- 1Xiyuan Hospital, China Academy of Chinese Medical Sciences, Beijing, China
- 2Nankou Hospital, Beijing, China
- 3Dongfang Hospital, Beijing University of Chinese Medicine, Beijing, China
We explored the mechanisms and molecular targets of Ejiao Siwu Decoction (EJSW) for treating primary immune thrombocytopenia (ITP) using network pharmacology and molecular docking. Active compounds of EJSW were identified by high-performance liquid chromatography-diode array detector (HPLC-DAD) and high-performance liquid chromatography-mass spectrometry (HPLC-MS) and their targets were obtained from HERB and SwissTargetPrediction, and ITP targets were obtained from Comparative Toxicogenomics Database (CTD) and GeneCards. STRING and Cytoscape were used for protein-protein interaction (PPI) network analysis. Gene Ontology (GO) and Kyoto Encyclopedia of Genes and Genomes (KEGG) analyses by WebGestalt yielded a gene-pathway network, Autodock molecular docking was applied to screen targets and active compounds, and cytokines were detected using a cytometric bead array (CBA) human inflammation kit. We identified 14 compounds and 129 targets, and 1,726 ITP targets. RAC-alpha serine/threonine-protein kinase (AKT1), tumour necrosis factor (TNF), interleukin-6 (IL6), caspase-3 (CASP3) and tumour suppressor protein (TP53) were core targets (nodes and edges). Functional annotation identified cofactor binding and coenzyme binding, and 20 significantly enriched pathways. Active compounds of EJSW were successfully docked with ITP targets. Tumour necrosis factor alpha (TNF-α) and interleukin-1 beta (IL-1β) were upregulated in ITP patients, vascular endothelial growth factor A (VEGF-A) and vascular endothelial growth factor D (VEGF-D) were downregulated, and EJSW treatment reversed these trends. EJSW may regulate key ITP targets based on the in silico analyses, and protect vascular integrity through AGE-RAGE signalling, complement and coagulation cascades, and VEGF signalling by downregulating TNF-α, IL-1β and other inflammatory factors.
Highlights
- Active compounds of Ejiao Siwu (EJSW) Decoction were detected by HPLC-DAD and HPLC-MS.
- EJSW may regulate key ITP targets, and protect vascular integrity through AGE-RAGE signalling, complement and coagulation cascades, and VEGF signalling by downregulating TNF-α, IL-1β and other inflammatory factors.
- EJSW treatment reversed the expression of cytokines in patients with ITP.
Introduction
Primary immune thrombocytopenia (ITP) is an autoimmune disease characterised by platelet destruction, insufficient platelet production, high risk of bleeding, fatigue and immune dysfunction (1–5). Decreased platelet count in ITP patients is caused by pathologic antiplatelet antibodies (6), damaged megakaryocytopoiesis (7), and immune disorders such as altered T helper 1/T helper 2 balance (8), unbalanced Th17/Treg (9), and lower Breg impression (10). Glucocorticoids are still the standard first-line treatment, but they cause serious side effects following long applications (11), and second-line therapies for ITP are still lack of enough reliable data in term of long-term safety and efficacy (12).
Traditional Chinese medicine (TCM) is considered an effective auxiliary strategy for treating chronic diseases, including ITP. It can increase PLT counts and reduce bleeding with significant efficacy (13, 14) and also increase the number of granulocytes megakaryocytes (15). Ejiao Siwu (EJSW) decoction is composed of five Chinese medicinal herbs: Angelicae Sinensis Radix (ASR, the dried root of Angelica sinensis [Oliv.] Diels), Chuanxiong Rhizoma (CR, the dried rhizome of Ligusticum chuanxiong Hort.), Paeoniae Radix Alba (PRA, the dried root of Paeonia lactiflora Pall.), Rehmanniae Radix Praeparata (RRP, the dried root of Rehmannia glutinosa Libosch.), and Asini Corii Colla (ACC, a product from the hide of Equus asinus L.). It has been reported that Angelicae Sinensis Radix, Chuanxiong Rhizoma, Paeoniae Radix Alba, Rehmanniae Radix Praeparata could substantially promote the proliferation of bone marrow haematopoietic cells in anaemic mice (16). Asini Corii Colla possesses a therapeutic effect in treating various haematologic diseases, including thrombocytopenia, which increases PLT counts and stimulates the activity of bone marrow stem cells, especially megakaryocytes (17). EJSW is frequently used to treat blood deficiencies, coughing, and associated symptoms including anaemia, asthenia, dizziness, fatigue, irregular menstruation, palpitations, muscle fatigue and pale complexion. In addition, EJSW can nourish platelets, improve endothelial function, and treat ITP in TCM clinical practice. However, the mechanisms and molecular targets of EJSW for treating of ITP are not yet clear, which is the main factor limiting the application and development of EJSW worldwide.
The chemical components of EJSW were analysed by HPLC-DAD and HPLC-MS techniques. This method is reliable and reproducible, and can more accurately determine the true content of chemical components. It is widely used in laboratories for quality control of traditional Chinese medicines. Network pharmacology is a new discipline integrating systems biology and pharmacology. Using extensive biological information, existing molecular biology data are systematically and comprehensively analysed, and interaction mechanisms linking organisms and diseases are deciphered based on protein abundance, gene expression, and levels of small molecules (metabolites) (18). It can be used for the development and mechanism research of multi-target drugs (19, 20). Most previous research on the pharmacodynamic basis and mechanisms of TCM has focussed on the regulation of single targets or signalling pathways, and it has proved difficult to reveal synergistic effects between TCM components. However, network pharmacology combines drug target network and bioinformatics network analyses from a multi-target perspective, which could help to reveal complicated TCM mechanisms and assist new drug research and development (21, 22).
In the present study, a network pharmacology approach was used to explore the mechanisms and molecular targets of EJSW for the treatment of ITP. Active compounds of EJSW were identified by HPLC-DAD and LC-MS and their targets were obtained using HERB1 and SwissTargetPrediction2. ITP-related targets were then derived from Genecards and Comparative Toxicogenomics Database (CTD). The mechanisms through which EJSW treats ITP were analysed by Gene Ontology (GO) and Kyoto Encyclopedia of Genes and Genomes (KEGG) pathway analyses. The findings lay a foundation for the clinical application of EJSW to treat ITP.
Materials and Methods
Preparation and Quantitative Analysis of the Chemical Components in Ejiao Siwu by High-Performance Liquid Chromatography-Diode Array Detector and High-Performance Liquid Chromatography-Mass Spectrometry
Chinese herbal medicine pieces of ASR, CR, PRA, RRP, ACC and their precision compound granules were provided by the Chinese Pharmacy of Xiyuan Hospital of China Academy of Chinese Medical Sciences. A 2.5 g of precision compound granules were taken and added 20 ml of 50% methanol for ultrasound for 40 min. After cooling, it was weighted with 50% methanol. The supernatant was taken and injected through 0.22 μm filter membrane for quantitative analysis by HPLC-DAD. The contents of gallic acid, albiflorin, paeoniflorin, senkyunolide I, ligustilide, oxidised paeoniflorin, catechin, caffeic acid, verbascoside, chlorogenic acid, alanine, L-proline, glycine and L-hydroxyproline as the markers were detected.
The chemical analysis was conducted on a high performance liquid chromatograph (HPLC, waters-2695, Waters Corporation, Milford, MA, United States) equipped with DAD detector. The column (Diamonsil C18, 250 × 4.6 mm, 5 μm) was used for chromatographic separation. The mobile phase was acetonitrile (B), 0.1% phosphoric acid aqueous solution (A), with a gradient elution procedure (0–3 min, 95%A; 3–15 min, 95–85%A; 15–40 min, 80%–0 A; 40–45 min, 0 A; 45–46 min, 0–95%A; 46–50 min, 95%A). The data was collected by scanning at full wavelength, and chromatograms with detection wavelengths of 276 and 324 nm were extracted, respectively. The flow rate was 1 ml/min, and the column temperature was 35°C, and the injection volume was 10 μL.
The powder of Ejiao was weighed for 2.69 g, accurately added with 25 ml of pure water, weighed, sonicated for 30 min, cooled, weighed, supplemented with pure water, shaken, filtered, and used for HPLC-MS.
The liquid system is Shimadzu ultra-high performance liquid chromatograph (Shimadzu LC30), and the mass spectrometry system is SCIEX 5,600 + mass spectrometer (United States, AB Sciex Instruments, Model TripleTOF 5,600 + Hybrid Quadrupole-TOF LC/MS/MS Mass Spectrometer). The chromatographic column: ACQUITY UPLC HILIC Column 1.7 μm, 2.1 mm × 100 mm; mobile phase: A is 0.1% formic acid water, B is acetonitrile, and the elution program is shown in the following Table 1. The column temperature is 35°C, the sampler temperature is 4°C, and the injection volume is 10 μl.
Mass spectrometry conditions: the mass spectrometry adopts positive ion mode (ESI+), independent data acquisition mode (IDA), the collision voltage was 35 V, the capillary voltage was 5,500 v (positive ion), the ion source temperature was 550°C (positive ion), and the de clustering voltage was 60 V. The curtain gas flow was 25 l/min, the gas flow of Gas 1 and Gas 2 were both 50 l/min, and all gases were nitrogen.
Obtaining Immune Thrombocytopenia-Related Targets
GeneCards (23)3 and CTD (24, 25)4 were used to identify targets related to ITP, and results overlapping both databases were selected as ITP-related targets.
Network Construction
A disease-compound-target network was constructed and visualised using Cytoscape 3.7.15 open source bioinformatics analysis software. In the resulting structured network nodes represent genes, proteins or molecules and edges between nodes represent interactions between these biomolecules. The degree of a node represents the connection between nodes in the network. The larger the number and degree, the more likely the target is to be a key target for compounds (26). Protein-protein interaction (PPI) data were obtained from the STRING database6, the protein type was set to Homo sapiens, and the lowest interaction threshold was set to medium confidence >0.4 (27). The other parameters were set by default to generate the PPI network, and the top 20 core targets from PPI networks were visualised with R software (Version 3.5.37).
Bioinformatic Analysis
Gene Ontology analysis of biological process (BP), cellular component (CC) and molecular function (MF) categories was carried out using the WebGestalt web-based gene set analysis toolkit8 (28). These functional categories were enriched within various genes (p < 0.05), relationships between genes and GO terms were visualised using a chord picture, and relationships among enriched GO terms were visualised using a DAG picture. WebGestalt assigned the KEGG database for pathway analysis, and pathways with significant changes (p < 0.05) were subjected to further analysis. Visualisation of the results of GO and KEGG analyses was constructed by Bioconductor (version 3.89) within R (version 3.5.3). Genes that significantly regulated pathways were selected for gene-pathway network analysis, and a gene-pathway network was constructed to screen key target genes through which EJSW treats ITP.
Molecular Docking of Active Compounds and Core Targets
The 3D structures of active compounds were obtained through the PubChem database10. Crystal structures of core target proteins were downloaded from the PDB database11, and operations such as dewatering, removing original ligands, adding hydrogens, and calculating charges were performed using PyMOL (version 2.4.012) and autodocktools (version 1.5.613). Molecular docking was performed using autodock Vina14, and PyMOL was employed for visualisation.
Verification of Network Pharmacology-Based Screening Results
In order to confirm the screening results based on network pharmacology, various cytokines including IL-1β, TNF-α, VEGF-A, and VEGF-D were assessed. Twenty-two ITP patients and 15 healthy volunteers (HV) were recruited from Xiyuan Hospital of China Academy of Chinese Medical Sciences (Beijing, China). All patients were treated with EJSW for 2 months. After this period, 2 mL of peripheral blood from ITP patients and HV group members was collected, centrifuged (1,500 rpm, 10 min), and the resulting serum was to assess IL-1β, TNF-α, VEGF-A and VEGF-D by a cytometric bead array (CBA) human inflammation kit according to the manufacturer’s instructions (BD PharMingen, San Diego, CA, United States) (29). Analysis was performed using the AimPlex Bead-based multiparametric flow cytometry instrument (EPICS-Elite, Beckman-Coulter, United States).
This study was approved by the Clinical Research Ethics Committee of Xiyuan Hospital, China Academy of Chinese Medical Sciences (Ethics approval number: 2015XLA108) and was conducted in compliance with the Declaration of Helsinki. All analyses were performed using SPSS 23.0 (SPSS Inc., Chicago, IL, United States). The threshold of significance was defined as two-sided p < 0.05.
Results
Identification of the Active Compounds in Ejiao Siwu by High-Performance Liquid Chromatography-Diode Array Detector and High-Performance Liquid Chromatography-Mass Spectrometry
We obtained a total of 14 active compounds of EJSW and they were gallic acid, albiflorin, paeoniflorin, senkyunolide I, ligustilide, oxidised paeoniflorin, catechin, caffeic acid, verbascoside, chlorogenic acid, alanine, L-proline, glycine and L-hydroxyproline. Among them, 10 active compounds including gallic acid, albiflorin, paeoniflorin, senkyunolide I, ligustilide, oxypaeoniflorin, catechin, caffeic acid, verbascoside, and chlorogenic acid were detected by HPLC-DAD, as shown in Figure 1. A shows the chromatogram of EJSW detected at 276 nm, and B shows the chromatogram of EJSW at 234 nm. The content of other four active compounds (alanine, L-proline, glycine and L-hydroxyproline) in ACC were 28.611, 11.575, 4.749, and 1.989 μg/g, as shown in Figure 2.
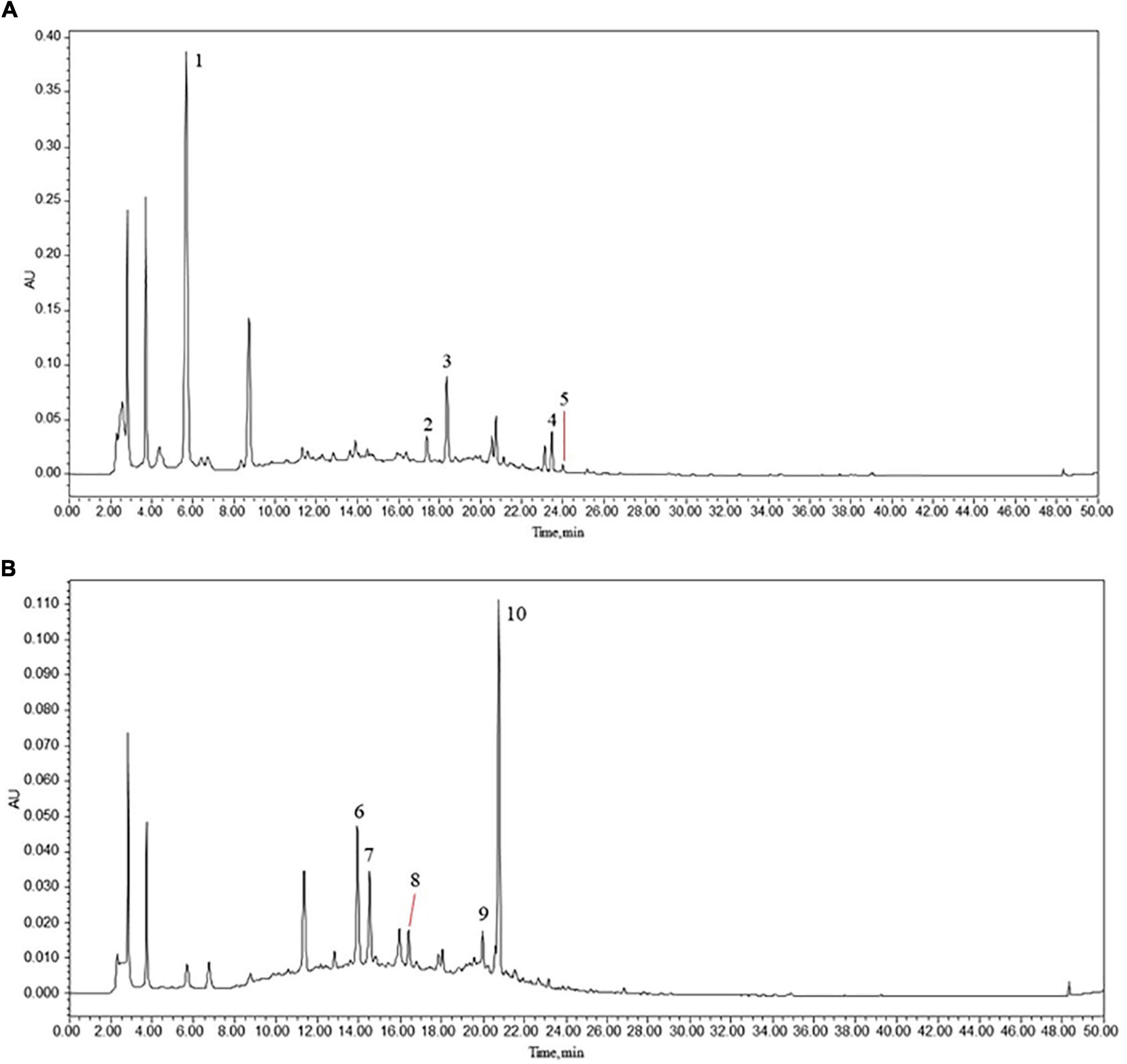
Figure 1. (1) Gallic acid, (2) albiflorin, (3) paeoniflorin, (4) senkyunolide I, (5) ligustilide, (6) oxypaeoniflorin, (7) catechin, (8) caffeic acid, (9) verbascoside, and (10) chlorogenic acid. HPLC-DAD chromatograms of various constituents in EJSW. (A) 276 nm. (B) 234 nm.
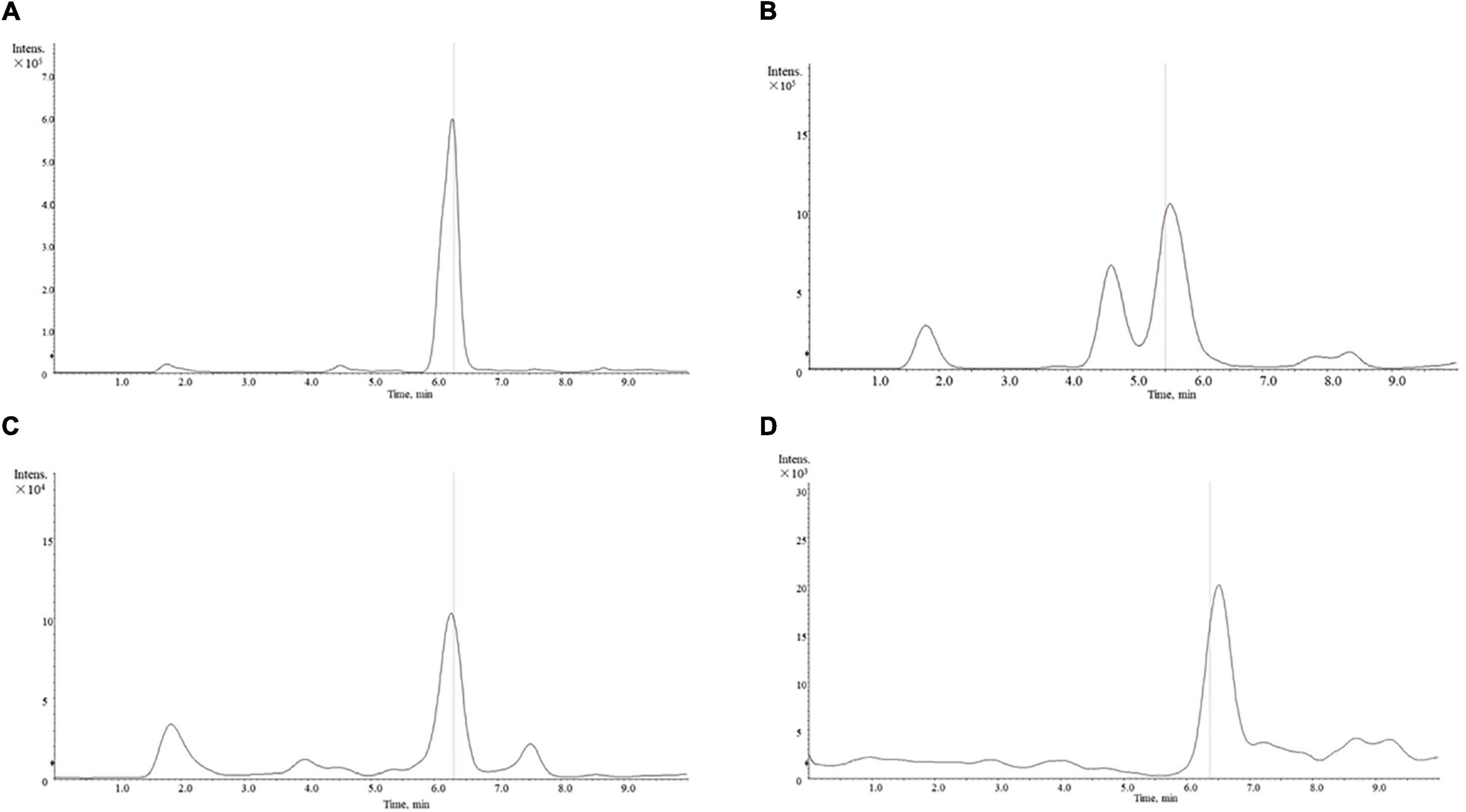
Figure 2. Mass spectrum of amino acid content in ACC. (A) Alanine. (B) L-proline. (C) L-hydroxyproline. (D) Glycine.
Compound-Target Network Analysis
Active compounds of EJSW were identified by HPLC-DAD and LC-MS and their targets were obtained using HERB (see text footnote 1) and SwissTargetPrediction (see text footnote 2). Eleven of the 14 active compounds had targets, and the remaining 3e had no corresponding targets. Overlapping results from Gene Cards and CTD databases yielded 1726 ITP-related targets (Figure 3), and 58 common targets of ITP and EJSW were identified. A disease-compound-target network for EJSW treatment of ITP was constructed from the screened compounds and their targets (Figure 4). The network contained 76 nodes and 161 edges representing compound-target interactions.
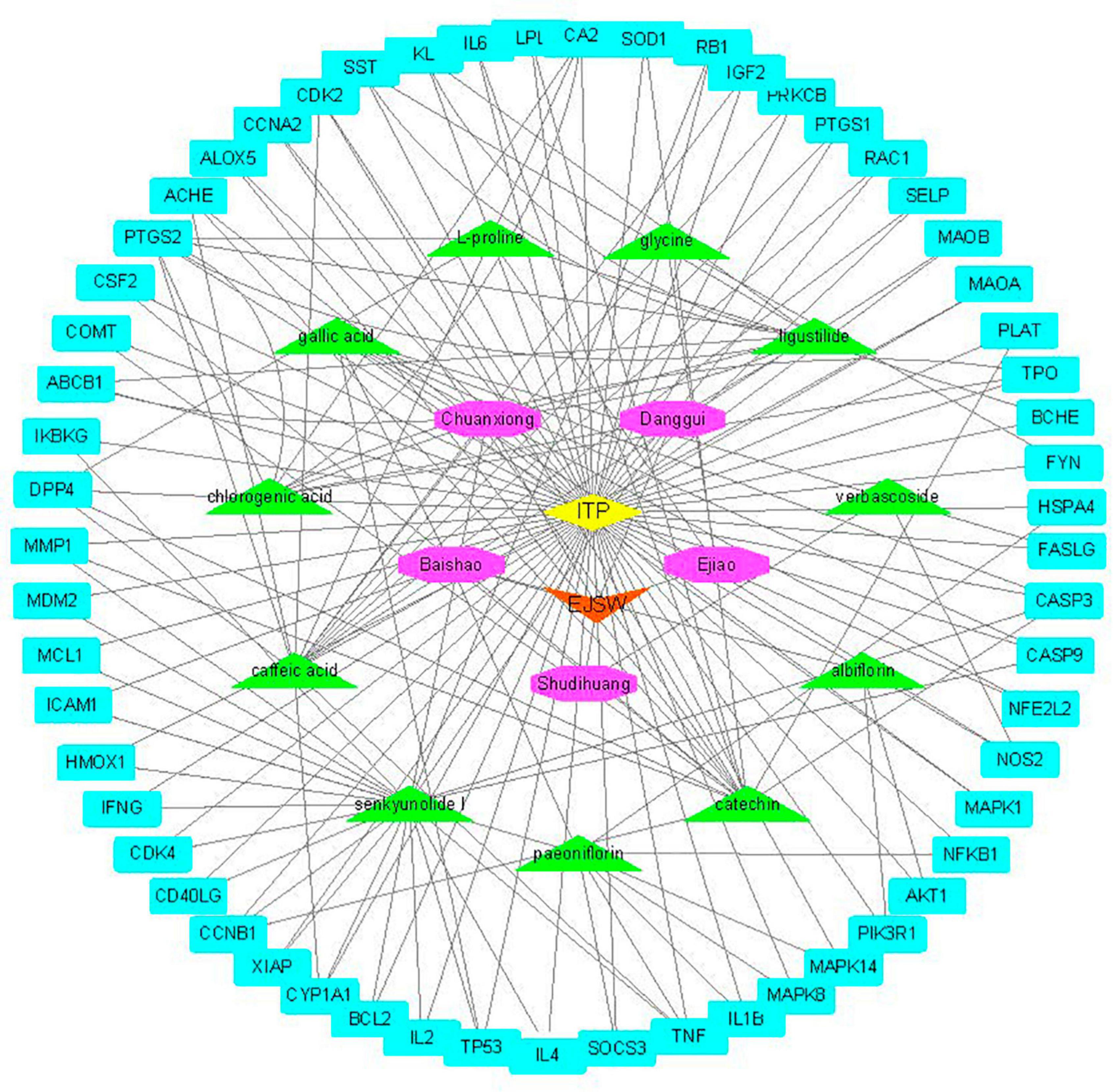
Figure 4. Disease-compound-target network of EJSW against. The blue rectangles represent targets. The green triangles represent the compounds. The purple octagons represents the five herbs of EJSW. The orange triangle represents EJSW and the yellow diamond represents ITP.
Protein-Protein Interaction Networks Analysis
Protein-protein interactions are crucial for many biological processes including cell-to-cell interactions, metabolic control and developmental regulation, and studying PPIs is a primary objective of system biology (30). Therefore, a PPI network of putative ITP-related EJSW targets obtained from in silico analyses was generated, which included 58 nodes and 518 edges (Figure 5A). As shown in Figures 5B,C, the top 20 targets were represented as nodes and edges, and some targets including AKT1, TNF, IL-6, CASP3, and TP53 were strongly linked to EJSW treatment of ITP.
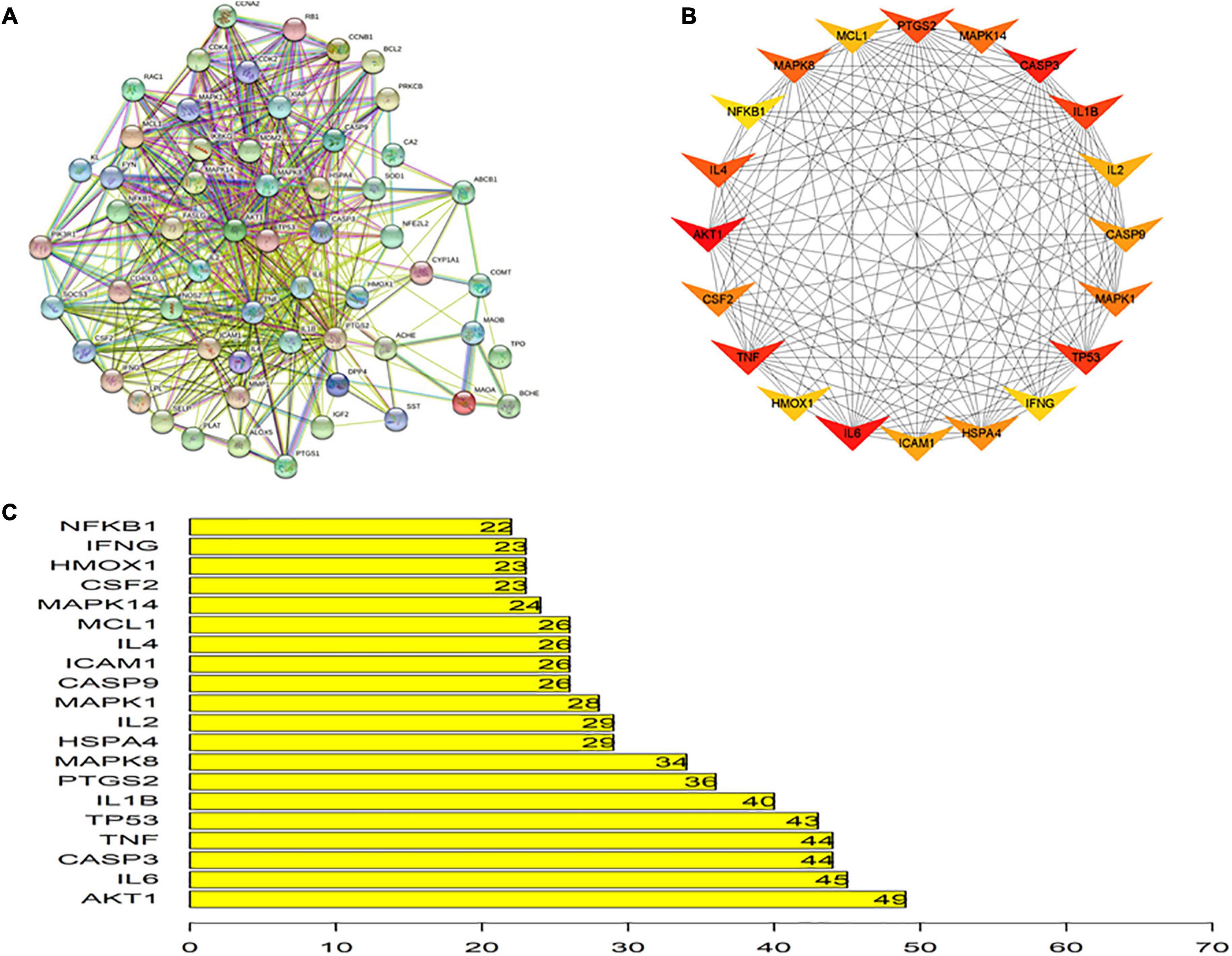
Figure 5. Protein-protein interaction network. (A) Protein-protein interaction network of putative targets of EJSW against ITP. (B) Top 20 of related targets. (C) The rank of top 20 related targets.
Biological Process Analysis
The results of GO and KEGG pathway enrichment analyses (top 20, p < 0.05) are shown in Figures 6, 7. Highly enriched GO terms included cofactor binding, coenzyme binding, carboxylic acid binding, organic acid binding, amide binding, vitamin binding, and serine hydrolase activity. Analysis of hierarchical relationships for GO terms showed that small-molecule metabolic process was upstream and cellular amino acid metabolic process was downstream (Figure 6C).
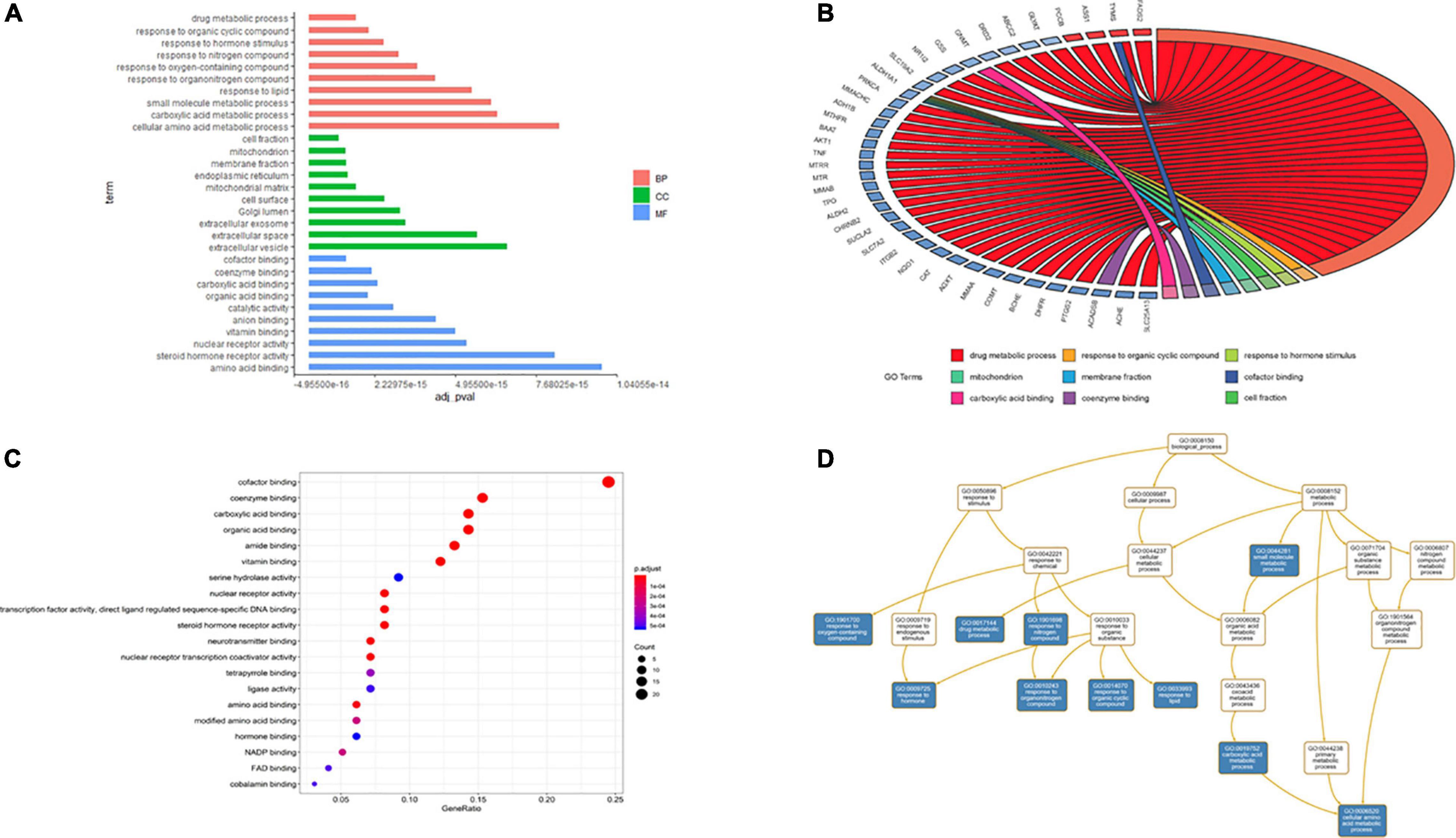
Figure 6. Gene ontology terms of candidate targets of EJSW against ITP. (A) The results of enrichment analysis containing BP, CC, MF. (B) The relationship of genes and GO terms (top3 of BP, CC and MF) based on the results of GO analysis. (C) The top 20 GO functional categories with p < 0.05 were selected. (D) The hierarchical relationships of GO terms enrichment.
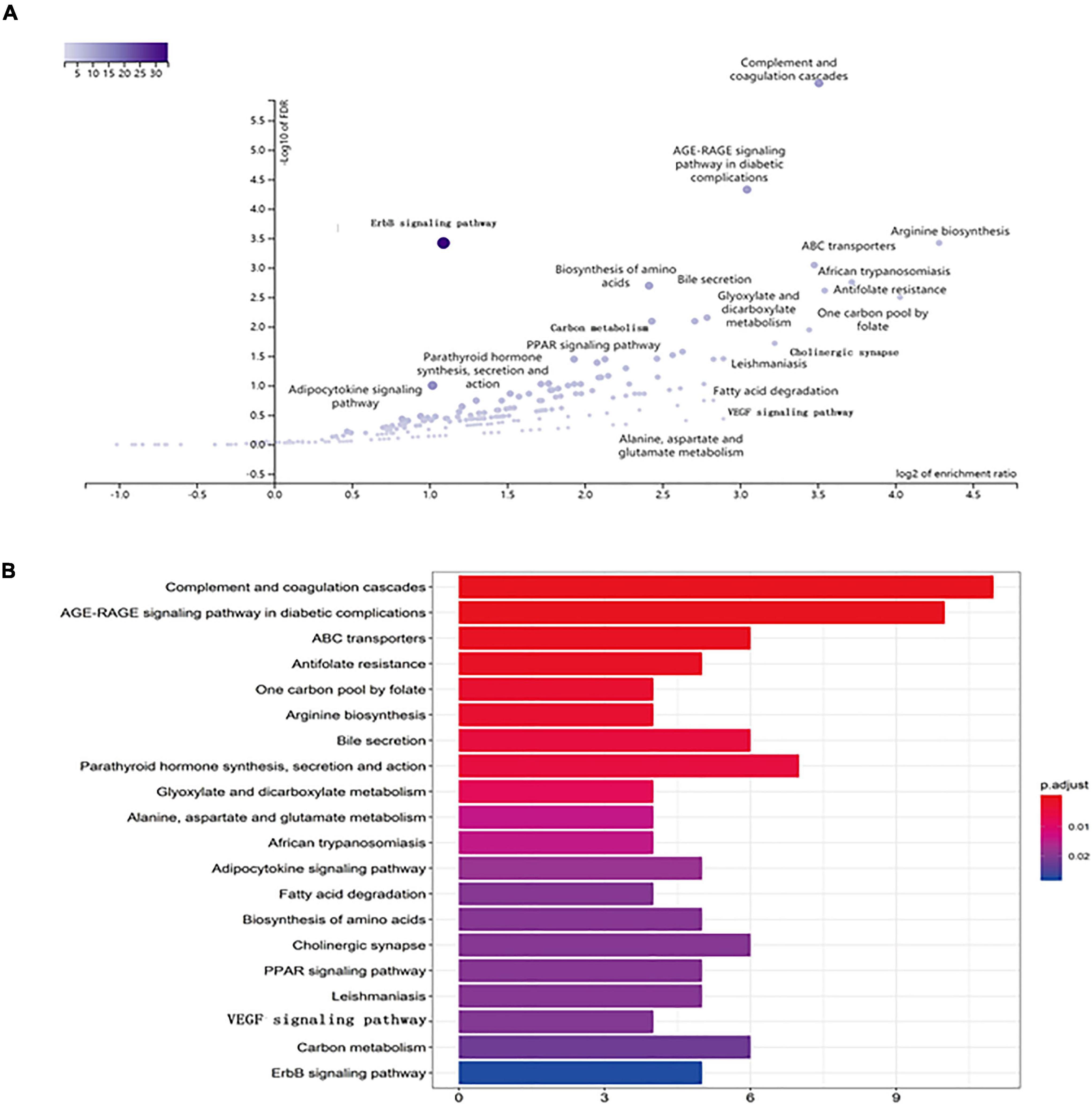
Figure 7. KEGG pathway enrichment of candidate targets of EJSW against ITP. (A) The volcano plot of KEGG pathway enrichment. (B) The top 20 pathways with p < 0.05 were selected.
Kyoto Encyclopedia of Genes and Genomes pathway analysis was performed to identify pathways via which EJSW influenced ITP treatment. Twenty significantly enriched pathways were identified (p < 0.05), including complement and coagulation cascades, AGE-RAGE signalling in diabetic complications, ABC transporters, antifolate resistance, one-carbon pool related to folate, arginine biosynthesis, bile secretion, parathyroid hormone synthesis, secretion and action, glyoxylate and dicarboxylate metabolism, and VEGF signalling. In Figure 7 the length of the bar represents the number of genes and the colour represents the p-value. The distribution of targets in the 20 pathways through which EJSW affects ITP treatment are shown in Figure 8. The main targets of EJSW against ITP are associated with the complement and coagulation cascade signalling pathway (Figures 8, 9). In Figure 9, red squares represent targets of EJSW against ITP.
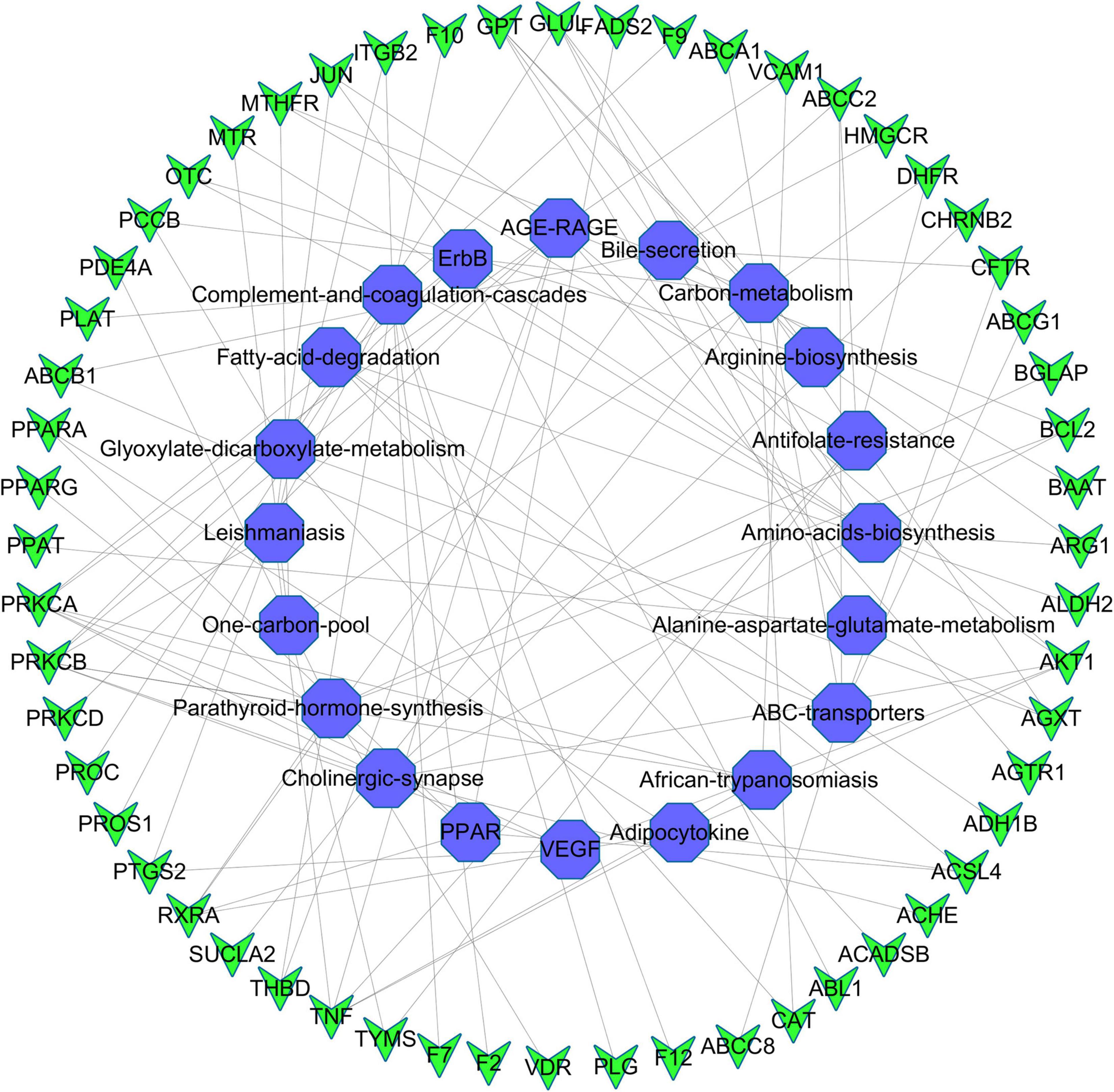
Figure 8. The top 20 pathways and the related gene targets by KEGG analysis. Purple symbols represent pathways and green were gene targets.
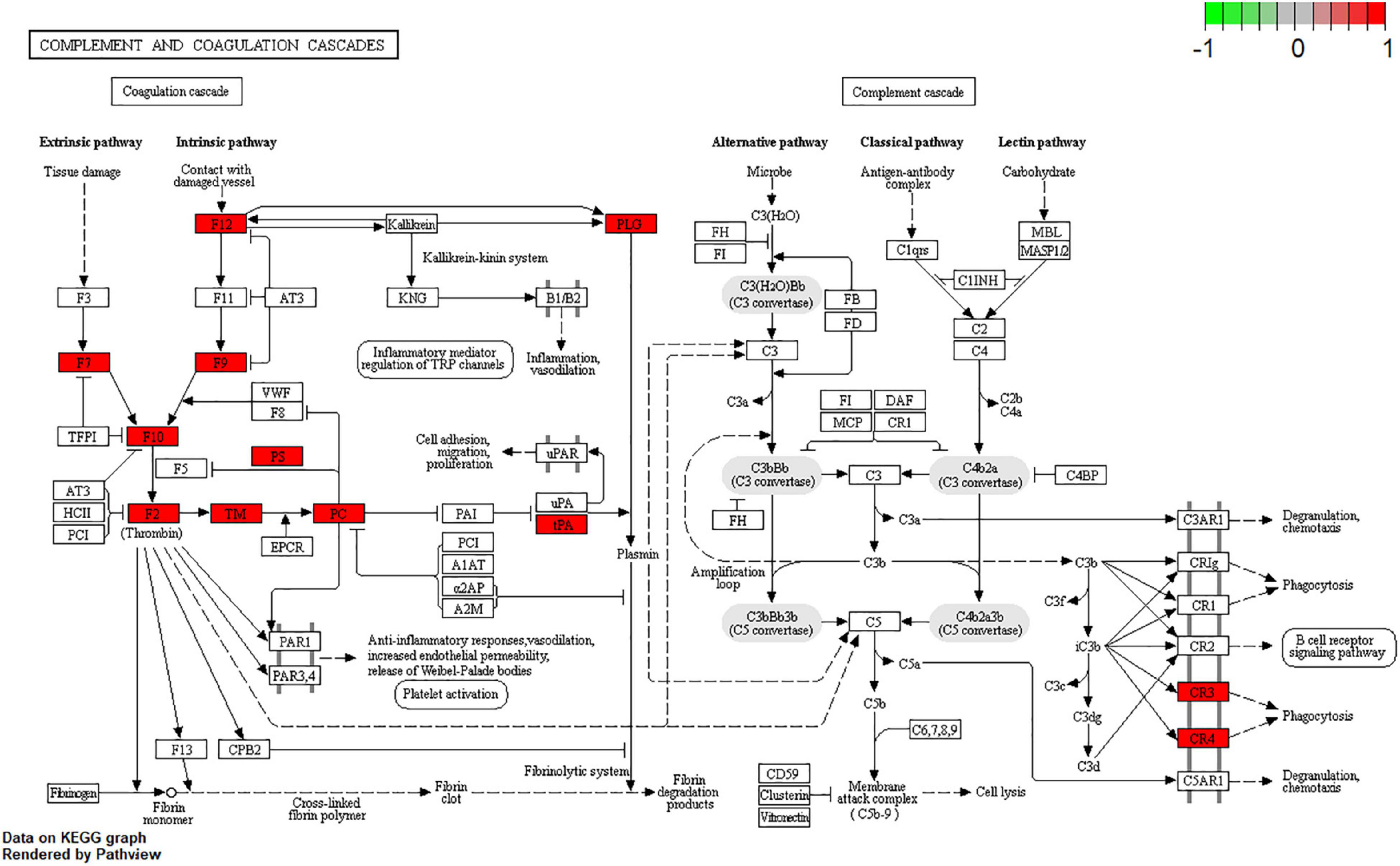
Figure 9. Complement and coagulation cascades signalling pathway. The red square represents targets of EJSW against ITP.
Molecular Docking
Five core targets (AKT1, TNF, IL-6, CASP3, and TP53) identified in the PPI network were validated by molecular docking with key compounds in EJSW. The results showed that the binding affinities of six key compounds to the core targets were below −5 kcal/mol (Table 2), which suggests that these six key components bind strongly to their core targets of ITP with high pharmacodynamic activity. These strong affinities suggest that EJSW may play a role in the treatment of ITP by acting on these core targets. The docking results for key compounds and core targets are shown in Figure 10.
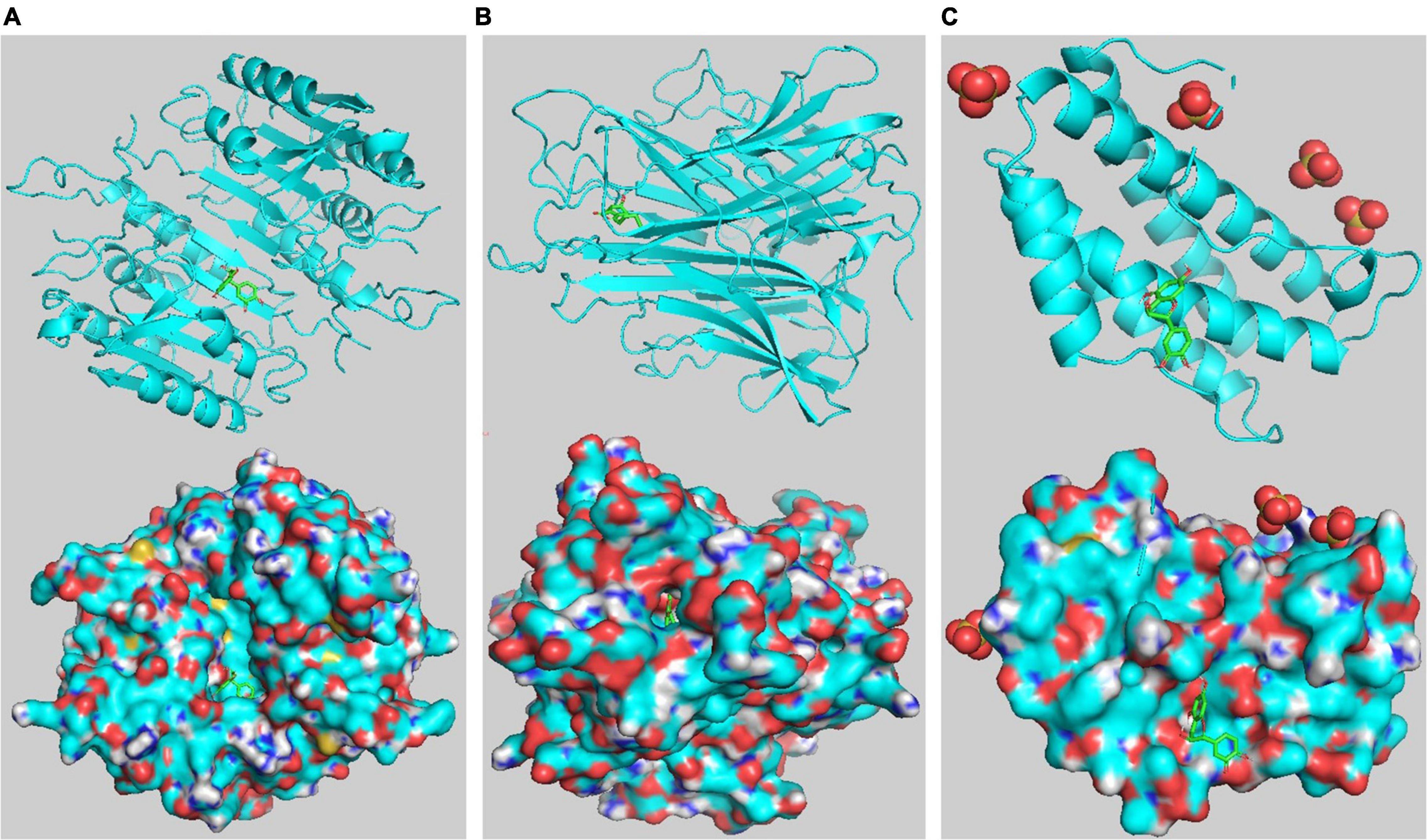
Figure 10. Target-compound interactions with the three highest molecular docking affinities. (A) CASP3- catechin. (B) TNF-senkyunolide I. (C) IL6- catechin. On the top shows the 3D structure of ligands and receptors, at the bottom shows the surface of the receptor and 3D structure of the ligands.
Detection of Cytokines, Platelet Counts and Observation of Bleeding
We measured TNF-α, IL-1β, VEDF-A, and VEGF-D expression levels in ITP patients (before and after treatment by EJSW) and HV group members. As shown in Figure 11, expression of TNF-α (7.87 ± 5.59 vs. 2.04 ± 0.23) and IL-1β (14.11 ± 2.35 vs. 2.50 ± 0.67) was increased in ITP patients, while expression of VEGF-A (11.44 ± 5.23 vs. 35.59 ± 14.73) and VEGF-D (4.15 ± 2.10 vs. 10.46 ± 5.16) was decreased compared with HV. By contrast, after treatment with EJSW for 2 months, expression of TNF-α (4.05 ± 2.42 vs. 7.87 ± 5.59) and IL-1β (5.20 ± 1.65 vs. 14.11 ± 2.35) was decreased, while expression of VEGF-A (23.70 ± 14.40 vs. 11.44 ± 5.23) and VEGF-D (7.06 ± 3.85 vs. 4.15 ± 2.10) was increased. However, expression of TNF-α (4.05 ± 2.42 vs. 2.04 ± 0.23) and IL-1β (5.20 ± 1.65 vs. 2.50 ± 0.67) was still higher and expression of VEGF-A (23.70 ± 14.40 vs. 35.59 ± 14.73) and VEGF-D (7.06 ± 3.85 vs. 10.46 ± 5.16) was still lower than in the HV group.
Meanwhile, we measured the platelet counts of these 22 ITP patients before and after EJSW treatment. As shown in Figure 12A, platelet counts were significantly higher in patients with ITP after treatment with EJSW compared to before treatment (60 ± 11.63 vs. 31 ± 8.22, p < 0.05). We also recorded bleeding in ITP patients before and after treatment with EJSW. WHO Bleeding Scale were used to document and evaluate bleeding in patients. As shown in Figure 12B, the number of patients with bleeding was significantly reduced and bleeding was significantly improved with EJSW compared to before treatment [grade 0: 81.8% (18/22) vs. 50% (11/22); grade 1: 18.2% (4/22) vs. 36.4%(8/22); grade 2: 0%(0/22) vs. 13.6%(3/22), χ2 = 6.023, p < 0.05].
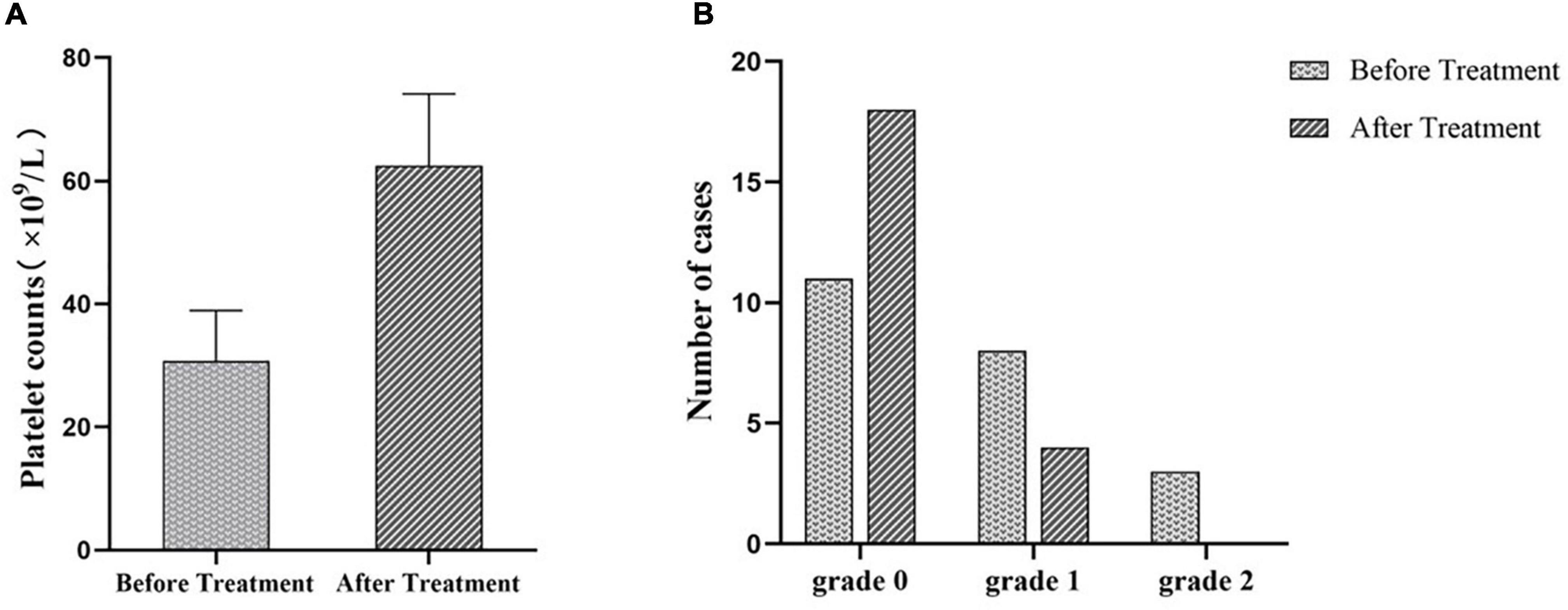
Figure 12. PLT counts and bleeding in patients with ITP after treatment with EJSW. (A) The platelet counts of ITP patients before and after treatment. (B) The number of cases of ITP patients with bleeding. Grade 0 = no bleeding; Grade 1 = petechiae; Grade 2 = mild blood loss.
Discussion
Immune thrombocytopenia is an autoimmune hemorrhagic disease. Specifically, the destruction of platelets by the monocyte-macrophage system in ITP patients, resulting from the production of anti-platelet auto-antibodies, is relevant to immune disorders. However, a decrease in platelet count increases the risk of bleeding, and ITP patients present with petechiae in the skin and other bleeding symptoms, such as epistaxis and gingival bleeding. Purpura and ecchymosis can occur in any part of the skin or mucosa, and commonly in the lower extremities and distal upper extremities. Although glucocorticoids have many undesirable side effects, such as osteoporosis, femoral head necrosis, and psychiatric symptoms, they remain the first-line treatment for ITP (31, 32). However, TCM offers a holistic approach for ITP patient treatment and care. TCM believes that disease is the result of internal imbalance between the body’s energy (Yang) and a given substance (Yin) (15), and it rebalances energy and metabolic functions. Therefore, multi-ingredient TCM formulae are particularly suited for the treatment of complex diseases (33). The idea of network pharmacology is basically consistent with the holistic view of TCM, which helps to systematically and comprehensively explain the mechanism by which EJSW treats ITP.
The medical theory system of TCM has been used for thousands of years to treat diseases in China. Combining two or more compatible TCMs can typically improve the therapeutic effect in clinical treatment through synergism. According to TCM theory, ITP is thought to be a disease characterised by bleeding and blood deficiency, hence haemostasis and blood enrichment are necessary to treat ITP. However, it is common knowledge that platelets influence haemostasis. Thus, promoting platelet production is as important as preventing platelet destruction in view of modern medicine.
We identified 14 compounds in EJSW, of which gallic acid was able to intervenes in major inflammatory pathobiologies (34). Platelet-activating factor (PAF) is a potent inflammatory agonist while gallic acid partially protected mice from platelet-activating factor PAF-induced death (35). Caffeic acid inhibits IL-1β induced inflammation responses through suppression of NF-κB and MAPK related JNK signalling pathways (36). Verbascoside could also inhibit oxidative stress and inflammation (37, 38).
A network pharmacology approach can be used to probe the multi-component and multi-target characteristics of TCM compounds. In the present study, this technique was used to explore the mechanisms of EJSW in the treatment of ITP. The results showed a complex and interlaced network among target proteins of EJSW. Fifty-eight common targets of EJSW related to ITP were identified, 20 of which were major targets, including AKT1, IL6, CASP3, TNF, TP53, IL1B, PTGS2, MAPK8, HSPA4, IL2, MAPK1, CASP9, ICAM1, IL4, MCL1, MAPK14, CSF2, HMOX1, IFNG, and NFKB1, according to cutoff scores.
AKT1 can influence platelet activation (39), while TNF is involved in the regulation of a wide spectrum of biological processes including cell proliferation, differentiation, apoptosis, lipid metabolism and coagulation, and it has been implicated in autoimmune diseases (40). IL6 is a very important factor in determining Th17/Treg balance, which acts as a potent pro-inflammatory cytokine in T cells through promotion of Th17 differentiation and inhibiting Treg differentiation, and the control of IL-6 normalises the balance between Th17 and Treg and may alleviate autoimmune symptoms (41). CASP3, a major regulator of apoptosis, is a well-known execution protease, responsible for the final steps in the apoptotic pathway (42). And studies have demonstrated the presence of elevated apoptosis markers-activated caspase-3 in platelets and megakaryocytes from ITP patients, which could be reversed by treatment with intravenous immunoglobulin and prednisone (43, 44). TP53 is also associated with inflammation. Chronic inflammation in the presence of TP53 is inefficient at inducing carcinogenesis, on the contrary, chronic inflammation in the absence of TP53 generates acinar, ductal, neuroendocrine and sarcomatoid tumours (45).
Molecular docking validation indicated strong binding between key compounds and their targets. These targets are involved in multiple functions in diverse biological processes, and the functions of the main targets can be summarised as inflammatory responses, angiogenesis, vascular integrity, and platelet apoptosis.
We performed GO analysis of the targets for EJSW against ITP. The results of enrichment and target attribution analyses showed that EJSW regulates complex biological processes, including metabolic processes, biological regulation, and multicellular organismal processes. Enriched cellular components included membrane, membrane-enclosed lumen and vesicle subcategories. The main molecular functions included protein binding, ion binding, nucleotide binding and hydrolase activity.
Thus, EJSW regulates some key steps in metabolic and immune function processes, such as cofactor binding, carboxylic acid binding, transcription factor activity, direct ligand regulation, nuclear receptor activity, coenzyme binding, vitamin binding, organic acid binding, and others. These processes may be linked to immune disorders, vascular endothelium defects, and platelet apoptotic processes.
Immune thrombocytopenia is a type of autoimmune disease. Some genetic diseases can also lead to abnormal immune regulation and developed autoimmune haematological disorders, such as haemolytic anaemia and immune thrombocytopenia. But the genetic symptoms often have typical features and a clear genetic basis (46), which is not completely consistent with ITP we discussed. The present results showed that abnormal T-cell immunity leading to platelet destruction and platelet dyspoiesis is one of the main pathogenic mechanisms of ITP. Expression of Th1/Th2 as well as Th17/Treg are obviously imbalanced in ITP patients, and Th1 and Th17 are upregulated while Th2 and Treg are downregulated compared with healthy people (8, 47, 48). In addition, Th1 and Th17 are believed to induce inflammation while Th2 and Treg inhibit inflammation. When ITP occurs, the body is in an inflammatory state (49). Research shows that disorder of the apoptotic process also results in the pathogenesis of ITP (50). Unbalanced T-cells can also lead to disorder of apoptotic processes. The pathogenesis of ITP has been linked to gene expression, regulation of apoptosis, regulation of cell proliferation, nucleoplasm, transcription factor binding, histone deacetylase binding, protein kinase binding, and core promoter binding (51, 52), all of which were significantly enriched in the present study. Therefore, EJSW may treat ITP by regulating the pathogenesis of ITP.
The results of KEGG pathway analysis also showed that EJSW influences ITP through multiple pathways, including complement and coagulation cascades, AGE-RAGE signalling in diabetic complications, ABC transporters, antifolate resistance, ErbB signalling, and VEGF signalling. These pathways are closely related to cancer, metabolism, angiogenesis, inflammation, autoimmune diseases, coagulation, and other processes.
Complement and coagulation cascades are intimately associated with inflammatory responses and ensuring haemostatic maintenance (53). Indeed, the complement system plays a key role in components related to innate immunity. It shares numerous interactions with components of the haemostatic pathway, and organisms contain wounds and limit bleeding via by employing innate defence mechanisms (54, 55). It has been found that classical complement pathway can be activated by platelet autoantibodies and lead to the development of ITP (56–58), and complement activation is strongly associated with the severity of ITP (59). Therefore, complement pathway have become a new target for the treatment of ITP, and clinical trials have proven that classical complement pathway inhibitors can improve platelet counts in patients with chronic ITP with rapid and durable responses (60, 61). These fully illustrate that the complement pathway plays an important role in the pathogenesis of ITP.
The AGE- RAGE signalling pathway has strong links with vascular injury and inflammatory reactions (62, 63). Inflammatory factors in this signalling pathway, such as TNF and IL-1B, are related to vascular injury. In fact, these inflammatory factors can be considered inducers of platelet activation and immune imbalance in ITP (49, 64, 65). Retrospective correlation with clinical data suggests that platelet clearance is associated with inflammatory mediators (66, 67). Platelets are believed to play a central role in repairing vascular damage and stopping acute blood loss by tethering and adhering to sites of injury, recruiting other platelets and blood cells to the developing clot, releasing vasoactive small molecules and proteins, and assembling and activating plasma coagulation proteins in a tightly regulated temporal and spatial manner (68). In other words, platelets are essential for the maintenance of vascular endothelial integrity. Research has also shown that platelets are important mediators of inflammation, and they serve as important inducers of vascular cells via the complement system (69, 70).
The VEGF signalling pathway is mainly associated with angiogenesis. Vascular endothelial growth factors (VEGFs) constitute a sub-family of growth factors that stimulate the growth of new blood vessels (71). VEGFs are important signalling proteins involved in both vasculogenesis (the de novo formation of the embryonic circulatory system) and angiogenesis (the growth of blood vessels from pre-existing vasculature) (72). VEGF-A was the first member of the VEGF family to be identified, and the family also includes VEGF-B, VEGF-C, VEGF-D, and placenta growth factor (PlGF) (73, 74). VEGFs can reflect the state of blood vessel networks, and are closely linked to bleeding issues.
Thus, inflammatory factors, VEGFs, and immune imbalance are all correlated with the development of ITP. According to TCM theory, another function of EJSW is tonifying blood and arresting bleeding. The functions of the above target genes and signalling pathways conform to TCM theory. Therefore, the opinion that downregulating inflammatory factors is helpful to reduce the risk of bleeding is supported, due to restoration of immune balance and decreased platelet apoptosis. EJSW may treat ITP by inhibiting the expression of TNF-α, IL-1β, and other inflammatory factors, and by protecting vascular integrity through AGE-RAGE signalling, VEGF signalling, and complement and coagulation cascade signalling pathways. In the present study, TNF-α and IL-1β were upregulated in ITP, while VEGF-A and VEGF-D were downregulated. Conversely, after effective treatment with EJSW for 2 months, TNF-α and IL-1β were downregulated while VEGF-A and VEGF-D were upregulated. However, expression of TNF-α and IL-1β was still increased and expression of VEGF-A and VEGF-D was still decreased compared with normal controls. These results are consistent with those of earlier studies (75) reporting increased expressions of inflammatory factors including TNF-α and IL-1β and decreased expression of VEGF-A and VEGF-D in ITP patients. The present study also demonstrated that after treatment by EJSW, inflammation and vascular endothelial injury are relieved. Therefore, downregulating inflammatory factors can help to reduce the risk of bleeding. EJSW may treat ITP by inhibiting the expression of TNF-α, IL-1β, and other inflammatory factors, and by protecting vascular integrity through VEGF signalling, AGE-RAGE signalling, and complement and coagulation cascade signalling pathways.
However, this study has certain limitations. We only analysed data from a bioinformatic approach, and further specific in vitro and in vivo functional experiments are needed to validate the data. Overall, we explored the mechanisms and molecular targets of EJSW for treating ITP using network pharmacology and molecular docking. And our study found that the therapeutic activity of EJSW against ITP might be related to downregulating inflammatory factors and reducing vascular endothelial injury and regulating relevant pathways (VEGF signalling, AGE- RAGE signalling, and complement and coagulation cascade signalling pathways). Therefore, this study provide a scientific basis for clinical treatment of ITP, a direction for screening indicators of the follow-up clinical efficacy of EJSW, and new ideas for exploring the potential mechanisms of EJSW.
Conclusion
The therapeutic activity of EJSW against ITP might be related to downregulating inflammatory factors and reducing vascular endothelial injury, which may enhance the platelet count. The effects of EJSW may involve regulating VEGF signalling, AGE- RAGE signalling, and complement and coagulation cascade signalling pathways. The results provide a scientific basis for clinical treatment of ITP, a direction for screening indicators of the follow-up clinical efficacy of EJSW, and new ideas for exploring the potential mechanisms of EJSW. We identified some core factors, but further screening and in vivo and in vitro experiments are needed to unequivocally identify the main regulatory targets of EJSW.
Data Availability Statement
The data presented in this study are deposited in the Figshare repository: https://doi.org/10.6084/m9.figshare.20069915.v1.
Ethics Statement
The studies involving human participants were approved by the Clinical Research Ethics Committee of Xiyuan Hospital, China Academy of Chinese Medical Sciences (Ethics approval number: 2015XLA108) and was conducted in compliance with the Declaration of Helsinki.
Author Contributions
MW and XH: conceptualisation and design of the work, writing, review, and editing. MS, JM, ZW, XD, HC, XZ, and YSo: data acquisition and analysis. MW, YSu, and YSo: writing original draft preparation. All authors have read and agreed to the published version of the manuscript.
Funding
This work was supported by the National Program on Key Basic Research Project (2015CB554403).
Conflict of Interest
The authors declare that the research was conducted in the absence of any commercial or financial relationships that could be construed as a potential conflict of interest.
Publisher’s Note
All claims expressed in this article are solely those of the authors and do not necessarily represent those of their affiliated organizations, or those of the publisher, the editors and the reviewers. Any product that may be evaluated in this article, or claim that may be made by its manufacturer, is not guaranteed or endorsed by the publisher.
Acknowledgments
We thank Elixigen CO., for English checking.
Footnotes
- ^ http://herb.ac.cn/
- ^ http://www.swisstargetprediction.ch/
- ^ https://www.genecards.org/
- ^ http://systemsdock.unit.oist.jp/iddp/home/index
- ^ https://cytoscape.org/
- ^ http://string-db.org/cgi/input.pl
- ^ https://www.R-project.org
- ^ http://www.webgestalt.org
- ^ http://www.bioconductor.org/
- ^ https://pubchem.ncbi.nlm.nih.gov/
- ^ https://www.rcsb.org/
- ^ https://pymol.org
- ^ https://autodock.scripps.edu/
- ^ https://vina.scripps.edu/
References
1. Rodeghiero F, Ruggeri M. ITP and international guidelines: what do we know, what do we need? Presse Med. (2014). 43(4 Pt 2):e61–7. doi: 10.1016/j.lpm.2014.02.004
2. Ballem PJ, Segal GM, Stratton JR, Gernsheimer T, Adamson JW, Slichter SJ. Mechanisms of thrombocytopenia in chronic autoimmune thrombocytopenic purpura. Evidence of both impaired platelet production and increased platelet clearance. J Clin Invest. (1987) 80:33–40. doi: 10.1172/jci113060
3. Bussel JB, Soff G, Balduzzi A, Cooper N, Lawrence T, Semple JWA. Review of romiplostim mechanism of action and clinical applicability. Drug Des Devel Ther. (2021) 15:2243–68. doi: 10.2147/dddt.S299591
4. Chang M, Nakagawa PA, Williams SA, Schwartz MR, Imfeld KL, Buzby JS, et al. Immune thrombocytopenic purpura (ITP) plasma and purified ITP monoclonal autoantibodies inhibit megakaryocytopoiesis in vitro. Blood. (2003) 102:887–95. doi: 10.1182/blood-2002-05-1475
5. McMillan R, Wang L, Tomer A, Nichol J, Pistillo J. Suppression of in vitro megakaryocyte production by antiplatelet autoantibodies from adult patients with chronic ITP. Blood. (2004) 103:1364–9. doi: 10.1182/blood-2003-08-2672
6. Shulman NR, Marder VJ, Weinrach RS. Similarities between known antiplatelet antibodies and the factor responsible for thrombocytopenia in idiopathic purpura. Physiologic, serologic and isotopic studies. Ann N Y Acad Sci. (1965) 124:499–542. doi: 10.1111/j.1749-6632.1965.tb18984.x
7. Khodadi E, Asnafi AA, Shahrabi S, Shahjahani M, Saki N. Bone marrow niche in immune thrombocytopenia: a focus on megakaryopoiesis. Ann Hematol. (2016) 95:1765–76. doi: 10.1007/s00277-016-2703-1
8. Ge F, Zhang Z, Hou J, Cao F, Zhang Y, Wang P, et al. Granulocyte colony-stimulating factor decreases the Th1/Th2 ratio in peripheral blood mononuclear cells from patients with chronic immune thrombocytopenic purpura in vitro. Thromb Res. (2016) 148:76–84. doi: 10.1016/j.thromres.2016.10.028
9. Guo NH, Fu X, Zi FM, Song Y, Wang S, Cheng J. The potential therapeutic benefit of resveratrol on Th17/Treg imbalance in immune thrombocytopenic purpura. Int Immunopharmacol. (2019) 73:181–92. doi: 10.1016/j.intimp.2019.04.061
10. Di ZX, Geng Y, Sun XL, Su AY, Guo K, Li Q. [Expression of CD19(+)B cells and involvement of serum breg in pathogenesis of immune thrombocytopenia]. Zhongguo Shi Yan Xue Ye Xue Za Zhi. (2019) 27:911–5. doi: 10.19746/j.cnki.issn.1009-2137.2019.03.044
11. Zufferey A, Kapur R, Semple JW. Pathogenesis and therapeutic mechanisms in immune thrombocytopenia (ITP). J Clin Med. (2017) 6:16. doi: 10.3390/jcm6020016
12. Lambert MP, Gernsheimer TB. Clinical updates in adult immune thrombocytopenia. Blood. (2017) 129:2829–35. doi: 10.1182/blood-2017-03-754119
13. Huang TP, Chang YH, Chen SH, Yang SL, Yen HR. Alternative therapy for persistent childhood immune thrombocytopenic purpura unresponsive to intravenous immunoglobulin. Complement Ther Med. (2013) 21:525–8. doi: 10.1016/j.ctim.2013.08.005
14. Shao KD, Zhou YH, Shen YP, Ye BD, Gao RL, Zhang Y. Treatment of 37 patients with refractory idiopathic thrombocytopenic purpura by Shengxueling. Chin J Integr Med. (2007) 13:33–6. doi: 10.1007/s11655-007-0033-9
15. Li Y, Li Y, Lu W, Li H, Wang Y, Luo H, et al. Integrated network pharmacology and metabolomics analysis of the therapeutic effects of Zi Dian Fang on immune thrombocytopenic purpura. Front Pharmacol. (2018) 9:597. doi: 10.3389/fphar.2018.00597
16. Tian Y, Xiang Y, Wan G, Wan D, Zhu H, Wang T, et al. Effects and mechanisms of Bazhen decoction, Siwu decoction, and Sijunzi decoction on 5-fluorouracil-induced anemia in mice. J Tradit Chin Med. (2016) 36:486–95. doi: 10.1016/s0254-6272(16)30066-8
17. Wang D, Ru W, Xu Y, Zhang J, He X, Fan G, et al. Chemical constituents and bioactivities of Colla corii asini. Drug Discov Ther. (2014) 8:201–7. doi: 10.5582/ddt.2014.01038
18. Hopkins AL. Network pharmacology: the next paradigm in drug discovery. Nat Chem Biol. (2008) 4:682–90. doi: 10.1038/nchembio.118
19. Liu H, Zeng L, Yang K, Zhang GA. Network pharmacology approach to explore the pharmacological mechanism of xiaoyao powder on anovulatory infertility. Evid Based Complement Alternat Med. (2016) 2016:2960372. doi: 10.1155/2016/2960372
20. Xu T, Li S, Sun Y, Pi Z, Liu S, Song F, et al. Systematically characterize the absorbed effective substances of Wutou decoction and their metabolic pathways in rat plasma using UHPLC-Q-TOF-MS combined with a target network pharmacological analysis. J Pharm Biomed Anal. (2017) 141:95–107. doi: 10.1016/j.jpba.2017.04.012
21. Li S, Zhang B. Traditional Chinese medicine network pharmacology: theory, methodology and application. Chin J Nat Med. (2013) 11:110–20. doi: 10.1016/s1875-5364(13)60037-0
22. Zeng L, Yang K, Liu H, Zhang G. A network pharmacology approach to investigate the pharmacological effects of Guizhi Fuling Wan on uterine fibroids. Exp Ther Med. (2017) 14:4697–710. doi: 10.3892/etm.2017.5170
23. Fishilevich S, Nudel R, Rappaport N, Hadar R, Plaschkes I, Iny Stein T, et al. GeneHancer: genome-wide integration of enhancers and target genes in genecards. Database (Oxford). (2017) 2017:bax028. doi: 10.1093/database/bax028
24. Hsin KY, Ghosh S, Kitano H. Combining machine learning systems and multiple docking simulation packages to improve docking prediction reliability for network pharmacology. PLoS One. (2013) 8:e83922. doi: 10.1371/journal.pone.0083922
25. Hsin KY, Matsuoka Y, Asai Y, Kamiyoshi K, Watanabe T, Kawaoka Y, et al. systemsDock: a web server for network pharmacology-based prediction and analysis. Nucleic Acids Res. (2016) 44:W507–13. doi: 10.1093/nar/gkw335
26. Shannon P, Markiel A, Ozier O, Baliga NS, Wang JT, Ramage D, et al. Cytoscape: a software environment for integrated models of biomolecular interaction networks. Genome Res. (2003) 13:2498–504. doi: 10.1101/gr.1239303
27. Szklarczyk D, Morris JH, Cook H, Kuhn M, Wyder S, Simonovic M, et al. The STRING database in 2017: quality-controlled protein-protein association networks, made broadly accessible. Nucleic Acids Res. (2017) 45:D362–8. doi: 10.1093/nar/gkw937
28. Liao Y, Wang J, Jaehnig EJ, Shi Z, Zhang B. WebGestalt 2019: gene set analysis toolkit with revamped UIs and APIs. Nucleic Acids Res. (2019) 47:W199–205. doi: 10.1093/nar/gkz401
29. Domvri K, Porpodis K, Tzimagiorgis G, Chatzopoulou F, Kontakiotis T, Kyriazis G, et al. Th2/Th17 cytokine profile in phenotyped Greek asthmatics and relationship to biomarkers of inflammation. Respir Med. (2019) 151:102–10. doi: 10.1016/j.rmed.2019.03.017
30. Rao VS, Srinivas K, Sujini GN, Kumar GN. Protein-protein interaction detection: methods and analysis. Int J Proteomics. (2014) 2014:147648. doi: 10.1155/2014/147648
31. Arnett MG, Muglia LM, Laryea G, Muglia LJ. Genetic approaches to hypothalamic-pituitary-adrenal axis regulation. Neuropsychopharmacology. (2016) 41:245–60. doi: 10.1038/npp.2015.215
32. Lembke A, Gomez R, Tenakoon L, Keller J, Cohen G, Williams GH, et al. The mineralocorticoid receptor agonist, fludrocortisone, differentially inhibits pituitary-adrenal activity in humans with psychotic major depression. Psychoneuroendocrinology. (2013) 38:115–21. doi: 10.1016/j.psyneuen.2012.05.006
33. Zhou X, Li Y, Peng Y, Hu J, Zhang R, He L, et al. Clinical phenotype network: the underlying mechanism for personalized diagnosis and treatment of traditional Chinese medicine. Front Med. (2014) 8:337–46. doi: 10.1007/s11684-014-0349-8
34. Appeldoorn CC, Bonnefoy A, Lutters BC, Daenens K, van Berkel TJ, Hoylaerts MF, et al. Gallic acid antagonizes P-selectin-mediated platelet-leukocyte interactions: implications for the French paradox. Circulation. (2005) 111:106–12. doi: 10.1161/01.Cir.0000151307.10576.02
35. Abhilasha KV, Sumanth MS, Chaithra VH, Jacob SP, Thyagarajan A, Sahu RP, et al. p38 MAP-kinase inhibitor protects against platelet-activating factor-induced death in mice. Free Radic Biol Med. (2019) 143:275–87. doi: 10.1016/j.freeradbiomed.2019.08.019
36. Huang X, Xi Y, Pan Q, Mao Z, Zhang R, Ma X, et al. Caffeic acid protects against IL-1β-induced inflammatory responses and cartilage degradation in articular chondrocytes. Biomed Pharmacother. (2018) 107:433–9. doi: 10.1016/j.biopha.2018.07.161
37. Zhu X, Sun M, Guo H, Lu G, Gu J, Zhang L, et al. Verbascoside protects from LPS-induced septic cardiomyopathy via alleviating cardiac inflammation, oxidative stress and regulating mitochondrial dynamics. Ecotoxicol Environ Saf. (2022) 233:113327. doi: 10.1016/j.ecoenv.2022.113327
38. Zhou H, Zhang C, Huang C. Verbascoside attenuates acute inflammatory injury caused by an intracerebral hemorrhage through the suppression of NLRP3. Neurochem Res. (2021) 46:770–7. doi: 10.1007/s11064-020-03206-9
39. O’Brien KA, Stojanovic-Terpo A, Hay N, Du X. An important role for Akt3 in platelet activation and thrombosis. Blood. (2011) 118:4215–23. doi: 10.1182/blood-2010-12-323204
40. Nie H, Zheng Y, Li R, Guo TB, He D, Fang L, et al. Phosphorylation of FOXP3 controls regulatory T cell function and is inhibited by TNF-α in rheumatoid arthritis. Nat Med. (2013) 19:322–8. doi: 10.1038/nm.3085
41. Kimura A, Kishimoto T. IL-6: regulator of Treg/Th17 balance. Eur J Immunol. (2010) 40:1830–5. doi: 10.1002/eji.201040391
42. Jeelani R, Chatzicharalampous C, Kohan-Ghadr HR, Bai D, Morris RT, Sliskovic I, et al. Hypochlorous acid reversibly inhibits caspase-3: a potential regulator of apoptosis. Free Radic Res. (2020) 54:43–56. doi: 10.1080/10715762.2019.1694675
43. Houwerzijl EJ, Blom NR, van der Want JJ, Esselink MT, Koornstra JJ, Smit JW, et al. Ultrastructural study shows morphologic features of apoptosis and para-apoptosis in megakaryocytes from patients with idiopathic thrombocytopenic purpura. Blood. (2004) 103:500–6. doi: 10.1182/blood-2003-01-0275
44. Winkler J, Kroiss S, Rand ML, Azzouzi I, Annie Bang KW, Speer O, et al. Platelet apoptosis in paediatric immune thrombocytopenia is ameliorated by intravenous immunoglobulin. Br J Haematol. (2012) 156:508–15. doi: 10.1111/j.1365-2141.2011.08973.x
45. Swidnicka-Siergiejko AK, Gomez-Chou SB, Cruz-Monserrate Z, Deng D, Liu Y, Huang H, et al. Chronic inflammation initiates multiple forms of K-Ras-independent mouse pancreatic cancer in the absence of TP53. Oncogene. (2017) 36:3149–58. doi: 10.1038/onc.2016.461
46. Giordano P, Lassandro G, Sangerardi M, Faienza MF, Valente F, Martire B. Autoimmune haematological disorders in two Italian children with Kabuki syndrome. Ital J Pediatr. (2014) 40:10. doi: 10.1186/1824-7288-40-10
47. Zhou H, Qi AP, Li HY, Ma L, Xu JH, Xue F, et al. CD72 gene expression in immune thrombocytopenia. Platelets. (2012) 23:638–44. doi: 10.3109/09537104.2011.633646
48. Zhou L, Xu F, Chang C, Tao Y, Song L, Li X. Interleukin-17-producing CD4+ T lymphocytes are increased in patients with primary immune thrombocytopenia. Blood Coagul Fibrinolysis. (2016) 27:301–7. doi: 10.1097/mbc.0000000000000423
49. Dal MS, Karakus A, Aydin BB, Ekmen MO, Ulas T, Ayyildiz O. Serum uric acid and inflammation in patients with immune thrombocytopenic purpura: preliminary results. Eur Rev Med Pharmacol Sci. (2015) 19:4385–9.
50. Qian C, Yan W, Li T, Cui Q, Liu P, Gu M, et al. Differential expression of MiR-106b-5p and MiR-200c-3p in newly diagnosed versus chronic primary immune thrombocytopenia patients based on systematic analysis. Cell Physiol Biochem. (2018) 45:301–18. doi: 10.1159/000486811
51. Deng G, Yu S, He Y, Sun T, Liang W, Yu L, et al. MicroRNA profiling of platelets from immune thrombocytopenia and target gene prediction. Mol Med Rep. (2017) 16:2835–43. doi: 10.3892/mmr.2017.6901
52. Zuo B, Zhai J, You L, Zhao Y, Yang J, Weng Z, et al. Plasma microRNAs characterising patients with immune thrombocytopenic purpura. Thromb Haemost. (2017) 117:1420–31. doi: 10.1160/th-16-06-0481
53. Wiegner R, Chakraborty S, Huber-Lang M. Complement-coagulation crosstalk on cellular and artificial surfaces. Immunobiology. (2016) 221:1073–9. doi: 10.1016/j.imbio.2016.06.005
54. Conway EM. Reincarnation of ancient links between coagulation and complement. J Thromb Haemost. (2015) 13 Suppl 1:S121–32. doi: 10.1111/jth.12950
55. Keragala CB, Draxler DF, McQuilten ZK, Medcalf RL. Haemostasis and innate immunity - a complementary relationship: a review of the intricate relationship between coagulation and complement pathways. Br J Haematol. (2018) 180:782–98. doi: 10.1111/bjh.15062
56. Sun S, Urbanus RT, Ten Cate H, de Groot PG, de Laat B, Heemskerk JWM, et al. Platelet activation mechanisms and consequences of immune thrombocytopenia. Cells. (2021) 10:3386. doi: 10.3390/cells10123386
57. Najaoui A, Bakchoul T, Stoy J, Bein G, Rummel MJ, Santoso S, et al. Autoantibody-mediated complement activation on platelets is a common finding in patients with immune thrombocytopenic purpura (ITP). Eur J Haematol. (2012) 88:167–74. doi: 10.1111/j.1600-0609.2011.01718.x
58. Audia S, Bonnotte B. Emerging therapies in immune thrombocytopenia. J Clin Med. (2021) 10:1004. doi: 10.3390/jcm10051004
59. Castelli R, Lambertenghi Delilliers G, Gidaro A, Cicardi M, Bergamaschini L. Complement activation in patients with immune thrombocytopenic purpura according to phases of disease course. Clin Exp Immunol. (2020) 201:258–65. doi: 10.1111/cei.13475
60. Gavriilaki E, Peffault de Latour R, Risitano AM. Advancing therapeutic complement inhibition in hematologic diseases: PNH and beyond. Blood. (2021) 2021012860. doi: 10.1182/blood.202101286
61. Kuter DJ. Novel therapies for immune thrombocytopenia. Br J Haematol. (2022) 196:1311–28. doi: 10.1111/bjh.17872
62. Sotokawauchi A, Ishibashi Y, Matsui T, Yamagishi SI. Aqueous extract of glucoraphanin-rich broccoli sprouts inhibits formation of advanced glycation end products and attenuates inflammatory reactions in endothelial cells. Evid Based Complement Alternat Med. (2018) 2018:9823141. doi: 10.1155/2018/9823141
63. Matsui T, Oda E, Higashimoto Y, Yamagishi S. Glyceraldehyde-derived pyridinium (GLAP) evokes oxidative stress and inflammatory and thrombogenic reactions in endothelial cells via the interaction with RAGE. Cardiovasc Diabetol. (2015) 14:1. doi: 10.1186/s12933-014-0162-3
64. Davizon-Castillo P, McMahon B, Aguila S, Bark D, Ashworth K, Allawzi A, et al. TNF-α-driven inflammation and mitochondrial dysfunction define the platelet hyperreactivity of aging. Blood. (2019) 134:727–40. doi: 10.1182/blood.2019000200
65. Zimmerman GA, McIntyre TM, Prescott SM, Stafforini DM. The platelet-activating factor signaling system and its regulators in syndromes of inflammation and thrombosis. Crit Care Med. (2002) 30(5 Suppl.):S294–301. doi: 10.1097/00003246-200205001-00020
66. Peerschke EI, Yin W, Ghebrehiwet B. Complement activation on platelets: implications for vascular inflammation and thrombosis. Mol Immunol. (2010) 47:2170–5. doi: 10.1016/j.molimm.2010.05.009
67. Qu M, Liu Q, Zhao HG, Peng J, Ni H, Hou M, et al. Low platelet count as risk factor for infections in patients with primary immune thrombocytopenia: a retrospective evaluation. Ann Hematol. (2018) 97:1701–6. doi: 10.1007/s00277-018-3367-9
68. Verschoor A, Langer HF. Crosstalk between platelets and the complement system in immune protection and disease. Thromb Haemost. (2013) 110:910–9. doi: 10.1160/th13-02-0102
69. Alexandru N, Popov D, Georgescu A. Platelet dysfunction in vascular pathologies and how can it be treated. Thromb Res. (2012) 129:116–26. doi: 10.1016/j.thromres.2011.09.026
70. Nording H, Langer HF. Complement links platelets to innate immunity. Semin Immunol. (2018) 37:43–52. doi: 10.1016/j.smim.2018.01.003
71. Waldner MJ, Neurath MF. Targeting the VEGF signaling pathway in cancer therapy. Expert Opin Ther Targets. (2012) 16:5–13. doi: 10.1517/14728222.2011.641951
72. Sung HK, Michael IP, Nagy A. Multifaceted role of vascular endothelial growth factor signaling in adult tissue physiology: an emerging concept with clinical implications. Curr Opin Hematol. (2010) 17:206–12. doi: 10.1097/MOH.0b013e32833865e6
73. Nieves BJ, D’Amore PA, Bryan BA. The function of vascular endothelial growth factor. Biofactors. (2009) 35:332–7. doi: 10.1002/biof.46
74. Takahashi H, Shibuya M. The vascular endothelial growth factor (VEGF)/VEGF receptor system and its role under physiological and pathological conditions. Clin Sci (Lond). (2005) 109:227–41. doi: 10.1042/cs20040370
Keywords: Ejiao Siwu decoction, primary immune thrombocytopenia, HPLC, network pharmacology, therapeutic mechanism, molecular docking
Citation: Wang MJ, Sun Y, Song Y, Ma JN, Wang ZQ, Ding XQ, Chen HY, Zhang XB, Song MM and Hu XM (2022) Mechanism and Molecular Targets of Ejiao Siwu Decoction for Treating Primary Immune Thrombocytopenia Based on High-Performance Liquid Chromatograph, Network Pharmacology, Molecular Docking and Cytokines Validation. Front. Med. 9:891230. doi: 10.3389/fmed.2022.891230
Received: 07 March 2022; Accepted: 15 June 2022;
Published: 13 July 2022.
Edited by:
Tomás José Gonzalez López, Burgos University Hospital, SpainReviewed by:
Paola Giordano, University of Bari Aldo Moro, ItalyMohamed A. Yassin, Hamad Medical Corporation, Qatar
William Mitchell, Children’s Hospital at Montefiore, United States
Yasuyuki Arai, Kyoto University, Japan
Copyright © 2022 Wang, Sun, Song, Ma, Wang, Ding, Chen, Zhang, Song and Hu. This is an open-access article distributed under the terms of the Creative Commons Attribution License (CC BY). The use, distribution or reproduction in other forums is permitted, provided the original author(s) and the copyright owner(s) are credited and that the original publication in this journal is cited, in accordance with accepted academic practice. No use, distribution or reproduction is permitted which does not comply with these terms.
*Correspondence: Min Min Song, minmin_song0824@163.com; Xiao Mei Hu, huxiaomei_2@163.com
†These authors have contributed equally to this work and share first authorship