- 1Department of Critical Care Medicine, Peking Union Medical College Hospital, Peking Union Medical College and Chinese Academy of Medical Science, Beijing, China
- 2Department of Health Care, Peking Union Medical College Hospital, Peking Union Medical College and Chinese Academy of Medical Sciences, Beijing, China
Point-of-care ultrasonography (POCUS) is performed by a treating clinician at the patient's bedside, provides a acquisition, interpretation, and immediate clinical integration based on ultrasonographic imaging. The use of POCUS is not limited to one specialty, protocol, or organ system. POCUS provides the treating clinician with real-time diagnostic and monitoring information. Visual rounds based on multiorgan POCUS act as an initiative to improve clinical practice in the Intensive Care Unit and are urgently needed as part of routine clinical practice.
Introduction
Patient care rounds are a central part of daily clinical routine and play a vital role in quality management in intensive care. Point-of-care ultrasonography (POCUS), an emerging tool performed by frontline clinicians caring for critically ill patients especially in the intensive care unit (ICU), has been strongly supported by a growing body of evidence in several disciplines (1). POCUS could rapidly and accurately access their patients' clinical status to guide therapy, especially in circulatory and respiratory failure. Multiorgan POCUS further combines organ functions such as the renal, gastrointestinal, and neurologic systems (2). Visual rounds based on multiorgan POCUS act as an initiative to improve clinical practice and are urgently needed for routine clinical care. We will describe in this review the use of POCUS as part of the daily round in the intensive care unit, and will highlight the emerging use of visual rounds based on multiorgan POCUS as an initiative to improve clinical practice in the ICU.
Materials and Methods
A literature search of Pubmed, Medline, Embase, Scopus and Cochrane library databases was conducted for the period Jan 1st 2000–2022 to identify all publications on point of care ultrasound in critical ill adult patients, using English language restriction, and the following MeSH query: [“point of care ultrasound” OR (“lung ultrasound”) OR “echocardiography” OR “cardiac ultrasound” OR “renal ultrasound” OR “gastrointestinal ultrasound” OR “abdominal ultrasound” OR “brain ultrasound” OR “neurological ultrasound”] AND [“critical care”]. Non-pertinent findings were discarded. Duplicates were removed. This strategy identified 873 records (Supplementary File 1).
Critical Care Ward Round
Critical care is always facing complex, life-threatening conditions and needs to make rapid decision on account of incomplete data. Patient care rounds in the ICU include discussions based on reviewing clinical data and concluding care plans (3). Rounds are a crucial portion in daily clinical schedule in ICU. During patient care rounds, essential diagnostic, therapeutic and organizational affairs of patient care should be discussed in a structured form (4). The critical cognition round ranged from basic clinical scenarios and professional courses to a multidisciplinary round. Rounds play a vital impact on patient safety and quality management in the ICU (5).
Point-of-Care Ultrasonography
POCUS is ubiquitous in critical care and advantages on its real-time and absence of radiation. POCUS has quickly emerged as a tool used by frontline clinicians caring for critically ill patients. It enables quickly identifying and guiding the management of conditions with hemodynamic instability or respiratory failure and is the standard of care for procedural guidance (6). POCUS developed from early identification to assessment admission to visual rounds, finally building a critical thinking system. Currently, more and more evidence strongly supports the significance of POCUS in numerous fields, especially highlighted in the management of COVID-19 (7, 8). Although respiratory abnormities are the most common performance, other organ systems may also be affected by COVID-19. POCUS is particularly appealing because it is not only rapid, bedside, and goal-oriented but also allows for the imaging of the cardiovascular, respiratory, abdominal, renal and neurological system (Table 1).
Visual Rounds Based on Multiorgan POCUS
Cardiovascular System
POCUS is not only a diagnostic tool but also helps making clinical decision in real time at the bedside. Critical care echocardiography (CCE) (9), including transthoracic echocardiography (TTE) and transesophageal echocardiography (TEE), facilitates the correct classification of shock (10, 11). 1. Volume Status: A perpetual challenge observed daily in the ICU is estimating volume status and assessing their potential of cardiac output augmentation with the infusion of intravascular volume. The respiratory variation of the inferior vena cava has been widely used to determine volume responsiveness, which should also be interpreted combining the patient's underlying pathophysiological status like the mode of ventilation/respiration, paitient‘s respiratory pattern, cardiac conditions and other factors like increased abdominal pressure (12). Variables based on stroke volume or cardiac output were also combined with other factors to predict flood responsiveness (13). 2. Ventricular Function: Assessment of left ventricular (LV) systolic function (as well as cardiac output), LV diastolic function (left atrial pressure) and right ventricular dysfunction (pulmonary hypertension) are skills required for CCE. LV systolic dysfunction, based on the regions involved, could be divided into regional wall motion abnormality (RWMA) and diffuse hypokinesia. 3. Organic Abnormality: Assessment of valvular stenosis and regurgitation are also skilled by CCE through the application of pulsed wave and continuous wave Doppler. Intensivists with CCE knowledge could assess evidence of pericardial effusion, even cardiac tamponade, which are common reasons of obstructive shock. Left ventricular outflow tract obstruction (LVOTO) can also be easily diagnosed through CCE which is often underestimated in ICU patients (Figure 1).
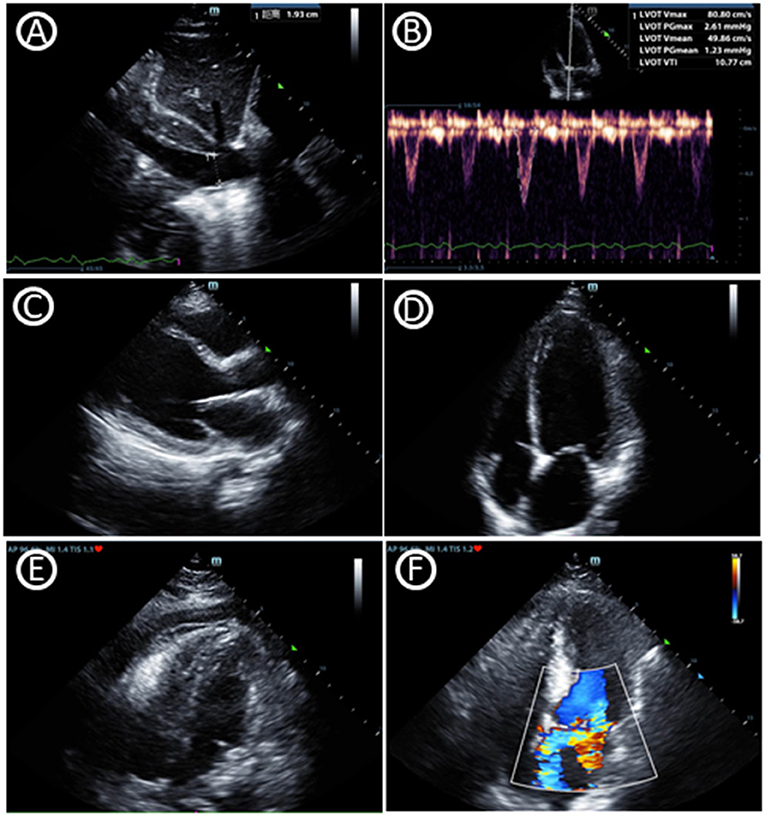
Figure 1. Critical care echocardiography imaging. (A) Inferior vena cava diameter. (B) Velocity-time integral of left ventricular outflow tract, which can help to gain cardiac output and fluid responsiveness. (C) Parasternal window parasternal long-axis view. (D) Apical window four-chamber view. (E) Pericardial effusion in subcostal window four-chamber view. (F) Mitral regurgitation in apical window four-chamber view.
Overall, CCE could efficiently assist in early shock diagnosis, typically categorized into hypovolemic, cardiogenic, obstructive, and distributive shock (14). Based on the instructions of CCE, intensivists could rapidly and accurately assess their patients' clinical state to guide therapy, such as fluid resuscitation, the application of inotropes, and even pericardiocentesis in cardiac tamponade or the use of thrombolytics for massive pulmonary embolism. Therefore, an increasing number of comprehensive reviews support use of CCE in the management of hemodynamic failure (10, 11, 15, 16). Vignon et al. also conducted a prospective multicenter study comparing hemodynamic assessment in sepsis patients through CCE and invasive transpulmonary thermodilution, the agreement was moderate to good (17). Moreover, the accuracy of critical care TEE was also confirmed compared with TEE or TTE performed by cardiologists (18). All of the above findings indicate that intensivists performing CCE should be a standard constitution of daily practice for critical care. However, some affairs should be taken into consideration when interpreting signs of CCE. A variety of abnormal signs found in critically ill patients could be not only the causes of hemodynamic instability, but also the consequences of preexisting cardiac diseases (19). What‘s more, CCE may also be influence by other factors like the respiratory state. For example, the respiratory variation of the inferior vena cava, which reflects circulatory capacity and cardiac function, is also related with respiratory effort and intrathoracic pressure.
Respiratory System
Although the use of lung ultrasonography (LUS) in critical care demonstrated by Dr. Daniel Lichtenstein in the 1990s (20), it has been especially highlighted in the management of COVID-19 in recent years (8). LUS is skilled in identifying lung conditions based on the constitution of air and fluid inside (Figure 2). 1. Pneumothorax: As a differential diagnosis for dyspnea in the ICU, pneumothoraces occur episodically. The presence of a “lung pulse” may exclude pneumothorax with 100% specificity and 66% sensitivity (21, 22). The presence of lung sliding may also exclude pneumothorax with a 100% negative predictive value (23, 24). 2. Interstitial Syndrome: B-lines in LUS are a type of comet-tail artifact, which are characterized in the interstitial syndrome. B-lines root from thickened interlobular septae, however the clinician must identify its etiology from fibrosis, pulmonary edema or inflammation. 3. Consolidation: Consolidation is easily accessed by LUS since lung tissue is airless. Clinician also need to identify its etiology from atelectasis, pneumonia and even pulmonary infarct. 4. Pleural Effusion: LUS can easily detect pleural effusion and provide more information on fluid characteristics. For instance, COVID-19-associated pneumonia present with heterogeneous B-line, irregular pleura and consolidations similar as other viral pneumonia (25).
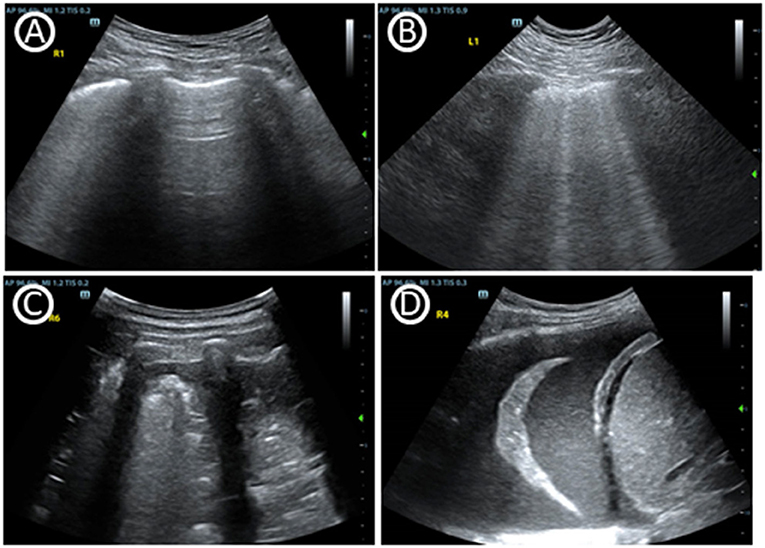
Figure 2. Basic signs of lung ultrasound. (A) A-lines, horizontal reverberation artifacts at a regular distance indicate a high gas–volume ratio below the parietal pleura. (B) Multiple B lines, are vertical artifacts deriving from the pleural line, moving synchronously with lung sliding, usually reaching the bottom of the screen and erasing A-lines. (C) Tissue-like pattern identifies lobar consolidation. (D) Pleural effusion.
As a worldwide effort to spread POCUS as a basic skill in ICU, LUS is outstanding for a wide variety of lung conditions. 1. Repiratory failure diagnosis: As is shown above, LUS is skilled in identifying lung conditions based on the constitution of air and fluid inside, to distinguish phenmothorax, interstitial syndrome, consolidation or pleural effusion. LUS has shown to be superior in evaluating patients with dyspnea (26), especially in detecting pulmonary congestion in heart failure (27, 28). LUS was recommened for patients with acute dyspnea especially by American College of Physicians and Society of Critical Care Medicine (Table 2) (29, 30). LUS is an invaluable tool in the intensivist's armamentarium as a valuable alternative to CXR or CT (31, 32). Quantitative LUS analysis was superior in the assessment of extravascular lung water than CT, which is advantage in physical density (33). LUS is also outstanding in diagnosis and management of acute respiratory distress syndrome (ARDS). ARDS can be easily confirmed by LUS through the recognition of a typical pattern characterized by B-lines, spared areas, pleural line thickening, and subpleural consolidations (34). LUS in conjunction with CCE could help to detect not only cardiogenic pulmonary edema but also acute pulmonary embolism (PE). 2. respiratory support strategies: In addition to establishing a diagnosis of acute dyspnea, LUS could also assist in respiratory support strategies (35). LUS has the advantages of predicting response to recruitment (36), prone positioning (37, 38), assessment of extravascular lung water and fluid management (39, 40), and even extracorporeal membrane oxygenation (ECMO) support (41). Combined with CCE, RV function is also important to be evaluated in the ventilation strategy and management in ARDS. 3.Ventilation weaning: Diaphragmatic ultrasound including diaphragmatic thickness, its fraction and mobility may be a valid tool (42). LUS combining with CCE and diaphragm ultrasound may effectively reduce ventilation-induced diaphragmatic injury (43, 44) and extubation failure (44–47). Diaphragmatic weakness and spontaneous respiration induced diaphragmatic injury are still need further studied and verified. LUS is a rapid and convenient imaging technology that has important applications for daily use in the ICU.
However, LUS is limited since it‘s only sensitive with lung lesions adjacent to the pleural surface. Although LUS provides relevant information on morphological and functional changes occurring in the lungs, it still need to be automated to reduce inter- and intra-observer variability. Visual LUS score could indicate pulmonary edema, but it‘s poorly correlated with pulmonary capillary wedge pressure and extravascular lung water at high PEEP (48). LUS could help score PEEP-induced lung recruitment, however it could not assess PEEP-induced hyperinflation which need to combine other imagine tools (49). In addition, use of LUS should take into account patient population and clinical conditions. For example, the sensitivity of LUS in the FAST exam in pediatric trauma might not be as reliable as in adults (50). In the initial evaluation of chest trauma, using LUS as the primary imaging modality should be considered with caution (51).
Renal Ultrasound
Acute kidney injury (AKI), shown as oliguria or elevated serum blood urea nitrogen or creatinine, is common in ICU. Renal ultrasound may help clinician to define the circumstances bedside (Figure 3) (52, 53). The CCE that was mentioned previously could guide shock differentiation and volume status assessment. Renal ultrasound could evaluate renal size, echogenicity, vascularity, and the presence of urinary tract obstruction, imaging both kidneys and the bladder. Resistive index (RI) of the arcuate and interlobar arteries was widely studied as a potential objective marker for renal pathology, and was shown to be a prognostic factor (54, 55). RI showed advantage in detecting hypoperfusion due to hypovolemia and hemodynamics titration to improve renal blood flow (56). However, although RI has been studied extensively, its interpretation remains particularly complex and is highly dependent on the clinical setting (57). Renal contrast-enhanced ultrasonography can be used to non-invasively evaluate renal macrocirculation and microcirculation in critical ill patients. Decrease in renal blood flow, particularly cortical blood flow, may be observed in septic AKI through the technology and may contribute to its development (58).
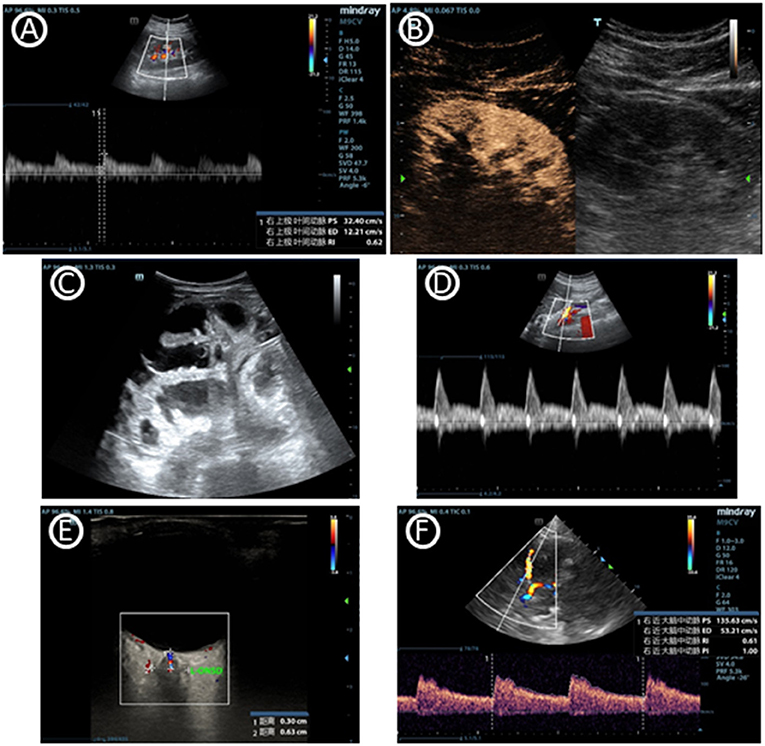
Figure 3. Images of multiorgan ultrasound. (A) Normal doppler tracing of the interlobar vessels in renal ultrasound. The renal resistive index can be measured using the maximal and the minimal arterial velocity during the cardiac cycle. (B) Renal contrast-enhanced ultrasonography. (C) Dilated fluid-filled bowel loops that indicates small bowel obstruction. (D) Blood flow curve of superior mesenteric vein. (E) Optic nerve sheath diameter, which can help to predict intracranial pressure. (F) Cerebral blood flow velocity of middle cerebral artery accessed by transcranial doppler ultrasonography.
Gastrointestinal Ultrasound
In addition to the Focused Assessment with Sonography in Trauma protocol (FAST) used in trauma patients, there is an additional focus on gastrointestinal (GI) failure or dysfunction that is evaluated by ultrasound (59–61). There is currently a lack of universally accepted criteria for gastrointestinal failure or dysfunction in critical care. Gastrointestinal ultrasound can provide its anatomical and functional information through the lumen, wall and the surrounding structures of the stomach and bowel. Evaluation of functional processes like peristalsis and blood flow may be used in combination (Figure 3) (62). Monitoring GI function through gastrointestinal ultrasound may effectively assess acute gastrointestinal injury, predict feeding intolerance and lead to appropriate therapeutic interventions. An interdisciplinary group of European experts also summarizes use of gastrointestinal ultrasound in identifying causes of acute abdomen like bowel obstruction, gastrointestinal perforation and acute ischemic bowel disease (Table 2) (63). Just like other ultrasound types, adequate training is needed to use and interpret the ultrasound images correctly. In gastrointestinal ultrasound, image quality was easily influenced by air-filled bowel or obesity.
Neurological Ultrasound
In the clinical management of several neurological diseases, intracranial pressure (ICP) is an important monitoring indicator. Since ICP is not conveniently monitored, non-invasive monitoring of ICP (nICP) may be helpful to improve the clinical management of these conditions. Cerebral blood flow velocity (FV) accessed by Transcranial Doppler ultrasonography (TCD) is an effective technique (64, 65). The optic nerve sheath diameter measurement may also reveal that a congested papilla develops with optic disc elevation when the increase in ICP persists (Figure 3) (66, 67). Neurological ultrasound combing with LUS also helps when intracranial hypertension and severe lung damage coexist in the same clinical scenario, since recommending ventilation strategies in ARDS may potentially increase intracranial hypertension. Combined use of LUS with optic nerve sheath diameter assessment and TCD can contribute to a tailored brain-protective ventilation strategy (68). However, some studies showed TCD may errorly affect clinical decision-making. Perhaps it is because of the different elastic modulus of different intracranial tissues that ICP cannot be measured like the liquid pressure in a closed container. ICP monitoring based on TCD is a potential research direction, but its clinical value is still further verified.
Perspectives and New Applications of POCUS
Key trends and future applications in POCUS technology may include handheld ultrasound system and artificial intelligence. Handheld ultrasound systems based on the telemedicine technology is becoming available to the frontline clinician (69). The number of probes necessary affects its clinical utility. Artificial intelligence based on quantitative analysis is advancing. Tele-ICU, which promoting the use of telemedicine in critically ill patients, has been vigorously development in recent years (70, 71). The use of telecommunications has become the prototype for telemedicine in health care practice and has been described since the advent of telecommunication. Imaging and visual rounds are of increasing importance. New prospects for use of POCUS not only as a diagnostic but also as a monitoring tool are approaching. Automatic and quantitative scoring system was developed to integrate and standardize the clinical assessment of the lung (72, 73). Classification of benchmark ultrasound images is required for training a convolutional artificial network (74). The 5G-powered robot-assisted teleultrasound diagnostic system has already been applied in intensive care units (75), which has an advantage as easy operation, good feasibility, comparable diagnosis, and deserves to promote widely in clinical practice.
However, the lack of standardized therapeutic plans based on POCUS results makes it‘s difficult to study the effect of POCUS on patient outcomes (1). What‘s more, the clinician using POCUS must be competent in it, POCUS training poses a great challenge as it is developing rapidly. The training curricula and methods to assess competence is imperative for the safe and effective use of such systems (76).
Conclusion
Recognization and estimation of critical manifestation is the principal objective in constructing a therapeutic plan. POCUS is now increasingly comprehensive and used in predicting pathogenesis and guiding multiorgan function monitoring. Circulatory and respiratory failure is the major concern of critical scenarios, POCUS is not only advantaged in analysis of this, but also combining organ functions such as the renal, gastrointestinal, and neurologic systems. POCUS is now recognized as a fundamental component of critical care. The wide range of applications of POCUS includes the evaluation of the critical cause as well as therapy monitoring. Future development on handheld ultrasound system and artificial intelligence point toward POCUS as a standard tool of the frontline clinician. Overall, the clearly delineated competencies of POCUS has been recommended for critical care fellowship training for more than a decade. Visual rounds based on multiorgan POCUS in the ICU are an urgently needed part of routine clinical care.
Author Contributions
D-WL designed the experiment. J-YM drafted and revised the manuscript. H-MZ and X-TW conceived of the study, participated in its design of the study. All authors have read the manuscript and approved of the version to be published.
Funding
This work was supported by grants from the Natural Science Foundation of Beijing Municipality (No. 7194306).
Conflict of Interest
The authors declare that the research was conducted in the absence of any commercial or financial relationships that could be construed as a potential conflict of interest.
Publisher's Note
All claims expressed in this article are solely those of the authors and do not necessarily represent those of their affiliated organizations, or those of the publisher, the editors and the reviewers. Any product that may be evaluated in this article, or claim that may be made by its manufacturer, is not guaranteed or endorsed by the publisher.
Acknowledgments
This manuscript has been edited and proofread by American Journal Experts.
Supplementary Material
The Supplementary Material for this article can be found online at: https://www.frontiersin.org/articles/10.3389/fmed.2022.869958/full#supplementary-material
Abbreviations
POCUS, point-of-care ultrasonography; ICU, intensive care unit; CCE, critical care echocardiography; TTE, transthoracic echocardiography; TEE, transesophageal echocardiography; LV, left ventricular; RWMA, regional wall motion abnormality; LVOTO, left ventricular outflow tract obstruction; LUS, lung ultrasonography; CT, computed tomography; CXR, chest x-ray; ARDS, acute respiratory distress syndrome; ECMO, extracorporeal membrane oxygenation; PE, pulmonary embolism; AKI, acute kidney injury; RI, resistive index; FAST, Focused Assessment with Sonography in Trauma; GI, gastrointestinal; ICP, intracranial pressure; FV, flow velocity; TCD, Transcranial Doppler Ultrasonography.
References
1. Díaz-Gómez JL, Mayo PH, Koenig SJ. Point-of-care ultrasonography. N Engl J Med. (2021) 385:1593–602. doi: 10.1056/NEJMra1916062
2. Pelosi P, Corradi F. Ultrasonography in the intensive care unit: looking at the world through colored glasses. Anesthesiology. (2012) 117:696–8. doi: 10.1097/ALN.0b013e318264c663
3. Lane D, Ferri M, Lemaire J, McLaughlin K, Stelfox HT. A systematic review of evidence-informed practices for patient care rounds in the ICU*. Crit Care Med. (2013) 41:2015–29. doi: 10.1097/CCM.0b013e31828a435f
4. Kumpf O, Braun JP, Brinkmann A, Bause H, Bellgardt M, Bloos F, et al. Quality indicators in intensive care medicine for Germany - third edition 2017. Ger Med Sci. (2017) 15:Doc10. doi: 10.3205/000251
5. Hillmann B, Schwarzkopf D, Manser T, Waydhas C, Riessen R. Structure and concept of ICU rounds: the VIS-ITS survey. Med Klin Intens Notfmed. (2021). doi: 10.1007/s00063-021-00830-3. [Epub ahead of print].
6. Guevarra K, Greenstein Y. Ultrasonography in the critical care unit. Curr Cardiol Rep. (2020) 22:145. doi: 10.1007/s11886-020-01393-z
7. Volpicelli G, Mayo P, Rovida S. Focus on ultrasound in intensive care. Intens Care Med. (2020) 46:1258–60. doi: 10.1007/s00134-020-06027-0
8. Hussain A, Via G, Melniker L, Goffi A, Tavazzi G, Neri L, et al. Multi-organ point-of-care ultrasound for COVID-19 (PoCUS4COVID): international expert consensus. Crit Care. (2020) 24:702. doi: 10.1186/s13054-020-03369-5
9. Mitchell C, Rahko PS, Blauwet LA, Canaday B, Finstuen JA, Foster MC, et al. Guidelines for performing a comprehensive transthoracic echocardiographic examination in adults: recommendations from the American Society of Echocardiography. J Am Soc Echocardiogr. (2019) 32:1–64. doi: 10.1016/j.echo.2018.06.004
10. Schmidt GA, Koenig S, Mayo PH. Shock: ultrasound to guide diagnosis and therapy. Chest. (2012) 142:1042–8. doi: 10.1378/chest.12-1297
11. Volpicelli G, Lamorte A, Tullio M, Cardinale L, Giraudo M, Stefanone V, et al. Point-of-care multiorgan ultrasonography for the evaluation of undifferentiated hypotension in the emergency department. Intens Care Med. (2013) 39:1290–8. doi: 10.1007/s00134-013-2919-7
12. Via G, Tavazzi G, Price S. Ten situations where inferior vena cava ultrasound may fail to accurately predict fluid responsiveness: a physiologically based point of view. Intens Care Med. (2016) 42:1164–7. doi: 10.1007/s00134-016-4357-9
13. Vignon P, Repessé X, Bégot E, Léger J, Jacob C, Bouferrache K, et al. Comparison of echocardiographic indices used to predict fluid responsiveness in ventilated patients. Am J Respir Crit Care Med. (2017) 195:1022–32. doi: 10.1164/rccm.201604-0844OC
14. Levitov A, Frankel HL, Blaivas M, Kirkpatrick AW, Su E, Evans D, et al. Guidelines for the appropriate use of bedside general and cardiac ultrasonography in the evaluation of critically ill patients-part II: cardiac ultrasonography. Crit Care Med. (2016) 44:1206–27. doi: 10.1097/CCM.0000000000001847
15. Vieillard-Baron A, Millington SJ, Sanfilippo F, Chew M, Diaz-Gomez J, McLean A, et al. A decade of progress in critical care echocardiography: a narrative review. Intens Care Med. (2019) 45:770–88. doi: 10.1007/s00134-019-05604-2
16. Via G, Hussain A, Wells M, Reardon R, ElBarbary M, Noble VE, et al. International evidence-based recommendations for focused cardiac ultrasound. J Am Soc Echocardiogr. (2014) 27:683.e1-.e33. doi: 10.1016/j.echo.2014.05.001
17. Vignon P, Begot E, Mari A, Silva S, Chimot L, Delour P, et al. Hemodynamic assessment of patients with septic shock using transpulmonary thermodilution and critical care echocardiography: a comparative study. Chest. (2018) 153:55–64. doi: 10.1016/j.chest.2017.08.022
18. Lau V, Priestap F, Landry Y, Ball I, Arntfield R. Diagnostic accuracy of critical care transesophageal echocardiography vs cardiology-led echocardiography in ICU patients. Chest. (2019) 155:491–501. doi: 10.1016/j.chest.2018.11.025
19. Ding X, Liu DW, Cao YG, Zhang HM, Chen H, Zhao H, et al. Ten things to be considered in practicing critical care echocardiography. Chin Med J. (2018) 131:1738–43. doi: 10.4103/0366-6999.235868
20. Lichtenstein D, Mézière G, Biderman P, Gepner A, Barré O. The comet-tail artifact. An ultrasound sign of alveolar-interstitial syndrome. Am J Respir Crit Care Med. (1997) 156:1640–6. doi: 10.1164/ajrccm.156.5.96-07096
21. Lichtenstein DA, Lascols N, Prin S, Mezière G. The “lung pulse”: an early ultrasound sign of complete atelectasis. Intens Care Med. (2003) 29:2187–92. doi: 10.1007/s00134-003-1930-9
22. Lichtenstein D, Mezière G, Biderman P, Gepner A. The “lung point”: an ultrasound sign specific to pneumothorax. Intens Care Med. (2000) 26:1434–40. doi: 10.1007/s001340000627
23. Sartori S, Tombesi P, Trevisani L, Nielsen I, Tassinari D, Abbasciano V. Accuracy of transthoracic sonography in detection of pneumothorax after sonographically guided lung biopsy: prospective comparison with chest radiography. Am J Roentgenol. (2007) 188:37–41. doi: 10.2214/AJR.05.1716
24. Rowan KR, Kirkpatrick AW, Liu D, Forkheim KE, Mayo JR, Nicolaou S. Traumatic pneumothorax detection with thoracic US: correlation with chest radiography and CT–initial experience. Radiology. (2002) 225:210–4. doi: 10.1148/radiol.2251011102
25. Volpicelli G, Lamorte A, Villén T. What's new in lung ultrasound during the COVID-19 pandemic. Intens Care Med. (2020) 46:1445–8. doi: 10.1007/s00134-020-06048-9
26. Koenig SJ, Narasimhan M, Mayo PH. Thoracic ultrasonography for the pulmonary specialist. Chest. (2011) 140:1332–41. doi: 10.1378/chest.11-0348
27. Maw AM, Hassanin A, Ho PM, McInnes MDF, Moss A, Juarez-Colunga E, et al. Diagnostic accuracy of point-of-care lung ultrasonography and chest radiography in adults with symptoms suggestive of acute decompensated heart failure: a systematic review and meta-analysis. JAMA Netw Open. (2019) 2:e190703. doi: 10.1001/jamanetworkopen.2019.0703
28. Platz E, Campbell RT, Claggett B, Lewis EF, Groarke JD, Docherty KF, et al. Lung ultrasound in acute heart failure: prevalence of pulmonary congestion and short- and long-term outcomes. JACC Heart Fail. (2019) 7:849–58. doi: 10.1016/j.jchf.2019.07.008
29. Qaseem A, Etxeandia-Ikobaltzeta I, Mustafa RA, Kansagara D, Fitterman N, Wilt TJ, et al. Appropriate use of point-of-care ultrasonography in patients with acute dyspnea in emergency department or inpatient settings: a clinical guideline from the American College of Physicians. Ann Int Med. (2021) 174:985–93. doi: 10.7326/M20-7844
30. Frankel HL, Kirkpatrick AW, Elbarbary M, Blaivas M, Desai H, Evans D, et al. Guidelines for the appropriate use of bedside general and cardiac ultrasonography in the evaluation of critically ill patients-part I: general ultrasonography. Crit Care Med. (2015) 43:2479–502. doi: 10.1097/CCM.0000000000001216
31. Oks M, Cleven KL, Cardenas-Garcia J, Schaub JA, Koenig S, Cohen RI, et al. The effect of point-of-care ultrasonography on imaging studies in the medical ICU: a comparative study. Chest. (2014) 146:1574–7. doi: 10.1378/chest.14-0728
32. Chiumello D, Mongodi S, Algieri I, Vergani GL, Orlando A, Via G, et al. Assessment of lung aeration and recruitment by CT scan and ultrasound in acute respiratory distress syndrome patients. Crit Care Med. (2018) 46:1761–8. doi: 10.1097/CCM.0000000000003340
33. Corradi F, Ball L, Brusasco C, Riccio AM, Baroffio M, Bovio G, et al. Assessment of extravascular lung water by quantitative ultrasound and CT in isolated bovine lung. Respir Physiol Neurobiol. (2013) 187:244–9. doi: 10.1016/j.resp.2013.04.002
34. Corradi F, Brusasco C, Pelosi P. Chest ultrasound in acute respiratory distress syndrome. Curr Opin Crit Care. (2014) 20:98–103. doi: 10.1097/MCC.0000000000000042
35. Bouhemad B, Mongodi S, Via G, Rouquette I. Ultrasound for “lung monitoring” of ventilated patients. Anesthesiology. (2015) 122:437–47. doi: 10.1097/ALN.0000000000000558
36. Bouhemad B, Brisson H, Le-Guen M, Arbelot C, Lu Q, Rouby JJ. Bedside ultrasound assessment of positive end-expiratory pressure-induced lung recruitment. Am J Respir Crit Care Med. (2011) 183:341–7. doi: 10.1164/rccm.201003-0369OC
37. Wang XT, Ding X, Zhang HM, Chen H, Su LX, Liu DW. Lung ultrasound can be used to predict the potential of prone positioning and assess prognosis in patients with acute respiratory distress syndrome. Crit Care. (2016) 20:385. doi: 10.1186/s13054-016-1558-0
38. Haddam M, Zieleskiewicz L, Perbet S, Baldovini A, Guervilly C, Arbelot C, et al. Lung ultrasonography for assessment of oxygenation response to prone position ventilation in ARDS. Intens Care Med. (2016) 42:1546–56. doi: 10.1007/s00134-016-4411-7
39. Zhao Z, Jiang L, Xi X, Jiang Q, Zhu B, Wang M, et al. Prognostic value of extravascular lung water assessed with lung ultrasound score by chest sonography in patients with acute respiratory distress syndrome. BMC Pulm Med. (2015) 15:98. doi: 10.1186/s12890-015-0091-2
40. Caltabeloti F, Monsel A, Arbelot C, Brisson H, Lu Q, Gu WJ, et al. Early fluid loading in acute respiratory distress syndrome with septic shock deteriorates lung aeration without impairing arterial oxygenation: a lung ultrasound observational study. Crit Care. (2014) 18:R91. doi: 10.1186/cc13859
41. Lashin H, Shepherd S, Smith A. Contrast-enhanced echocardiography application in patients supported by extracorporeal membrane oxygenation (ECMO): a narrative review. J Cardiothorac Vasc Anesth. (2021). doi: 10.1053/j.jvca.2021.04.031. [Epub ahead of print].
42. Corradi F, Vetrugno L, Orso D, Bove T, Schreiber A, Boero E, et al. Diaphragmatic thickening fraction as a potential predictor of response to continuous positive airway pressure ventilation in Covid-19 pneumonia: a single-center pilot study. Respir Physiol Neurobiol. (2021) 284:103585. doi: 10.1016/j.resp.2020.103585
43. Dres M, Goligher EC, Heunks LMA, Brochard LJ. Critical illness-associated diaphragm weakness. Intens Care Med. (2017) 43:1441–52. doi: 10.1007/s00134-017-4928-4
44. Li C, Li X, Han H, Cui H, Wang G, Wang Z. Diaphragmatic ultrasonography for predicting ventilator weaning: a meta-analysis. Medicine. (2018) 97:e10968. doi: 10.1097/MD.0000000000010968
45. Ferré A, Guillot M, Lichtenstein D, Mezière G, Richard C, Teboul JL, et al. Lung ultrasound allows the diagnosis of weaning-induced pulmonary oedema. Intens Care Med. (2019) 45:601–8. doi: 10.1007/s00134-019-05573-6
46. Soummer A, Perbet S, Brisson H, Arbelot C, Constantin JM, Lu Q, et al. Ultrasound assessment of lung aeration loss during a successful weaning trial predicts postextubation distress*. Crit Care Med. (2012) 40:2064–72. doi: 10.1097/CCM.0b013e31824e68ae
47. Bouhemad B, Mojoli F, Nowobilski N, Hussain A, Rouquette I, Guinot PG, et al. Use of combined cardiac and lung ultrasound to predict weaning failure in elderly, high-risk cardiac patients: a pilot study. Intens Care Med. (2020) 46:475–84. doi: 10.1007/s00134-019-05902-9
48. Corradi F, Brusasco C, Vezzani A, Santori G, Manca T, Ball L, et al. Computer-aided quantitative ultrasonography for detection of pulmonary edema in mechanically ventilated cardiac surgery patients. Chest. (2016) 150:640–51. doi: 10.1016/j.chest.2016.04.013
49. Luecke T, Corradi F, Pelosi P. Lung imaging for titration of mechanical ventilation. Curr Opin Anaesthesiol. (2012) 25:131–40. doi: 10.1097/ACO.0b013e32835003fb
50. Vasquez DG, Berg GM, Srour SG, Ali K. Lung ultrasound for detecting pneumothorax in injured children: preliminary experience at a community-based Level II pediatric trauma center. Pediatr Radiol. (2020) 50:329–37. doi: 10.1007/s00247-019-04509-y
51. Santorelli JE, Chau H, Godat L, Casola G, Doucet JJ, Costantini TW. Not so FAST-Chest ultrasound underdiagnoses traumatic pneumothorax. J Trauma Acute Care Surg. (2022) 92:44–8. doi: 10.1097/TA.0000000000003617
52. Wilson JG, Breyer KE. Critical care ultrasound: a review for practicing nephrologists. Adv Chronic Kidney Dis. (2016) 23:141–5. doi: 10.1053/j.ackd.2016.01.015
53. Karakala N, Córdoba D, Chandrashekar K, Lopez-Ruiz A, Juncos LA. Point-of-care ultrasound in acute care nephrology. Adv Chronic Kidney Dis. (2021) 28:83–90. doi: 10.1053/j.ackd.2021.06.003
54. Gigante A, Barbano B, Di Mario F, Rosato E, Simonelli M, Rocca AR, et al. Renal parenchymal resistance in patients with biopsy proven glomerulonephritis: correlation with histological findings. Int J Immunopathol Pharmacol. (2016) 29:469–74. doi: 10.1177/0394632016645590
55. Parolini C, Noce A, Staffolani E, Giarrizzo GF, Costanzi S, Splendiani G. Renal resistive index and long-term outcome in chronic nephropathies. Radiology. (2009) 252:888–96. doi: 10.1148/radiol.2523080351
56. Corradi F, Via G, Tavazzi G. What's new in ultrasound-based assessment of organ perfusion in the critically ill: expanding the bedside clinical monitoring window for hypoperfusion in shock. Intens Care Med. (2020) 46:775–9. doi: 10.1007/s00134-019-05791-y
57. Beaubien-Souligny W, Denault A, Robillard P, Desjardins G. The role of point-of-care ultrasound monitoring in cardiac surgical patients with acute kidney injury. J Cardiothorac Vasc Anesth. (2019) 33:2781–96. doi: 10.1053/j.jvca.2018.11.002
58. Selby NM, Duranteau J. New imaging techniques in AKI. Curr Opin Crit Care. (2020) 26:543–8. doi: 10.1097/MCC.0000000000000768
59. Perez-Calatayud AA, Carrillo-Esper R, Anica-Malagon ED, Briones-Garduño JC, Arch-Tirado E, Wise R, et al. Point-of-care gastrointestinal and urinary tract sonography in daily evaluation of gastrointestinal dysfunction in critically ill patients (GUTS Protocol). Anaesthesiol Intensive Ther. (2018) 50:40–8. doi: 10.5603/AIT.a2017.0073
60. Wong A, Yusuf GT, Malbrain M. Future developments in the imaging of the gastrointestinal tract: the role of ultrasound. Curr Opin Crit Care. (2021) 27:147–56. doi: 10.1097/MCC.0000000000000815
61. Gao T, Cheng MH Xi FC, Chen Y, Cao C, Su T, et al. Predictive value of transabdominal intestinal sonography in critically ill patients: a prospective observational study. Crit Care. (2019) 23:378. doi: 10.1186/s13054-019-2645-9
62. Wang X, Liu D. Hemodynamic influences on mesenteric blood flow in shock conditions. Am J Med Sci. (2021) 362:243–51. doi: 10.1016/j.amjms.2021.04.014
63. Hollerweger A, Maconi G, Ripolles T, Nylund K, Higginson A, Serra C, et al. Gastrointestinal ultrasound (GIUS) in intestinal emergencies - An EFSUMB position paper. Ultraschall Med. (2020) 41:646–57. doi: 10.1055/a-1147-1295
64. Cardim D, Robba C, Bohdanowicz M, Donnelly J, Cabella B, Liu X, et al. Non-invasive monitoring of intracranial pressure using transcranial doppler ultrasonography: is it possible? Neurocrit Care. (2016) 25:473–91. doi: 10.1007/s12028-016-0258-6
65. Robba C, Goffi A, Geeraerts T, Cardim D, Via G, Czosnyka M, et al. Brain ultrasonography: methodology, basic and advanced principles and clinical applications. A narrative review. Intens Care Med. (2019) 45:913–27. doi: 10.1007/s00134-019-05610-4
66. Lochner P, Czosnyka M, Naldi A, Lyros E, Pelosi P, Mathur S, et al. Optic nerve sheath diameter: present and future perspectives for neurologists and critical care physicians. Neurol Sci. (2019) 40:2447–57. doi: 10.1007/s10072-019-04015-x
67. Liu D, Li Z, Zhang X, Zhao L, Jia J, Sun F, et al. Assessment of intracranial pressure with ultrasonographic retrobulbar optic nerve sheath diameter measurement. BMC Neurol. (2017) 17:188. doi: 10.1186/s12883-017-0964-5
68. Corradi F, Robba C, Tavazzi G, Via G. Combined lung and brain ultrasonography for an individualized “brain-protective ventilation strategy” in neurocritical care patients with challenging ventilation needs. Crit Ultrasound J. (2018) 10:24. doi: 10.1186/s13089-018-0105-4
69. Salerno A, Tupchong K, Verceles AC, McCurdy MT. Point-of-care teleultrasound: a systematic review. Telemed J E Health. (2020) 26:1314–21. doi: 10.1089/tmj.2019.0177
70. Vranas KC, Slatore CG, Kerlin MP. Telemedicine coverage of intensive care units: a narrative review. Ann Am Thorac Soc. (2018) 15:1256–64. doi: 10.1513/AnnalsATS.201804-225CME
71. Noritomi DT, Ranzani OT, Ferraz LJR, Dos Santos MC, Cordioli E, Albaladejo R, et al. TELE-critical Care verSus usual Care On ICU PErformance (TELESCOPE): protocol for a cluster-randomised clinical trial on adult general ICUs in Brazil. BMJ Open. (2021) 11:e042302. doi: 10.1136/bmjopen-2020-042302
72. Brusasco C, Santori G, Bruzzo E, Trò R, Robba C, Tavazzi G, et al. Quantitative lung ultrasonography: a putative new algorithm for automatic detection and quantification of B-lines. Crit Care. (2019) 23:288. doi: 10.1186/s13054-019-2569-4
73. Mongodi S, De Luca D, Colombo A, Stella A, Santangelo E, Corradi F, et al. Quantitative lung ultrasound: technical aspects and clinical applications. Anesthesiology. (2021) 134:949–65. doi: 10.1097/ALN.0000000000003757
74. Blaivas M, Arntfield R, White M, DIY AI. deep learning network development for automated image classification in a point-of-care ultrasound quality assurance program. J Am Coll Emerg Physicians Open. (2020) 1:124–31. doi: 10.1002/emp2.12018
75. Duan S, Liu L, Chen Y, Yang L, Zhang Y, Wang S, et al. A 5G-powered robot-assisted teleultrasound diagnostic system in an intensive care unit. Crit Care. (2021) 25:134. doi: 10.1186/s13054-021-03563-z
Keywords: point-of-care ultrasonography, visual round, intensive care unit, multiorgan, clinical practice
Citation: Mao J-Y, Zhang H-M, Liu D-W and Wang X-T (2022) Visual Rounds Based on Multiorgan Point-of-Care Ultrasound in the ICU. Front. Med. 9:869958. doi: 10.3389/fmed.2022.869958
Received: 05 February 2022; Accepted: 15 April 2022;
Published: 25 May 2022.
Edited by:
Claudio Tana, SS Annunziata Polyclinic Hospital, ItalyReviewed by:
Huiqing Ge, Sir Run Run Shaw Hospital, ChinaFrancesco Forfori, University of Pisa, Italy
Copyright © 2022 Mao, Zhang, Liu and Wang. This is an open-access article distributed under the terms of the Creative Commons Attribution License (CC BY). The use, distribution or reproduction in other forums is permitted, provided the original author(s) and the copyright owner(s) are credited and that the original publication in this journal is cited, in accordance with accepted academic practice. No use, distribution or reproduction is permitted which does not comply with these terms.
*Correspondence: Xiao-Ting Wang, aWN1dGluZ0AxNjMuY29t