- 1Laboratory of Histology-Embryology, Medical School, University of Crete, Heraklion, Greece
- 2Immunology Laboratory, Victor Babes National Institute of Pathology, Bucharest, Romania
- 3Colentina Hospital, Bucharest, Romania
- 4Doctoral School, University of Bucharest, Bucharest, Romania
- 5Forensic Science Department, Medical School, University of Crete, Heraklion, Greece
Basal cell carcinoma (BCC) and cutaneous squamous cell carcinoma (cSCC), referred to as keratinocyte carcinomas, are skin cancer with the highest incidence. BCCs, rarely metastasize; whereas, though generally not characterized by high lethality, approximately 2–4% of primary cSCCs metastasize with patients exhibiting poor prognosis. The extracellular matrix (ECM) serves as a scaffold that provides structural and biological support to cells in all human tissues. The main components of the ECM, including fibrillar proteins, proteoglycans (PGs), glycosaminoglycans (GAGs), and adhesion proteins such as fibronectin, are secreted by the cells in a tissue-specific manner, critical for the proper function of each organ. The skin compartmentalization to the epidermis and dermis compartments is based on a basement membrane (BM), a highly specialized network of ECM proteins that separate and unify the two compartments. The stiffness and assembly of BM and tensile forces affect tumor progenitors' invasion at the stratified epithelium's stromal border. Likewise, the mechanical properties of the stroma, e.g., stiffness, are directly correlated to the pathogenesis of the keratinocyte carcinomas. Since the ECM is a pool for various growth factors, cytokines, and chemokines, its' intense remodeling in the aberrant cancer tissue milieu affects biological functions, such as angiogenesis, adhesion, proliferation, or cell motility by regulating specific signaling pathways. This review discusses the structural and functional modulations of the keratinocyte carcinoma microenvironment. Furthermore, we debate how ECM remodeling affects the pathogenesis of these skin cancers.
Introduction
“Keratinocyte carcinomas” refers to non-melanoma skin cancers (NMSCs), and the term usually defines basal cell carcinoma (BCC) and cutaneous squamous cell carcinoma (cSCC) (1). BCC is the most common malignancy worldwide, and cSCC is the most common metastatic skin cancer, the incidence of both increasing globally (2). BCC develops from basal cells, the deepest part of the epidermis layer, which moves upward to replenish the skin's barrier. cSCC arises from differentiated squamous keratinocytes populating the upper epidermis layers (3). Though generally not characterized by high lethality (1), approximately 2–4% of primary cSCCs metastasize. The prognosis of patients with metastatic cSCC is poor, highlighting the urgent need for novel therapeutic approaches and aggressive behavior biomarkers (4). In addition, in some cases, BCC attains metastasis potential and resistance to therapy due to smoothened (SMO) inhibitor resistance (5).
UV irradiation, the most common cause of NMSCs is correlated to the high mutation rate of cSCC (6). Moreover, additional factors, such as non-coding RNAs expression, are required to progress premalignant lesion, actinic keratosis (AK), to cSCC in situ (cSCCIS). In addition, UV-radiation triggering of oxidant production induces modulation of inflammatory signaling, resulting in immune suppression (7). Indeed, UV-radiation enhances transforming growth factor-β1 (TGF-β1) upregulation resulting in angiogenesis, inflammation, cancer-associated fibroblasts generation, and immune suppression, thus facilitating cancer survival, immune evasion, and finally metastasis (8). Moreover, several skin pathologic conditions such as recessive dystrophic epidermolysis bullosa (RDEB) (9), severe burns, or chronic ulcers may favor NMSC development (2). Both males and females are affected equally, while incidence increases with age.
The extracellular matrix (ECM) serves as a scaffold that provides structural and biological support to cells in all human tissues. The main components of the ECM, including fibrillar proteins proteoglycans (PGs), glycosaminoglycans (GAGs), and adhesion proteins such as fibronectin, are secreted by the cells in a tissue-specific manner, critical for the proper function of each organ (10). The ECM is classified into the pericellular, interstitial, and highly organized basement membranes (BM) (10).
Intriguingly, the biomolecules that constitute the ECM have attained structural and physicochemical properties that specifically facilitate the execution of their tissue-related biological functions (11). Thus, the abundant collagen fibers determine tissue's stiffness and mechanical strength. Furthermore, the heteropolysaccharides, GAGs, form molecular bridges between collagen fibrils to affect ECM viscoelasticity and contribute to tissues' compressive stiffness. Indeed, alterations in the orientation, density, crosslinking, and interactions of fibers induce aberrant matrix stiffness correlated to malignant tissue transformation (12).
Likewise, the ECM is a pool for various growth factors, cytokines, and chemokines (11). Therefore, the intense remodeling of the ECM in the aberrant cancer tissue milieu (12) affects biological functions such as angiogenesis, adhesion, proliferation, or cell motility by regulating specific signaling pathways (13, 14). In addition, the remodeling of ECM and tumor microenvironment facilitates the progression from premalignant forms such as actinic keratosis to invasive and metastatic cSCC (15).
Multilayer Epithelium Mechanics in NMSCs
Mammalian skin comprises a multi-layered epithelium, e.g., the epidermis, dermis, and underlying connective tissue. The epidermis primarily consists of epithelial keratinocytes interconnected with enforced cell junctions (16). The skin compartmentalization to the epidermis and dermis compartments is based on a BM, a highly specialized network of ECM proteins that separate and unify the two compartments. The architecture of the BM is involved in tumor progression (17). Thus, BCCs, dependent on SmoM2 constitutive activators of Sonic hedgehog signaling (Shh), bud inward into the stroma, retaining their BM and rarely metastasizing (18). In contrast, RAS/MAPK-dependent SCCs commence as bidirectional tissue folds and exhibit invasiveness (19). The generation of SmoM2 and Ras respective mutants show changes in the expression of collagen 4a1/2 (COL4a1/2), Nidogen 1 (Nid1), and Sparc BM components.
Furthermore, in SCC, the mechanical forces exerted by overlying differentiated cells are sufficiently strong to confer loss of membrane integrity. Therefore, the stiffness and assembly of BM and tensile forces affect tumor progenitors' invasion at the stratified epithelium's stromal border (5, 20). In a separate study, Nid1 and COL4 expressions were decreased around the tumor nest of SCC but increased around the nest of BCC, further supporting BM input to the carcinoma phenotype. The strong expression of Nid1 and COL4 by stromal cells surrounding BCC, in contrast to cSCC, may prevent BCC cells from degrading the BM and invading the dermis (21).
Mechanical Remodeling of NMSCs Stroma
The dermis consists of an abundant ECM, into which blood vessels, skin appendages, fibroblasts, and other mesenchymal cells are embedded (16). Notably, the mechanical organization of the ECM surrounding NMKC cells significantly differs compared to normal skin ECM (22). In contrast to epithelial cells, mesenchymal cells exhibit extensive cell-matrix interactions, generating strong contractile forces with increased cytoskeletal activities (23). At the injury site, fibroblasts transform into highly contractile myofibroblasts, characterized by enhanced cytoskeleton allowing them to exert more pronounced mechanical force to the surrounding ECM, which enhances physiological goals such as wound closure but promotes carcinogenesis (23, 24).
Indeed, the stroma's mechanical properties, e.g., stiffness, are directly correlated to the pathogenesis of the NMKCs. Thus, patients with the highly disabling genodermatosis, the recessive dystrophic epidermolysis bullosa (RDEB), carry mutations in the COL7A1 gene encoding for type seven collagen, the main component of anchoring fibrils supporting cell binding to the BM. The majority of these patients progress to a highly aggressive type of cSCC (9). Thus, the resulting incompetence of the BM results in an inflammatory stroma profile where activated dermal fibroblasts promote cSCC development by creating a stiff, fibrotic ECM consisting of thick COL1 bundles, fibrinogen, and tenascin-C (25). Aspects of matrix-derived mechanotransduction signals are schematically depicted in Figure 1. Therefore, modifying the mechanotransduction signals could lead to novel therapeutic approaches for skin disease.
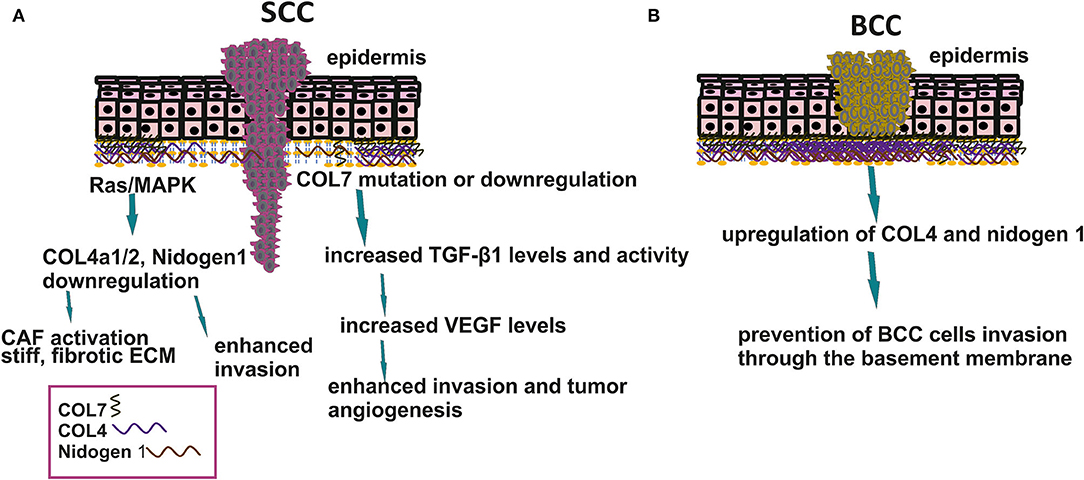
Figure 1. Basement membrane organization in SCC and BCC. (A) Downregulation in BM components enhances SCC cell invasion to the stroma affects matrix stiffness and tumor angiogenesis. (B) Upregulation of COL4 and nidogen 1 prevents BCC cells to metastasize.
ECM Effectors in NMSCs
Hyaluronic Acid (HA) and Its Receptors
Hyaluronan (HA) is a significant component of skin ECM. This GAG is a linear, non-sulfated chain composed of repeating disaccharides of N-acetylglucosamine and glucuronic acid units (26). HA is synthesized by the three Hyaluronan Synthases (HAS1,2 and 3) isoforms as a high molecular weight product (HMWHA) (27). HASs are localized to the plasma membrane, and the newly synthesized HA chain is protruded to the ECM (26, 27). The degradation of HA to lower molecular weight HA (LMWHA) fragments is mediated by glycosidases, denominated hyaluronidases (HYALs).
HA exerts its biological functions via specific receptors, including the cluster of differentiation 44 (CD44) encoded by one gene but presenting several isoforms due to alternative splicing (28). HA also acts through the receptor for hyaluronan mediated motility (RHAMM), upregulated in cSCC (29). Toll-like receptor 4 (TLR4) is a transmembrane HA receptor whose signaling has been correlated to both skin inflammation (14) and cSCC progression (30). HA exerts strictly size-dependent biological effects. Thus, HMWHA exhibits anti-inflammatory, immunosuppressive, and anti-angiogenic properties, while LMWHA induces angiogenic, inflammatory, and tumorigenic impact (27).
HA metabolism is correlated to the pathogenesis of NMKCs. Thus, SSC tumors cells express alternative isoforms of CD44, associated with enhanced cell growth and cancer development (31). Furthermore, CD44 receptors can bind HA of a broad molecular weight range resulting in discrete downstream signaling. Thus, HMWHA through CD44/Rac-signaling in UV exposed mice promoted keratinocyte survival, normal differentiation, and DNA repair. In contrast, LMWHA-CD44 interactions facilitated a RhoA activation/NFκB/Stat-3 signaling/miR-21 production axis, leading to inflammation, abnormal keratinocyte proliferation, and SCC progression (31). Moreover, exposure of mice to chronic UV irradiation, the most common cause of cSCC, increased CD44 expression in mouse epidermis, while 20% of the exposed animals developed SCC tumors. Indeed, in this study, UV also upregulated HAS levels and HA synthesis, indicating the existence of a CD44/HA signaling axis (32). A similar upregulation of HAS2 and 3 was observed upon UV treatment in primary NHEK(F) cells. However, blocking interleukin-1β (Il-1β) activity minimized this effect, implying an interaction of HA metabolism with inflammatory signaling (33).
Interestingly, a combination of CD29 and CD44 was suggested to identify cancer stem-like cells (CSC) undergoing EMT in SCC. Thus, CD29high/CD44high expressing SCC cells were found to exhibit molecular markers of EMT, indicating that CSC-associated mechanisms contribute to the process of EMT (34). Moreover, in a separate study, a high CD44 phenotype in SCC is suggested to identify candidates for implementing targeted therapy aiming at CSCs (34).
Even though early studies did not detect a correlation between the CD44v isoform expression and SCC metastatic potential, recent reports show an enhanced expression of CD44v3-10, CD44v6-10, and CD44v8-10 isoforms co-expressed with CD44s and podoplanin in human SCC cell lines (35). Moreover, CD44 and the cell membrane glycoprotein, podoplanin, facilitated directional motility in epithelial cells and were shown to enhance SCC cell's directional migration.
Early studies implementation immunohistology show that BCCs displayed very low expression of CD44s, whereas the expression of CD44v6 exhibited a heterogeneous distribution pattern enhanced in the peripheral palisading tumor cells. In superficial BCCs, the labeling intensity for CD44v6 increased with the size of the tumor nests. These authors suggested that CD44v6 does not correlate with BCC metastatic potential, while the low CD44s is indicated as attenuating for BCCs metastasis (36). Moreover, in BCC, CD44 expression was reduced in metastasizing compared to non-metastasizing tumors. Together with increased Twist1 expression, this may suggest a critical EMT input in metastasizing tumors and characterize a metastatic phenotype (37). On the other hand, CD44 was one of the markers upregulated in BCC tumors compared to margins and controls (38). These data highlight the need for further study on the implications of the CD44/HA signaling axis and especially the determination of the expression of specific CD44 isoforms (39).
Notably, RHAMM has been related to keratinocyte motility, essential for wound healing and metastasis (40).
TLR4 is widely known for its implication in skin inflammatory responses, including allergic dermatitis (10). Moreover, in NMSC, it is overexpressed (41), whereas; in TLR4 knock-out mice, the progression of NKCS tumors was attenuated (42). The above data highlight the critical role of HA and its receptors in the mechanisms regulating the onset of NKCSs (Figure 2).
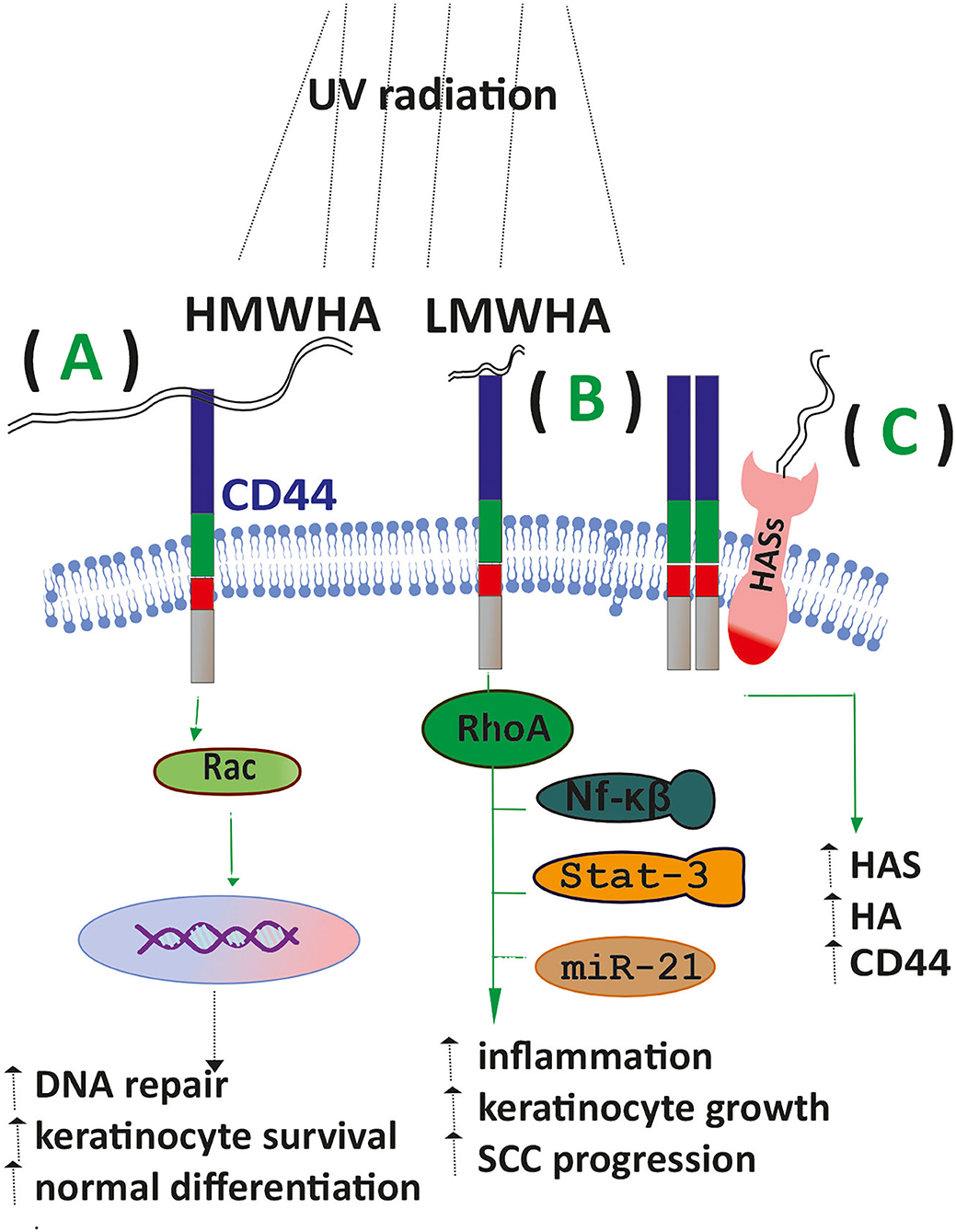
Figure 2. HA signaling affects SCC progression. (A) Upon exposure of keratinocytes to UV radiation, the binding of HMWHA to the CD44 receptor enhances DNA repair, keratinocyte survival, and normal differentiation. (B) LMWHA/CD44 binding in exposed keratinocytes promotes the inflammatory response, keratinocyte proliferation, and SCC progression. (C) Exposed keratinocytes show increased expression of HAS enzymes CD44 and increased HA deposition.
Collagen Matrix Is Altered in NMSC
Collagens (COLs) are a primary group of ECM fiber proteins consisting of twenty-eight different types. COL participation is shown in various types of cancer, including NMSC (43). The skin content of collagen is reduced with age and is also affected by sunlight, especially UV exposure.
Indeed, in actinic keratosis, a loss of COL14 and 15 from the BM suggests that the ECM remodeling is an early sign of cSCC progression. Furthermore, the actinic keratosis stroma is negative for COL14 and 15, whose expression is evident later in the stroma of established cSCC tumors (44). Moreover, COL18 is detected in SCCs from grade one onwards, while normal keratinocytes in premalignant lesions were negative for this COL type (44).
Recessive dystrophic epidermolysis bullosa (RDEB) is a skin fragility disease caused by mutations that affect the function and/or the amount of COL 7, the primary component of anchoring fibrils. Hallmarks of RDEB are unremitting blistering and chronic wounds leading to tissue fibrosis and scarring. Nearly all patients with severe RDEB develop highly metastatic cSCC, the leading cause of death. Accumulating evidence from a murine RDEB model and human RDEB cells demonstrates that the lack of COL 7 also directly alters the wound healing process. Non-healing RDEB wounds are characterized by increased inflammation, high transforming growth factor-beta 1 (TGF-β1) levels and activity, and are heavily populated by fibroblast-derived, myofibroblasts responsible for enhanced fibrogenesis and matrix stiffness. In the next stage, fibroblasts are transformed into cancer-associated fibroblasts (CAF), contributing to cancer invasion and progression (45). Moreover, the in vitro downregulation of COL 7, which is crucial for the attachment of basal keratinocytes to the dermis, has been related to enhanced invasion in SCC (46). COL7 also suppresses TGF-β mediated downregulation of vascular endothelial growth factor (VEGF), responsible for SCC vascularization (47).
Collagen 17 (COL17), a dermo-epidermal junction structural component, is a type II hemidesmosomal transmembrane protein that stabilizes the hemidesmosome complex and basal keratinocyte binding to the BM (48). In addition to basal keratinocytes, the squamous keratinocytes around invasive tumor fronts, the adjacent tumor stroma, endothelium, and histiocytes exhibit upregulated COL7. Furthermore, aberrant shedding of COL7 ectodomain in SCC patients correlates to metastasis (49, 50). Indeed, the released COL17 endodomain enhances proliferation and survival, whereas the shedded ectodomain facilitates the invasiveness of SC cells (51).
An in vitro study with murine COL17a-/-keratinocytes revealed PI3K signaling activation. The upstream activators are the β4 integrin subunit of hemidesmosomal a6b4 integrin and the focal adhesion kinase (FAK), resulting in consequent Rac1 activation. This signaling pathway attenuated the ability of keratinocytes to migrate (52). Indeed, these authors suggest that COL17 facilitates keratinocyte adhesion and directed motility by interfering with the integrin-dependent PI3K activation and stabilizing lamellipodia in invasive cSCC. Moreover, the activity of integrin-β1 and COL17 expression at the intercellular site promotes contact following during the collective invasion of an SC cell population (53).
Reports mention the downregulation of total collagen deposition in NMSC patients (54, 55). Indeed, the total collagen levels are not significantly altered in a precancer state, suggesting that collagen downregulation could be considered a biomarker for cSCC occurrence. A possible explanation is the enhanced release of the collagen-degrading MMPs (55).
Proteoglycans Mediate Keratinocyte-Tumor Progression
PGs are complex molecules whose protein core is glycosylated with one or more GAG chains (56). These versatile molecules regulate both the mechanical properties of tissues and act as key mediators of biological function. PGs are either secreted to the ECM or located to the cell membrane with the exception of serglycin, an intracellular PG (56). Due to their mediation of the cell/matrix interface PGs are involved in the pathogenesis of a multitude of cancer types (57–59).
Syndecans are a family of four transmembrane heparan sulfate PGs (HSPGs) exhibiting related structures. Indeed, all syndecan members consist of a variable N-terminal ectodomain, and highly conserved transmembrane and C-terminal cytoplasmic domains (60). Syndecans have been directly implicated in various tumor development (60, 61).
Syndecan-1 was shown to regulate intercellular and cell/matrix adhesion (60). Early studies on cutaneous biopsies of SCC detected a decrease of syndecan-1 expression with decreased adhesion of SCC cells suggesting that its' expression is inversely correlated to cSCC invasion into the dermis (62). This was supported by following studies showing that in invasive cSCC both syndecan-1 and cadherin E expressions were decreased with the loss of keratinocyte differentiation, especially in the acantholytic areas of tumors where interestingly these molecules were localized to the cytoplasm and not to the cell membrane (63), Indeed, syndecan-1 expression was identified to be discrete between keratoacanthoma and invasive SCC. Thus, syndecan-1 staining was diminished in invasive SCC compared to in situ SCC and keratoacanthoma (63). However, in a separate study, it was determined that syndecan-1 expression was decreased in BCC, cSCC, and metastatic human skin cancers compared to normal human skin (64). Indeed, Stepp et al. indicate that syndecan-1 is a significant factor in the early development and progression of skin cancers, suggesting that the expression of this PG could be utilized as a progression marker for cancerous skin lesions (64).
Interestingly, pioneer studies of the field demonstrated that basement membrane HSPGs and chondroitin sulfate PGs (CSPGs) were significantly decreased in cSCC compared to the normal epidermis (65).
Collectively, these data indicate that PGs, well established to regulate the cell to matrix adhesion as well as intercellular contacts important for epithelial cohesion, are important factors in keratinocyte carcinoma development and invasion to the dermis. Further studies defining their roles could improve the assessment of specific skin lesion prognoses.
Matrix Metalloproteinases (MMPs)-Key Regulators of the Tumor Microenvironment
MMPs are zinc-containing endopeptidases that exhibit a wide range of substrate specificities, meditating, among others, the degradation of the different components of the ECM (66). However, the MMPs also act on membrane receptors, signaling molecules, and ligands modulating their activities and can thus be defined as cell signal regulators (67). Keratinocytes and dermal fibroblasts secrete these enzymes in response to various stimuli, including UV radiation, oxidative stress, and cytokines (68). For example, the exposure of keratinocytes to UV-radiation initiates critical cellular signaling pathways, including the nuclear factor-kappa beta (NF-kB), the mitogen-activated protein kinase (MAPK), the JAK/STAT (signal transduction and activation of transcription), and the nuclear factor erythroid 2-related factor 2 (Nrf2), critical regulators of inflammation and cancer (69). In continuation, exposed keratinocytes secrete active regulators that, in turn, activate the release of MMPs by dermal fibroblasts (70). The content of dermis ECM being remodeled by the MMPs facilitates an intricate balance that maintains the skin's homeostasis or its' response to injury. However, aberrant MMPs expression and activity in cancer tissues promote essential tumor-related functions (71).
Indeed, the invasion of tumor cells, a complex, multifaceted process, is initiated by MMPs degrading the BM and the surrounding ECM (72, 73). It is well established that the gelatinase MMP members, including MMP-2,−7, and−9, participate in BM degradation due to their capability to cleave key BM components such as COL4 (74–76). In addition, cancer-stroma interactions are essential at this point as MMP-2 is secreted chiefly by the surrounding fibroblast-like stromal cells and seldom by keratinocytes and BCC cells (77). Likewise, MMP-9 is secreted mainly by tumor-associated macrophages (TAMs), not BCC tumor cells (78). Interestingly, a higher number of macrophages, able to initiate BCC cells' MMP-9 secretion, utilizing the p38 MAPK/NF-kB/COX-2 cascade, was detected in aggressive forms of BCC compared to lees aggressive phenotypes (79). Furthermore, CAFs secrete MMP-1, MMP-3, MMP-7, MMP-9, and MMP-13, releasing ECM-bound growth factors such as VEGF supporting tumor angiogenesis (80).
Notably, MMP-1, MMP-7, MMP-9, MMP-13, and MMP-14 overexpression correlated to intensive remodeling of the BCC matrix was identified (81). Interestingly, a correlation between the MMP-2 and MMP-9 expression between the stroma and tumor suggests that tumor cells affect stroma MMPs deposition in BCC (82). However, in this study, no correlation between the MMP expression and the presence of the peritumoral cleft was shown (82). In chronic wounds, MMP-7, MMP-12, and MMP-13, but not MMP-1, MMP-3, MMP-8, MMP-9, and MMP-10 expressions, were identified as diagnostic clues for discerning SCCs from nonmalignant wounds (83).
A separate study suggested that MMP-1 has the highest COL1-degrading activity generating high molecular weight collagen fragments. There is an accumulation of these fragments as MMP-2 and MMP-9 have lower activities. The deposition of high molecular weight collagen fragments attenuates stromal fibroblasts' functions correlated with BCC progression (84). MMP-13 and MMP-1 also played a significant role in tumor progression of eyelid BCC in a clinical study, as immunoreaction positivity was identified in most patients (85). Likewise, MMP-11 was overexpressed in the biopsies of both cSCC and BCC patients compared to normal skin (86).
Interestingly the complement system seems to be involved in the MMP-dependent progression of non-melanoma skin cancers. Thus, the knock-out of the serine proteinase C1r, a complement system constituent, in cSCC led to these cells attenuating proliferation, migration, and invasion through collagen type I. Moreover, the knock-out of C1r downregulated the expression of MMP-1, MMP-13, MMP-10, and MMP-12 by cSCC cells in culture. In addition, knock-out of C1r decreased the expression of MMP-13, inhibited the invasion of cSCC, and decreased collagen cSCC xenografts (87). Therefore, the authors suggest that the complement facilitates SCC cells' invasion by enhancing MMPs' expression.
Intriguingly, in NMSC patients, antibodies against MMP-7 were detected. As these autoantibodies are being utilized as an early marker for other malignancies, it was suggested that they could also be a biomarker for NMSCs. Notably, the expression of MMP7 autoantibodies was not followed by changes in the MMP-7 mRNA levels in both BCC and cSCC patients (88). On the other hand, at the protein level, the expression of MMP-7 and CD44 was found to be inversely correlated in cSCC and BCC sections. Indeed, in well-differentiated SCC, the MMP-7 staining was weak, while CD44 expression was high. In poorly differentiated SCC, increased MMP-7 staining was identified while the expression of CD44 was weak (89).
MMPs expression is correlated to the progression and recurrence of these tumor types. cSCC patients' tumor tissues exhibited a notably higher level of MMP-13 compared to control tissues. In addition, these patients showed significantly increased MMP-13 levels compared to healthy controls. Moreover, patients with invasive SCC had increased serum MMP-13 levels compared to in situ cSCC patients (90). Therefore Wang et al. suggest that serum MMP-13 is a relevant diagnostic marker for cSCC (90). In cSCC and BCC biopsies, MMP-2 expression was correlated to the depth of invasion, whereas the expression of MMP-2, MMP-9, TIMP-1 with inflammation and microvessel density (91). Likewise, Gozdzialska et al. show that MMP-2 protein expression in the tumor stroma was higher in high-risk BCCs than low-risk BCCs, whereas MMP-2 deposition was enhanced in both the tumor tissue and stroma in SCC when compared to BCC (92). These data point to a crucial role of MMP-2 in skin cancer invasion (92).
MMPs Involvement in Immune-Related Processes in NMSCs
As already highlighted, the tumor microenvironment plays an essential role in the interplay between tumors and the elements of the immune system, mainly the immune-infiltrating cells and their immune molecules (93, 94). In recent years, as the gathered information regarding anti-tumoral immunity is piling up, the importance of immune-infiltrating cells in tumors (TIL), including skin cancers (95), is steadily increasing. A recent study that has analyzed data from various databases (e.g., The Cancer Genome Atlas, ONCOMINE) showed that immune cells' infiltration, MMPs expression, and tumor prognosis are associated in many types of cancers (96).
Immune Cells Infiltration in NMSCs
Although new investigation methods have recently entered the clinical management of NMSC patients (97), new insights into the local and circulatory immunity are needed (98). Several immune cell families infiltrate the tumor, out of which tumor-associated macrophages (TAM) and lymphocytes comprise the majority of immune cells. Notably, the relation between MMP14 expression and tumor-infiltrating lymphocytes (TILs) was investigated in several cancers. The findings indicate that MMPs, more specifically MMP14, can be a good prognosis, diagnosis, and treatment biomarker in various solid tumors (99).
It was shown, during SCC progression, that TAMs secrete several pro-carcinogenesis molecules such as endothelial vascular growth factor (VEGF)-C and MMPs, with a predominance of MMP9, MMP10, and MMP11. This array of TAM-related molecules encourages other inflammatory cells to enter the tumor and release cytokines that further promote tumor growth and angiogenesis (100, 101). Furthermore, a rich infiltrate with TAMs expressing predominantly M2 type induces lymph node metastasis in SCC (102); concomitantly high MMP expression is associated with advanced stages of SCC (103).
A recent in vitro cellular model study tested antibodies specific to MMP-1 conjugated to iron-gold bimetallic nanoparticles. In a cell line of human tongue squamous cell carcinoma (HSC-3), these antibodies triggered a high percentage of tumor cells death (104). Indeed, tumor cells attenuate the immune anti-tumoral action by MMP-2, MMP-9, MMP-13, and MMP-14 secretion, leading to T-cell activity hindrance (105).
In BCC, it was demonstrated that the tumor harbors immature dendritic cells with low potency in antigen presentation and an array of Th2-type immunosuppressive cytokines (77, 106). Earlier studies have shown that MMPs have various roles in the epidermal–mesenchymal transition processes and the immune response during the progression of BCC. Indeed, cocultivation of fibroblasts and melanoma cells has resulted in MMP-2 high overexpression, whereas; keratinocytes and BCC cells cocultivation led to a moderate upregulation of MMP-2 expression. However, in the absence of cellular contact, when only molecular communication was established between fibroblasts with BCC cells, a decrease in fibroblasts' MMP-2 expression was observed (78). These data suggest that cell-cell signaling is obligatory in regulating MMP-2 expression in this system.
In immunocompetent subjects, MMP-1, MMP-9, and TIMP-1 expression associated with stromal macrophages in BCC (107) and TILs comprise CD4 + T-helper cells and FoxP3 + T-regulatory (Tregs); these immune cell populations can also be found in the peritumoral inflammatory infiltrates. The proportion of these cellular immune populations is associated with the recurrence risk in this skin cancer. A high proportion of cytotoxic CD8+ T cells in TILs characterizes an antitumor response, while a lower ratio is associated with an increased risk of recurrence (108). An earlier study performed in BCC animal models has shown that tumor growth is promoted when TILs have low cutaneous macrophages and dendritic cells proportion (109). Additionally, an increased number of immunosuppressive Tregs is associated with an unfavorable outcome (110, 111), but it is still unknown if TILs in BCC come from skin resident cells or circulating lymphocytes (110).
MMPs are involved in the immune response flow in this immune tissue portrayal. Thus, MMPs can cleave an important T cellular receptor, such as IL-2Rα, and hence impede this effector cell proliferation. Moreover, MMP-11 can participate in the increased survival of BCC tumor cells even when NK cells release cytotoxic molecules (111). Cancer-associated fibroblasts (CAFs) are another essential cell population found in tumors. In BCC's stroma and the peritumoral area, CAFs can secrete an array of MMPs (e.g., MMP-1, MMP-2, MMP-3, MMP-9, MMP-11, MMP-13, MMP-14, MMP-19), all of them promoting ECM remodeling (105, 111) and favoring tumor escape from the anti-tumoral action of the immune cells (109).
Various immune-related surface molecules are involved in delivering an efficient anti-tumoral action. Programmed cell death-1 protein (PD-1) is a vital surface molecule from the CD28 superfamily highly involved in the anti-tumoral T cell activity (112). In BCCs, the expression of PD-1 and PDL-1 was found on tumor cells in 22% of cases and over 80% of TILs and TAMs (113). A recent study on esophageal SCC has shown that upregulation of several key molecules (CTLA-4, PD-1, PD-L1, TIM-3, LAG-3) are associated with MMP-13 expression and correlated to tumor progression and invasion (114).
Similarly, to BCC, in cSCC' TILs display specific patterns owing to the immune-suppressive tumor environment. This tumor environment induces decreased proliferation of TILs, impairs cytokine secretion, and hinders their capability to effectively kill tumor cells (115). cSCC has a predominantly Th2 type immune response, with low interferon (IFN) gamma (106) so that M2 type TAMs are activated, attracting new Tregs cellular populations and further sustaining the immune-suppressive milieu (116), processes correlated with an unfavorable prognosis (117). In laryngeal SCC, it was shown that tumor cells interact with ECM and that MMPs destroy the ECM scaffold to promote tumoral invasion (118).
MMP-9 is secreted by leukocytes and is associated with various tumor cells' aberrant behavior (119). In laryngeal SCC, MMP-9 expression is correlated with recurrence prognosis and poor outcome (120). A recent study showed that the expressions of GRK2, TRAF2, and MMP-9 in laryngeal SCC clinical samples were found significantly increased compared to normal tissues. These results proved that two immune-related signaling pathways cross-talk, TNF-α and PGE2. PGE2 increased the pathway TNF-α-TRAF2-MMP-9 signaling. Therefore TRAF2 and GRK2 co-expression induce proliferation, migration, and invasion of tumor cells, further promoting MMP-9 expression (121).
Circulatory MMPs and Immune-Related Markers in NMSC
Tumor-associated antigens (TAAs), discovered more than 20 years ago, are over-expressed in neoplastic transformed tissue and circulate in the peripheral blood (122). The immune system recognizes TAAs, as transformed antigens and synthesizes/secretes specific autoantibodies (123).
In the last ten years, serum autoantibodies against TAAs were evaluated in various cancers, but the first study focusing on NMSC's evaluation of TAA autoantibodies was published in 2021. In this study, autoantibodies against p53, MMP-7, and Hsp70 were significantly higher in NMSCs patients than normal subjects. Moreover, this panel of TAA autoantibodies can establish early diagnosis of NMSC (88).
A similar study has shown that cSCC patients display high serum MMP-13. After surgery, the MMP-13 level decreases and, when raising again, can predict an invasive SCC and lymph node metastasis; thus MMP-13 can represent a reliable biomarker for invasiveness and tumor progression monitoring (90).
Several years ago, in SCC, circulating immune cell populations were reported as altered compared to normal subjects; the proportion of circulatory sub-populations and their activation pathways deregulations were identified (83). Recently, we have shown that there are critical circulatory disturbances among immune cells in oral SCC. Hence, pre-surgery patients display significantly higher suppressive T lymphocytes and increased circulating NK (CD16+) cells than post-surgery levels. The results aid the immuno-inflammatory portrait of SCC (124).
The novel, recent players that provide information regarding the inflammatory status of NMSCs, are the non-coding RNAs (nc-RNAs). These molecules have epigenetic regulatory action and are involved in skin cancers (125) as well as associated with the inflammatory processes related to skin's UV irradiation. Hence upon UVB radiation of the skin, it was shown that nc886 expression decreases, which drives uncontrolled PKR activity and increases in the MMP-9 expression and other inflammatory mediators production (e.g., type IV collagenase, COX-2). This recent finding indicates that ncRNAs could be a new therapeutic target in skin inflammation and carcinogenesis (126). The MMP's involvement in cSCCs progression is depicted in Figure 3.
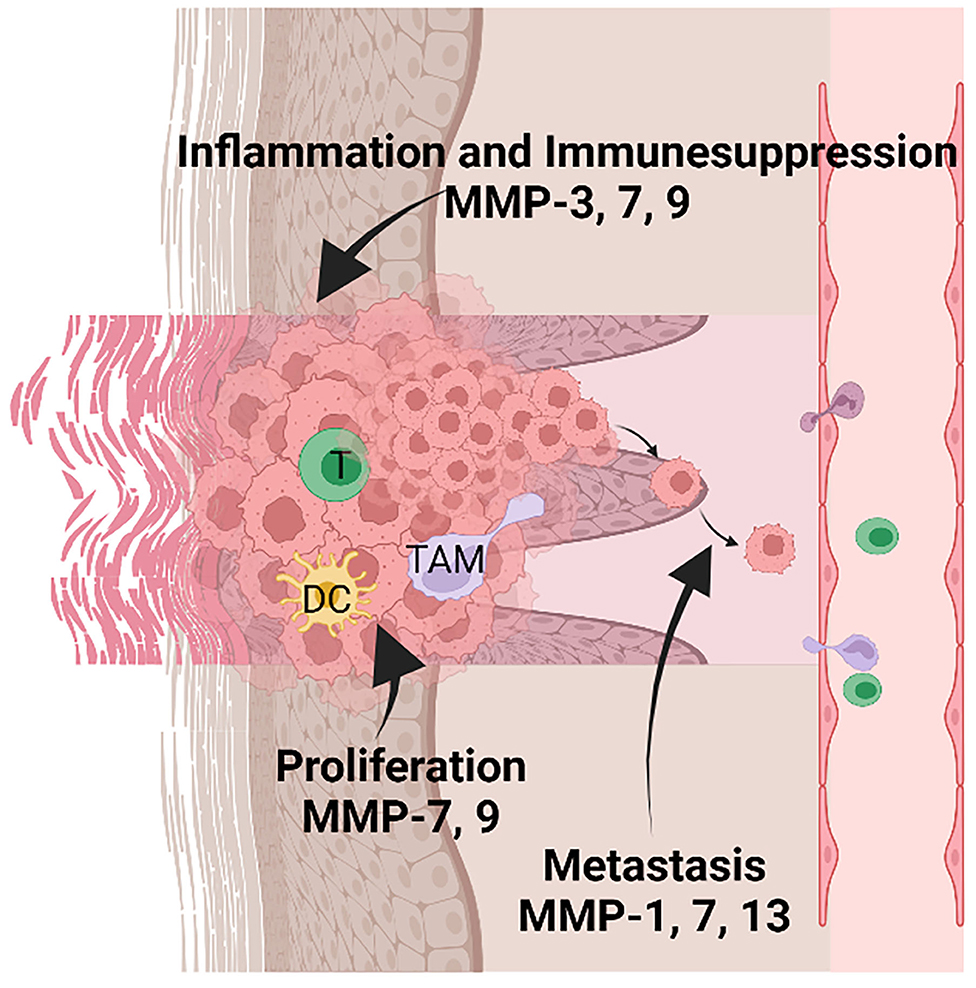
Figure 3. MMPs' involvement in inflammation, tumor proliferation, and metastasis in keratinocyte-derived tumors. Tumor-infiltrating immune cells are comprised of several populations such as T lymphocytes (T), dendritic cells (DC), and tumor-associated macrophages (TAM). MMP-3, 7, and 9 are mainly involved in sustaining the inflammatory microenvironment and suppressing the anti-tumoral action of the immune cells. In addition, MMP-7 and 9 sustain the proliferation of tumor cells; MMP-1, 7, 13 favor the metastatic processes by activating the invasion of tumor cells in other tissues. Created with BioRender.”
Therapies Based on MMP Targeting
Immune checkpoint blockade therapy is already applied to manage several skin cancers (127). As molecules like CTLA4, PD-1, and PD-L1 are already therapy targets (128), improvements are sought to treat non-responsive patients (129). Hence, as previously shown, MMPs are associated with tumor microenvironment and infiltrating immune cells. Notably, recent studies have shown that inhibition of MMP2/9 can reduce tumor burden and improve survival, promoting anti-tumoral immunity. Upon inhibiting these MMPs, it was demonstrated that mRNA and protein levels of PD-L1 expression were reduced (130).
Epoxy-taglines are a novel class of compounds with anti-cancer properties to be used in skin tumors. The compound targets keratinocyte-derived tumors and promotes re-epithelialization after tumor tissue destruction. A recent in vitro study has shown that epoxy-tiglianes modulate MMP and cytokine gene expression along with other molecular processes that favor keratinocyte healing responses (131).
Conclusion: Challenges and Developments
The changes in the structure/function of the ECM in NMSC are well documented. The ECM alterations affect both multilayer epithelium mechanics and the mechanical remodeling of the stroma. In addition, the changes in ECM components either by generating matrikines or releasing growth factors, cytokines, and other signaling mediators essentially affect all steps in these tumors' development and dissemination. Notably, increasing evidence demonstrates that matrix-associated molecules can be utilized as diagnosis/prognosis markers and therapy venues. Thus, CD44 and CD29 have been determined as cancer-stem cell markers (132) and a high CD44 phenotype in SCC is suggested to identify candidates for implementing targeted therapy (34). Likewise, MMP-9 is indicated as a marker of aggressive BCC forms (79). Matrix molecules such as MMP-13 (90) and MMP-7 (88) are identified as TAAs circulating in the peripheral blood. Recently, nc-RNAs have been determined as positive regulators of inflammatory mediators including MMP-9 (126) suggesting that these molecules could be a new therapeutic target in skin inflammation and carcinogenesis.
However, further studies need to be performed to correlate discrete ECM molecules' characteristics that can predict facets of tumor behavior regarding their aggressiveness and susceptibility to discrete therapeutic strategies. Moreover, systemic treatment may be indicated in the case of locally advanced or metastatic disease. Indeed, valid systemic therapy for keratinocyte carcinomas remains an unmet clinical need. Therefore, better defining the molecular mechanisms supporting tumor growth and development is crucial for the needed advances.
Author Contributions
R-MK and DN conceived, designed the structure, and contributed to the writing of the paper. MN, CC, AM, and MS contributed to the writing of the paper and the preparation of figures. GT, AM, and DN edited the paper. All authors contributed to the article and approved the submitted version.
Funding
The APC was funded by grant NASR, [PN 19.29.01.01] (50%) and by the Romanian Company Dialab Solutions (50%). Dialab Solutions was not involved in the study design, collection, analysis, interpretation of data, the writing of this article or the decision to submit it for publication. DN was partially funded by the Research Committee of the University of Crete (ELKE), grant number (KA:10393). Some research noted in text has been partly supported by the above grants.
Conflict of Interest
The authors declare that the research was conducted in the absence of any commercial or financial relationships that could be construed as a potential conflict of interest.
Publisher's Note
All claims expressed in this article are solely those of the authors and do not necessarily represent those of their affiliated organizations, or those of the publisher, the editors and the reviewers. Any product that may be evaluated in this article, or claim that may be made by its manufacturer, is not guaranteed or endorsed by the publisher.
References
1. Liu-Smith F, Jia J, Zheng Y. UV-induced molecular signaling differences in melanoma and non-melanoma skin cancer. Adv Exp Med Biol. (2017) 996:27–40. doi: 10.1007/978-3-319-56017-5_3
2. Nehal KS, Bichakjian CK. Update on keratinocyte carcinomas. N Engl J Med. (2018) 379:363–74. doi: 10.1056/NEJMra1708701
3. Jones PH, Harper S, Watt FM. Stem cell patterning and fate in human epidermis. Cell. (1995) 80:83–93. doi: 10.1016/0092-8674(95)90453-0
4. Venables ZC, Autier P, Nijsten T, Wong KF, Langan SM, Rous B, et al. Nationwide incidence of metastatic cutaneous squamous cell carcinoma in England. JAMA Dermatol. (2019) 155:298–306. doi: 10.1001/jamadermatol.2018.4219
5. Yao CD, Haensel D, Gaddam S, Patel T, Atwood SX, Sarin KY, et al. AP-1 and TGFss cooperativity drives non-canonical Hedgehog signaling in resistant basal cell carcinoma. Nat Commun. (2020) 11:5079. doi: 10.1038/s41467-020-18762-5
6. Durinck S, Ho C, Wang NJ, Liao W, Jakkula LR, Collisson EA, et al. Temporal dissection of tumorigenesis in primary cancers. Cancer Discov. (2011) 1:137–43. doi: 10.1158/2159-8290.CD-11-0028
7. Blohm-Mangone K, Burkett NB, Tahsin S, Myrdal PB, Aodah A, Ho B, et al. Pharmacological TLR4 antagonism using topical resatorvid blocks solar UV-induced skin tumorigenesis in SKH-1 mice. Cancer Prev Res. (2018) 11:265–78. doi: 10.1158/1940-6207.CAPR-17-0349
8. Ke Y, Wang X-J. TGFβ signaling in photoaging and UV-induced skin cancer. J Invest Dermatol. (2021)0.141:1104–10. doi: 10.1016/j.jid.2020.11.007
9. Guerra L, Odorisio T, Zambruno G, Castiglia D. Stromal microenvironment in type VII collagen-deficient skin: the ground for squamous cell carcinoma development. Matrix Biol. (2017) 63:1–10. doi: 10.1016/j.matbio.2017.01.002
10. Kavasi RM, Berdiaki A, Spyridaki I, Corsini E, Tsatsakis A, Tzanakakis G, et al. HA metabolism in skin homeostasis and inflammatory disease. Food Chem Toxicol. (2017) 101:128–38. doi: 10.1016/j.fct.2017.01.012
11. Jarvelainen H, Sainio A, Koulu M, Wight TN, Penttinen R. Extracellular matrix molecules: potential targets in pharmacotherapy. Pharmacol Rev. (2009) 61:198–223. doi: 10.1124/pr.109.001289
12. Chen GY, Nunez G. Sterile inflammation: sensing and reacting to damage. Nat Rev Immunol. (2010) 10:826–37. doi: 10.1038/nri2873
13. Kouvidi K, Nikitovic D, Berdiaki A, Tzanakakis GN. Hyaluronan/RHAMM interactions in mesenchymal tumor pathogenesis: role of growth factors. Adv Cancer Res. (2014) 123:319–49. doi: 10.1016/B978-0-12-800092-2.00012-5
14. Kavasi RM, Berdiaki A, Spyridaki I, Papoutsidakis A, Corsini E, Tsatsakis A, et al. Contact allergen (PPD and DNCB)-induced keratinocyte sensitization is partly mediated through a low molecular weight hyaluronan (LMWHA)/TLR4/NF-kappaB signaling axis. Toxicol Appl Pharmacol. (2019) 15:114632. doi: 10.1016/j.taap.2019.114632
15. Piipponen M, Heino J, Kähäri V-M, Nissinen L. Long non-coding RNA PICSAR decreases adhesion and promotes migration of squamous carcinoma cells by downregulating α2β1 and α5β1 integrin expression. Biol Open. (2018) 7:bio037044. doi: 10.1242/bio.037044
16. Petreaca M, Martins-Green M. Cell-ECM Interactions in Repair and regeneration. Principles of Regenerative Medicine. Amsterdam: Elsevier (2011). p.19–65.
17. Tanner K. Regulation of the basement membrane by epithelia generated forces. Phys Biol. (2012) 9:065003. doi: 10.1088/1478-3975/9/6/065003
18. Atwood SX, Chang ALS, Oro AE. Hedgehog pathway inhibition and the race against tumor evolution. J cell Biol. (2012) 15:193–7. doi: 10.1083/jcb.201207140
19. Li S, Balmain A, Counter CM. A model for RAS mutation patterns in cancers: finding the sweet spot. Nat Rev Cancer. (2018) 18:767–77. doi: 10.1038/s41568-018-0076-6
20. Fiore VF, Krajnc M, Quiroz FG, Levorse J, Pasolli HA, Shvartsman SY, et al. Mechanics of a multilayer epithelium instruct tumour architecture and function. Nature. (2020) 585:433–9. doi: 10.1038/s41586-020-2695-9
21. Hirakawa Y, Futaki S, Tanizaki H, Furukawa F, Maemura K, Kondo Y, et al. Enhanced expression of nidogen 1 around the nest of basal cell carcinoma compared with that around squamous cell carcinoma. Med Mol Morphol. (2019) 52:99–105. doi: 10.1007/s00795-018-0207-x
22. Silver FH, Deshmukh T, Benedetto D, Kelkar N. Mechano-vibrational spectroscopy of skin: are changes in collagen and vascular tissue components early signs of basal cell carcinoma formation? Skin Res Technol. (2021) 27:227–33. doi: 10.1111/srt.12921
23. Harn HIC, Wang YK, Hsu CK, Ho YT, Huang YW, Chiu WT, et al. Mechanical coupling of cytoskeletal elasticity and force generation is crucial for understanding the migrating nature of keloid fibroblasts. Exp Dermatol. (2015) 24:579–84. doi: 10.1111/exd.12731
24. Harn HIC, Ogawa R, Hsu CK, Hughes MW, Tang MJ, Chuong CM. The tension biology of wound healing. Exp Dermatol. (2019) 28:464–71. doi: 10.1111/exd.13460
25. Wipff P-J, Rifkin DB, Meister J-J, Hinz B. Myofibroblast contraction activates latent TGF-β1 from the extracellular matrix. J Cell Biol. (2007) 179:1311–23. doi: 10.1083/jcb.200704042
26. Weigel PH, Hascall VC, Tammi M. Hyaluronan synthases. J BiolChem. (1997) 272:13997–4000. doi: 10.1074/jbc.272.22.13997
27. Stern R, Jedrzejas MJ. Hyaluronidases: their genomics, structures, and mechanisms of action. Chem Rev. (2006) 106:818–39. doi: 10.1021/cr050247k
28. Naor D, Nedvetzki S, Walmsley M, Yayon A, Turley EA, Golan I, et al. CD44 involvement in autoimmune inflammations: the lesson to be learned from CD44-targeting by antibody or from knock-out mice. Ann N Y Acad Sci. (2007) 1110:233–47. doi: 10.1196/annals.1423.025
29. Hauser-Kawaguchi A, Tolg C, Peart T, Milne M, Turley EA, Luyt LG, et al. truncated RHAMM protein for discovering novel therapeutic peptides. Bioorg Med Chem. (2018) 26:5194–203. doi: 10.1016/j.bmc.2018.09.018
30. Iotzova-Weiss G, Freiberger SN, Johansen P, Kamarachev J, Guenova E, Dziunycz PJ, et al. TLR4 as a negative regulator of keratinocyte proliferation. PLoS ONE. (2017) 12:e0185668. doi: 10.1371/journal.pone.0185668
31. Bourguignon LY, Bikle D. Selective hyaluronan–CD44 signaling promotes miRNA-21 expression and interacts with vitamin D function during cutaneous squamous cell carcinomas progression following UV irradiation. Front Immunol. (2015) 6:224. doi: 10.3389/fimmu.2015.00224
32. Siiskonen H, Törrönen K, Kumlin T, Rilla K, Tammi MI, Tammi RH. Chronic UVR causes increased immunostaining of CD44 and accumulation of hyaluronan in mouse epidermis. J HistochemCytochem. (2011) 59:908–17. doi: 10.1369/0022155411417874
33. Kakizaki I, Itano N, Kimata K, Hanada K, Kon A, Yamaguchi M, et al. Up-regulation of hyaluronan synthase genes in cultured human epidermal keratinocytes by UVB irradiation. Arch Biochem Biophys. (2008) 471:85–93. doi: 10.1016/j.abb.2007.12.004
34. Erfani E, Roudi R, Rakhshan A, Sabet MN, Shariftabrizi A, Madjd Z. Comparative expression analysis of putative cancer stem cell markers CD44 and ALDH1A1 in various skin cancer subtypes. Int J Biol Markers. (2016) 31:e53–61. doi: 10.5301/jbm.5000165
35. Montero-Montero L, Renart J, Ramírez A, Ramos C, Shamhood M, Jarcovsky R, et al. Interplay between Podoplanin, CD44s and CD44v in squamous carcinoma cells. Cells. (2020) 9:2200. doi: 10.3390/cells9102200
36. Baum HP, Schmid T, Schock G, Reichrath J. Expression of CD44 isoforms in basal cell carcinomas. Br J Dermatol. (1996) 134:465–8. doi: 10.1111/j.1365-2133.1996.tb16231.x
37. Mochel MC, Liaquat S, Moore JB, Hoang MP. Metastasizing basal cell carcinoma: a clinicopathologic and immunohistochemical study of 22 cases. J Cutan Pathol. (2021) 48:374–83. doi: 10.1111/cup.13888
38. Milosevic M, Lazarevic M, Toljic B, Simonovic J, Trisic D, Nikolic N, et al. Characterization of stem-like cancer cells in basal cell carcinoma and its surgical margins. Exp Dermatol. (2018) 27:1160–5. doi: 10.1111/exd.13755
39. Nikitovic D, Kouvidi K, Kavasi RM, Berdiaki A, Tzanakakis GN. Hyaluronan/Hyaladherins - a promising axis for targeted drug delivery in cancer. Curr Drug Deliv. (2016) 13:500–11. doi: 10.2174/1567201813666151109103013
40. Tolg C, Liu M, Cousteils K, Telmer P, Alam K, Ma J, et al. Cell-specific expression of the transcriptional regulator RHAMM provides a timing mechanism that controls appropriate wound re-epithelialization. J Biol Chem. (2020) 295:5427–48. doi: 10.1074/jbc.RA119.010002
41. Janda J, Burkett NB, Blohm-Mangone K, Huang V, Curiel-Lewandrowski C, Alberts DS, et al. Resatorvid-based pharmacological antagonism of cutaneous TLR4 blocks UV-induced NF-κB and AP-1 signaling in keratinocytes and mouse skin. Photochem Photobiol. (2016) 92:816–25. doi: 10.1111/php.12659
42. Mittal D, Saccheri F, Vénéreau E, Pusterla T, Bianchi ME, Rescigno M. TLR4-mediated skin carcinogenesis is dependent on immune and radioresistant cells. EMBO J. (2010) 29:2242–52. doi: 10.1038/emboj.2010.94
43. Tzanakakis G, Kavasi RM, Voudouri K, Berdiaki A, Spyridaki I, Tsatsakis A, et al. Role of the extracellular matrix in cancer-associated epithelial to mesenchymal transition phenomenon. Dev Dyn. (2018) 247:368–81. doi: 10.1002/dvdy.24557
44. Karppinen SM, Honkanen HK, Heljasvaara R, Riihilä P, Autio-Harmainen H, Sormunen R, et al. Collagens XV and XVIII show different expression and localisation in cutaneous squamous cell carcinoma: type XV appears in tumor stroma, while XVIII becomes upregulated in tumor cells and lost from microvessels. Exp Dermatol. (2016) 25:348–54. doi: 10.1111/exd.12913
45. Gascard P, Tlsty TD. Carcinoma-associated fibroblasts: orchestrating the composition of malignancy. Genes Dev. (2016) 30:1002–19. doi: 10.1101/gad.279737.116
46. Martins VL, Vyas JJ, Chen M, Purdie K, Mein CA, South AP, et al. Increased invasive behaviour in cutaneous squamous cell carcinoma with loss of basement-membrane type VII collagen. J Cell Sci. (2009) 122:1788–99. doi: 10.1242/jcs.042895
47. Martins VL, Caley MP, Moore K, Szentpetery Z, Marsh ST, Murrell DF, et al. Suppression of TGFbeta and angiogenesis by type VII collagen in cutaneous SCC. J Natl Cancer Inst. (2016) 108:djv293. doi: 10.1093/jnci/djv293
48. Powell A, Sakuma-Oyama Y, Oyama N, Black M. Collagen XVII/BP180: a collagenous transmembrane protein and component of the dermoepidermal anchoring complex. Clin Exp Dermatol. (2005) 30:682–7. doi: 10.1111/j.1365-2230.2005.01937.x
49. Stelkovics E, Korom I, Marczinovits I, Molnar J, Rasky K, Raso E, et al. Collagen XVII/BP180 protein expression in squamous cell carcinoma of the skin detected with novel monoclonal antibodies in archived tissues using tissue microarrays and digital microscopy. Appl Immunohistochem Mol Morphol. (2008) 16:433–41. doi: 10.1097/PAI.0b013e318162f8aa
50. Jones VA, Patel PM, Gibson FT, Cordova A, Amber KT. The role of collagen XVII in cancer: squamous cell carcinoma and beyond. Front Oncol. (2020) 10:352. doi: 10.3389/fonc.2020.00352
51. Galiger C, Löffek S, Stemmler MP, Kroeger JK, Mittapalli VR, Fauth L, et al. Targeting of cell surface proteolysis of collagen XVII impedes squamous cell carcinoma progression. Mol Ther. (2018) 26:17–30. doi: 10.1016/j.ymthe.2017.09.022
52. Loffek S, Hurskainen T, Jackow J, Sigloch FC, Schilling O, Tasanen K, et al. Transmembrane collagen XVII modulates integrin dependent keratinocyte migration via PI3K/Rac1 signaling. PLoS ONE. (2014) 9:e87263. doi: 10.1371/journal.pone.0087263
53. Kumagai Y, Nio-Kobayashi J, Ishida-Ishihara S, Tachibana H, Omori R, Enomoto A, et al. The intercellular expression of type-XVII collagen, laminin-332, and integrin-β1 promote contact following during the collective invasion of a cancer cell population. Biochem Biophys Res Commun. (2019) 514:1115–21. doi: 10.1016/j.bbrc.2019.05.058
54. Fox SA, Shanblatt AA, Beckman H, Strasswimmer J, Terentis AC. Raman spectroscopy differentiates squamous cell carcinoma (SCC) from normal skin following treatment with a high-powered CO2 laser. Lasers Surg Med. (2014) 46:757–72. doi: 10.1002/lsm.22288
55. Feng X, Moy AJ, Nguyen HTM, Zhang Y, Zhang J, Fox MC, et al. Raman biophysical markers in skin cancer diagnosis. J Biomed Opt. (2018) 23:1–10. doi: 10.1117/1.JBO.23.5.057002
56. Iozzo RV, Schaefer L. Proteoglycan form and function: A comprehensive nomenclature of proteoglycans. Matrix Biol. (2015) 42:11–55. doi: 10.1016/j.matbio.2015.02.003
57. Tzanakakis G, Neagu M, Tsatsakis A, Nikitovic D. Proteoglycans and Immunobiology of Cancer-Therapeutic Implications. Front Immunol. (2019) 10:875. doi: 10.3389/fimmu.2019.00875
58. Nikitovic D, Berdiaki A, Spyridaki I, Krasanakis T, Tsatsakis A, Tzanakakis GN. Proteoglycans-Biomarkers and Targets in Cancer Therapy. Front Endocrinol. (2018) 9:69. doi: 10.3389/fendo.2018.00069
59. Giatagana EM, Berdiaki A, Tsatsakis A, Tzanakakis GN, Nikitovic D. Lumican in carcinogenesis-revisited. Biomolecules. (2021) 11:1319. doi: 10.3390/biom11091319
60. Afratis NA, Nikitovic D, Multhaupt HA, Theocharis AD, Couchman JR, Karamanos NK. Syndecans - key regulators of cell signaling and biological functions. FEBS J. (2017) 284:27–41. doi: 10.1111/febs.13940
61. Mytilinaiou M, Bano A, Nikitovic D, Berdiaki A, Voudouri K, Kalogeraki A, et al. Syndecan-2 is a key regulator of transforming growth factor beta 2/Smad2-mediated adhesion in fibrosarcoma cells. IUBMB Life. (2013) 65:134–43. doi: 10.1002/iub.1112
62. Bayer-Garner IB, Sanderson RD, Smoller BR. Syndecan-1 expression is diminished in acantholytic cutaneous squamous cell carcinoma. J Cutan Pathol. (1999) 26:386–90. doi: 10.1111/j.1600-0560.1999.tb01862.x
63. Bayer-Garner IB, Smoller BR. The expression of syndecan-1 is preferentially reduced compared with that of E-cadherin in acantholytic squamous cell carcinoma. J Cutan Pathol. (2001) 28:83–9. doi: 10.1034/j.1600-0560.2001.280204.x
64. Stepp MA, Pal-Ghosh S, Tadvalkar G, Rajjoub L, Jurjus RA, Gerdes M, et al. Loss of syndecan-1 is associated with malignant conversion in skin carcinogenesis. Mol Carcinog. (2010) 49:363–73. doi: 10.1002/mc.20609
65. Oguro K, Kazama T, Isemura M, Nakamura T, Akai S, Sato Y. Immunohistochemical alterations in basement membrane components of squamous cell carcinoma. J Invest Dermatol. (1991) 96:250–4. doi: 10.1111/1523-1747.ep12462197
66. Kessenbrock K, Wang C-Y, Werb Z. Matrix metalloproteinases in stem cell regulation and cancer. Matrix Biol. (2015) 44:184–90. doi: 10.1016/j.matbio.2015.01.022
67. Laronha H, Caldeira J. Structure and function of human matrix metalloproteinases. Cells. (2020) 9:1076. doi: 10.3390/cells9051076
68. Pittayapruek P, Meephansan J, Prapapan O, Komine M, Ohtsuki M. Role of matrix metalloproteinases in photoaging and photocarcinogenesis. Int J Mol Sci. (2016) 17:868. doi: 10.3390/ijms17060868
69. Bosch R, Philips N, Suárez-Pérez JA, Juarranz A, Devmurari A, Chalensouk-Khaosaat J, et al. Mechanisms of photoaging and cutaneous photocarcinogenesis, and photoprotective strategies with phytochemicals. Antioxidants. (2015) 4:248–68. doi: 10.3390/antiox4020248
70. Wang X, Bi Z, Chu W, Wan Y. IL-1 receptor antagonist attenuates MAP kinase/AP-1 activation and MMP1 expression in UVA-irradiated human fibroblasts induced by culture medium from UVB-irradiated human skin keratinocytes. Int J Mol Med. (2005) 16:1117–24. doi: 10.3892/ijmm.16.6.1117
71. Airola K, Johansson N, Kariniemi A-L, Kähäri V-M, Saarialho-Kere UK. Human collagenase-3 is expressed in malignant squamous epithelium of the skin. J Invest Dermatol. (1997) 109:225–31. doi: 10.1111/1523-1747.ep12319441
72. Chu CY, Cha ST, Chang CC, Hsiao CH, Tan CT, Lu YC, et al. Involvement of matrix metalloproteinase-13 in stromal-cell-derived factor 1 alpha-directed invasion of human basal cell carcinoma cells. Oncogene. (2007) 26:2491–501. doi: 10.1038/sj.onc.1210040
73. Nan H, Niu T, Hunter DJ, Han J. Missense polymorphisms in matrix metalloproteinase genes and skin cancer risk. Cancer Epidemiol Biomarkers Prev. (2008) 17:3551–7. doi: 10.1158/1055-9965.EPI-08-0606
74. de Oliveira Poswar F, de Carvalho Fraga CA, Gomes ES, Farias LC, Souza LW, Santos SH, et al. de-Paula AM, Guimarães AL. Protein expression of MMP-2 and MT1-MMP in actinic keratosis, squamous cell carcinoma of the skin, and basal cell carcinoma. Int J Surg Pathol. (2015) 23:20–5. doi: 10.1177/1066896914540998
75. Chuang HC, Su CY, Huang HY, Huang CC, Chien CY, Du YY, et al. Active matrix metalloproteinase-7 is associated with invasion in buccal squamous cell carcinoma. Mod Pathol. (2008) 21:1444–50. doi: 10.1038/modpathol.2008.99
76. Poswar FO, Fraga CA, Farias LC, Feltenberger JD, Cruz VP, Santos SH, et al. Immunohistochemical analysis of TIMP-3 and MMP-9 in actinic keratosis, squamous cell carcinoma of the skin, and basal cell carcinoma. Pathol Res Pract. (2013) 209:705–9. doi: 10.1016/j.prp.2013.08.002
77. Chen GS, Lu MP, Wu MT. Differential expression of matrix metalloproteinase-2 by fibroblasts in co-cultures with keratinocytes, basal cell carcinoma and melanoma. J Dermatol. (2006) 33:609–15. doi: 10.1111/j.1346-8138.2006.00141.x
78. Boyd S, Tolvanen K, Virolainen S, Kuivanen T, Kyllönen L, Saarialho-Kere U. Differential expression of stromal MMP-1, MMP-9 and TIMP-1 in basal cell carcinomas of immunosuppressed patients and controls. Virchows Arch. (2008) 452:83–90. doi: 10.1007/s00428-007-0526-0
79. Tjiu JW, Chen JS, Shun CT, Lin SJ, Liao YH, Chu CY, et al. Tumor-associated macrophage-induced invasion and angiogenesis of human basal cell carcinoma cells by cyclooxygenase-2 induction. J Invest Dermatol. (2009) 129:1016–25. doi: 10.1038/jid.2008.310
80. Yamaguchi H, Sakai R. Direct Interaction between Carcinoma Cells and Cancer Associated Fibroblasts for the Regulation of Cancer Invasion. Cancers. (2015) 7:2054–62. doi: 10.3390/cancers7040876
81. Ciazyńska M, Bednarski IA, Wódz K, Kolano P, Narbutt J, Sobjanek M, et al. Proteins involved in cutaneous basal cell carcinoma development. Oncol Lett. (2018) 16:4064–72. doi: 10.3892/ol.2018.9126
82. Manola I, Mataic A, Drvar DL, Pezelj I, Dzombeta TR, Kruslin B. Peritumoral clefting and expression of MMP-2 and MMP-9 in basal cell carcinoma of the skin. In Vivo. (2020) 34:1271–5. doi: 10.21873/invivo.11901
83. Impola U, Jeskanen L, Ravanti L, Syrjänen S, Baldursson B, Kähäri VM, et al. Expression of matrix metalloproteinase (MMP)-7 and MMP-13 and loss of MMP-19 and p16 are associated with malignant progression in chronic wounds. Br J Dermatol. (2005) 152:720–6. doi: 10.1111/j.1365-2133.2005.06447.x
84. Yucel T, Mutnal A, Fay K, Fligiel SE, Wang T, Johnson T, et al. Matrix metalloproteinase expression in basal cell carcinoma: relationship between enzyme profile and collagen fragmentation pattern. Exp Mol Pathol. (2005) 79:151–60. doi: 10.1016/j.yexmp.2005.05.003
85. Mercut I-M, Simionescu CE, Stepan AE, Andreiana BC, Ciurea A-M, Mercut R, et al. The immunoexpression of MMP-1 and MMP-13 in eyelid basal cell carcinoma. Rom J Morphol Embryol. (2020) 61:1221. doi: 10.47162/RJME.61.4.23
86. Greco M, Arcidiacono B, Chiefari E, Vitagliano T, Ciriaco AG, Brunetti FS, et al. HMGA1 and MMP-11 Are overexpressed in human non-melanoma skin cancer. Anticancer Res. (2018) 38:771–8. doi: 10.21873/anticanres.12283
87. Viiklepp K, Nissinen L, Ojalill M, Riihilä P, Kallajoki M, Meri S, et al. C1r upregulates production of matrix metalloproteinase-13 and promotes invasion of cutaneous squamous cell carcinoma. J Invest Dermatol. (2021) 28:S0022-202X02379-4. doi: 10.1016/j.jid.2021.10.008
88. Yang S-H, Liu C-T, Hong C-Q, Huang Z-Y, Wang H-Z, Wei L-F, et al. Autoantibodies against p53, MMP-7, and Hsp70 as Potential Biomarkers for Detection of Nonmelanoma Skin Cancers. Dis Markers. (2021)2021:5592693. doi: 10.1155/2021/5592693
89. Hartmann-Petersen S, Tammi RH, Tammi MI, Kosma VM. Depletion of cell surface CD44 in nonmelanoma skin tumours is associated with increased expression of matrix metalloproteinase 7. Br J Dermatol. (2009) 160:1251–7. doi: 10.1111/j.1365-2133.2009.09031.x
90. Wang H, Li H, Yan Q, Gao S, Gao J, Wang Z, et al. Serum matrix metalloproteinase-13 as a diagnostic biomarker for cutaneous squamous cell carcinoma. BMC Cancer. (2021) 21:816. doi: 10.1186/s12885-021-08566-1
91. O'Grady A, Dunne C, O'Kelly P, Murphy GM, Leader M, Kay E. Differential expression of matrix metalloproteinase (MMP)-2, MMP-9 and tissue inhibitor of metalloproteinase (TIMP)-1 and TIMP-2 in non-melanoma skin cancer: implications for tumour progression. Histopathology. (2007) 51:793–804. doi: 10.1111/j.1365-2559.2007.02885.x
92. Gozdzialska A, Wojas-Pelc A, Drag J, Brzewski P, Jaśkiewicz J, Pastuszczak M. Expression of metalloproteinases (MMP-2 and MMP-9) in basal-cell carcinoma. Mol Biol Rep. (2016) 43:1027–33. doi: 10.1007/s11033-016-4040-9
93. Quail DF, Joyce JA. Microenvironmental regulation of tumor progression and metastasis. Nat Med. (2013) 19:1423–37. doi: 10.1038/nm.3394
94. Bulman A, Neagu M, Constantin C. Immunomics in skin cancer - improvement in diagnosis, prognosis and therapy monitoring. Curr Prot. (2013) 10:202–17. doi: 10.2174/1570164611310030003
95. Lee N. Zakka LR. Mihm MCJr, Schatton T. Tumour infiltrating lymphocytes in melanoma prognosis and cancer immunotherapy. Pathology. (2016) 48:177–87. doi: 10.1016/j.pathol.2015.12.006
96. Li M, Li S, Zhou L, Yang L, Wu X, Tang B, et al. Immune infiltration of MMP14 in pan cancer and its prognostic effect on tumors. Front Oncol. (2021) 11:717606. doi: 10.3389/fonc.2021.717606
97. Ghita MA, Caruntu C, Rosca AE, Kaleshi H, Caruntu A, Moraru L, et al. Reflectance confocal microscopy and dermoscopy for in vivo, noninvasive skin imaging of superficial basal cell carcinoma. Oncology Lett. (2016) 11:3019–24. doi: 10.3892/ol.2016.4354
98. Georgescu SR, Tampa M, Mitran CI, Mitran MI, Caruntu C, Caruntu A, et al. Tumour Microenvironment in Skin Carcinogenesis. Adv Exp Med Biol. (2020) 1226:123–42. doi: 10.1007/978-3-030-36214-0_10
99. Pettersen JS, Fuentes-Duculan J, Suárez-Fariñas M, Pierson KC, Pitts-Kiefer A, Fan L, et al. Tumor-associated macrophages in the cutaneous SCC microenvironment are heterogeneously activated. J Invest Dermatol. (2011) 131:1322–30. doi: 10.1038/jid.2011.9
100. Tampa M, Mitran MI, Mitran CI, Sarbu MI, Matei C, Nicolae I, et al. Mediators of Inflammation–A Potential Source of Biomarkers in Oral Squamous Cell Carcinoma. J Immunol Res. (2018) 2018:1061780. doi: 10.1155/2018/1061780
101. Alves AM, Diel LF, Lamers ML. Macrophages and prognosis of oral squamous cell carcinoma: a systematic review. J Oral Pathol Med. (2018) 47:460–67. doi: 10.1111/jop.12643
102. Koontongkaew S. The tumor microenvironment contribution to development, growth, invasion and metastasis of head and neck squamous cell carcinomas. J Cancer. (2013) 4:66–83. doi: 10.7150/jca.5112
103. Tsai MT, Sun YS, Keerthi M, Panda AK, Dhawan U, Chang YH, et al. Oral Cancer Theranostic Application of FeAu Bimetallic Nanoparticles Conjugated with MMP-1 Antibody. Nanomaterials. (2021) 12:1–14. doi: 10.3390/nano12010061
104. Gonzalez-Avila G, Sommer B, García-Hernández AA, Ramos C. Matrix Metalloproteinases' Role in Tumor Microenvironment. Adv Exp Med Biol.(2020). 1245:97–131. doi: 10.1007/978-3-030-40146-7_5
105. Tampa M, Georgescu SR, Mitran MI, Mitran CI, Matei C, Caruntu A, et al. Current Perspectives on the Role of Matrix Metalloproteinases in the Pathogenesis of Basal Cell Carcinoma. Biomolecules. (2021) 11:903. doi: 10.3390/biom11060903
106. Pellegrini C, Orlandi A, Costanza G, Di Stefani A, Piccioni A, Di Cesare A, et al. Expression of IL-23/Th17-Related Cytokines in Basal Cell Carcinoma and in the Response to Medical Treatments. PLoS ONE. (2017) 12:e0183415. doi: 10.1371/journal.pone.0183415
107. Beksaç B, Ilter N, Erdem Ö, Çakmak P, Çenetoglu S, Yapar D. Sparsity of dendritic cells and cytotoxic T cells in tumor microenvironment may lead to recurrence in basal cell carcinoma. Int J Dermatol. (2020) 59:1258–63. doi: 10.1111/ijd.15065
108. König S, Nitzki F, Uhmann A, Dittmann K, Theiss-Suennemann J, Herrmann M, et al. Depletion of cutaneous macrophages and dendritic cells promotes growth of basal cell carcinoma in mice. PLoS ONE. (2014) 9:e93555. doi: 10.1371/journal.pone.0093555
109. Omland SH. Local immune response in cutaneous basal cell carcinoma. Dan Med J. (2016) 64:B5412.
110. Nissinen L, Kähäri VM. Matrix metalloproteinases in inflammation. Biochim Biophys Acta. (2014) 1840:2571–80. doi: 10.1016/j.bbagen.2014.03.007
111. Gonzalez-Avila G, Sommer B, Mendoza-Posada DA, Ramos C, Garcia-Hernandez AA, Falfan-Valencia R. Matrix metalloproteinases participation in the metastatic process and their diagnostic and therapeutic applications in cancer. Crit Rev Oncol Hematol. (2019) 137:57–83. doi: 10.1016/j.critrevonc.2019.02.010
112. Jin HT, Ahmed R, Okazaki T. Role of PD-1 in regulating T-cell immunity. Curr Top Microbiol Immunol. (2011) 350:17–37. doi: 10.1007/82_2010_116
113. Lipson EJ, Lilo MT, Ogurtsova A, Esandrio J, Xu H, Brothers P, et al. Basal cell carcinoma: PD-L1/PD-1 checkpoint expression and tumor regression after PD-1 blockade. J Immunother Cancer. (2017) 5:23. doi: 10.1186/s40425-017-0228-3
114. Mahmoudian RA, Mozhgani S, Abbaszadegan MR, Mokhlessi L, Montazer M, Gholamin M. Correlation between the immune checkpoints and EMT genes proposes potential prognostic and therapeutic targets in ESCC. J Mol Histol. (2021) 52:597–609. doi: 10.1007/s10735-021-09971-3
115. Caruntu A, Moraru L, Surcel M, Munteanu A, Tanase C, Constantin C, et al. Assessment of immune cell populations in tumor tissue and peripheral blood samples from head and neck squamous cell carcinoma patients. Anal Cell Pathol. (2021) 2021:2328218. doi: 10.1155/2021/2328218
116. Boxberg M, Leising L, Steiger K, Jesinghaus M, Alkhamas A, Mielke M, et al. Composition and clinical impact of the immunologic tumor microenvironment in oral squamous cell carcinoma. J Immunol. (2019) 202:278–91. doi: 10.4049/jimmunol.1800242
117. Hu Y, He MY, Zhu LF, Yang CC, Zhou ML, Wang Q, et al. Tumor-associated macrophages correlate with the clinicopathological features and poor outcomes via inducing epithelial to mesenchymal transition in oral squamous cell carcinoma. J Exp Clin Cancer Res. (2016) 35:12. doi: 10.1186/s13046-015-0281-z
118. Bai XY Li S, Wang M, Li X, Yang Y, Xu Z, et al. Krüppel-like factor 9 down-regulates matrix metalloproteinase 9 transcription and suppresses human breast cancer invasion. Cancer Lett. (2018) 412:224–35. doi: 10.1016/j.canlet.2017.10.027
119. Tsai CL, Chen WC, Hsieh HL, Chi PL, Hsiao LD, Yang CM. TNF-α induces matrix metalloproteinase-9-dependent soluble intercellular adhesion molecule-1 release via TRAF2-mediated MAPKs and NF-κB activation in osteoblast-like MC3T3-E1 cells. J Biomed Sci. (2014) 21:12. doi: 10.1186/1423-0127-21-12
120. Gou X, Chen H, Jin F, Wu W, Li Y, Long J, et al. Expressions of CD147, MMP-2 and MMP-9 in laryngeal carcinoma and its correlation with poor prognosis. Pathol Oncol Res. (2014) 20:475–81. doi: 10.1007/s12253-013-9720-3
121. Li YF, Han CC, Wang Y, Cui DQ, Luo TT, Zhang YW, et al. Combined PGE2 with TNF-α promotes laryngeal carcinoma progression by enhancing GRK2 and TRAF2 interaction. Neoplasma. (2020) 67:354–63. doi: 10.4149/neo_2020_190526N463
122. Costa-Pinheiro P, Montezuma D, Henrique R, Jerónimo C. Diagnostic and prognostic epigenetic biomarkers in cancer. Epigenomics. (2015) 7:1003–15. doi: 10.2217/epi.15.56
123. Tan EM, Zhang J. Autoantibodies to tumor-associated antigens: reporters from the immune system. Immunol Rev. (2008) 222:328–40. doi: 10.1111/j.1600-065X.2008.00611.x
124. Caruntu A, Moraru L, Surcel M, Munteanu A, Costache DO, Tanase C, et al. Persistent changes of peripheral blood lymphocyte subsets in patients with oral squamous cell carcinoma. Healthcare. (2022) 10:342.” doi: 10.3390/healthcare10020342
125. Neagu M, Constantin C, Cretoiu SM, Zurac S. miRNAs in the Diagnosis and prognosis of skin cancer. Front Cell Dev Biol. (2020) 8:71. doi: 10.3389/fcell.2020.00071
126. Lee KS, Shin S, Cho E, Im WK, Jeon SH, Kim Y, et al. nc886, a non-coding RNA, inhibits UVB-induced MMP-9 and COX-2 expression via the PKR pathway in human keratinocytes. Biochem Biophys Res Commun. (2019) 512:647–52. doi: 10.1016/j.bbrc.2019.01.068
127. Xiao Q, Li X, Li Y, Wu Z, Xu C, Chen Z, et al. Biological drug and drug delivery-mediated immunotherapy. Acta Pharm Sin B. (2021) 11:941–60. doi: 10.1016/j.apsb.2020.12.018
128. Gordon SR, Maute RL, Dulken BW, Hutter G, George BM, McCracken MN, et al. PD-1 Expression by tumour-associated macrophages inhibits phagocytosis and tumour immunity. Nature. (2017) 545:495–99. doi: 10.1038/nature22396
129. Topalian SL, Drake CG, Pardoll DM. Immune checkpoint blockade: a common denominator approach to cancer therapy. Cancer Cell. (2015) 27:450–61. doi: 10.1016/j.ccell.2015.03.001
130. Ye Y, Kuang X, Xie Z, Liang L, Zhang Z, Zhang Y, et al. Small-molecule MMP2/MMP9 inhibitor SB-3CT modulates tumor immune surveillance by regulating PD-L1. Genome Med. (2020) 12:83. doi: 10.1186/s13073-020-00780-z
131. Moses RL, Boyle GM, Howard-Jones RA, Errington RJ, Johns JP, Gordon V, et al. Novel epoxy-tiglianes stimulate skin keratinocyte wound healing responses and re-epithelialization via protein kinase C activation. Biochem Pharmacol. (2020) 178:114048. s.s doi: 10.1016/j.bcp.2020.114048
Keywords: extracellular matrix, hyaluronan, collagen, proteoglycans, metalloproteinases, skin
Citation: Kavasi R-M, Neagu M, Constantin C, Munteanu A, Surcel M, Tsatsakis A, Tzanakakis GN and Nikitovic D (2022) Matrix Effectors in the Pathogenesis of Keratinocyte-Derived Carcinomas. Front. Med. 9:879500. doi: 10.3389/fmed.2022.879500
Received: 19 February 2022; Accepted: 11 April 2022;
Published: 29 April 2022.
Edited by:
Ivan V. Litvinov, McGill University, CanadaReviewed by:
Joanna Czuwara, Medical University of Warsaw, PolandManuel Valdebran Canales, Medical University of South Carolina, United States
Copyright © 2022 Kavasi, Neagu, Constantin, Munteanu, Surcel, Tsatsakis, Tzanakakis and Nikitovic. This is an open-access article distributed under the terms of the Creative Commons Attribution License (CC BY). The use, distribution or reproduction in other forums is permitted, provided the original author(s) and the copyright owner(s) are credited and that the original publication in this journal is cited, in accordance with accepted academic practice. No use, distribution or reproduction is permitted which does not comply with these terms.
*Correspondence: Dragana Nikitovic, nikitovic@uoc.gr