155–9. doi: 10.1002/uog.5389
119. Gyselaers W, Mesens T. Renal interlobar vein impedance index: a potential new Doppler parameter in the prediction of preeclampsia? J Matern Fetal Neonatal Med. (2009) 22:1219–21. doi: 10.3109/14767050903019635
120. Gyselaers W, Tomsin K, Staelens A, Mesens T, Oben J, Molenberghs G. Maternal venous hemodynamics in gestational hypertension and preeclampsia. BMC Pregnancy Childbirth. (2014) 14:212. doi: 10.1186/1471-2393-14-212
121. Gyselaers W, Staelens A, Mesens T, Tomsin K, Oben J, Vonck S, et al. Maternal venous Doppler characteristics are abnormal in pre-eclampsia but not in gestational hypertension. Ultrasound Obstet Gynecol. (2015) 45:421–6. doi: 10.1002/uog.13427
122. Brans YW, Shannon DL, Ramamurthy RS. Neonatal polycythemia: II. Plasma, blood and red cell volume estimates in relation to hematocrit levels and quality of intrauterine growth. Pediatrics. (1981) 68:175–82.
123. Maertzdorf WJ, Aldenhuyzen-Dorland W, Slaaf DW, Tangelder GJ, Blanco CE. Circulating blood volume in appropriate and small for gestational age full term and preterm polycythaemic infants. Acta Paediatr Scand. (1991) 80:620–7. doi: 10.1111/j.1651-2227.1991.tb11920.x
124. Chamley LW, Holland OJ, Chen Q, Viall CA, Stone PR, Abumaree M. Review: where is the maternofetal interface? Placenta. (2014) 35(Suppl.):S74–80. doi: 10.1016/j.placenta.2013.10.014
125. Rafaeli-Yehudai T, Imterat M, Douvdevani A, Tirosh D, Benshalom-Tirosh N, Mastrolia SA, et al. Maternal total cell-free DNA in preeclampsia and fetal growth restriction: evidence of differences in maternal response to abnormal implantation. PLoS One. (2018) 13:e0200360. doi: 10.1371/journal.pone.0200360
126. Hromadnikova I, Kotlabova K, Hympanova L, Krofta L. Gestational hypertension, preeclampsia and intrauterine growth restriction induce dysregulation of cardiovascular and cerebrovascular disease associated microRNAs in maternal whole peripheral blood. Thromb Res. (2016) 137:126–40. doi: 10.1016/j.thromres.2015.11.032
127. Wallner W, Sengenberger R, Strick R, Strissel PL, Meurer B, Beckmann MW, et al. Angiogenic growth factors in maternal and fetal serum in pregnancies complicated by intrauterine growth restriction. Clin Sci (Lond). (2007) 112:51–7. doi: 10.1042/CS20060161
128. Morris RK, Bilagi A, Devani P, Kilby MD. Association of serum PAPP-A levels in first trimester with small for gestational age and adverse pregnancy outcomes: systematic review and meta-analysis. Prenat Diagn. (2017) 37:253–65. doi: 10.1002/pd.5001
129. Gaudineau A. Prévalence, facteurs de risque et morbi-mortalité materno-fśtale des troubles de la croissance fśtale [Prevalence, risk factors, maternal and fetal morbidity and mortality of intrauterine growth restriction and small-for-gestational age]. J Gynecol Obstet Biol Reprod (Paris). (2013) 42:895–910. doi: 10.1016/j.jgyn.2013.09.013
130. Meah VL, Backx K, Davenport MH, International Working Group on Maternal Hemodynamics. Functional hemodynamic testing in pregnancy: recommendations of the international working group on maternal hemodynamics. Ultrasound Obstet Gynecol. (2018) 51:331–40. doi: 10.1002/uog.18890
131. Bijl RC, Valensise H, Novelli GP, Vasapollo B, Wilkinson I, Thilaganathan B, et al. Methods and considerations concerning cardiac output measurement in pregnant women: recommendations of the international working group on maternal hemodynamics. Ultrasound Obstet Gynecol. (2019) 54:35–50. doi: 10.1002/uog.20231
132. Ling HZ, Guy GP, Bisquera A, Poon LC, Nicolaides KH, Kametas NA. Maternal hemodynamics in screen-positive and screen-negative women of the ASPRE trial. Ultrasound Obstet Gynecol. (2019) 54:51–7. doi: 10.1002/uog.20125
133. Audette MC, Kingdom JC. Screening for fetal growth restriction and placental insufficiency. Semin Fetal Neonatal Med. (2018) 23:119–25. doi: 10.1016/j.siny.2017.11.004
134. Groom KM, David AL. The role of aspirin, heparin, and other interventions in the prevention and treatment of fetal growth restriction. Am J Obstet Gynecol. (2018) 218:S829–40. doi: 10.1016/j.ajog.2017.11.565
135. Leitich H, Egarter C, Husslein P, Kaider A, Schemper M. A meta-analysis of low dose aspirin for the prevention of intrauterine growth retardation. Br J Obstet Gynaecol. (1997) 104:450–9. doi: 10.1111/j.1471-0528.1997.tb11497.x
136. Tenório MB, Ferreira RC, Moura FA, Bueno NB, Goulart MOF, Oliveira ACM. Oral antioxidant therapy for prevention and treatment of preeclampsia: meta-analysis of randomized controlled trials. Nutr Metab Cardiovasc Dis. (2018) 28:865–76. doi: 10.1016/j.numecd.2018.06.002
137. Melzer K, Schutz Y, Boulvain M, Kayser B. Physical activity and pregnancy: cardiovascular adaptations, recommendations and pregnancy outcomes. Sports Med. (2010) 40:493–507. doi: 10.2165/11532290-000000000-00000
138. Krabbendam I, Maas ML, Thijssen DH, Oyen WJ, Lotgering FK, Hopman MT, et al. Exercise-induced changes in venous vascular function in nonpregnant formerly preeclamptic women. Reprod Sci. (2009) 16:414–20. doi: 10.1177/1933719109332091
139. Scholten RR, Hopman MT, Lotgering FK, Spaanderman ME. Aerobic exercise training in formerly preeclamptic women: effects on venous reserve. Hypertension. (2015) 66:1058–65. doi: 10.1161/HYPERTENSIONAHA.115.05786
140. Garnæs KK, Mørkved S, Salvesen Ø, Moholdt T. Exercise training and weight gain in obese pregnant women: a randomized controlled trial (ETIP trial). PLoS Med. (2016) 13:e1002079. doi: 10.1371/journal.pmed.1002079
141. Haakstad LA, Edvardsen E, Bø K. Effect of regular exercise on blood pressure in normotensive pregnant women. A randomized controlled trial. Hypertens Pregnancy. (2016) 35:170–80. doi: 10.3109/10641955.2015.1122036
142. May LE, McDonald S, Forbes L, Jones R, Newton E, Strickland D, et al. Influence of maternal aerobic exercise during pregnancy on fetal cardiac function and outflow. Am J Obstet Gynecol MFM. (2020) 2:100095. doi: 10.1016/j.ajogmf.2020.100095
143. Brislane Á, Jones H, Holder SM, Low DA, Hopkins ND. The Effect of exercise during pregnancy on maternal and offspring vascular outcomes: a pilot study. Reprod Sci. (2020). doi: 10.1007/s43032-020-00302-7 [Epub ahead of print].
144. Duan L, Ng A, Chen W, Spencer HT, Lee MS. Beta-blocker subtypes and risk of low birth weight in newborns. J Clin Hypertens (Greenwich). (2018) 20:1603–9. doi: 10.1111/jch.13397
145. Fitton CA, Steiner MFC, Aucott L, Pell JP, Mackay DF, Fleming M, et al. In-utero exposure to antihypertensive medication and neonatal and child health outcomes: a systematic review. J Hypertens. (2017) 35:2123–37. doi: 10.1097/HJH.0000000000001456
146. Meidahl Petersen K, Jimenez-Solem E, Andersen JT, Petersen M, Brødbæk K, Køber L, et al. β-blocker treatment during pregnancy and adverse pregnancy outcomes: a nationwide population-based cohort study. BMJ Open. (2012) 2:e001185. doi: 10.1136/bmjopen-2012-001185
147. Nakhai-Pour HR, Rey E, Bérard A. Antihypertensive medication use during pregnancy and the risk of major congenital malformations or small-for-gestational-age newborns. Birth Defects Res B Dev Reprod Toxicol. (2010) 89:147–54. doi: 10.1002/bdrb.20238
148. Ruys TP, Maggioni A, Johnson MR, Sliwa K, Tavazzi L, Schwerzmann M, et al. Cardiac medication during pregnancy, data from the ROPAC. Int J Cardiol. (2014) 177:124–8. doi: 10.1016/j.ijcard.2014.09.013
149. Grewal J, Siu SC, Lee T, D’Souza R, Dvir M, Singer J, et al. Impact of beta-blockers on birth weight in a high-risk cohort of pregnant women with CVD. J Am Coll Cardiol. (2020) 75:2751–2. doi: 10.1016/j.jacc.2020.03.068
150. Kayser A, Beck E, Hoeltzenbein M, Zinke S, Meister R, Weber-Schoendorfer C, et al. Neonatal effects of intrauterine metoprolol/bisoprolol exposure during the second and third trimester: a cohort study with two comparison groups. J Hypertens. (2020) 38:354–61. doi: 10.1097/HJH.0000000000002256
151. Frishman WH. Beta-adrenergic receptor blockers in hypertension: alive and well. Prog Cardiovasc Dis. (2016) 59:247–52. doi: 10.1016/j.pcad.2016.10.005
152. Cornette J, Duvekot JJ, Roos-Hesselink JW, Hop WC, Steegers EA. Maternal and fetal haemodynamic effects of nifedipine in normotensive pregnant women. BJOG. (2011) 118:510–40. doi: 10.1111/j.1471-0528.2010.02794.x
153. Tiralongo GM, Pisani I, Vasapollo B, Khalil A, Thilaganathan B, Valensise H. Effect of a nitric oxide donor on maternal hemodynamics in fetal growth restriction. Ultrasound Obstet Gynecol. (2018) 51:514–8. doi: 10.1002/uog.17454
154. Valensise H, Vasapollo B, Novelli GP, Giorgi G, Verallo P, Galante A, et al. Maternal and fetal hemodynamic effects induced by nitric oxide donors and plasma volume expansion in pregnancies with gestational hypertension complicated by intrauterine growth restriction with absent end-diastolic flow in the umbilical artery. Ultrasound Obstet Gynecol. (2008) 31:55–64. doi: 10.1002/uog.5234
155. Pang CC. Autonomic control of the venous system in health and disease: effects of drugs. Pharmacol Ther. (2001) 90:179–230. doi: 10.1016/s0163-7258(01)00138-3
156. Ferguson KK, Kamai EM, Cantonwine DE, Mukherjee B, Meeker JD, McElrath TF. Associations between repeated ultrasound measures of fetal growth and biomarkers of maternal oxidative stress and inflammation in pregnancy. Am J Reprod Immunol. (2018) 80:e13017. doi: 10.1111/aji.13017
157. Rebelo F, Schlüssel MM, Vaz JS, Franco-Sena AB, Pinto TJ, Bastos FI, et al. C-reactive protein and later preeclampsia: systematic review and meta-analysis taking into account the weight status. J Hypertens. (2013) 31:16–26.
158. Wang XY, Zhang F, Zhang C, Zheng LR, Yang J. The biomarkers for acute myocardial infarction and heart failure. Biomed Res Int. (2020) 2020:2018035.
159. Kleinrouweler CE, Wiegerinck MM, Ris-Stalpers C, Bossuyt PM, van der Post JA, von Dadelszen P, et al. Accuracy of circulating placental growth factor, vascular endothelial growth factor, soluble fms-like tyrosine kinase 1 and soluble endoglin in the prediction of pre-eclampsia: a systematic review and meta-analysis. BJOG. (2012) 119:778–87.
160. Ma WQ, Wang Y, Han XQ, Zhu Y, Liu NF. Association of genetic polymorphisms in vascular endothelial growth factor with susceptibility to coronary artery disease: a meta-analysis. BMC Med Genet. (2018) 19:108. doi: 10.1186/s12881-018-0628-3
161. Draker N, Torry DS, Torry RJ. Placenta growth factor and sFlt-1 as biomarkers in ischemic heart disease and heart failure: a review. Biomark Med. (2019) 13:785–99.
162. Alahakoon TI, Zhang W, Trudinger BJ, Lee VW. Discordant clinical presentations of preeclampsia and intrauterine fetal growth restriction with similar pro- and anti-angiogenic profiles. J Matern Fetal Neonatal Med. (2014) 27:1854–9. doi: 10.3109/14767058.2014.880882
163. Ikemoto T, Hojo Y, Kondo H, Takahashi N, Hirose M, Nishimura Y, et al. Plasma endoglin as a marker to predict cardiovascular events in patients with chronic coronary artery diseases. Heart Vessels. (2012) 27:344–51.
164. Kasam S, Shen Z, Cao H, Sudeep MV. Umbilical vein and maternal serum inhibin A, activin A, and follistatin concentrations in IUGR due to placental dysfunction pregnancies. J Obstet Gynaecol India. (2013) 63:26–31. doi: 10.1007/s13224-012-0190-4
165. Muttukrishna S, North RA, Morris J, Schellenberg JC, Taylor RS, Asselin J, et al. Serum inhibin A and activin A are elevated prior to the onset of pre-eclampsia. Hum Reprod. 2000 Jul;15(7):1640-5. Erratum Hum Reprod. (2001) 16:2477.
166. Sugatani T. Systemic activation of activin A signaling causes chronic kidney disease-mineral bone disorder. Int J Mol Sci. (2018) 19:E2490.
167. Ferrero S, Mazarico E, Valls C, Di Gregorio S, Montejo R, Ibáñez L, et al. Relationship between foetal growth restriction and maternal nutrition status measured by dual-energy X-ray absorptiometry, leptin, and insulin-like growth factor. Gynecol Obstet Invest. (2015) 80:54–9. doi: 10.1159/000371761
168. Taylor BD, Ness RB, Olsen J, Hougaard DM, Skogstrand K, Roberts JM, et al. Serum leptin measured in early pregnancy is higher in women with preeclampsia compared with normotensive pregnant women. Hypertension. (2015) 65:594–9.
169. Katsiki N, Mikhailidis DP, Banach M. Leptin, cardiovascular diseases and type 2 diabetes mellitus. Acta Pharmacol Sin. (2018) 39:1176–88.
170. Docheva N, Romero R, Chaemsaithong P, Tarca AL, Bhatti G, Pacora P, et al. The profiles of soluble adhesion molecules in the “great obstetrical syndromes”. J Matern Fetal Neonatal Med. (2019) 32:2113–36. doi: 10.1080/14767058.2018.1427058
171. Bersinger NA, Smárason AK, Muttukrishna S, Groome NP, Redman CW. Women with preeclampsia have increased serum levels of pregnancy-associated plasma protein A (PAPP-A), inhibin A, activin A and soluble E-selectin. Hypertens Pregnancy. (2003) 22:45–55.
172. Roldán V, Marín F, Lip GY, Blann AD. Soluble E-selectin in cardiovascular disease and its risk factors. A review of the literature. Thromb Haemost. (2003) 90:1007–20.
173. Pihl K, Larsen T, Krebs L, Christiansen M. First trimester maternal serum PAPP-A, beta-hCG and ADAM12 in prediction of small-for-gestational-age fetuses. Prenat Diagn. (2008) 28:1131–5. doi: 10.1002/pd.2141
174. Nakamura Y, Kita S, Tanaka Y, Fukuda S, Obata Y, Okita T, et al. A disintegrin and metalloproteinase 12 prevents heart failure by regulating cardiac hypertrophy and fibrosis. Am J Physiol Heart Circ Physiol. (2020) 318:H238–51. doi: 10.1152/ajpheart.00496.2019
175. Tsikas D, Bollenbach A, Savvidou MD. Inverse correlation between maternal plasma asymmetric dimethylarginine (ADMA) and birthweight percentile in women with impaired placental perfusion: circulating ADMA as an NO-independent indicator of fetal growth restriction? Amino Acids. (2018) 50:341–51. doi: 10.1007/s00726-017-2522-2
176. Bian Z, Shixia C, Duan T. First-trimester maternal serum levels of sFLT1, PGF and ADMA predict preeclampsia. PLoS One. (2015) 10:e0124684. doi: 10.1371/journal.pone.0124684
177. Sibal L, Agarwal SC, Home PD, Boger RH. The role of asymmetric dimethylarginine (ADMA) in endothelial dysfunction and cardiovascular disease. Curr Cardiol Rev. (2010) 6:82–90.
178. Gagnon A, Wilson RD, SOCIETY OF OBSTETRICIANS AND GYNAECOLOGISTS OF CANADA GENETICS COMMITTEE. Obstetrical complications associated with abnormal maternal serum markers analytes. J Obstet Gynaecol Can. (2008) 30:918–32.
179. Li Y, Zhou C, Zhou X, Li L, Hui R. Pregnancy-associated plasma protein A predicts adverse vascular events in patients with coronary heart disease: a systematic review and meta-analysis. Arch Med Sci. (2013) 9:389–97.
180. Matson BC, Caron KM. Adrenomedullin and endocrine control of immune cells during pregnancy. Cell Mol Immunol. (2014) 11:456–9.
Keywords: maternal hemodynamic changes in pregnancy, fetal growth, intravascular volume, cardiac output, venous hemodynamics, vascular resistance, body water volume
Citation: Gyselaers W and Lees C (2022) Maternal Low Volume Circulation Relates to Normotensive and Preeclamptic Fetal Growth Restriction. Front. Med. 9:902634. doi: 10.3389/fmed.2022.902634
Received: 23 March 2022; Accepted: 04 May 2022;
Published: 09 June 2022.
Edited by:
Javier Caradeux, Santa Maria Clinic, ChileReviewed by:
Emma Janssen, Maastricht University Medical Centre (MUMC), NetherlandsRalph Scholten, Radboud University Nijmegen Medical Centre, Netherlands
Herbert Valensise, University of Rome Tor Vergata, Italy
Copyright © 2022 Gyselaers and Lees. This is an open-access article distributed under the terms of the Creative Commons Attribution License (CC BY). The use, distribution or reproduction in other forums is permitted, provided the original author(s) and the copyright owner(s) are credited and that the original publication in this journal is cited, in accordance with accepted academic practice. No use, distribution or reproduction is permitted which does not comply with these terms.
*Correspondence: Wilfried Gyselaers, V2lsZnJpZWQuZ3lzZWxhZXJzQHVoYXNzZWx0LmJl