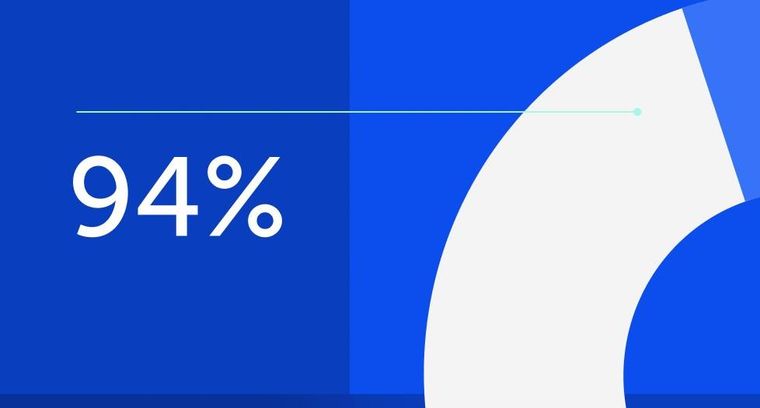
94% of researchers rate our articles as excellent or good
Learn more about the work of our research integrity team to safeguard the quality of each article we publish.
Find out more
REVIEW article
Front. Med., 28 April 2022
Sec. Gastroenterology
Volume 9 - 2022 | https://doi.org/10.3389/fmed.2022.886996
This article is part of the Research TopicClinicopathological Factors and Staging in Gastrointestinal CancersView all 74 articles
Colorectal cancer (CRC) is one of the most commonly diagnosed and deadly malignancies worldwide. The incidence of CRC has been increasing, especially in young people. Although great advances have been made in managing CRC, the prognosis is unfavorable. Numerous studies have shown that berberine (BBR) is a safe and effective agent presenting significant antitumor effects. Nevertheless, the detailed underlying mechanism in treating CRC remains indistinct. In this review, we herein offer beneficial evidence for the utilization of BBR in the management and treatment of CRC, and describe the underlying mechanism(s). The review emphasizes several therapeutic effects of BBR and confirms that BBR could suppress CRC by modulating gene expression, the cell cycle, the inflammatory response, oxidative stress, and several signaling pathways. In addition, BBR also displays antitumor effects in CRC by regulating the gut microbiota and mucosal barrier function. This review emphasizes BBR as a potentially effective and safe drug for CRC therapy.
Colorectal cancer (CRC), one of the most common cancers worldwide, has been reported as the third most common cancer in men (10%) and second most common in women (9.2%) (1), accounting for the fourth most common cause of cancer-related death (2). It is a heterogeneous disease with multifarious, hereditary, and biological characteristics. Subtypes of CRC have been demonstrated to present different prognoses and therapeutic responses (3). The incidence rate of CRC has been decreasing in many developed countries; nevertheless, the prevalence has increased in adults younger than 50 years of age in numerous economically underdeveloped countries (4, 5). Although tremendous improvements have been made in the diagnosis and management of CRC, the prognosis remains poor (6). Currently, treatment for CRC mainly includes surgery, radiotherapy, and chemotherapy (7). For unresectable tumors, chemotherapy is a fundamental and indispensable therapeutic regimen. The common cytotoxic chemotherapy drugs include 5-fluorouracil (5-FU), irinotecan, oxaliplatin, raltitrexed, and lonsurf, as well as molecular-targeted agents, such as anti-vascular endothelial growth factor (VEGF) therapies (e.g., bevacizumab, Ziv-aflibercept, ramucirumab, and regorafenib) and anti-epidermal growth factor receptor (EGFR) drugs (e.g., cetuximab and panitumumab) (8). However, these cytotoxic chemotherapy drugs kill tumor cells by direct cytotoxicity and have poor selectivity for tumors; therefore, these drugs not only kill tumor cells but also kill normal cells (9). Moreover, the mucosal barrier tends to be disrupted by common chemotherapeutic drugs, initiating intestinal mucositis (IM). This disruption is unfavorable for the later treatment of CRC (10). In addition, chemotherapy usually causes gastrointestinal (GI) epithelium injury, which is mediated at least in part by the activation of an inflammatory cascade (10). Furthermore, several limitations and adverse effects have also been reported during molecular-targeted therapy. Molecular-targeted therapy is relatively expensive, and patients might require multiple targeted agents. Level 3 or level 4 adverse events can be infrequently detected, and their incidence might increase when two or more targeted agents are combined (11). Furthermore, CRC involves multiple pathways, whereas conventional drugs only target one aspect. In addition, there is a substantially increased economic burden due to management. Therefore, more effective treatment approaches and medical interferences with safety and high efficacy are urgently needed. Traditional Chinese medicine (TCM) has been extensively acknowledged as a typical complementary and alternative treatment in China for patients with tumors (12). As a typical Chinese herbal medicine-derived phytochemical, berberine (BBR) and its derivatives have attracted increasing attention in cancer treatment. The current review highlights several beneficial properties of BBR and could provide novel insights into CRC treatment (13, 14).
According to a report from 40 European countries, an estimated 3.91 million new cases of cancer occurred in Europe in 2018, including 500,000 CRC cases. There were ~272,000 (13.2%) cases in men and 228,000 (12.3%) cases in women (15). In addition, 1.9 million incident cases and 0.9 million deaths worldwide were estimated to occur in 2020 (3). The overall incidence of CRC has been declining in many economically advanced countries; however, reports from the United States and other economically advanced countries, such as Australia, Canada, and Norway, have demonstrated that the prevalence is increasing in adults younger than 50 years of age (4, 5, 16–20). For example, the incidence of colon cancer increased by 1.8, 2.9, 2.9, and 3.1% per year in the United Kingdom (UK), New Zealand, Australia, and Denmark, respectively, in people younger than 50 years old, and the incidence of rectal cancer in the same age group also increased by 1.4, 2.6, and 3.4% per year in the UK, Australia, and Canada, respectively (20). Moreover, it has been reported that the incidence of colon cancer in individuals aged 20–29 years old increased by 9.3% every year from 2004 to 2014 in Australia, while the incidence of rectal cancer increased by 7.1% each year between 1993 and 2014 (17). The mortality of CRC has been reported to be decreased in many longstanding and economically advanced countries, such as the United States, Australia, New Zealand, most Western European countries (Austria and the UK.), some Asian countries (Japan), and Eastern European countries (the Czech Republic and Slovakia.) (21, 22). Effective prevention, early diagnosis through screening, interventions to reduce risk factors, and/or improvement of treatment strategies could contribute to reduced mortality (23). Nevertheless, mortality is still on the rise in both men and women in many low-income countries and regions, such as Mexico, Chile, and elsewhere in Latin America (21, 24, 25). CRC represents a huge burden globally on family and society and has a positive relationship with socioeconomic status (26–28). This burden is estimated to continue to increase due to the growth and aging of the population, as well as due to the adoption of high-risk behaviors and lifestyles, particularly in less economically developed countries (29, 30).
Colorectal cancer is usually observed in the proximal colon (41%), distal colon (22%), and rectum (28%) (31). Nevertheless, there can be differences in the location depending on age and gender (32). It has been reported that the most frequent sites of CRC in young patients are the rectum and the sigmoid colon (33). Genetic and environmental risk factors have been demonstrated to play critical roles in the progression and development of CRC. A number of molecular genetic markers have been identified for the diagnosis, prognosis, and treatment of CRC, such as KRAS, ERBB2, MLH, and NAV2/TCF7L (34, 35). An increasing amount of evidence has supported associations between a positive family history of CRC and an increased risk for CRC (36–38). The reported heritability of CRC ranges from 12 to 35% according to twin and family studies (39, 40). Although tremendous advances have been made in the genome-wide association studies of CRC (41, 42), there remain many elusive factors affecting heritability (43). Hereditary colorectal cancer syndromes affect people with a high lifetime risk of developing CRC caused by an inherited or de novo germline mutation, accounting for 5–10% of all CRCs (44, 45). This subgroup can be divided into nonpolyposis and polyposis syndromes. The former includes Lynch syndrome, and the latter contains familial adenomatous polyposis (FAP), Peutz-Jeghers syndrome, and MUTYH-associated polyposis (MAP). Polyposis syndromes are more effortlessly diagnosed by doctors due to the number of polyps; however, nonpolyposis syndromes are commonly overlooked because these patients present few adenomas, and these adenomas are morphologically similar to sporadic lesions. Hence, systematic molecular investigation in subjects of any age or subgroup of subjects <70 years of age might improve the diagnosis of this genetic syndrome. Moreover, some basic diseases, such as inflammatory bowel disease (IBD) and type 2 diabetes mellitus (T2DM), are also well known to be associated with CRC (46, 47). In addition, several acquired environmental lifestyles have been recognized as important risk factors for CRC, such as obesity and overweight (48), lack of physical exercise (49), excessive drinking (50), smoking (51, 52), high Diet Inflammatory Index (DII) scores (53, 54), and sedentary lifestyles (55). Additionally, some factors, such as healthy diet structure (e.g., fiber-containing foods, calcium supplements, milk intake, vitamins, fish intake, and phenol intake) and physical activity, have been described to reduce the risk factors of CRC (56–59). However, some of the data have been inconsistent, and the effect on rectal cancer vs. colorectal cancer may also be different. Furthermore, healthy lifestyle habits have been shown to improve the prognosis and mortality of CRC survivors (60, 61). In addition, male sex, increasing age, race, and medical intake have also been demonstrated to play critical roles in the progression and development of CRC (62–64) (Figure 1).
There are three distinctive oncogenic pathways involved in CRC, including the adenoma-carcinoma sequence, serrated pathway, and inflammatory pathway (Figure 2). The adenoma-carcinoma sequence is a typical pathway that can explain ~60–90% of sporadic CRC. In this classic pathway, normal cells are driven to transfer to small adenomas, then to large adenomas, and finally to malignancies by the gradual accumulation of genetic and epigenetic changes (65). Several factors have been confirmed to play imperative roles in this pathway, including male sex, increasing age, positioning in the distal colon, tobacco and alcohol use, and a high-fat diet (66, 67). This pathway is primarily characterized by chromosomal instability (CIN) and is related to the development of the CIN-positive subtype. Inactivating mutations in adenomatous polyposis coli (APC), a well-known tumor suppressor gene, have been demonstrated in more than 70% of adenomas and CRCs (68, 69). In addition, some mutations in other genes, such as KRAS, TP53, SMAD4, and PIK3CA, also contribute to this model (70, 71). Recently, the serrated pathway was described as one of the CRC subsets, accounting for ~10–15% of sporadic CRCs (30). In this model, serrated polyps are considered precursor lesions to CRC, and they are characterized by the transformation of normal cells to hyperplastic polyps (HPs), then to sessile serrated adenoma (SSA), and ultimately to CRC (72, 73). The BRAF mutation and CpG island methylator phenotype (CIMP) have significant involvement in this pathway (72, 74). The inflammatory pathway is another distinctive oncogenic pathway involved in CRC. It is highlighted by the progression of normal cells to indefinite dysplasia, then to low-grade dysplasia, next to high-grade dysplasia, and finally to CRC, stimulated by chronic inflammation (75). However, this pathway only accounts for <2% of all CRCs (30, 76). IBD, especially ulcerative colitis (UC), is representative of this pathway. Unlike the above two precursor lesions, chronic inflammation-induced dysplasia usually appears as a flat mucosa with multifocality. Different from the adenoma-carcinoma sequence, mutations of TP53 are an early event in this model (75).
Endoscopic resection can be performed as a minimally invasive method for early-stage colon cancer. It includes endoscopic mucosal resection (EMR), endoscopic submucosal dissection (ESD), and endoscopic full-thickness resection. The choice of endoscopic resection method depends on the size of T1 cancers. However, some individuals with T1 cancers who experience endoscopic resection require additional colectomy with lymph node dissection due to lymph node metastases (77). Surgery is the cornerstone and one of the most effective management options for CRC. However, the surgical approach for colon cancer is dependent on the tumor location and anatomic relationship with blood vessels. Currently, laparoscopy has become a standard method for CRC in many countries. Compared to conventional open surgery, laparoscopic resection is a minimally invasive technique that can expedite gastrointestinal function recovery and reduce the length of hospital stay, particularly for elderly patients (78). In addition, it has been shown that laparoscopic resection is associated with reduced postoperative mortality, and there are no adverse effects on long-term survival (79, 80). More recently, robotic colectomy has emerged as a popular method for the treatment of CRC (81). Although robotic colectomy can decrease the length of stay, it costs more and requires a longer operation time than laparoscopic colectomy, and no significant differences have been observed in perioperative and short- or long-term outcomes (82, 83). In addition to surgery for CRC, several drugs have been permitted for CRC management in clinical trials. The Food and Drug Administration (FDA)-approved anticancer drugs include 5 cytotoxics (5-FU, capecitabine, TAS-102, irinotecan, and oxaliplatin) and eight biologics/targets (cetuximab, panitumumab, bevacizumab, Ziv-aflibercept, regorafenib, ramucirumab, pembrolizumab, and nivolumab). As a standard first-line chemotherapy drug, 5-FU plays significant role in palliative and adjuvant systemic therapy for CRC. Nevertheless, some adverse effects of these drug treatments have been reported, such as diarrhea, gastrointestinal tract injury, stomachache, and fever (84–86). Compared to chemotherapy, immunotherapy could prompt the memory function of the adaptive immune system and trigger the immune system against tumors, achieving long-term durable responses. Furthermore, fewer adverse effects have been observed in immunotherapy because of immune tolerance.
Berberine, also known as Coptis rhizome, is a pentacyclic isoquinoline alkaloid. It can be found in the Chinese herb Coptis chinensis and numerous Berberis plants, such as Berberis aristata (81), Berberis vulgaris (87), and Berberis darwinii (88). The molecular formula of BBR is C20H18NO4, and the molecular weight is 336.337 g/mol. The isoquinoline alkaloids in Huanglian extracts include BBR, xanthophylline, epiberberine, and pharmacophorine. BBR is mainly metabolized in the intestine and liver (89, 90). With the involvement of cytochrome and UDP glucuronosyltransferase (UGT), BBR could be metabolized in liver cells (91). There are four major types of BBR metabolites: berberrubine, thalifendine, demethyleneberberine, and jatrorrhizine (92). However, the number of metabolites varies by species. For example, 16 and 11 metabolites were identified in rats (93) and mice (91), respectively.
Berberine has been used as an important TCM for a long period of time owing to its extraordinary efficiency, such as in alleviating fever, dispelling fire, drying dampness, cooling blood, and detoxifying toxins. Recently, an increasing number of studies have reported regarding the novel pharmacological effects of BBR. For instance, BBR exerts remarkable anti-inflammatory (94–96), antiviral (97), antioxidant (98), antidiabetic (99), immunosuppressive (100), cardiovascular (101, 102), and neuroprotective (103) activities. Therefore, BBR could be utilized to treat various disorders (104–107), including metabolic, cardiovascular, digestive, and neurological diseases. It has been demonstrated that BBR displays protective effects against digestive diseases by inhibiting toxins and bacteria and fortifying the intestinal mucosa (108). Moreover, BBR has been confirmed to regulate glucolipid metabolism, ameliorate energy consumption, and decrease body weight (106). Furthermore, BBR also presents strong cardiovascular protection and neuroprotective effects by improving cardiovascular hemodynamics, reducing hypertension, and attenuating atherosclerosis progression (101, 109).
Emerging evidence has shown that BBR exerts anticancer effects in several malignancies (110–112). BBR has been reported to inhibit cancer cell proliferation by affecting the cell cycle and autophagy and stimulating cell apoptosis. For example, BBR could induce G1 cycle arrest in A549 lung cancer cells by decreasing the levels of cyclin D1 and cyclin E1 (113). BBR could inhibit the expression of cyclin D1 in HepG2 liver cancer cells (114). BBR also induced G1 cycle arrest by inhibiting cyclin B1 expression and CDC2 kinase in some cancer cells (115). Moreover, BBR has been suggested to induce autophagy in glioblastoma by targeting the AMP-activated protein kinase (AMPK)/mechanistic target of rapamycin (mTOR)/ULK1 pathway (116) and in liver cancer cells by stimulating the release of beclin-1 from the Bcl-2/beclin-1 complex (117). In addition, BBR has been revealed to stimulate apoptosis in leukemia by upregulation of caspase-8 and caspase-9 (118) and in skin squamous cell carcinoma A431 cells by increasing cytochrome C levels (119). In addition, BBR has been confirmed to inhibit cell migration and invasion by inhibiting the expression of epithelial–mesenchymal transition (EMT) and metastasis-related proteins, such as matrix metalloproteinases (MMPs) and E-cadherin, the tumor microenvironment, and/or the caspase-1/interleukin (IL)-1β and nuclear factor kappa B (NF-κB) signaling pathways (120–123). Interestingly, canadine, a derivative of flavopiridol, has been shown to improve cancer-induced muscle wasting (124). Furthermore, BBR has shown antitumor effects by interacting with microRNAs (125) and inhibiting telomerase activity (126).
Numerous studies have revealed that BBR is a safe and effective treatment for CRC (127–129). The reported mechanisms include regulation of gene expression (microRNAs, long noncoding RNAs (lncRNAs), and mRNAs) (130–133), growth factors (EGFR) (134), cell cycles (135), signaling pathways (AMPK, JAK2/signal transducer and activator of transcription 3 (STAT3), Wnt/β-catenin, IL-6/STAT3/NF-κB, and cyclooxygenase-2 (COX-2)/prostaglandin E2 (PGE2) pathways) (13, 129, 136), inflammation, and oxidative stress (Figure 3). The effects and molecular mechanism of BBR in the treatment of CRC are summarized in Table 1.
Figure 3. Molecular mechanism of BBR in the treatment of CRC. CRC, colorectal cancer; BBR, berberine; ROS, reactive oxygen species; AMPK, AMP-activated protein kinase; STAT3, signal transducer and activator of transcription 3; COX-2, cyclooxygenase-2; PGE2, prostaglandin E2; IL-6, interleukin 6; NF-κB, nuclear factor kappa B.
Abnormal expression of miRNAs, lncRNAs, and mRNAs has been implicated in cancer development, including CRC. Based on the recently established evidence, BBR shows important regulatory effects on gene expression that are dysregulated in CRC, making it a potential agent for managing cancers. For example, BBR was reported to regulate the miR-21/integrin β4 (ITGβ4)/programmed cell death 4 (PDCD4) axis to exert anticancer effects on the CRC cell line HCT116 (137). In addition, BBR could decrease the expression levels of miR-429 while upregulating the expression of E-cadherin and partitioning defective 3 (Par-3) in CRC (132). Moreover, the combination of NVP-AUY922 and BBR induced cell growth arrest by suppressing cyclin-dependent kinase 4 (CDK4) expression and inducing miR-296-5p-mediated inhibition of the peptidylprolyl cis/trans isomerase NIMA-interacting 1 (Pin1)-β-catenin-cyclin D1 signaling pathway in CRC (129). In addition, BBR has been confirmed to prevent the proliferation and migration of CRC cells by decreasing glucose-regulated protein 78 (GRP78) (131). Furthermore, the lncRNA cancer susceptibility 2 (CASC2)/zeste 2 polycomb repressive complex 2 subunit (EZH2) /Bcl-2 axis was identified in BBR-induced CRC cell apoptosis (138). Huang et al. also suggested that DNA (cytosine-5)-methyltransferase PliMCI (DNMTs) and its targeted miRNAs were involved in the therapeutic effects of BBR on CRC (139). The cell cycle is an extremely conserved, ordered, and complex process that controls DNA replication and mitosis. It is regulated by many mechanisms to ensure that correct cell division occurs (146). Several reports have suggested that BBR can induce cell cycle arrest to inhibit cancer development. For instance, Huang et al. demonstrated that BBR induced G0/G1 cell cycle arrest in CRC and then further inhibited cell proliferation (130). Samad et al. found that BBR could also cause G0/G1 cell cycle arrest by regulating CCDN1 and CDK4 in the CRC cell line HCT116 and inhibiting telomerase activity (135). Soffar suggested that BBR diminished CRC cancer cell growth by modulating G1-phase cell cycle delay (140). In addition, Liu and coauthors observed that BBR suppressed cell proliferation by inducing G0/G1 phase cell cycle arrest in CRC cells (141). Therefore, the anti-proliferation effect of BBR might largely contribute to G0/G1 cell cycle arrest in CRC.
An important relationship has been confirmed between chronic inflammation and CRC development. It has been estimated that ~15–20% of cancer-related deaths involve an inflammatory response (147). CRC is colitis-associated cancer and patients with Crohn's disease (CD) or UC who develop CRC present an unfavorable prognosis (148, 149). This phenomenon might be explained by the chronic inflammatory response in the intestinal tract being able to trigger tumorigenesis and stimulate malignancy development (13). In addition, oxidative stress also plays a very important role in IBD carcinogenesis (66). During inflammation, activated inflammatory cells (neutrophils, macrophages, etc.) produce high levels of reactive oxygen species (ROS), including superoxide radicals, hydroxyl radicals, and hydrogen peroxide, which are important substances that contribute to tumorigenesis. Oxygen radicals can lead to abnormal DNA and RNA synthesis, and abnormal protein assembly and DNA repair (150). Moreover, oxygen radicals can also cause microsatellite instability (MSI) (151) and hypermethylation (152). In addition, oxygen radicals can activate genes that promote the production of free radicals, such as nitric oxide (NO) synthase and COX-2, allowing for a progressive inflammatory response and carcinogenesis (153). Therefore, inhibition of the inflammatory response and oxidative stress are beneficial for cancer treatment. BBR was reported to inhibit azoxymethane and dextran sulfate sodium (AOM/DSS)-induced CRC in a mouse model by suppressing COX-2 expression and regulating the AMPK pathway (142). Another discovery indicated that BBR inhibited the migration and invasion of CRC cells via the COX-2/PGE2-mediated JAK2/STAT3 signaling pathway (136). Additionally, Li and coauthors confirmed that BBR inhibited colitis-associated CRC by inhibiting inflammatory responses and subsequently suppressed EGFR signaling-involved tumor cell growth (143). Furthermore, BBR exerts its anti-inflammatory role in UC by modulating the IL-6/STAT3/NF-κB signaling pathway (154). BBR was described to inhibit the proliferation of CRC cells by inactivating the Wnt/β-catenin signaling pathway (144). Thus, BBR could be used as a critical anti-inflammatory and antioxidant agent in CRC.
The colon is covered with a mass of microorganisms. It has been reported that more than 500–1,000 species and a total of 1013 bacteria settle in the colon and other parts of the large intestine of adult humans. The diversity and composition of the microbiota could be changed by a variety of factors, such as age, dietary habits, pharmacotherapy, and psychological stress. (155, 156). The intestinal microflora is often considered an important organ acquired by the human body, which can protect the host from pathogenic bacteria, promote the host's digestion and absorption, affect drug metabolism and carcinogenesis, influence the absorption and distribution of fat, and regulate energy metabolism and the innate and acquired immune systems (157). Dysbiosis is an imbalance in the function or structure of gut microbiota and has been responsible for many disorders, including CRC. Noteworthy changes have been reported in specific bacterial clusters in CRC subjects (158). For instance, commensal bacterial species (such as Faecalibacterium, Blautia, and Roseburia) are found to be decreased, while harmful bacterial inhabitants (such as Akkermansia, Fusobacterium nucleatum, and Clostridium difficile) are extensively enriched in CRC (159). Dysbiosis in the gut microbiota could contribute to the development of CRC by modulating several different mechanisms, such as the inflammatory response, immune regulation, DNA damage, and the production of metabolites responsible for cancer development or suppression (160–164). Beneficial bacteria might compete for attachment sites to reduce the abundance of pathogenic bacteria and avoid infection. However, the pathogenic bacteria could increase intestinal permeation and are very closely related to the colon inflammatory response, which might be an important issue for the promotion of CRC (165). Furthermore, microbial metabolites, such as short-chain fatty acids (SCFAs), might also participate in the development of CRC. SCFAs have been reported to regulate the differentiation of Th1/Th17 cells and production of IL-10 by increasing the expression of transcription factor B lymphocyte-induced maturation protein 1 (Blimp-1) to maintain intestinal homeostasis (166). In addition, Fusobacterium nucleatum changed microbial structures and activated JAK-STAT and mitogen-activated protein kinase (MAPK) pathways, promoting the release of inflammatory factors (128). Moreover, the gut microbiota causes immune repair by modulating the intestinal barrier.
Common chemotherapeutic drugs tend to disrupt the mucosal barrier, which is detrimental to the late treatment of CRC (10). In addition, chemotherapy generally induces gastrointestinal (GI) epithelium damage that is at least partially intermediated by the activation of the inflammatory cascade (10). For example, 5-FU, a standard first-line treatment for CRC individuals, promotes apoptosis of epithelial cells and inhibits mucosal proliferation, while apoptotic cells can further promote inflammation. In contrast, BBR can inhibit tumor growth through meditation of the intestinal flora and mucosal barrier, and generally and ultimately improve weight loss (167). Moreover, BBR has been reported to modulate the composition of intestinal flora and significantly reduce flora diversity. For instance, BBR was demonstrated to inhibit the relative abundance of some of the intestinal flora, such as Desulfovibrio, Lactobacillus acidophilus, Eubacterium, Lactococcus lactis, and Bacteroides (168, 169), while it enriched Bacteroides in the colon and terminal ileum of C57BL/6 mice (169). In addition, it has been also demonstrated that BBR significantly reduced the relative abundances of both Firmicutes (such as Lactobacillus sp.) and Bacteroidetes in the gut of high-fat diet (HFD)-fed mice, indicating that the antimicrobial activity of BBR may be responsible for its anti-obesity effects (170). Akkermansia muciniphila (A. muciniphila) is a member of beneficial microbiota and a dedicated intestinal mucin degrader (171). Abnormal production and expression of mucin damage the mucinous layer, bringing bacteria into close contact with the intestinal epithelial cells and possibly triggering adverse host response and subsequent CRC development (172). BBR has been revealed to increase the growth of the populations of the symbiotic genus Akkermansia (173) and may further have effects on mucin expression. Moreover, BBR could modulate the gut microbiota in the colon by impacting the Treg/Th17 balance, reducing the levels of proinflammatory factors, including IL-17, IL-21, IL-22, IL-23, and IL-25, and increasing the levels of anti-inflammatory factors, such as IL-10 (174). In addition, BBR reduced bacterial endotoxins in the blood and alleviated the inflammatory response (175). Furthermore, it has been demonstrated that BBR decreases mucosal damage by modulating the levels of polyamines. Wu et al. (176) found that BBR could downregulate the levels of polyamine metabolism-associated proteins, including ornithine decarboxylase (ODC), c-myc, and hypoxia-inducible factor 1 subunit alpha (HIF-1α), and upregulate the levels of polyamine metabolic enzymes, including ornithine decarboxylase antizyme 1 (OAZ1) and spermidine/spermine N1-acetyltransferase (SSAT). At the same time, BBR could decrease the permeability of intestinal mucosa by modulating zonula occludens-1 (ZO1) and occludin (OCLN) (176). More recently, Deng and coauthors found that preadministration of BBR suppressed CRC development by inhibiting inflammation and proliferation and maintaining intestinal homeostasis (145).
Indoleamine 2,3-dioxygenase (IDO) is a key inflammatory cytokine-inducible rate-limiting enzyme of tryptophan catabolism, which includes three types, namely, IDO1, IDO2, and tryptophan 2,3-dioxygenase (TDO2). IDO has been demonstrated to play significant roles in the inhibition of intracellular pathogen replication and immunomodulation (177, 178). IDO is constitutively expressed in several human and mouse cells. It can catalyze the oxidative catabolism of tryptophan to kynurenine (179). In addition, IDO1 exerts its immunosuppressive effect by inhibiting the macrophage response and effector T cells by tryptophan starvation of sensitive T cells or accumulation of toxic metabolites (kynurenine) produced by tryptophan metabolism, subsequently inducing cell cycle arrest and effector T-cell death in the tumor microenvironment (180). In addition, the activity of IDO1 has been reported to directly stimulate cancer growth and proliferation by the production of kynurenine and the activation of β-catenin signaling (181). Previous studies discovered that IDO1 was highly expressed in CRC and was also correlated with impaired clinical outcomes (182–185). Therefore, targeting IDO1 for the treatment of cancer could be considered as an immunosuppressive-targeted strategy (177). Interestingly, BBR revealed uncompetitive and reversible inhibitory activity on IDO1, which might be due to direct binding to heme iron or occupation of the presumed tryptophan-binding site (186). Recently, a series of novel BBR derivatives targeting IDO1 has been designed to reduce the activity of IDO1. Wang et al. observed that compounds 2i and 2n of BBR displayed anticancer activity by increasing the specific lysis of natural killer (NK) cells to A549 cells through IDO1 (187). Both compounds repressed interferon (IFN)-γ-induced IDO1 expression via activation of AMPK and inhibition of STAT1 phosphorylation. Nevertheless, there have been no related studies about the effects of BBR on CRC via inhibition of IDO1 activity. Hence, compounds 2i and 2n could be selected as IDO1 modulators for small-molecule CRC immunotherapy for further investigation.
Although BBR presents valuable and promising biological effects in the management of cancers, the side effects of BBR cannot be overlooked (188). BBR should not be administered to infants with jaundice, pregnant women, or nursing women because of the potential risk of bilirubin-induced brain injury (189). In addition, intravenous administration of BBR can cause allergic reactions (190). Additionally, arrhythmia has been reported in hypervagotonic individuals after the administration of BBR (191). Other side effects, including nausea, cramping, diarrhea, flatulence, vomiting, rash, fever, constipation, and stomachache, have also been reported (192–194). Furthermore, a high dosage of BBR could cause low blood pressure, dyspnea, flu-like symptoms, and cardiac injury (190). Moreover, BBR can regulate the activity of P-glycoprotein (P-gp), and potential drug-drug interactions (DDIs) are observed when BBR is coadministered with P-gp substrates (195–197). For example, BBR in combination with P-gp inhibitor tetrandrine (Tet) can significantly improve the pharmacokinetics and hypoglycemic efficacy of BBR (196). Codelivery of the P-gp inhibitor tariquidar and BBR reversed the multiple drug resistance (MDR) in the K562/DOXO cell line (198). In addition, the efficiency of BBR is limited by its low bioavailability due to its poor absorption rate in the gut, low solubility in water, and fast metabolism. Studies have shown that the oral bioavailability of BBR is 0.68% in rats (199). Therefore, searching for novel methods to improve the absorption of BBR in the gut might be beneficial for the treatment of cancer. Previous studies have suggested complexation with C60 fullerene (200), solid lipid nanoparticles (SLNs) encompassing BBR by the spray-drying method (201), combining it with p-gp inhibitors (such as tariquidar and tetrandrine) (196, 198), and modification to berberine organic acid salts (BOAs) (168). However, the above-mentioned strategies were mostly tested in animals and must be demonstrated in human clinical trials.
In recent years, increasing evidence has suggested that nanoparticle (NP)-based delivery systems could be a great potential strategy to improve the therapeutic effects of BBR as an anticancer drug (202, 203). NPs, with a small diameter of 5–200 nm, are important artificial components of nanomedicine and play a key role as a therapeutic drug delivery system. NPs can effectively detect cancer and elucidate cancer-related mechanisms, especially as therapeutic delivery vehicles (204). NPs have unique physical and chemical properties, as well as powerful biological functions. They can effectively protect cargo from the degradation of enzymes and mechanisms (205). Several categories of nanoscale drug delivery vehicles have been demonstrated, and some of them have been applied in nanomedicine for tumor management, such as liposomes, carbon nanotubes, hydrogels, polymeric nanoparticles, and magnetic nanoparticles (206). BBR-loaded polymeric NPs (polyamidoamine dendrimers, chitosan NPs, and dextran NPs), metal NPs (iron-oxide NPs and mesoporous silica NPs), lipid NPs (liposomes), and carbon NPs (carbon dots and graphene NPs) have been revealed to have potential antitumor effects in different trials and experiments (203). Nonetheless, further studies regarding the efficacy of BBR in combination with different conventional anticancer drugs will pave the way for the use of BBR as a component of cancer chemotherapy.
Berberine exerts widespread pharmacological activities in different disorders. In this review, the functions of BBR are systematically explored and the antitumor effects on CRC, as well as the underlying mechanisms, are delineated. This review confirms that BBR has antibacterial, anti-inflammatory, antioxidant, antitumor, and hypoglycemic effects and cardiovascular and cerebrovascular protective functions. Furthermore, BBR exerts antitumor effects in CRC by modulating miRNA, lncRNA, and mRNA expression, inducing cell cycle arrest, inhibiting cell proliferation, stimulating cell apoptosis, and suppressing inflammation and oxidative stress via several signaling pathways. In addition to the above-mentioned functions, BBR has also revealed powerful antitumor activity in CRC by modulating the gut microbiota and mucosal barrier, which is good news for the search for natural antitumor drugs; however, to date, studies of the antitumor effects of BBR have been mainly performed in in vitro and a few in vivo models. Hence, in vivo studies, especially human studies, are warranted to further elucidate and confirm the therapeutic effects of BBR. Moreover, innovative approaches to adjust the BBR structure into more promising derivatives with stronger antitumor effects or to synergize with other chemotherapeutic drugs to improve the anticancer effects of BBR are needed. In summary, BBR is a safe, inexpensive, and effective long-term application for the treatment of cancers.
YS conceived the project and drafted the manuscript. XJ and ZJ performed research studies. MJ collected background information. All authors reviewed and approved the manuscript.
The authors declare that the research was conducted in the absence of any commercial or financial relationships that could be construed as a potential conflict of interest.
All claims expressed in this article are solely those of the authors and do not necessarily represent those of their affiliated organizations, or those of the publisher, the editors and the reviewers. Any product that may be evaluated in this article, or claim that may be made by its manufacturer, is not guaranteed or endorsed by the publisher.
1. Wild CP, Stewart BW, Wild C. World Cancer Report 2014: World Health Organization Geneva, Switzerland (2014).
2. Maida M, Macaluso FS, Ianiro G, Mangiola F, Sinagra E, Hold G, et al. Screening of colorectal cancer: present and future. Expert Rev Anticancer Ther. (2017) 17:1131–46. doi: 10.1080/14737140.2017.1392243
3. Xi Y, Xu P. Global colorectal cancer burden in 2020 and projections to 2040. Transl Oncol. (2021) 14:101174. doi: 10.1016/j.tranon.2021.101174
4. Siegel RL, Fedewa SA, Anderson WF, Miller KD, Ma J, Rosenberg PS, et al. Colorectal cancer incidence patterns in the United States, 1974-2013. J Ntl Cancer Inst. (2017) 109:djw322. doi: 10.1093/jnci/djw322
5. Larsen IK, Bray F. Trends in colorectal cancer incidence in Norway 1962-2006: an interpretation of the temporal patterns by anatomic subsite. Int J Cancer. (2010) 126:721–32. doi: 10.1002/ijc.24839
6. Sałaga M, Zatorski H, Sobczak M, Chen C, Fichna J. Chinese herbal medicines in the treatment of IBD and colorectal cancer: a review. Curr Treat Options Oncol. (2014) 15:405–20. doi: 10.1007/s11864-014-0288-2
7. Zhang Y, Chen Z, Li J. The current status of treatment for colorectal cancer in China: a systematic review. Medicine. (2017) 96:e8242. doi: 10.1097/MD.0000000000008242
8. Yau TO. Precision treatment in colorectal cancer: Now and the future. JGH Open. (2019) 3:361–9. doi: 10.1002/jgh3.12153
9. Chari RV. Targeted cancer therapy: conferring specificity to cytotoxic drugs. Acc Chem Res. (2008) 41:98–107. doi: 10.1021/ar700108g
10. Lee CS, Ryan EJ, Doherty GA. Gastro-intestinal toxicity of chemotherapeutics in colorectal cancer: the role of inflammation. World J Gastroenterol. (2014) 20:3751–61. doi: 10.3748/wjg.v20.i14.3751
11. Xie YH, Chen YX, Fang JY. Comprehensive review of targeted therapy for colorectal cancer. Signal Transduct Targeted Ther. (2020) 5:22. doi: 10.1038/s41392-020-0116-z
12. Kong MY Li LY, Lou YM, Chi HY, Wu JJ. Chinese herbal medicines for prevention and treatment of colorectal cancer: From molecular mechanisms to potential clinical applications. J Integr Med. (2020) 18:369–84. doi: 10.1016/j.joim.2020.07.005
13. Hallajzadeh J, Maleki Dana P, Mobini M, Asemi Z, Mansournia MA, Sharifi M, et al. Targeting of oncogenic signaling pathways by berberine for treatment of colorectal cancer. Med Oncol (Northwood, London, England). (2020) 37:49. doi: 10.1007/s12032-020-01367-9
14. Chen YX, Gao QY, Zou TH, Wang BM, Liu SD, Sheng JQ, et al. Berberine versus placebo for the prevention of recurrence of colorectal adenoma: a multicentre, double-blinded, randomised controlled study. Lancet Gastroenterol Hepatol. (2020) 5:267–75. doi: 10.1016/S2468-1253(19)30409-1
15. Ferlay J, Colombet M, Soerjomataram I, Dyba T, Randi G, Bettio M, et al. Cancer incidence and mortality patterns in Europe: Estimates for 40 countries and 25 major cancers in 2018. Eur J Cancer (Oxford, England: 1990). (2018) 103:356–87. doi: 10.1016/j.ejca.2018.07.005
16. Bailey CE, Hu CY, You YN, Bednarski BK, Rodriguez-Bigas MA, Skibber JM, et al. Increasing disparities in the age-related incidences of colon and rectal cancers in the United States, 1975-2010. JAMA Surg. (2015) 150:17–22. doi: 10.1001/jamasurg.2014.1756
17. Feletto E, Yu XQ. Trends in Colon and Rectal Cancer Incidence in Australia from 1982 to 2014: Analysis of Data on Over 375,000 Cases. Cancer Epidemiol Biomarkers Prev. (2019) 28:83–90. doi: 10.1158/1055-9965.EPI-18-0523
18. Brenner DR, Ruan Y, Shaw E, De P, Heitman SJ, Hilsden RJ. Increasing colorectal cancer incidence trends among younger adults in Canada. Prevent Med. (2017) 105:345–9. doi: 10.1016/j.ypmed.2017.10.007
19. Dairi O, Anderson JC, Butterly LF. Why is colorectal cancer increasing in younger age groups in the United States? Expert Rev Gastroenterol Hepatol. (2021) 15:623–32. doi: 10.1080/17474124.2021.1876561
20. Araghi M, Soerjomataram I, Bardot A, Ferlay J, Cabasag CJ, Morrison DS, et al. Changes in colorectal cancer incidence in seven high-income countries: a population-based study. Lancet Gastroenterol Hepatol. (2019) 4:511–8. doi: 10.1016/S2468-1253(19)30147-5
21. Center MM, Jemal A, Smith RA, Ward E. Worldwide variations in colorectal cancer. CA Cancer J Clin. (2009) 59:366–78. doi: 10.3322/caac.20038
22. Bosetti C, Levi F, Rosato V, Bertuccio P, Lucchini F, Negri E, et al. Recent trends in colorectal cancer mortality in Europe. Int J Cancer. (2011) 129:180–91. doi: 10.1002/ijc.25653
23. Edwards BK, Ward E, Kohler BA, Eheman C, Zauber AG, Anderson RN, et al. Annual report to the nation on the status of cancer, 1975-2006, featuring colorectal cancer trends and impact of interventions (risk factors, screening, and treatment) to reduce future rates. Cancer. (2010) 116:544–73. doi: 10.1002/cncr.24760
24. Bosetti C, Bertuccio P, Malvezzi M, Levi F, Chatenoud L, Negri E, et al. Cancer mortality in Europe, 2005-2009, and an overview of trends since 1980. Ann Oncol. (2013) 24:2657–71. doi: 10.1093/annonc/mdt301
25. Chatenoud L, Bertuccio P, Bosetti C, Malvezzi M, Levi F, Negri E, et al. Trends in mortality from major cancers in the Americas: 1980–2010. Ann Oncol. (2014) 25:1843–53. doi: 10.1093/annonc/mdu206
26. Sharma R. An examination of colorectal cancer burden by socioeconomic status: evidence from GLOBOCAN 2018. EPMA J. (2020) 11:95–117. doi: 10.1007/s13167-019-00185-y
27. Douaiher J, Ravipati A, Grams B, Chowdhury S, Alatise O, Are C. Colorectal cancer-global burden, trends, and geographical variations. J Surg Oncol. (2017) 115:619–30. doi: 10.1002/jso.24578
28. Luengo-Fernandez R, Leal J, Gray A, Sullivan R. Economic burden of cancer across the European Union: a population-based cost analysis. Lancet Oncol. (2013) 14:1165–74. doi: 10.1016/S1470-2045(13)70442-X
29. Favoriti P, Carbone G, Greco M, Pirozzi F, Pirozzi RE, Corcione F. Worldwide burden of colorectal cancer: a review. Updates Surg. (2016) 68:7–11. doi: 10.1007/s13304-016-0359-y
30. Keum N, Giovannucci E. Global burden of colorectal cancer: emerging trends, risk factors and prevention strategies. Nature reviews Gastroenterology & hepatology. (2019) 16:713–32. doi: 10.1038/s41575-019-0189-8
31. Cheng L, Eng C, Nieman LZ, Kapadia AS, Du XL. Trends in colorectal cancer incidence by anatomic site and disease stage in the United States from 1976 to 2005. Am J Clin Oncol. (2011) 34:573–80. doi: 10.1097/COC.0b013e3181fe41ed
32. Thanikachalam K, Khan G. Colorectal Cancer and Nutrition. Nutrients. (2019) 11:164. doi: 10.3390/nu11010164
33. O'Connell JB, Maggard MA, Livingston EH, Yo CK. Colorectal cancer in the young. Am J Surg. (2004) 187:343–8. doi: 10.1016/j.amjsurg.2003.12.020
34. Comprehensive molecular characterization of human colon and rectal cancer. Nature. (2012) 487:330–7. doi: 10.1038/nature11252
35. Kudryavtseva AV, Lipatova AV, Zaretsky AR, Moskalev AA, Fedorova MS, Rasskazova AS, et al. Important molecular genetic markers of colorectal cancer. Oncotarget. (2016) 7:53959–83. doi: 10.18632/oncotarget.9796
36. Henrikson NB, Webber EM, Goddard KA, Scrol A. Piper M. Family history and the natural history of colorectal cancer: systematic review. Genet Med. (2015) 17:702–12. doi: 10.1038/gim.2014.188
37. Slattery ML, Levin TR, Ma K, Goldgar D, Holubkov R, Edwards S. Family history and colorectal cancer: predictors of risk. Cancer Causes Control. (2003) 14:879–87. doi: 10.1023/B:CACO.0000003840.94591.76
38. Pesola F, Eloranta S, Martling A, Saraste D, Smedby KE. Family history of colorectal cancer and survival: a Swedish population-based study. J Intern Med. (2020) 287:723–33. doi: 10.1111/joim.13036
39. Czene K, Lichtenstein P, Hemminki K. Environmental and heritable causes of cancer among 96 million individuals in the Swedish Family-Cancer Database. Int J Cancer. (2002) 99:260–6. doi: 10.1002/ijc.10332
40. Lichtenstein P, Holm NV, Verkasalo PK, Iliadou A, Kaprio J, Koskenvuo M, et al. Environmental and heritable factors in the causation of cancer—analyses of cohorts of twins from Sweden, Denmark, and Finland. N Engl J Med. (2000) 343:78–85. doi: 10.1056/NEJM200007133430201
41. Thomas M, Sakoda LC, Hoffmeister M, Rosenthal EA, Lee JK, van Duijnhoven FJB, et al. Genome-wide modeling of polygenic risk score in colorectal cancer risk. Am J Hum Genet. (2020) 107:432–44. doi: 10.1016/j.ajhg.2020.07.006
42. Simmer F, Brinkman AB, Assenov Y, Matarese F, Kaan A, Sabatino L, et al. Comparative genome-wide DNA methylation analysis of colorectal tumor and matched normal tissues. Epigenetics. (2012) 7:1355–67. doi: 10.4161/epi.22562
43. Jiao S, Peters U, Berndt S, Brenner H, Butterbach K, Caan BJ, et al. Estimating the heritability of colorectal cancer. Hum Mol Genet. (2014) 23:3898–905. doi: 10.1093/hmg/ddu087
44. Chew MH, Tan WS, Liu Y, Cheah PY, Loi CT, Tang CL. Genomics of Hereditary Colorectal Cancer: Lessons Learnt from 25 Years of the Singapore Polyposis Registry. Ann Acad Med Singapore. (2015) 44:290–6.
45. Syngal S, Brand RE, Church JM, Giardiello FM, Hampel HL, Burt RW. ACG clinical guideline: Genetic testing and management of hereditary gastrointestinal cancer syndromes. The American journal of gastroenterology. (2015) 110:223–62. doi: 10.1038/ajg.2014.435
46. Dyson JK, Rutter MD. Colorectal cancer in inflammatory bowel disease: what is the real magnitude of the risk? World J Gastroenterol. (2012) 18:3839–48. doi: 10.3748/wjg.v18.i29.3839
47. Krämer HU, Schöttker B, Raum E, Brenner H. Type 2 diabetes mellitus and colorectal cancer: meta-analysis on sex-specific differences. Eur J Cancer (Oxford, England: 1990). (2012) 48:1269–82. doi: 10.1016/j.ejca.2011.07.010
48. Kyrgiou M, Kalliala I, Markozannes G, Gunter MJ, Paraskevaidis E, Gabra H, et al. Adiposity and cancer at major anatomical sites: umbrella review of the literature. BMJ (Clinical research ed). (2017) 356:j477. doi: 10.1136/bmj.j477
49. Keighley MR, O'Morain C, Giacosa A, Ashorn M, Burroughs A, Crespi M, et al. Public awareness of risk factors and screening for colorectal cancer in Europe. Eur J Cancer Prevent. (2004) 13:257–62. doi: 10.1097/01.cej.0000136575.01493.9b
50. Cai S, Li Y, Ding Y, Chen K, Jin M. Alcohol drinking and the risk of colorectal cancer death: a meta-analysis. Eur J Cancer Prevent. (2014) 23:532–9. doi: 10.1097/CEJ.0000000000000076
51. Botteri E, Borroni E, Sloan EK, Bagnardi V, Bosetti C, Peveri G, et al. Smoking and Colorectal Cancer Risk, Overall and by Molecular Subtypes: a Meta-Analysis. Am J Gastroenterol. (2020) 115:1940–9. doi: 10.14309/ajg.0000000000000803
52. Botteri E, Iodice S, Bagnardi V, Raimondi S, Lowenfels AB, Maisonneuve P. Smoking and colorectal cancer: a meta-analysis. JAMA. (2008) 300:2765–78. doi: 10.1001/jama.2008.839
53. Luu HN, Wang R, Shivappa N, Hébert JR, Koh W-P, Yuan J-M, et al. prospective study of dietary inflammatory index scores and colorectal cancer risk: The Singapore Chinese Health Study. AACR. (2018). doi: 10.1158/1538-7445.AM2018-5264
54. Chan DS, Lau R, Aune D, Vieira R, Greenwood DC, Kampman E, et al. Red and processed meat and colorectal cancer incidence: meta-analysis of prospective studies. PLoS ONE. (2011) 6:e20456. doi: 10.1371/journal.pone.0020456
55. Nguyen LH, Liu PH, Zheng X, Keum N, Zong X, Li X, et al. Sedentary behaviors, TV viewing time, and risk of young-onset colorectal cancer. JNCI Cancer Spectrum. (2018) 2:pky073. doi: 10.1093/jncics/pky073
56. Alegria-Lertxundi I, Aguirre C, Bujanda L, Fernández FJ, Polo F, Ordovás JM, et al. Food groups, diet quality and colorectal cancer risk in the Basque Country. World J Gastroenterol. (2020) 26:4108–25. doi: 10.3748/wjg.v26.i28.4108
57. Baena R, Salinas P. Diet and colorectal cancer. Maturitas. (2015) 80:258–64. doi: 10.1016/j.maturitas.2014.12.017
58. Azeem S, Gillani SW, Siddiqui A, Jandrajupalli SB, Poh V, Syed Sulaiman SA. Diet and colorectal cancer risk in Asia—a systematic review. Asian Pac J Cancer Prevent. (2015) 16:5389–96. doi: 10.7314/APJCP.2015.16.13.5389
59. Borzì AM, Biondi A, Basile F, Luca S. Olive oil effects on colorectal cancer. Nutrients. (2018) 11:32. doi: 10.3390/nu11010032
60. Balhareth A, Aldossary MY. Impact of physical activity and diet on colorectal cancer survivors' quality of life: a systematic review World J Surg Oncol. (2019) 17:153. doi: 10.1186/s12957-019-1697-2
61. Schlesinger S, Walter J, Hampe J, von Schönfels W, Hinz S, Küchler T, et al. Lifestyle factors and health-related quality of life in colorectal cancer survivors. Cancer Causes Control. (2014) 25:99–110. doi: 10.1007/s10552-013-0313-y
62. Brenner H, Hoffmeister M, Arndt V, Haug U. Gender differences in colorectal cancer: implications for age at initiation of screening. Br J Cancer. (2007) 96:828–31. doi: 10.1038/sj.bjc.6603628
63. Low EE, Demb J, Liu L, Earles A, Bustamante R, Williams CD, et al. Risk factors for early-onset colorectal cancer. Gastroenterology. (2020) 159:492–501.e7. doi: 10.1053/j.gastro.2020.01.004
64. Sineshaw HM, Robbins AS, Jemal A. Disparities in survival improvement for metastatic colorectal cancer by race/ethnicity and age in the United States. Cancer Causes Control. (2014) 25:419–23. doi: 10.1007/s10552-014-0344-z
65. Bae JM, Kim JH, Kang GH. Molecular subtypes of colorectal cancer and their clinicopathologic features, with an emphasis on the serrated neoplasia pathway. Arch Pathol Lab Med. (2016) 140:406–12. doi: 10.5858/arpa.2015-0310-RA
66. Zauber AG, Winawer SJ, O'Brien MJ, Lansdorp-Vogelaar I, van Ballegooijen M, Hankey BF, et al. Colonoscopic polypectomy and long-term prevention of colorectal-cancer deaths. N Engl J Med. (2012) 366:687–96. doi: 10.1056/NEJMoa1100370
67. Vacante M, Ciuni R, Basile F. Gut microbiota and colorectal cancer development: a closer look to the adenoma-carcinoma sequence. Biomedicines. (2020) 8:489. doi: 10.3390/biomedicines8110489
68. Kinzler KW, Vogelstein B. Lessons from hereditary colorectal cancer. Cell. (1996) 87:159–70. doi: 10.1016/S0092-8674(00)81333-1
69. Fearon ER. Molecular genetics of colorectal cancer. Annu Rev Pathol. (2011) 6:479–507. doi: 10.1146/annurev-pathol-011110-130235
70. Vacante M, Borzì AM, Basile F, Biondi A. Biomarkers in colorectal cancer: current clinical utility and future perspectives. World J Clinical Cases. (2018) 6:869–81. doi: 10.12998/wjcc.v6.i15.869
71. Sievers CK, Grady WM, Halberg RB, Pickhardt PJ. New insights into the earliest stages of colorectal tumorigenesis. Expert Rev Gastroenterol Hepatol. (2017) 11:723–9. doi: 10.1080/17474124.2017.1330150
72. Leggett B, Whitehall V. Role of the serrated pathway in colorectal cancer pathogenesis. Gastroenterology. (2010) 138:2088–100. doi: 10.1053/j.gastro.2009.12.066
73. Kedrin D, Gala MK. Genetics of the serrated pathway to colorectal cancer. Clin Transl Gastroenterol. (2015) 6:e84. doi: 10.1038/ctg.2015.12
74. Yamane L, Scapulatempo-Neto C, Reis RM, Guimarães DP. Serrated pathway in colorectal carcinogenesis. World J Gastroenterol. (2014) 20:2634–40. doi: 10.3748/wjg.v20.i10.2634
75. Itzkowitz SH, Yio X. Inflammation and cancer IV. Colorectal cancer in inflammatory bowel disease: the role of inflammation. Am J Physiol Gastrointest Liver Physiol. (2004) 287:G7–17. doi: 10.1152/ajpgi.00079.2004
76. Triantafillidis JK, Nasioulas G, Kosmidis PA. Colorectal cancer and inflammatory bowel disease: epidemiology, risk factors, mechanisms of carcinogenesis and prevention strategies. Anticancer Res. (2009) 29:2727–37.
77. Watanabe T, Muro K, Ajioka Y, Hashiguchi Y, Ito Y, Saito Y, et al. Japanese Society for Cancer of the Colon and Rectum (JSCCR) guidelines 2016 for the treatment of colorectal cancer. Int J Clin Oncol. (2018) 23:1–34. doi: 10.1007/s10147-017-1101-6
78. Itatani Y. Kawada K. Treatment of Elderly Patients with Colorectal Cancer. Biomed Res Int. (2018) 2018:2176056. doi: 10.1155/2018/2176056
79. Panis Y, Maggiori L, Caranhac G, Bretagnol F, Vicaut E. Mortality after colorectal cancer surgery: a French survey of more than 84,000 patients. Ann Surg. (2011) 254:738–43. doi: 10.1097/SLA.0b013e31823604ac
80. Vallance AE, Keller DS, Hill J, Braun M, Kuryba A, van der Meulen J, et al. Role of emergency laparoscopic colectomy for colorectal cancer: a population-based study in England. Ann Surg. (2019) 270:172–9. doi: 10.1097/SLA.0000000000002752
81. Addison P, Agnew JL, Martz J. Robotic Colorectal Surgery. Surg Clin North Am. (2020) 100:337–60. doi: 10.1016/j.suc.2019.12.012
82. Ezekian B, Sun Z, Adam MA, Kim J, Turner MC, Gilmore BF, et al. Robotic-assisted versus laparoscopic colectomy results in increased operative time without improved perioperative outcomes. J Gastrointest Surg. (2016) 20:1503–10. doi: 10.1007/s11605-016-3124-0
83. Park JS, Kang H, Park SY, Kim HJ, Woo IT, Park IK., et al. Long-term oncologic after robotic versus laparoscopic right colectomy: a prospective randomized study. Surg Endosc. (2019) 33:2975–81. doi: 10.1007/s00464-018-6563-8
84. Chamseddine AN, Ducreux M, Armand JP, Paoletti X, Satar T, Paci A, et al. Intestinal bacterial β-glucuronidase as a possible predictive biomarker of irinotecan-induced diarrhea severity. Pharmacol Ther. (2019) 199:1–15. doi: 10.1016/j.pharmthera.2019.03.002
85. Cheng KW, Tseng CH, Tzeng CC, Leu YL, Cheng TC, Wang JY, et al. Pharmacological inhibition of bacterial β-glucuronidase prevents irinotecan-induced diarrhea without impairing its antitumor efficacy in vivo. Pharmacol Res. (2019) 139:41–9. doi: 10.1016/j.phrs.2018.10.029
86. Hattori T, Imaoka A. Irinotecan-induced gastrointestinal damage impairs the absorption of dabigatran etexilate. Biopharm Drug Dispos. (2019) 40:315–24. doi: 10.1002/bdd.2205
87. Suau R, Rico R, López-Romero JM, Nájera F, Cuevas A. Isoquinoline alkaloids from Berberis vulgaris subsp. australis. Phytochemistry. (1998) 49:2545–9. doi: 10.1016/S0031-9422(98)00121-6
88. Habtemariam S. The therapeutic potential of Berberis darwinii stem-bark: quantification of berberine and in vitro evidence for Alzheimer's disease therapy. Nat Prod Commun. (2011) 6:1089–90. doi: 10.1177/1934578X1100600809
89. Liu Y, Hao H, Xie H, Lv H, Liu C, Wang G. Oxidative demethylenation and subsequent glucuronidation are the major metabolic pathways of berberine in rats. J Pharm Sci. (2009) 98:4391–401. doi: 10.1002/jps.21721
90. Wang K, Feng X, Chai L, Cao S, Qiu F. The metabolism of berberine and its contribution to the pharmacological effects. Drug Metab Rev. (2017) 49:139–57. doi: 10.1080/03602532.2017.1306544
91. Guo Y, Li F, Ma X, Cheng X, Zhou H, Klaassen CD. CYP2D plays a major role in berberine metabolism in liver of mice and humans. Xenobiotica. (2011) 41:996–1005. doi: 10.3109/00498254.2011.597456
92. Qiu F, Zhu Z, Kang N, Piao S, Qin G, Yao X. Isolation and identification of urinary metabolites of berberine in rats and humans. Drug Metab Dispos. (2008) 36:2159–65. doi: 10.1124/dmd.108.021659
93. Kwon M, Lim DY, Lee CH, Jeon J-H, Choi M-K, Song I-S. Enhanced intestinal absorption and pharmacokinetic modulation of berberine and its metabolites through the inhibition of p-glycoprotein and intestinal metabolism in rats using a berberine mixed micelle formulation. Pharmaceutics. (2020) 12:882. doi: 10.3390/pharmaceutics12090882
94. Kuo CL, Chi CW, Liu TY. The anti-inflammatory potential of berberine in vitro and in vivo. Cancer Lett. (2004) 203:127–37. doi: 10.1016/j.canlet.2003.09.002
95. Haftcheshmeh SM, Abedi M, Mashayekhi K, Mousavi MJ, Navashenaq JG, Mohammadi A, et al. Berberine as a natural modulator of inflammatory signaling pathways in the immune system: Focus on NF-κB, JAK/STAT, and MAPK signaling pathways. Phytother Res. (2022) 36:1216–30. doi: 10.1002/ptr.7407
96. Mohammadian Haftcheshmeh S. Momtazi-Borojeni AA. Berberine as a promising natural compound for the treatment of periodontal disease: a focus on anti-inflammatory properties. J Cell Mol Med. (2021) 25:11333–7. doi: 10.1111/jcmm.17019
97. Warowicka A, Nawrot R, Gozdzicka-Józefiak A. Antiviral activity of berberine. Arch Virol. (2020) 165:1935–45. doi: 10.1007/s00705-020-04706-3
98. Rajasekhar K, Samanta S, Bagoband V, Murugan NA, Govindaraju T. Antioxidant berberine-derivative inhibits multifaceted amyloid toxicity. iScience. (2020) 23:101005. doi: 10.1016/j.isci.2020.101005
99. Ni WJ, Ding HH, Tang LQ. Berberine as a promising anti-diabetic nephropathy drug: an analysis of its effects and mechanisms. Eur J Pharmacol. (2015) 760:103–12. doi: 10.1016/j.ejphar.2015.04.017
100. Li YH, Xiao HT, Hu DD, Fatima S, Lin CY, Mu HX, et al. Berberine ameliorates chronic relapsing dextran sulfate sodium-induced colitis in C57BL/6 mice by suppressing Th17 responses. Pharmacol Res. (2016) 110:227–39. doi: 10.1016/j.phrs.2016.02.010
101. Feng X, Sureda A, Jafari S, Memariani Z, Tewari D, Annunziata G, et al. Berberine in cardiovascular and metabolic diseases: from mechanisms to therapeutics. Theranostics. (2019) 9:1923–51. doi: 10.7150/thno.30787
102. Fatahian A, Haftcheshmeh SM, Azhdari S, Farshchi HK, Nikfar B, Momtazi-Borojeni AA. Promising anti-atherosclerotic effect of berberine: evidence from in vitro, in vivo, and clinical studies. Rev Physiol Biochem Pharmacol. (2020) 178:83–110. doi: 10.1007/112_2020_42
103. Yuan NN, Cai CZ, Wu MY, Su HX Li M, Lu JH. Neuroprotective effects of berberine in animal models of Alzheimer's disease: a systematic review of pre-clinical studies. BMC Complement Altern Med. (2019) 19:109. doi: 10.1186/s12906-019-2510-z
104. Neag MA, Mocan A, Echeverría J, Pop RM, Bocsan CI, Crişan G, et al. Berberine: botanical occurrence, traditional uses, extraction methods, and relevance in cardiovascular, metabolic, hepatic, and renal disorders. Front Pharmacol. (2018) 9:557. doi: 10.3389/fphar.2018.00557
105. Wang S, Xu Z, Cai B, Chen Q. Berberine as a Potential multi-target agent for metabolic diseases: a review of investigations for berberine. Endocr Metab Immune Disord Drug Targets. (2021) 21:971–9. doi: 10.2174/1871530320666200910105612
106. Och A, Och M, Nowak R, Podgórska D, Podgórski R, Berberine. A herbal metabolite in the metabolic syndrome: the risk factors, course, and consequences of the disease. Molecules. (2022) 27:1351. doi: 10.3390/molecules27041351
107. Song D, Hao J, Fan D. Biological properties and clinical applications of berberine. Front Med. (2020) 14:564–82. doi: 10.1007/s11684-019-0724-6
108. Yu M, Jin X, Liang C, Bu F, Pan D, He Q, et al. Berberine for diarrhea in children and adults: a systematic review and meta-analysis. Therap Adv Gastroenterol. (2020) 13:1756284820961299. doi: 10.1177/1756284820961299
109. Aski ML, Rezvani ME, Khaksari M, Hafizi Z, Pirmoradi Z, Niknazar S, et al. Neuroprotective effect of berberine chloride on cognitive impairment and hippocampal damage in experimental model of vascular dementia. Iran J Basic Med Sci. (2018) 21:53–8.
110. Liu D, Meng X, Wu D, Qiu Z, Luo H A. Natural Isoquinoline Alkaloid With Antitumor Activity: Studies of the Biological Activities of Berberine. Front Pharmacol. (2019) 10:9. doi: 10.3389/fphar.2019.00009
111. Qi HW, Xin LY, Xu X, Ji XX, Fan LH. Epithelial-to-mesenchymal transition markers to predict response of Berberine in suppressing lung cancer invasion and metastasis. J Transl Med. (2014) 12:22. doi: 10.1186/1479-5876-12-22
112. Li H, Guo L, Jie S, Liu W, Zhu J, Du W, et al. Berberine inhibits SDF-1-induced AML cells and leukemic stem cells migration via regulation of SDF-1 level in bone marrow stromal cells. Biomed Pharmacother. (2008) 62:573–8. doi: 10.1016/j.biopha.2008.08.003
113. Xiao Y, Tian C, Huang T, Han B, Wang M, Ma H, et al. 8-Cetylberberine inhibits growth of lung cancer in vitro and in vivo. Life Sci. (2018) 192:259–69. doi: 10.1016/j.lfs.2017.11.012
114. Wang N, Wang X, Tan HY, Li S, Tsang CM, Tsao SW, et al. Berberine suppresses cyclin D1 expression through proteasomal degradation in human hepatoma cells. Int J Mol Sci. (2016) 17:1899. doi: 10.3390/ijms17111899
115. Li XK, Motwani M, Tong W, Bornmann W, Schwartz GK. Huanglian, A chinese herbal extract, inhibits cell growth by suppressing the expression of cyclin B1 and inhibiting CDC2 kinase activity in human cancer cells. Mol Pharmacol. (2000) 58:1287–93. doi: 10.1124/mol.58.6.1287
116. Wang J, Qi Q, Feng Z, Zhang X, Huang B, Chen A, et al. Berberine induces autophagy in glioblastoma by targeting the AMPK/mTOR/ULK1-pathway. Oncotarget. (2016) 7:66944–58. doi: 10.18632/oncotarget.11396
117. Wang N, Feng Y, Zhu M, Tsang CM, Man K, Tong Y, et al. Berberine induces autophagic cell death and mitochondrial apoptosis in liver cancer cells: the cellular mechanism. J Cell Biochem. (2010) 111:1426–36. doi: 10.1002/jcb.22869
118. Okubo S, Uto T, Goto A, Tanaka H, Nishioku T, Yamada K, et al. Berberine Induces apoptotic cell death via activation of caspase-3 and−8 in HL-60 human leukemia cells: nuclear localization and structure-activity relationships. Am J Chin Med. (2017) 45:1497–511. doi: 10.1142/S0192415X17500811
119. Li DX, Zhang J, Zhang Y, Zhao PW, Yang LM. Inhibitory effect of berberine on human skin squamous cell carcinoma A431 cells. Genet Mol Res. (2015) 14:10553–68. doi: 10.4238/2015.September.8.17
120. Jin H, Jin X, Cao B, Wang W. Berberine affects osteosarcoma via downregulating the caspase-1/IL-1β signaling axis. Oncol Rep. (2017) 37:729–36. doi: 10.3892/or.2016.5327
121. Ma W, Zhu M, Zhang D, Yang L, Yang T, Li X, et al. Berberine inhibits the proliferation and migration of breast cancer ZR-75-30 cells by targeting Ephrin-B2. Phytomedicine. (2017) 25:45–51. doi: 10.1016/j.phymed.2016.12.013
122. Chu SC Yu CC, Hsu LS, Chen KS, Su MY, Chen PN. Berberine reverses epithelial-to-mesenchymal transition and inhibits metastasis and tumor-induced angiogenesis in human cervical cancer cells. Mol Pharmacol. (2014) 86:609–23. doi: 10.1124/mol.114.094037
123. Liu JF, Lai KC. Berberine Inhibits Human Melanoma A375.S2 Cell Migration and Invasion via Affecting the FAK, uPA, and NF-κB Signaling Pathways and Inhibits PLX4032 Resistant A375.S2 Cell Migration In Vitro. Molecules. (2018) 23:2019. doi: 10.3390/molecules23082019
124. Lee H, Lee SJ, Bae GU, Baek NI, Ryu JH. Canadine from corydalis turtschaninovii stimulates myoblast differentiation and protects against myotube atrophy. Int J Mol Sci. (2017) 18:2748. doi: 10.3390/ijms18122748
125. Wang N, Zhu M, Wang X, Tan HY, Tsao SW, Feng Y. Berberine-induced tumor suppressor p53 up-regulation gets involved in the regulatory network of MIR-23a in hepatocellular carcinoma. Biochim Biophys Acta. (2014) 1839:849–57. doi: 10.1016/j.bbagrm.2014.05.027
126. Fu L, Chen W, Guo W, Wang J, Tian Y, Shi D, et al. Berberine Targets AP-2/hTERT, NF-κB/COX-2, HIF-1α/VEGF and Cytochrome-c/Caspase Signaling to Suppress Human Cancer Cell Growth. PLoS ONE. (2013) 8:e69240. doi: 10.1371/journal.pone.0069240
127. Tong M, Liu H, Hao J, Fan D. Comparative pharmacoproteomics reveals potential targets for berberine, a promising therapy for colorectal cancer. Biochem Biophys Res Commun. (2020) 525:244–50. doi: 10.1016/j.bbrc.2020.02.052
128. Yu YN, Yu TC, Zhao HJ, Sun TT, Chen HM, Chen HY, et al. Berberine may rescue Fusobacterium nucleatum-induced colorectal tumorigenesis by modulating the tumor microenvironment. Oncotarget. (2015) 6:32013–26. doi: 10.18632/oncotarget.5166
129. Su Y-H, Tang W-C, Cheng Y-W, Sia P, Huang C-C, Lee Y-C, et al. Targeting of multiple oncogenic signaling pathways by Hsp90 inhibitor alone or in combination with berberine for treatment of colorectal cancer. Biochimica et Biophysica Acta (BBA)-Mol Cell Res. (2015) 1853:2261–72. doi: 10.1016/j.bbamcr.2015.05.012
130. Zhang Y, Liu X, Yu M, Xu M, Xiao Y, Ma W, et al. Berberine inhibits proliferation and induces G0/G1 phase arrest in colorectal cancer cells by downregulating IGF2BP3. Life Sci. (2020) 260:118413. doi: 10.1016/j.lfs.2020.118413
131. Gong C, Hu X, Xu Y, Yang J, Zong L, Wang C, et al. Berberine inhibits proliferation and migration of colorectal cancer cells by downregulation of GRP78. Anticancer Drugs. (2020) 31:141–9. doi: 10.1097/CAD.0000000000000835
132. Liu H, Huang C, Wu L, Wen B. Effect of evodiamine and berberine on miR-429 as an oncogene in human colorectal cancer. Onco Targets Ther. (2016) 9:4121–7. doi: 10.2147/OTT.S104729
133. Dai W, Mu L, Cui Y, Li Y, Chen P, Xie H, et al. Berberine promotes apoptosis of colorectal cancer via regulation of the long non-coding RNA (lncRNA) cancer susceptibility candidate 2 (CASC2)/AU-Binding Factor 1 (AUF1)/B-Cell CLL/Lymphoma 2 (Bcl-2) Axis. Med Sci Monit Int Med J Exp Clin Res. (2019) 25:730–8. doi: 10.12659/MSM.912082
134. Wang L, Cao H, Lu N, Liu L, Wang B, Hu T, et al. Berberine inhibits proliferation and down-regulates epidermal growth factor receptor through activation of Cbl in colon tumor cells. PLoS ONE. (2013) 8:e56666. doi: 10.1371/journal.pone.0056666
135. Samad MA, Saiman MZ, Abdul Majid N. Berberine inhibits telomerase activity and induces cell cycle arrest and telomere erosion in colorectal cancer cell line, HCT 116. Molecules. (2021) 26:376. doi: 10.3390/molecules26020376
136. Liu X, Ji Q, Ye N, Sui H, Zhou L, Zhu H, et al. Berberine inhibits invasion and metastasis of colorectal cancer cells via COX-2/PGE2 mediated JAK2/STAT3 signaling pathway. PLoS ONE. (2015) 10:e0123478. doi: 10.1145/2818302
137. Lü Y, Han B, Yu H, Cui Z, Li Z, Wang J. Berberine regulates the microRNA-21-ITG?4-PDCD4 axis and inhibits colon cancer viability. Oncol Lett. (2018) 15:5971–6.
138. Dai W, Mu L, Cui Y, Li Y, Chen P, Xie H, et al. Long non-coding RNA CASC2 enhances berberine-induced cytotoxicity in colorectal cancer cells by silencing BCL2. Mol Med Rep. (2019) 20:995–1006. doi: 10.3892/mmr.2019.10326
139. Huang C, Liu H, Gong XL, Wu LY, Wen B. Effect of evodiamine and berberine on the interaction between DNMTs and target microRNAs during malignant transformation of the colon by TGF-β1. Oncol Rep. (2017) 37:1637–45. doi: 10.3892/or.2017.5379
140. Soffar AA. Berberine attenuates cancer cell growth via modulating the cell cycle dynamics but not apoptosis in human colorectal HCT-116 3D spheroid model. J Biosci Appl Res. (2019) 5:236–45. doi: 10.21608/jbaar.2019.146799
141. Liu Y, Hua W, Li Y, Xian X, Zhao Z, Liu C, et al. Berberine suppresses colon cancer cell proliferation by inhibiting the SCAP/SREBP-1 signaling pathway-mediated lipogenesis. Biochem Pharmacol. (2020) 174:113776. doi: 10.1016/j.bcp.2019.113776
142. Li W, Hua B, Saud SM, Lin H, Hou W, Matter MS, et al. Berberine regulates AMP-activated protein kinase signaling pathways and inhibits colon tumorigenesis in mice. Mol Carcinog. (2015) 54:1096–109. doi: 10.1002/mc.22179
143. Li D, Zhang Y, Liu K, Zhao Y, Xu B, Xu L, et al. Berberine inhibits colitis-associated tumorigenesis via suppressing inflammatory responses and the consequent EGFR signaling-involved tumor cell growth. Lab Invest. (2017) 97:1343–53. doi: 10.1038/labinvest.2017.71
144. Wu K, Yang Q, Mu Y, Zhou L, Liu Y, Zhou Q, et al. Berberine inhibits the proliferation of colon cancer cells by inactivating Wnt/β-catenin signaling. Int J Oncol. (2012) 41:292–8.
145. Deng J, Zhao L, Yuan X, Li Y, Shi J, Zhang H, et al. Pre-administration of berberine exerts chemopreventive effects in aom/dss-induced colitis-associated carcinogenesis mice via modulating inflammation and intestinal microbiota. Nutrients. (2022) 14:726. doi: 10.3390/nu14040726
146. Vermeulen K, Van Bockstaele DR, Berneman ZN. The cell cycle: a review of regulation, deregulation and therapeutic targets in cancer. Cell Prolif. (2003) 36:131–49. doi: 10.1046/j.1365-2184.2003.00266.x
147. Shacter E, Weitzman SA. Chronic inflammation and cancer. Oncology (Williston Park, NY). (2002) 16:217–26.
148. Watanabe T, Konishi T, Kishimoto J, Kotake K, Muto T, Sugihara K. Ulcerative colitis-associated colorectal cancer shows a poorer survival than sporadic colorectal cancer: a nationwide Japanese study. Inflamm Bowel Dis. (2011) 17:802–8. doi: 10.1002/ibd.21365
149. Zhen Y, Luo C, Zhang H. Early detection of ulcerative colitis-associated colorectal cancer. Gastroenterol Rep (Oxf). (2018) 6:83–92. doi: 10.1093/gastro/goy010
150. Carini F, Mazzola M, Rappa F, Jurjus A, Geagea AG, Al Kattar S, et al. Colorectal carcinogenesis: role of oxidative stress and antioxidants. Anticancer Res. (2017) 37:4759–66. doi: 10.21873/anticanres.11882
151. Jackson AL, Chen R, Loeb LA. Induction of microsatellite instability by oxidative DNA damage. Proc Natl Acad Sci USA. (1998) 95:12468–73. doi: 10.1073/pnas.95.21.12468
152. Kraus S, Arber N. Inflammation and colorectal cancer. Curr Opin Pharmacol. (2009) 9:405–10. doi: 10.1016/j.coph.2009.06.006
153. Federico A, Morgillo F, Tuccillo C, Ciardiello F, Loguercio C. Chronic inflammation and oxidative stress in human carcinogenesis. Int J Cancer. (2007) 121:2381–6. doi: 10.1002/ijc.23192
154. Zhu L, Gu P, Shen H. Protective effects of berberine hydrochloride on DSS-induced ulcerative colitis in rats. Int Immunopharmacol. (2019) 68:242–51. doi: 10.1016/j.intimp.2018.12.036
155. Azimirad M, Rostami-Nejad M, Rostami K, Naji T, Zali MR. The susceptibility of celiac disease intestinal microbiota to clostridium difficile infection. Am J Gastroenterol. (2015) 110:1740–1. doi: 10.1038/ajg.2015.360
156. Rostami Nejad M, Ishaq S, Al Dulaimi D, Zali MR, Rostami K. The role of infectious mediators and gut microbiome in the pathogenesis of celiac disease. Arch Iran Med. (2015) 18:244–9.
157. Li D, Wang P, Wang P, Hu X, Chen F. The gut microbiota: a treasure for human health. Biotechnol Adv. (2016) 34:1210–24. doi: 10.1016/j.biotechadv.2016.08.003
158. Saffarian A, Mulet C, Regnault B, Amiot A, Tran-Van-Nhieu J, Ravel J. Crypt- and mucosa-associated core microbiotas in humans and their alteration in colon cancer patients. mBio. (2019) 10:e01315–19. doi: 10.1128/mBio.01315-19
159. Wang T, Cai G, Qiu Y, Fei N, Zhang M, Pang X, et al. Structural segregation of gut microbiota between colorectal cancer patients and healthy volunteers. ISME J. (2012) 6:320–9. doi: 10.1038/ismej.2011.109
160. Rubinstein MR, Wang X, Liu W, Hao Y, Cai G, Han YW. Fusobacterium nucleatum promotes colorectal carcinogenesis by modulating E-cadherin/β-catenin signaling via its FadA adhesin. Cell Host Microbe. (2013) 14:195–206. doi: 10.1016/j.chom.2013.07.012
161. Kostic AD, Chun E, Robertson L, Glickman JN, Gallini CA, Michaud M, et al. Fusobacterium nucleatum potentiates intestinal tumorigenesis and modulates the tumor-immune microenvironment. Cell Host Microbe. (2013) 14:207–15. doi: 10.1016/j.chom.2013.07.007
162. Wong SH. Yu J. Gut microbiota in colorectal cancer: mechanisms of action and clinical applications. Nat Rev Gastroenterol Hepatol. (2019) 16:690–704. doi: 10.1038/s41575-019-0209-8
163. Cipe G, Idiz UO, Firat D, Bektasoglu H. Relationship between intestinal microbiota and colorectal cancer. World J Gastrointest Oncol. (2015) 7:233–40. doi: 10.4251/wjgo.v7.i10.233
164. Loke YL, Chew MT, Ngeow YF, Lim WWD, Peh SC. Colon carcinogenesis: the interplay between diet and gut microbiota. Front Cell Infect Microbiol. (2020) 10:603086. doi: 10.3389/fcimb.2020.603086
165. Dzutsev A, Goldszmid RS, Viaud S, Zitvogel L, Trinchieri G. The role of the microbiota in inflammation, carcinogenesis, and cancer therapy. Eur J Immunol. (2015) 45:17–31. doi: 10.1002/eji.201444972
166. Sun M, Wu W, Chen L, Yang W, Huang X, Ma C, et al. Microbiota-derived short-chain fatty acids promote Th1 cell IL-10 production to maintain intestinal homeostasis. Nat Commun. (2018) 9:3555. doi: 10.1038/s41467-018-05901-2
167. Habtemariam S. Berberine pharmacology and the gut microbiota: a hidden therapeutic link. Pharmacol Res. (2020) 155:104722. doi: 10.1016/j.phrs.2020.104722
168. Cui HX, Hu YN, Li JW, Yuan K, Guo Y. Preparation and evaluation of antidiabetic agents of berberine organic acid salts for enhancing the bioavailability. Molecules (Basel, Switzerland). (2018) 24:103. doi: 10.3390/molecules24010103
169. Guo Y, Zhang Y, Huang W, Selwyn FP, Klaassen CD. Dose-response effect of berberine on bile acid profile and gut microbiota in mice. BMC Complement Altern Med. (2016) 16:394. doi: 10.1186/s12906-016-1367-7
170. Xie W, Gu D, Li J, Cui K, Zhang Y. Effects and action mechanisms of berberine and Rhizoma coptidis on gut microbes and obesity in high-fat diet-fed C57BL/6J mice. PLoS ONE. (2011) 6:e24520. doi: 10.1371/journal.pone.0024520
171. van Passel MW, Kant R, Zoetendal EG, Plugge CM, Derrien M, Malfatti SA, et al. The genome of Akkermansia muciniphila, a dedicated intestinal mucin degrader, and its use in exploring intestinal metagenomes. PLoS ONE. (2011) 6:e16876. doi: 10.1371/journal.pone.0016876
172. Coleman OI, Haller D. Microbe-Mucus Interface in the Pathogenesis of Colorectal Cancer. Cancers. (2021) 13:616. doi: 10.3390/cancers13040616
173. Wang H, Guan L, Li J, Lai M, Wen X. The effects of berberine on the gut microbiota in Apc (min/+) mice fed with a high fat diet. Molecules (Basel, Switzerland). (2018) 23:2298. doi: 10.3390/molecules23092298
174. Cui H, Cai Y, Wang L, Jia B, Li J, Zhao S, et al. Berberine regulates Treg/Th17 balance to treat ulcerative colitis through modulating the gut microbiota in the colon. Front Pharmacol. (2018) 9:571. doi: 10.3389/fphar.2018.00571
175. He Y, Yuan X, Zuo H, Sun Y, Feng A. Berberine exerts a protective effect on gut-vascular barrier via the modulation of the Wnt/beta-catenin signaling pathway during sepsis. Cell Physiol Biochem. (2018) 49:1342–51. doi: 10.1159/000493412
176. Wu YY Li TM, Zang LQ, Liu B, Wang GX. Effects of berberine on tumor growth and intestinal permeability in HCT116 tumor-bearing mice using polyamines as targets. Biomed Pharmacother. (2018) 107:1447–53. doi: 10.1016/j.biopha.2018.08.130
177. Selvan SR, Dowling JP, Kelly WK, Lin J. Indoleamine 2,3-dioxygenase (IDO): biology and target in cancer immunotherapies. Curr Cancer Drug Targets. (2016) 16:755–64. doi: 10.2174/1568009615666151030102250
178. King NJ, Thomas SR. Molecules in focus: indoleamine 2,3-dioxygenase. Int J Biochem Cell Biol. (2007) 39:2167–72. doi: 10.1016/j.biocel.2007.01.004
179. Ciorba MA. Indoleamine 2,3 dioxygenase in intestinal disease. Curr Opin Gastroenterol. (2013) 29:146–52. doi: 10.1097/MOG.0b013e32835c9cb3
180. Moffett JR, Namboodiri MA. Tryptophan and the immune response. Immunol Cell Biol. (2003) 81:247–65. doi: 10.1046/j.1440-1711.2003.t01-1-01177.x
181. Thaker AI, Rao MS, Bishnupuri KS, Kerr TA, Foster L, Marinshaw JM, et al. IDO1 metabolites activate β-catenin signaling to promote cancer cell proliferation and colon tumorigenesis in mice. Gastroenterology. (2013) 145:416–25.e1–4. doi: 10.1053/j.gastro.2013.05.002
182. Hacking S, Vitkovski T, Jain S, Jin C, Chavarria H, Wu D, et al. Clinical significance of program death ligand-1 and indoleamine-2,3-dioxygenase expression in colorectal carcinoma. Appl Immunohistochem Mol Morphol. (2021) 29:201–8. doi: 10.1097/PAI.0000000000000868
183. Ferdinande L, Decaestecker C, Verset L, Mathieu A, Moles Lopez X, Negulescu AM, et al. Clinicopathological significance of indoleamine 2,3-dioxygenase 1 expression in colorectal cancer. Br J Cancer. (2012) 106:141–7. doi: 10.1038/bjc.2011.513
184. Chen IC, Lee KH. Expression Pattern and Clinicopathological Relevance of the Indoleamine 2,3-Dioxygenase 1/Tryptophan 2,3-Dioxygenase Protein in Colorectal Cancer. Dis Markers. (2016) 2016:8169724. doi: 10.1155/2016/8169724
185. Ma WJ, Wang X, Yan WT, Zhou ZG, Pan ZZ, Chen G, et al. Indoleamine-2,3-dioxygenase 1/cyclooxygenase 2 expression prediction for adverse prognosis in colorectal cancer. World J Gastroenterol. (2018) 24:2181–90. doi: 10.3748/wjg.v24.i20.2181
186. Yu CJ, Zheng MF, Kuang CX, Huang WD, Yang Q. Oren-gedoku-to and its constituents with therapeutic potential in Alzheimer's disease inhibit indoleamine 2, 3-dioxygenase activity in vitro. J Alzheimer's Dis. (2010) 22:257–66. doi: 10.3233/JAD-2010-100684
187. Wang YX, Pang WQ, Zeng QX, Deng ZS, Fan TY, Jiang JD, et al. Synthesis and biological evaluation of new berberine derivatives as cancer immunotherapy agents through targeting IDO1. Eur J Med Chem. (2018) 143:1858–68. doi: 10.1016/j.ejmech.2017.10.078
188. Mohammadzadeh N, Mehri S, Hosseinzadeh H. Berberis vulgaris and its constituent berberine as antidotes and protective agents against natural or chemical toxicities. Iran J Basic Med Sci. (2017) 20:538–51.
189. Tan YZ, Chan WA, Tan BY, Heng WJ, Tang LF, Hao LJ, et al. Study on the interactions of berberine displace other drug from their plasma proteins binding sites. Chin Pharmacol Bull. (2002) 5:576–8.
190. Bagade A, Tumbigeremutt V, Pallavi G. Cardiovascular effects of berberine: a review of the literature. J Restor Med. (2017) 6:37–45. doi: 10.14200/jrm.2017.6.0100
191. Cannillo M, Frea S, Fornengo C, Toso E, Mercurio G, Battista S, et al. Berberine behind the thriller of marked symptomatic bradycardia. World J Cardiol. (2013) 5:261–4. doi: 10.4330/wjc.v5.i7.261
192. Yin J, Xing H, Ye J. Efficacy of berberine in patients with type 2 diabetes mellitus. Metabolism. (2008) 57:712–7. doi: 10.1016/j.metabol.2008.01.013
193. Dong H, Wang N, Zhao L, Lu F. Berberine in the treatment of type 2 diabetes mellitus: a systemic review and meta-analysis. Evid Based Complement Alternat Med. (2012) 2012:591654. doi: 10.1155/2012/591654
194. Fang L-H, Wang J-H, Du G-H. Berberine. Natural Small Molecule Drugs from Plants. Berlin: Springer (2018). p. 371–7. doi: 10.1007/978-981-10-8022-7_62
195. Qiu W, Jiang XH, Liu CX, Ju Y, Jin JX. Effect of berberine on the pharmacokinetics of substrates of CYP3A and P-gp. Phytother Res. (2009) 23:1553–8. doi: 10.1002/ptr.2808
196. Shan YQ, Zhu YP, Pang J, Wang YX, Song DQ, Kong WJ, et al. Tetrandrine potentiates the hypoglycemic efficacy of berberine by inhibiting P-glycoprotein function. Biol Pharm Bull. (2013) 36:1562–9. doi: 10.1248/bpb.b13-00272
197. Zhang Y, Guo L, Huang J. Inhibitory effect of berberine on broiler p-glycoprotein expression and function: in situ and in vitro studies. Int J Mol Sci. (2019) 20:1966. doi: 10.3390/ijms20081966
198. Vanti G, Coronnello M, Bani D. Co-delivery of berberine chloride and tariquidar in nanoliposomes enhanced intracellular berberine chloride in a doxorubicin-resistant K562 cell line due to P-gp overexpression. Pharmaceutics. (2021) 13:306. doi: 10.3390/pharmaceutics13030306
199. Chen W, Miao YQ, Fan DJ, Yang SS, Lin X, Meng LK, et al. Bioavailability study of berberine and the enhancing effects of TPGS on intestinal absorption in rats. AAPS PharmSciTech. (2011) 12:705–11. doi: 10.1208/s12249-011-9632-z
200. Grebinyk A, Prylutska S, Grebinyk S, Evstigneev M, Krysiuk I, Skaterna T, et al. Antitumor efficiency of the natural alkaloid Berberine complexed with C60 fullerene in Lewis lung carcinoma in vitro and in vivo. Cancer Nanotechnol. (2021) 12:1–18. doi: 10.1186/s12645-021-00096-6
201. Nguyen MC-N, Le KN-M, Huynh NT-T, Nguyen TT-T, Bui HT-X, Nguyen VH editors. Solid lipid nanoparticles containing berberine by spray-drying method. Cham: Springer International Publishing (2022). doi: 10.1007/978-3-030-75506-5_25
202. Sahibzada MUK, Sadiq A, Faidah HS, Khurram M, Amin MU, Haseeb A, et al. Berberine nanoparticles with enhanced in vitro bioavailability: characterization and antimicrobial activity. Drug Des Devel Ther. (2018) 12:303–12. doi: 10.2147/DDDT.S156123
203. Majidzadeh H, Araj-Khodaei M, Ghaffari M, Torbati M, Ezzati Nazhad Dolatabadi J, Hamblin MR. Nano-based delivery systems for berberine: a modern anti-cancer herbal medicine. Colloids Surf B, Biointer. (2020) 194:111188. doi: 10.1016/j.colsurfb.2020.111188
204. Xin Y, Yin M, Zhao L, Meng F, Luo L. Recent progress on nanoparticle-based drug delivery systems for cancer therapy. Cancer Biol Med. (2017) 14:228–41. doi: 10.20892/j.issn.2095-3941.2017.0052
205. Bregoli L, Movia D, Gavigan-Imedio JD, Lysaght J, Reynolds J, Prina-Mello A. Nanomedicine applied to translational oncology: a future perspective on cancer treatment. Nanomed Nanotechnol Biol Med. (2016) 12:81–103. doi: 10.1016/j.nano.2015.08.006
Keywords: berberine, colorectal cancer, antitumor, gut microbiota, mucosal barrier
Citation: Jiang X, Jiang Z, Jiang M and Sun Y (2022) Berberine as a Potential Agent for the Treatment of Colorectal Cancer. Front. Med. 9:886996. doi: 10.3389/fmed.2022.886996
Received: 01 March 2022; Accepted: 29 March 2022;
Published: 28 April 2022.
Edited by:
Qi Liu, Fudan University, ChinaReviewed by:
Dhirendra Pratap Singh, National Institute of Occupational Health (ICMR), IndiaCopyright © 2022 Jiang, Jiang, Jiang and Sun. This is an open-access article distributed under the terms of the Creative Commons Attribution License (CC BY). The use, distribution or reproduction in other forums is permitted, provided the original author(s) and the copyright owner(s) are credited and that the original publication in this journal is cited, in accordance with accepted academic practice. No use, distribution or reproduction is permitted which does not comply with these terms.
*Correspondence: Yan Sun, eWFuczE5ODZAc2luYS5jb20=
Disclaimer: All claims expressed in this article are solely those of the authors and do not necessarily represent those of their affiliated organizations, or those of the publisher, the editors and the reviewers. Any product that may be evaluated in this article or claim that may be made by its manufacturer is not guaranteed or endorsed by the publisher.
Research integrity at Frontiers
Learn more about the work of our research integrity team to safeguard the quality of each article we publish.