- 1Malaria Branch, Division of Parasitic Diseases and Malaria, Centers for Disease Control and Prevention, Atlanta, GA, United States
- 2National Malaria Control Program, Luanda, Angola
- 3Burnet Institute, Melbourne, VIC, Australia
- 4Central Clinical School, Monash University, Melbourne, VIC, Australia
- 5Department of Medicine, University of Melbourne, Melbourne, VIC, Australia
- 6London School of Hygiene & Tropical Medicine, London, United Kingdom
- 7U.S. President’s Malaria Initiative, Centers for Disease Control and Prevention, Atlanta, GA, United States
Immunoglobulin (Ig) production during and after infection with Plasmodium parasites is one of the greatest adaptive immune defenses the human host has against this parasite. Infection with P. falciparum has been shown to induce different B cell maturation responses dependent upon the age of the patient, number of previous exposures, and severity of the disease. Described here are dynamics of Ig responses to a panel of 32 P. falciparum antigens by patients followed for 42 days and classified individuals as showing characteristics of an apparent first P. falciparum infection (naïve) or a repeat exposure (non-naïve). Six parameters were modeled to characterize the dynamics of IgM, IgG1, IgG3, and IgA for these two exposure groups with differences assessed among Ig isotypes/subclasses and unique antigens. Naïve patients had significantly longer periods of time to reach peak Ig titer (range 4–7 days longer) and lower maximum Ig titers when compared with non-naïve patients. Modeled time to seronegativity was significantly higher in non-naïve patients for IgM and IgA, but not for the two IgG subclasses. IgG1 responses to Rh2030, HSP40, and PfAMA1 were at the highest levels for non-naïve participants and may be used to predict previous or nascent exposure by themselves. The analyses presented here demonstrate the differences in the development of the Ig response to P. falciparum if the infection represents a boosting response or a primary exposure. Consistency in Ig isotype/subclasses estimates and specific data for P. falciparum antigens can better guide interpretation of seroepidemiological data among symptomatic persons.
Introduction
The human host mounts a vigorous adaptive immune response to the Plasmodium falciparum parasite, and B cell responses through antibody-mediated immunity have been shown to be protective against malaria (1), and even passive transfer of serum antibodies from persons living in endemic settings reduces P. falciparum parasite burden of symptomatic children (2). As malaria infection is a bloodborne infectious disease, anti-P. falciparum immunoglobulin (Ig) titers are generally highest for IgG in humans upon natural exposure, though both IgM and IgA antibodies are observed in substantial quantities as well (3–5). The IgG response to P. falciparum can be further subdivided by the four subclasses of this isotype in humans, with highest serum levels of IgG1 followed by IgG3, IgG4, and IgG2 (6–9); however, the relative abundance of IgG subclass response varies for different malaria antigens. IgG1 and IgG3 are generally the predominant response and effectively mediate interactions with complement and Fcγ-receptors expressed on immune cells, which play roles in immunity (10, 11). Understanding the induction, function, and dynamics of the Ig response against P. falciparum antigens has greatly enhanced vaccine development (12–14) and interpretation of seroepidemiological studies (15, 16).
In P. falciparum-endemic areas throughout the world, infants or children may become exposed to this parasite at a very early age. Passive placental transfer of IgG to the fetus provides a degree of clinical protection early in life (17), though these antibodies are generally lost by 6 months of age (18, 19). Formation and maturation of the host anti-P. falciparum B cell response is an area of active research for several decades, though human studies have obvious limitations due to the inherent need for immediate treatment when any infection is diagnosed. The response in humans appears to follow many of the classical assumptions regarding class switching, affinity maturation, and clonal selection, though recent work has emphasized the contribution of atypical B cell populations early in development which appear be able to respond faster to antigen challenge, but are less efficient at establishing protective and long-term antibody production (20–22). Evidence has been presented showing the early B activation in response to P. falciparum in naïve humans to be dominated by short-lived and metabolically-active plasmablasts which have the capacity for prolific antibody secretion, but may inhibit the formation of durable immunity (23). Additionally, as with B cell maturation to many other immunogenic agents, the importance of CD4 + T follicular helper cells has been documented for the development of the P. falciparum antibody response with T helper (Th) cell Th1 and Th2 subsets likely playing distinct roles (24, 25). Recent studies have also highlighted the prominence of IgM responses to malaria infection, including repeat infections and the persistence of IgM responses over time (26, 27).
Individual P. falciparum antigens have been identified for their specific abilities to induce B cell responses and antibody production in exposed endemic populations (16, 28–31), as well as controlled human malaria infections (CHMIs) (24, 26, 32, 33). The study presented here investigates the short-term immunoglobulin response to natural P. falciparum infection by categorizing a study population of children into first or repeat infection and comparing Ig responses for 42 days following antimalarial treatment. This study aims to understand the Ig dynamics arising from a B cell response in the nascent host vs. a host with previous immunological memory. Ig responses were broadly investigated in a population of children against a panel of 32 P. falciparum antigens encompassing all life stages in the human host was investigated for the ability to bind IgM, IgG1, IgG3, and IgA in blood samples in order to obtain detail on the specificity and nature of immune responses. These data are used to estimate the nascent B cell response to P. falciparum exposure through the dynamics of Ig production to multiple parasite antigens, and how this differs from individuals experiencing a repeat P. falciparum infection.
Materials and methods
Study design
Dried blood spots were collected during a therapeutic efficacy monitoring study (TES) in Angola in 2017 (34). Samples from all three sentinel TES sites were included: high-transmission M’Banza Congo, Zaire Province and Saurimo, Lunda Sul Province; and low\mid-transmission Benguela, Benguela Province. Due to different transmission levels, the parasite density criteria for enrollment were lower in Benguela province (1,000–100,000 p/μL blood) vs. Lunda Sul and Zaire (2,000–200,000 p/μL blood). Children aged 6 months to 11 years old with microscopically confirmed acute P. falciparum infection were treated with one of three artemisinin-based combination therapies (ACT) and followed weekly for 28 (participants treated with artemether-lumefantrine or artesunate-amodiaquine) or 42 (participants treated with dihydroartemisinin-piperaquine) days. Patients with severe or complicated malaria infections were excluded from enrollment. Participant samples were collected on Days 0 (enrollment), 2, 3, 7, 14, 21, 28, 35, and 42 after initiation of ACT.
Ethics approval
Study participants consented to collection of malaria data from provided blood samples. The study received human subjects approval from the Angolan Ministry of Health. Secondary analysis of anonymized samples was approved by the office of the Associate Director of Science in the Center for Global Health at the CDC (Project ID: 0900f3eb8193aa9d).
Laboratory analysis
Samples were assayed for antibody responses to a panel of P. falciparum antigens using a multiple bead-based assay as described previously (5). Assay signal was provided as mean fluorescent intensity (MFI) minus the signal from blank wells on each plate to provide a final signal of MFI-bg for analysis.
Statistical analysis
To classify study participants into P. falciparum naïve (first lifetime infection) and non-naïve (repeat infection) categories, the assay signals for baseline (Day 0) samples for IgG1 response to PfMSP1 and PfAMA1 were compared. Natural exposure to PfMSP1 and PfAMA1 is highly immunogenic in humans, and IgG1 responses to these two antigens are long-lived and generally considered to be indicative of any prior exposure to P. falciparum (5, 35–37). MFI-bg seropositivity thresholds for PfMSP1 and PfAMA1 were 115 and 113, respectively, and generated as described previously (5). Children that had IgG1 responses to both the PfMSP1 and PfAMA1 antigens below the seropositivity threshold at Day 0 were considered “naive” and it was assumed that their presenting P. falciparum infection was their first-ever P. falciparum infection. This classification scheme is also supported by the three TES enrollment sites being located in meso- to high-endemic P. falciparum settings in Angola (34), and the relatively young ages of participants, meaning it is unlikely they would have had time for IgG1 seroreversion from a previous P. falciparum exposure. In order to have higher confidence in the classification scheme, children seropositive for PfMSP1 and/or PfAMA1 IgG1 but having assay signal less than one log10 fold greater than the seropositivity threshold (MFI-bg value of 1,150 and 1,130, respectively) were classified as indeterminate and excluded from further analysis. All other children with high IgG1 assay signals to these P. falciparum antigens were considered “non-naive” with strong evidence for previous P. falciparum blood-stage infection.
For all antigens included in the panel, individual decay curves were characterized with six key parameters: Cmax, the maximum antibody signal; ΔC, the difference between Cmax and the antibody signal at Day 0; Cend, the antibody signal at last day of follow up; tmax, the time in days to maximum antibody signal; t1/2, the post-peak half-life; and tneg, the expected time to seronegativity (5). The distribution of the estimates for these parameters across all antigens was compared between children classified as naive and non-naive. The analysis was separately done for IgG1, IgG3, IgM, and IgA. Though IgG2, IgG4, IgE, and IgD responses were also measured in these same samples, these isotypes/subclasses were either undetectable (at 1:100 serum concentration) or too few children displayed responses for these Igs to allow for parameter estimates (5).
Differences in the empirical distributions were assessed using the Kolmogorov–Smirnov non-parametric test. Heatmaps were generated to simultaneously assess clustering patterns of antibody responses by antigen and participant. All analysis was done in R version 3.6.0 (R Foundation for Statistical Computing, Vienna, Austria).
Results
Classification of study population into Plasmodium falciparum naïve and non-naïve
Of 104 enrolled participants, 89 (85.6%) provided samples for the entire 42-day follow-up period (Days 0, 2, 3, 7, 14, 21, 28, 35, and 42 after enrollment), while 2 (1.9%) provided samples only up to 35 days, 1 (1.0%) to 28 days, 6 (5.8%) to 21 days, and 6 (5.8%) to 14 days. As described and listed previously, a total of 32 P. falciparum antigens were utilized for the multiplex antibody detection assay (5). The distribution of IgG1 responses to PfMSP1 and PfAMA1 for the entire study population were bimodal at Day 0, and by the end of follow-up sampling, nearly all participants registered high responses to both of these antigens (Figure 1). A similar pattern was observed for the IgG3 response to both antigens, but the distributions were not as distinct for IgM and IgA antibodies (Supplementary Figure 1). As described in “Materials and methods,” the bivariate correlation for participant IgG1 responses to PfMSP1 and PfAMA1 at day of enrollment (Day 0) was considered in order to classify participants as previously exposed to P. falciparum (participant was non-naïve), or if the current infection represented potential first P. falciparum exposure (participant was naïve). Using the PfMSP1 and PfAMA1 assay signal thresholds for IgG1, of the 104 participants, 26 (25%) were classified as naïve (seronegative to both antigens), 66 (63%) as non-naïve (high levels of IgG1 to either), and 12 as indeterminate (seropositive to either, but of lower assay signal) (Figure 2). Of the 66 persons classified as non-naive, 64 (97.0%) were seropositive to both of these antigens. For the remaining two individuals, one was IgG1 seropositive to PfMSP1 only, and one was seropositive to PfAMA1 only. Participants classified as exposure naïve were on average 1.2 years younger than non-naive participants (mean age 2.3 years vs. 3.5, Student’s t-test p-value 0.001). Parasite density at Day 0 and sex were not statistically different between the two classification groups (Table 1).
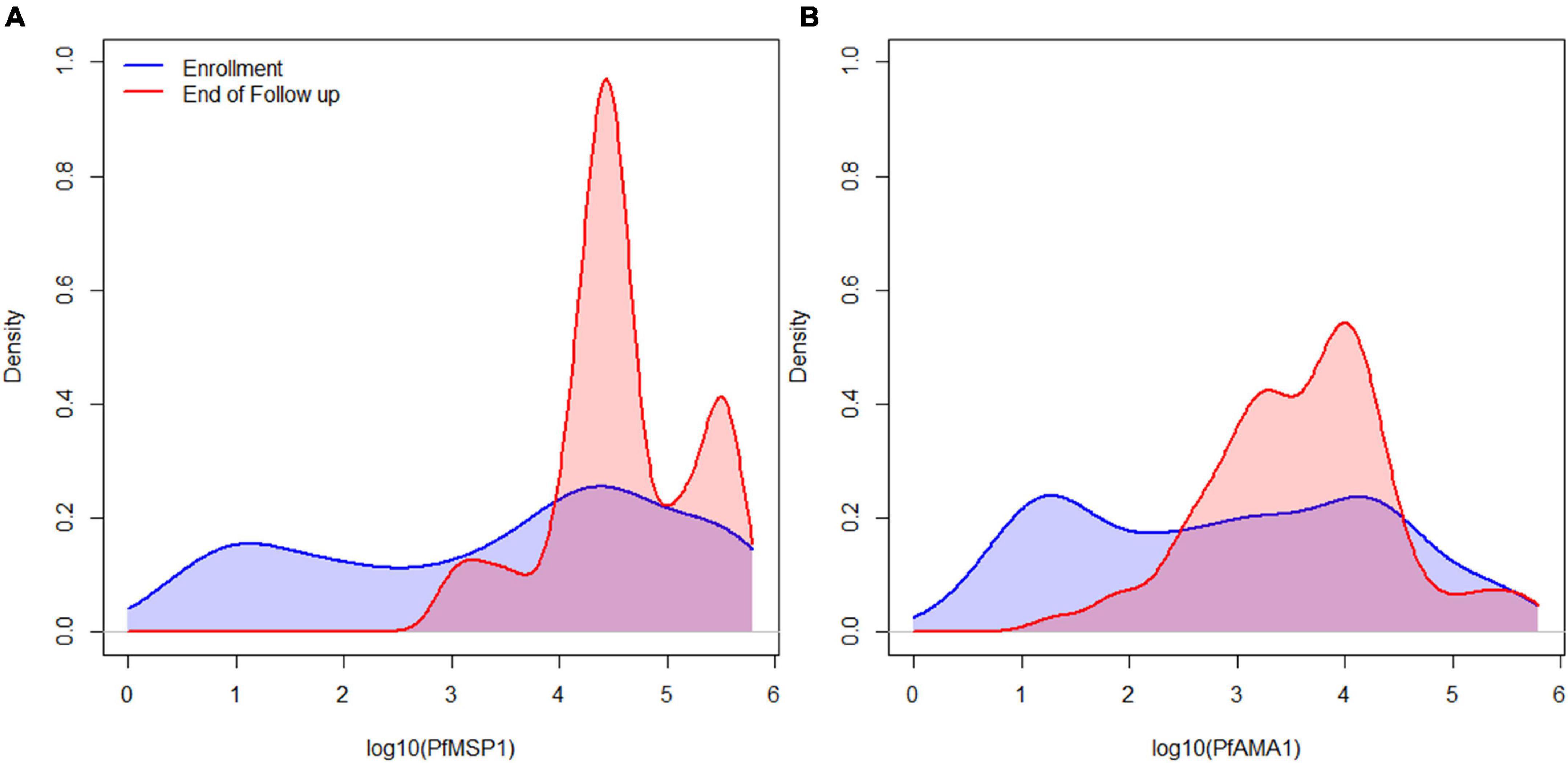
Figure 1. IgG1 antibody levels for all participants for the immunogenic P. falciparum PfMSP1 and PfAMA1 antigens. Smoothed distribution of IgG1 antibody responses to PfMSP1 (A) and PfAMA1 (B) at enrollment (baseline) in blue, and last day of follow-up in red for children treated for P. falciparum infection.
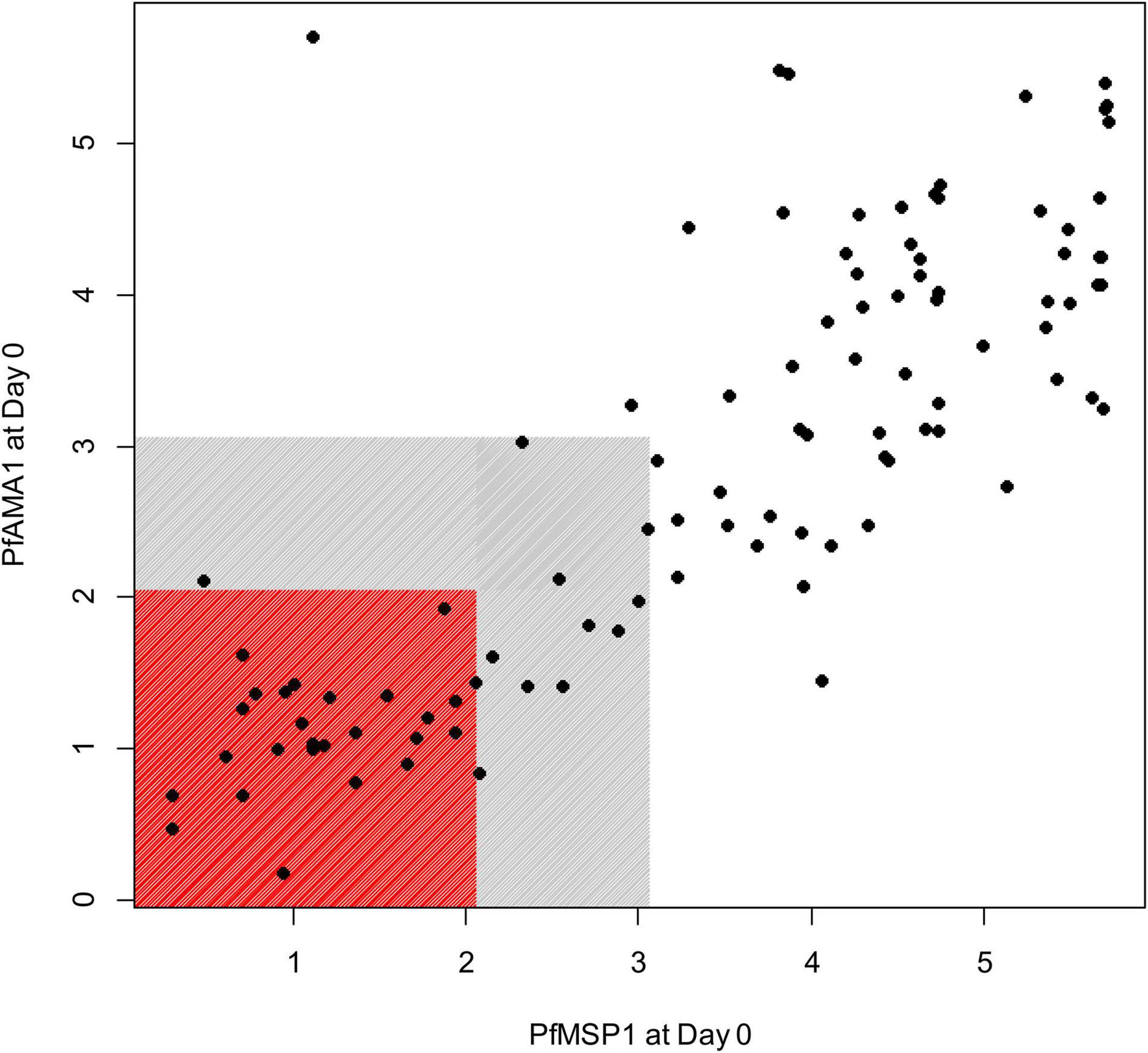
Figure 2. Relationship of the IgG1 antibody responses to PfMSP1 and PfAMA1 at the first day of follow-up (Day 0) in children treated for P. falciparum infection. Axes display assay log10-transformed assay signal to the two respective P. falciparum antigens. Children IgG1 seronegative for both antigens are shown by markers in the red box, and children nominally IgG1 seropositive to either (or both) antigens shown in gray box. Children seronegative to both targets are classified as P. falciparum naïve, and children with assay signals greater than the gray shading classified as non-naïve.
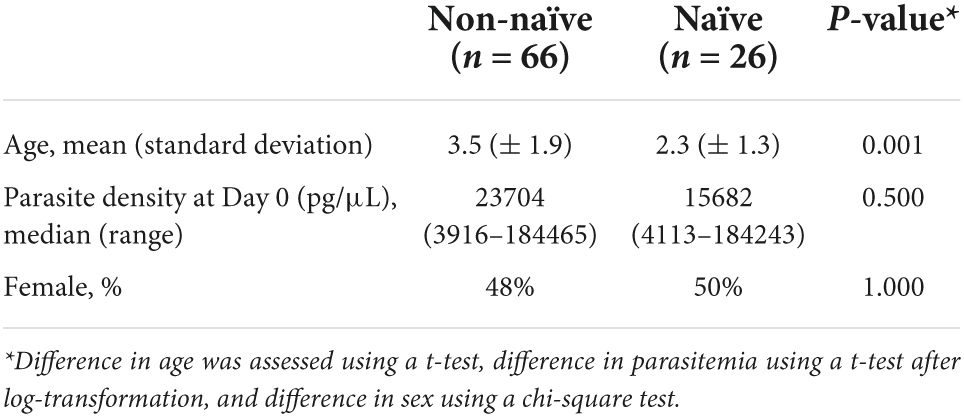
Table 1. Characteristics of children with P. falciparum infection stratified into exposure naïve and exposure non-naïve.
Differences in parameter estimates by Ig isotype and subclass
When stratifying by Ig isotype and subclass for IgG1, IgG3, IgM, and IgA, considerable differences were observed in many of the six parameters used to describe Ig dynamics between the naïve and non-naïve groups for the aggregate responses to the 32 P. falciparum antigens (Figure 3). For IgG1, five of the six parameters showed statistically significant differences between the naïve and non-naïve groups (Table 2). Of particular note was the difference in IgG1 Cmax (MFI-bg of 407 for non-naïve vs. 117 for naïve) and in tmax, which was 7 days later for the naïve IgG1 response. Aggregate estimates for three of the five parameters for dynamics in IgG3 response were significantly different with Cmax again higher for non-naives, and tmax again 1 week later for naives. For IgM, five of the six parameters showed significant differences in aggregate estimates, and for IgA, all six parameters were significantly different between the two groups. When considering the six parameter estimates for all malaria antigens and Ig isotypes together, naïve participants had significantly lower maximum antibody responses (Cmax), higher absolute changes in antibody response (ΔC), took longer to reach maximum antibody response (tmax), and were seropositive for a shorter period of time (tneg) compared to non-naive participants (p-values < 0.001) (Supplementary Figure 2).
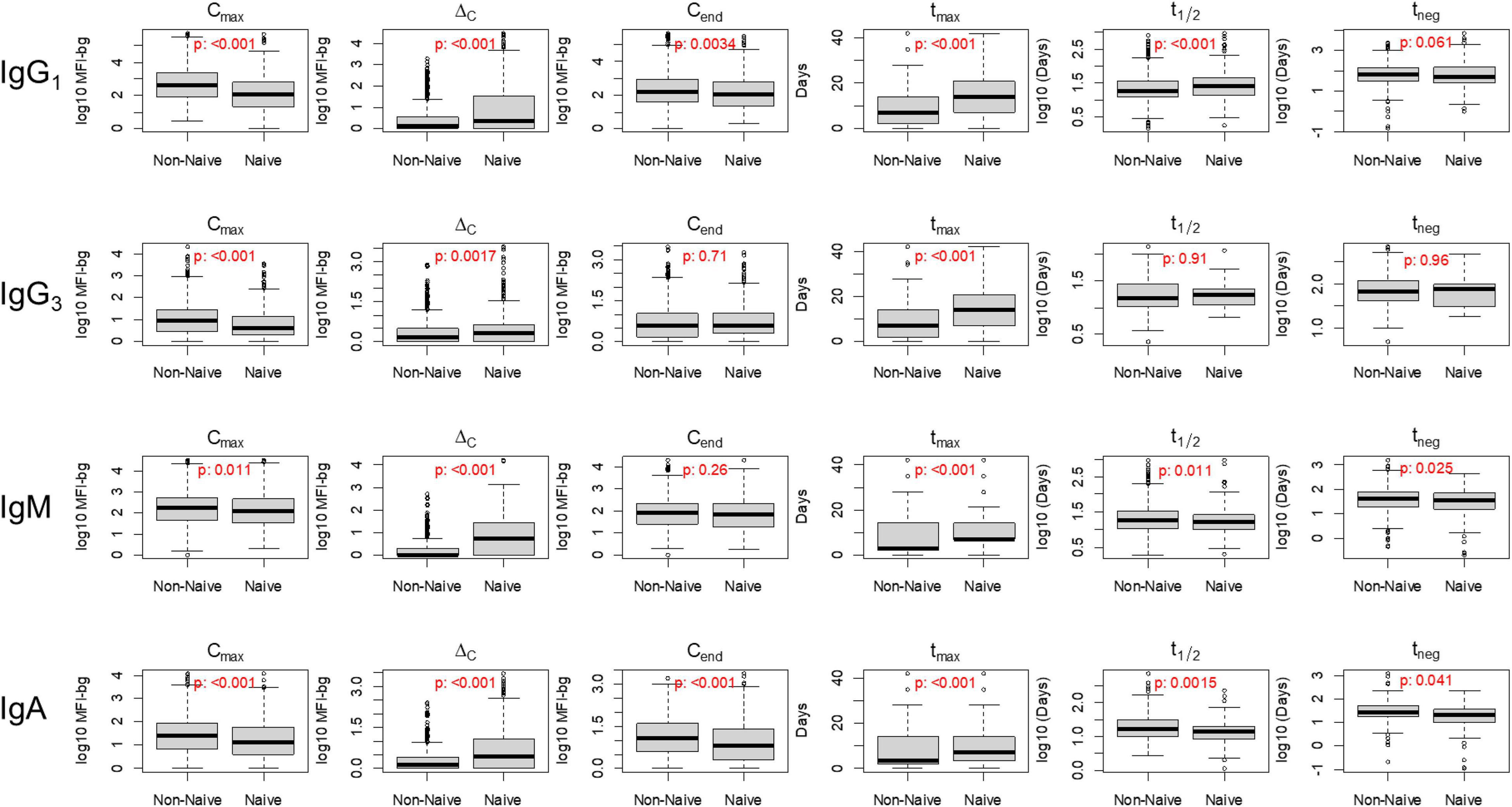
Figure 3. Distribution of six key post-treatment clearance parameters in non-naïve and naïve Angolan children treated for malaria, stratifying by immunoglobulin (Ig) class/subclass. P-value for Kolmogorov–Smirnov test for difference in empiric distribution between non-naïve and naïve participants. Mean values between non-naïve and naïve categories are displayed in Table 2.
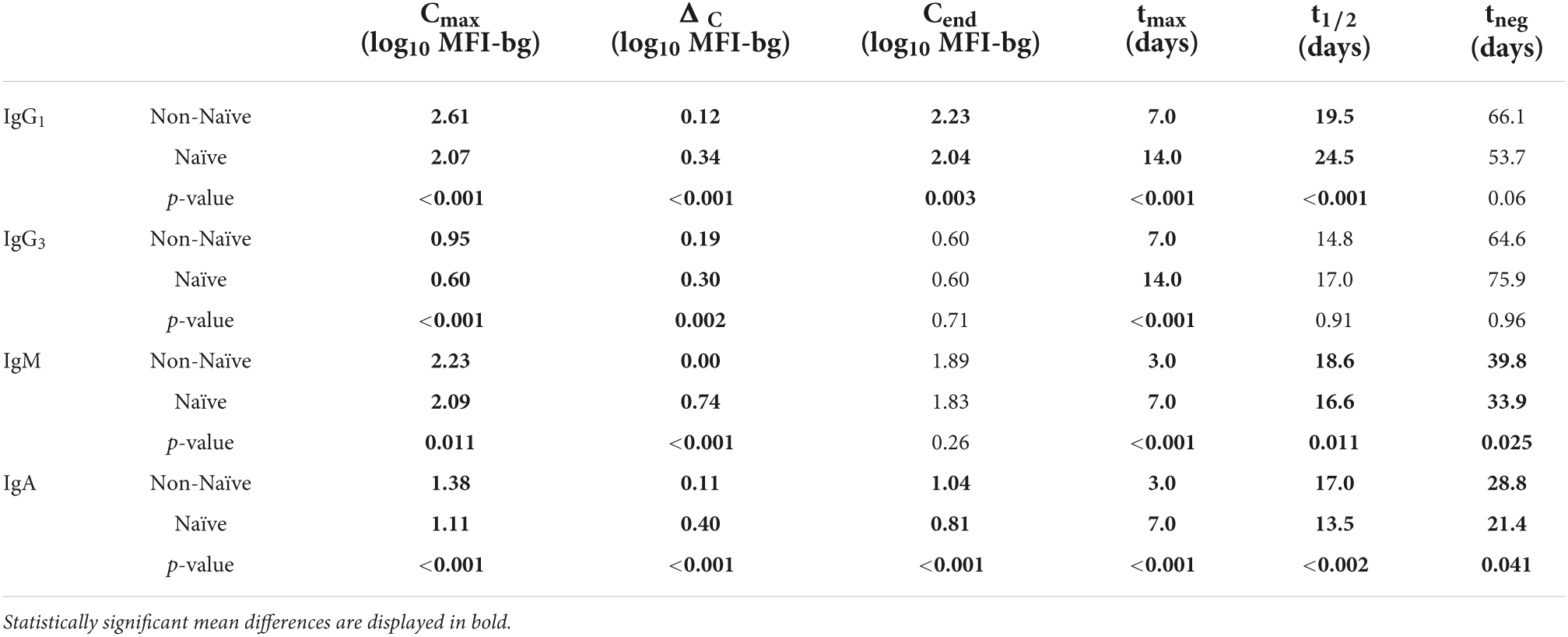
Table 2. Mean values for each parameter by immunoglobulin isotype/subclass as aggregate for all P. falciparum antigen responses estimated for the naïve and non-naïve children.
Differences in specific Plasmodium falciparum antigen Ig responses by participant classification
When assessing differences in Ig dynamics parameter estimates for individual P. falciparum antigens, some general trends were observed, but with variability within each isotype/subclass. Certain antigens were particularly discriminatory between naive and non-naive participants. For example, HSP40, Rh2030 and PfAMA1 tended to have a substantially higher Cmax and Cend in non-naive than naive participants for the IgG1 response (Figure 4 and Supplementary Figure 3). All three of these antigens also stimulated significantly higher IgA responses on the last day of follow-up in non-naive vs. naive participants. Interestingly, Cmax for the anti-PfMSP1 IgM response was substantially higher in naïve participants, which translated to significantly higher ΔC and Cend estimates in naïve persons. Estimates for tmax, t1/2, and tneg yielded some striking findings for individual antigens: tmax 21 days longer for MSP2_Dd2 for IgG1 in naïve persons, t1/2 30 days longer for Rh_2030 for IgG1 in naïve persons, t1/2 15 days longer for Etramp5 Ag1 for IgG3 in naïve persons, and t1/2 significantly longer for IgM (25 days) and IgA (39 days) against PfMSP1 in non-naïve persons. In time to apex antibody levels (tmax), all isotypes showed the predominance of naïve children taking longer to reach this apex with statistically significant differences for 13/32 (40.6%) of antigens for IgG1 detection, 7/32 (21.9%) of antigens for IgG3, 11/32 (34.4%) for IgM, and 8/32 antigens (25.0%) for IgA. Antibodies against the PfMSP1, PfAMA1, GLURP Ro, Etramp4Ag2, and Etramp5Ag1 antigens were all significantly delayed in reaching apex levels in naïve children for all four Ig isotypes/sub-classes.
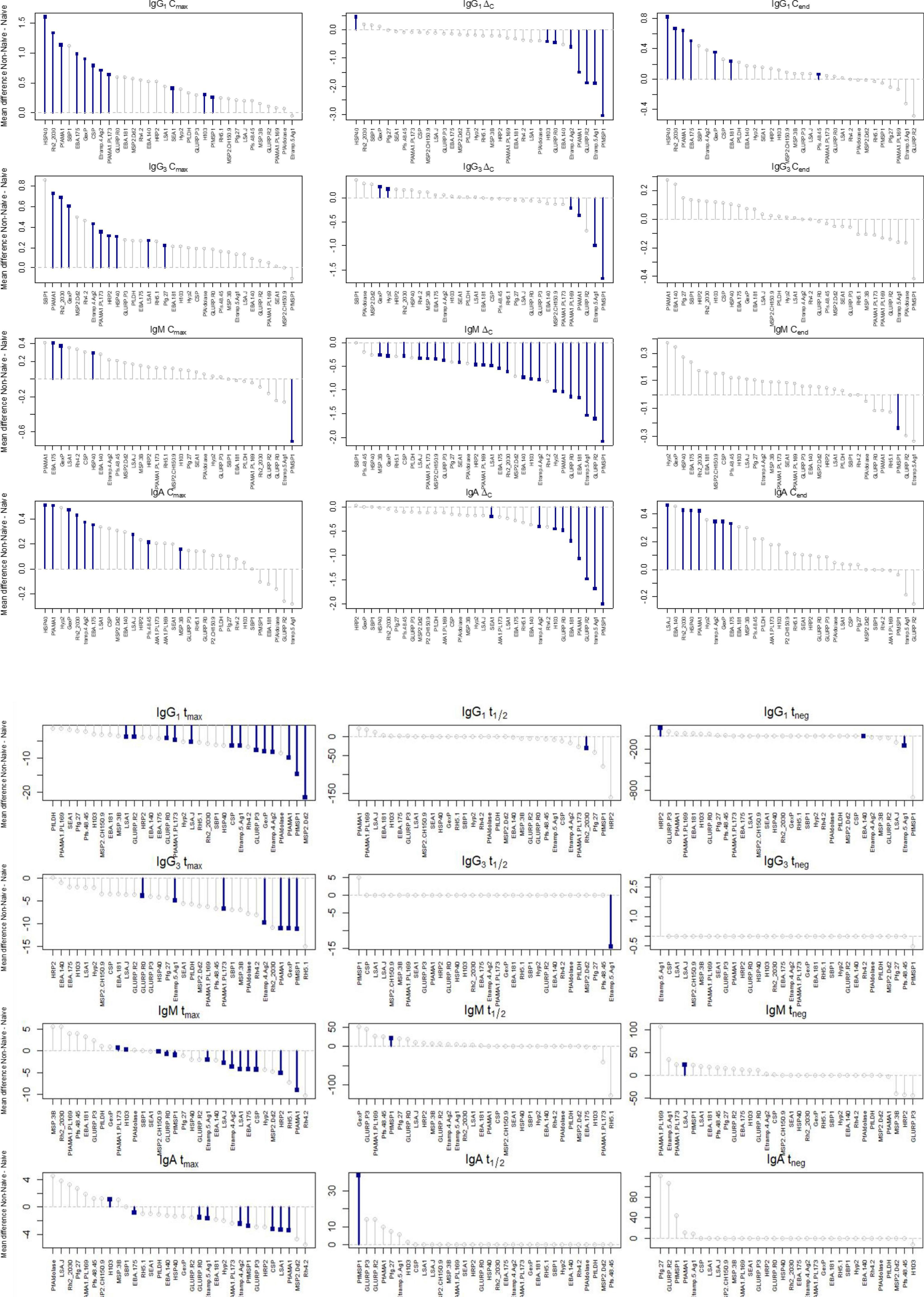
Figure 4. Mean differences for each parameter by Ig response for non-naïve and naïve children followed post-treatment. Positive values represent higher values in non-naïves, and lines with blue squares denote statistically significant differences.
Hierarchical clustering based on Ig response parameters
Estimates among the panel of P. falciparum antigens for ΔC for IgG1 and IgM were sufficiently consistent to allow clustering based on naïve or non-naïve classification (Figure 5). Clustering based on IgG1 ΔC was largely driven by higher estimates in the naïves for PfMSP1, PfAMA1, and Etramp5Ag1, and lower estimates in Rh_2030, HSP40, HRP2, Etramp4Ag2, and the three EBA antigens. Clustering based on IgM ΔC was largely driven by the higher estimates in naïves for PfMSP1, GLURP Ro, Etramp5Ag1, and PfAMA1. For children classified as indeterminate (neither naïve or non-naïve), the IgG1 ΔC estimates among antigens did not cluster with either naïve/non-naïve categories, though the IgM ΔC estimates were more similar with the children classified as naïve.
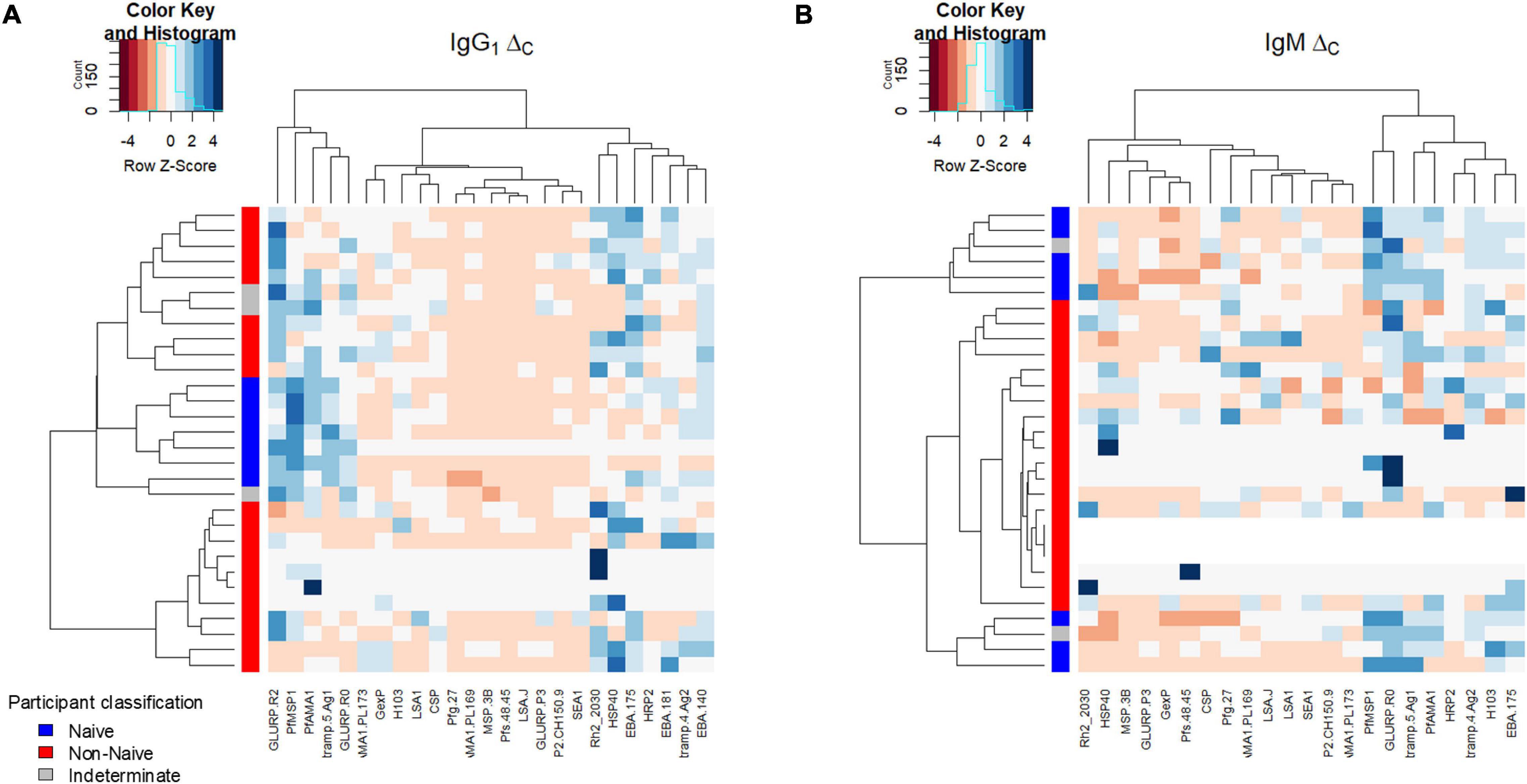
Figure 5. Hierarchical relationship among study participants shown for the ΔC parameter. Clustering of ΔC by P. falciparum antigen response (columns) and participants (rows) for IgG1 (A) and IgM (B). Intensity of color shading within the plot indicated by the magnitude of the standard score (Z score).
Discussion
Here is described the quantitative comparison of six parameters describing Ig dynamics upon successful treatment of P. falciparum infection when study participants were classified as previously exposed to P. falciparum or if the current P. falciparum infection was their first exposure. Infection with P. falciparum parasites is known to induce a robust IgG response in humans with IgG1 levels showing the highest titers followed by IgG3, IgG4, and IgG2 (6–9). Multiple individual P. falciparum antigens have been identified as immunogens in the human host and utilized in candidate malaria vaccine development, association with clinical disease, or seroepidemiological studies (15, 16, 26, 38, 39). Previous work has estimated that upon natural exposure to P. falciparum and generation of IgG1 antibodies, children would remain seropositive to this subclass for an estimated 408 days for PfMSP1 and 153 days for PfAMA1 (5). Studies by others evaluating all age ranges (likely from persons with multiple past infections) have also confirmed this longevity of IgG to these two antigens with estimates for IgG half-life in the host of years to decades (40, 41). Ultimately, quantitative empirical estimates for an individual’s retention of IgG antibodies against any P. falciparum antigen would be a factor of numerous immunological and parasitological factors, so broad assumptions could not be made for a human population. Interestingly, though known to both be among the longer-lasting anti-Plasmodium antibodies, IgG levels to the PfMSP1 and PfAMA1 antigens show low correlation in individuals (42), and it has been hypothesized that different factors control antibody responses to these antigens (43). Based on this information from previous studies, binary classification for this study population to estimate P. falciparum exposure appeared to be appropriate based on IgG1 responses to both of these antigens. To reduce classification error, an additional margin was added to the binary categorization, so only those children with much higher IgG1 levels (well beyond the seropositivity threshold) were considered as non-naïve for P. falciparum. This classification scheme is also supported by the three TES enrollment sites being located in meso- to high-endemic P. falciparum settings in Angola (34), and the relatively young ages of participants (6 months to 11 years old), meaning it is unlikely they would have had enough years of life for IgG1 seroreversion from a previous P. falciparum exposure. A previous report has also shown rapid acquisition of total IgG against P. falciparum antigens with time spent in Angola (44), indicating the high endemic nature of P. falciparum in this setting.
Between these two classification groups, no significant difference was noted between the peripheral parasite densities at presentation to the health facility. It may be expected that previous malaria exposure would suppress parasite burden (1, 32), but all children enrolled in this study were symptomatic, so the distributions presented here are not inclusive of lower-density asymptomatic infections in the general population (45, 46). Classification of the study population into naïve and non-naïve categories found many differences that highlighted classical assumptions of human adaptive humoral immunity. Additionally, as only children older than 6 months old were included in this study, any maternal antibodies would have likely been eliminated by this time (18, 19), and this Ig data most certainly represents true host response. The current P. falciparum infection for non-naïve individuals appeared to have served as an antibody boosting event for IgG1, IgG3, IgM, and IgA antibodies when compared to naïve persons, with maximum antibody levels (Cmax) significantly higher for all four of these Igs, though IgM boosting appears to be the most subdued when compared to the other isotypes/subclasses. Similar to IgG boosting seen in humans after successive P. falciparum infections (37, 47), IgG1 antibodies showed the most antigen targets with Cmax values significantly higher (11/32, 34.4% of all antigens) for non-naïve vs. naïve children, though IgG3 and IgA also showed multiple antigen targets (9 each) exhibiting boosting characteristics. Specifically, the IgG1 responses to Rh2030, HSP40, and PfAMA1 were the highest boosted levels for non-naïve participants, and may (collectively or individually) be used to predict previous or nascent exposure. A prior study showed that in previously exposed individuals, the Rh2030 and PfAMA1 responses were highly correlated with each other and predictive of the P. falciparum pre-patent period (32). Recently, IgG sero-responses to all three of these antigens were significantly correlated to asymptomatic infection, whereas responses to the Etramp5Ag1 and PfMSP1 antigen were correlated with clinical disease (48), giving evidence that the non-naïve children in this current study had some form of previous P. falciparum exposure and were boosted during the current infection.
Recent work has expanded on contribution of the IgM response to P. falciparum infection with findings of IgM-positive memory B cell subsets being predominant in children, IgM inhibiting parasite invasion in a complement-dependent manner, and persistence of IgM response to merozoite surface antigen over time (26, 27). Among all four Ig isotypes/subclasses tested here, estimates for change in day of enrollment to peak Ig levels (ΔC) were universally higher for IgM response in the naïve individuals, with 23/32 (71.9%) P. falciparum antigen ΔC responses reaching statistical significance. These data suggest the presence of IgM + memory B cell response in non-naïve children (49), as the IgM response in naïve children is practically non-existent at the day of enrollment and 11/32 (34.4%) of IgM tmax estimates higher in naïves. Induction of IgA during natural (3, 50) and malaria vaccine (51) exposure has been well documented, though it’s unclear if there’s a specific immunological role this isotype plays in response to P. falciparum infection. Previous work to assess the value of IgA in protecting the host against malaria pathogenesis found no significant benefit from anti-PfMSP1 IgA when infecting mice with transgenic P. berghei expressing the PfMSP1 antigen (52). In the same manner as IgM, ΔC estimates for IgA were nearly all higher in naïve children, but more similar to IgG1 and IgG3, Cmax of nearly all antigens were higher in non-naïves. This data shows evidence for IgA boosting upon re-exposure similar to IgG subclasses with PfAMA1, Rh_2030, and HSP40 boosting as providing some of the strongest markers for previous exposure.
A limitation of this study was that presence and magnitude of IgG1 response against two P. falciparum antigens were used as the only proxy for classification of any previous exposure. However, although previous studies from endemic settings have followed up persons and assessed Ig dynamics over long periods of time (8), previous malaria history (if assessed) comes from clinical episodes, and would miss asymptomatic infections. Additionally, infection events from longitudinal studies are typically noted by sparse intervals or clinical episodes, so true P. falciparum exposure could be missed by the sampling design. This current study only measures the absolute level of antibodies binding to specific P. falciparum antigens, and experiments were not performed to measure binding strength among different antigens or the naïve/non-naïve groups. Proportions of B cell subsets were not able to be evaluated, nor was antibody functional activity (inhibition of parasite invasion, complement activation, etc.) assessed. The results presented here are data only from symptomatic Angolan children infected with P. falciparum from Angola, so it is possible that persons of older ages, persons living in different transmission settings, or different host and parasite genotypes would provide different outputs than the ones observed here. More robust statistical methods for looking across data from multiple Ig classes and antigens are also needed to gain a comprehensive understanding of the human B cell response to P. falciparum infection.
Classification of children into P. falciparum naïve and non-naïve categories and assessment of antibody dynamics weeks after resolved infection showed stark differences in Ig levels and temporal trends between these two groups. This data helps to elucidate Ig dynamics in a human population naturally exposed to P. falciparum malaria and provides generalizable results which can better assist in translating findings from seroepidemiological studies. Presentation here of Ig results by individual P. falciparum antigens will also aid future research studies utilizing these specific targets to put serological data into context
Data availability statement
The original contributions presented in this study are included in the article/Supplementary Material, further inquiries can be directed to the corresponding author.
Author contributions
PD and MP designed and coordinated the field study. ER and MP designed the laboratory study, conceptualized the experiments, drafted the manuscript, and performed statistical analyses. ER and DN performed laboratory assays. BW, JB, CD, and KT provided antigens and scientific expertise. All authors reviewed and approved the final version of the manuscript.
Funding
MP was supported by the U.S. President’s Malaria Initiative. JB was supported by a Senior Research Fellowship of the National Health and Medical Research Council of Australia.
Conflict of interest
The authors declare that the research was conducted in the absence of any commercial or financial relationships that could be construed as a potential conflict of interest.
Publisher’s note
All claims expressed in this article are solely those of the authors and do not necessarily represent those of their affiliated organizations, or those of the publisher, the editors and the reviewers. Any product that may be evaluated in this article, or claim that may be made by its manufacturer, is not guaranteed or endorsed by the publisher.
Acknowledgments
We thank Christine Langer and Linda Reiling (Burnet Institute) for their assistance with recombinant protein expression and purification. We thank Jan Pohl at CDC for the assistance with antigen production. We also thank Jing Jin and Simon Draper (University of Oxford, United Kingdom) for the provision of RH5.1 protein.
Supplementary material
The Supplementary Material for this article can be found online at: https://www.frontiersin.org/articles/10.3389/fmed.2022.869028/full#supplementary-material
References
1. Doolan DL, Dobano C, Baird JK. Acquired immunity to malaria. Clin Microbiol Rev. (2009) 22:13–36.
2. Cohen S, Mc GI, Carrington S. Gamma-globulin and acquired immunity to human malaria. Nature. (1961) 192:733–7.
3. Leoratti FM, Durlacher RR, Lacerda MV, Alecrim MG, Ferreira AW, Sanchez MC, et al. Pattern of humoral immune response to Plasmodium falciparum blood stages in individuals presenting different clinical expressions of malaria. Malar J. (2008) 7:186. doi: 10.1186/1475-2875-7-186
4. Ubillos I, Jimenez A, Vidal M, Bowyer PW, Gaur D, Dutta S, et al. Optimization of incubation conditions of Plasmodium falciparum antibody multiplex assays to measure IgG, IgG1-4, IgM and IgE using standard and customized reference pools for sero-epidemiological and vaccine studies. Malar J. (2018) 17:219. doi: 10.1186/s12936-018-2369-3
5. Rogier E, Nace D, Dimbu PR, Wakeman B, Pohl J, Beeson JG, et al. Framework for characterizing longitudinal antibody response in children after plasmodium falciparum infection. Front Immunol. (2021) 12:617951. doi: 10.3389/fimmu.2021.617951
6. Braga EM, Barros RM, Reis TA, Fontes CJ, Morais CG, Martins MS, et al. Association of the IgG response to Plasmodium falciparum merozoite protein (C-terminal 19 kD) with clinical immunity to malaria in the Brazilian Amazon region. Am J Trop Med Hyg. (2002) 66:461–6. doi: 10.4269/ajtmh.2002.66.461
7. Rouhani M, Zakeri S, Mehrizi AA, Djadid ND. Comparative analysis of the profiles of IgG subclass-specific responses to Plasmodium falciparum apical membrane antigen-1 and merozoite surface protein-1 in naturally exposed individuals living in malaria hypoendemic settings, Iran. Malar J. (2015) 14:58. doi: 10.1186/s12936-015-0547-0
8. Ssewanyana I, Rek J, Rodriguez I, Wu L, Arinaitwe E, Nankabirwa JI, et al. Impact of a rapid decline in malaria transmission on antimalarial IgG subclasses and avidity. Front Immunol. (2020) 11:576663. doi: 10.3389/fimmu.2020.576663
9. Dobano C, Quelhas D, Quinto L, Puyol L, Serra-Casas E, Mayor A, et al. Age-dependent IgG subclass responses to Plasmodium falciparum EBA-175 are differentially associated with incidence of malaria in Mozambican children. Clin Vaccine Immunol. (2012) 19:157–66. doi: 10.1128/CVI.05523-11
10. Boyle MJ, Reiling L, Feng G, Langer C, Osier FH, Aspeling-Jones H, et al. Human antibodies fix complement to inhibit Plasmodium falciparum invasion of erythrocytes and are associated with protection against malaria. Immunity. (2015) 42:580–90. doi: 10.1016/j.immuni.2015.02.012
11. Osier FH, Feng G, Boyle MJ, Langer C, Zhou J, Richards JS, et al. Opsonic phagocytosis of Plasmodium falciparum merozoites: mechanism in human immunity and a correlate of protection against malaria. BMC Med. (2014) 12:108. doi: 10.1186/1741-7015-12-108
12. Pholcharee T, Oyen D, Flores-Garcia Y, Gonzalez-Paez G, Han Z, Williams KL, et al. Structural and biophysical correlation of anti-NANP antibodies with in vivo protection against P. falciparum. Nat Commun. (2021) 12:1063. doi: 10.1038/s41467-021-21221-4
13. Livingstone MC, Bitzer AA, Giri A, Luo K, Sankhala RS, Choe M, et al. In vitro and in vivo inhibition of malaria parasite infection by monoclonal antibodies against Plasmodium falciparum circumsporozoite protein (CSP). Sci Rep. (2021) 11:5318.
14. Murugan R, Buchauer L, Triller G, Kreschel C, Costa G, Pidelaserra Marti G, et al. Clonal selection drives protective memory B cell responses in controlled human malaria infection. Sci Immunol. (2018) 3:eaa8029. doi: 10.1126/sciimmunol.aap8029
15. van den Hoogen LL, Stresman G, Presume J, Romilus I, Mondelus G, Elisme T, et al. Selection of antibody responses associated with plasmodium falciparum infections in the context of malaria elimination. Front Immunol. (2020) 11:928. doi: 10.3389/fimmu.2020.00928
16. Helb DA, Tetteh KK, Felgner PL, Skinner J, Hubbard A, Arinaitwe E, et al. Novel serologic biomarkers provide accurate estimates of recent Plasmodium falciparum exposure for individuals and communities. Proc Natl Acad Sci USA. (2015) 112:E4438–47. doi: 10.1073/pnas.1501705112
17. Dobbs KR, Dent AE. Plasmodium malaria and antimalarial antibodies in the first year of life. Parasitology. (2016) 143:129–38.
18. Dent AE, Malhotra I, Wang X, Babineau D, Yeo KT, Anderson T, et al. Contrasting patterns of serologic and functional antibody dynamics to plasmodium falciparum antigens in a Kenyan birth cohort. Clin Vaccine Immunol. (2016) 23:104–16. doi: 10.1128/CVI.00452-15
19. Moussiliou A, Turner L, Cottrell G, Doritchamou J, Gbedande K, Fievet N, et al. Dynamics of PfEMP1 antibody profile from birth to 12 months of age in beninese infants. J Infect Dis. (2020) 221:2010–7. doi: 10.1093/infdis/jiaa043
20. Portugal S, Tipton CM, Sohn H, Kone Y, Wang J, Li S, et al. Malaria-associated atypical memory B cells exhibit markedly reduced B cell receptor signaling and effector function. Elife. (2015) 4:e07218. doi: 10.7554/eLife.07218
21. Sutton HJ, Aye R, Idris AH, Vistein R, Nduati E, Kai O, et al. Atypical B cells are part of an alternative lineage of B cells that participates in responses to vaccination and infection in humans. Cell Rep. (2021) 34:108684. doi: 10.1016/j.celrep.2020.108684
22. Pérez-Mazliah D, Gardner PJ, Schweighoffer E, McLaughlin S, Hosking C, Tumwine I, et al. Plasmodium-specific atypical memory B cells are short-lived activated B cells. Elife. (2018) 7:e39800. doi: 10.7554/eLife.39800
23. Vijay R, Guthmiller JJ, Sturtz AJ, Surette FA, Rogers KJ, Sompallae RR, et al. Infection-induced plasmablasts are a nutrient sink that impairs humoral immunity to malaria. Nat Immunol. (2020) 21:790–801. doi: 10.1038/s41590-020-0678-5
24. Chan JA, Loughland JR, de Labastida Rivera F, SheelaNair A, Andrew DW, Dooley NL, et al. Th2-like T follicular helper cells promote functional antibody production during plasmodium falciparum infection. Cell Rep Med. (2020) 1:100157. doi: 10.1016/j.xcrm.2020.100157
25. Obeng-Adjei N, Portugal S, Tran TM, Yazew TB, Skinner J, Li S, et al. Circulating Th1-Cell-type Tfh Cells that exhibit impaired B cell help are preferentially activated during acute malaria in children. Cell Rep. (2015) 13:425–39. doi: 10.1016/j.celrep.2015.09.004
26. Boyle MJ, Chan JA, Handayuni I, Reiling L, Feng G, Hilton A, et al. IgM in human immunity to Plasmodium falciparum malaria. Sci Adv. (2019) 5:eaax4489.
27. Hopp CS, Sekar P, Diouf A, Miura K, Boswell K, Skinner J, et al. Plasmodium falciparum-specific IgM B cells dominate in children, expand with malaria, and produce functional IgM. J Exp Med. (2021) 218:e20200901. doi: 10.1084/jem.20200901
28. Fonseca AM, Quinto L, Jiménez A, González R, Bardají A, Maculuve S, et al. Multiplexing detection of IgG against Plasmodium falciparum pregnancy-specific antigens. PLoS One. (2017) 12:e0181150. doi: 10.1371/journal.pone.0181150
29. Kerkhof K, Canier L, Kim S, Heng S, Sochantha T, Sovannaroth S, et al. Implementation and application of a multiplex assay to detect malaria-specific antibodies: a promising tool for assessing malaria transmission in Southeast Asian pre-elimination areas. Malar J. (2015) 14:338. doi: 10.1186/s12936-015-0868-z
30. Richards JS, Arumugam TU, Reiling L, Healer J, Hodder AN, Fowkes FJ, et al. Identification and prioritization of merozoite antigens as targets of protective human immunity to Plasmodium falciparum malaria for vaccine and biomarker development. J Immunol. (2013) 191:795–809. doi: 10.4049/jimmunol.1300778
31. Crompton PD, Kayala MA, Traore B, Kayentao K, Ongoiba A, Weiss GE, et al. A prospective analysis of the Ab response to Plasmodium falciparum before and after a malaria season by protein microarray. Proc Natl Acad Sci USA. (2010) 107:6958–63. doi: 10.1073/pnas.1001323107
32. Achan J, Reuling IJ, Yap XZ, Dabira E, Ahmad A, Cox M, et al. Serologic markers of previous malaria exposure and functional antibodies inhibiting parasite growth are associated with parasite kinetics following a plasmodium falciparum controlled human infection. Clin Infect Dis. (2020) 70:2544–52. doi: 10.1093/cid/ciz740
33. van den Hoogen LL, Walk J, Oulton T, Reuling IJ, Reiling L, Beeson JG, et al. Antibody responses to antigenic targets of recent exposure are associated with low-density parasitemia in controlled human plasmodium falciparum infections. Front Microbiol. (2018) 9:3300. doi: 10.3389/fmicb.2018.03300
34. Davlantes E, Dimbu PR, Ferreira CM, Florinda Joao M, Pode D, Felix J, et al. Efficacy and safety of artemether-lumefantrine, artesunate-amodiaquine, and dihydroartemisinin-piperaquine for the treatment of uncomplicated Plasmodium falciparum malaria in three provinces in Angola, 2017. Malar J. (2018) 17:144. doi: 10.1186/s12936-018-2290-9
35. Egan AF, Chappel JA, Burghaus PA, Morris JS, McBride JS, Holder AA, et al. Serum antibodies from malaria-exposed people recognize conserved epitopes formed by the two epidermal growth factor motifs of MSP1(19), the carboxy-terminal fragment of the major merozoite surface protein of Plasmodium falciparum. Infect Immun. (1995) 63:456–66. doi: 10.1128/iai.63.2.456-466.1995
36. Stanisic DI, Richards JS, McCallum FJ, Michon P, King CL, Schoepflin S, et al. Immunoglobulin G subclass-specific responses against Plasmodium falciparum merozoite antigens are associated with control of parasitemia and protection from symptomatic illness. Infect Immun. (2009) 77:1165–74. doi: 10.1128/IAI.01129-08
37. White MT, Griffin JT, Akpogheneta O, Conway DJ, Koram KA, Riley EM, et al. Dynamics of the antibody response to Plasmodium falciparum infection in African children. J Infect Dis. (2014) 210:1115–22.
38. Taghavian O, Jain A, Joyner CJ, Ketchum S, Nakajima R, Jasinskas A, et al. Antibody profiling by proteome microarray with multiplex isotype detection reveals overlap between human and aotus nancymaae controlled malaria infections. Proteomics. (2018) 18:201700277.
39. Fowkes FJ, Richards JS, Simpson JA, Beeson JG. The relationship between anti-merozoite antibodies and incidence of Plasmodium falciparum malaria: a systematic review and meta-analysis. PLoS Med. (2010) 7:e1000218. doi: 10.1371/journal.pmed.1000218
40. Drakeley CJ, Corran PH, Coleman PG, Tongren JE, McDonald SL, Carneiro I, et al. Estimating medium- and long-term trends in malaria transmission by using serological markers of malaria exposure. Proc Natl Acad Sci USA. (2005) 102:5108–13. doi: 10.1073/pnas.0408725102
41. Ondigo BN, Hodges JS, Ireland KF, Magak NG, Lanar DE, Dutta S, et al. Estimation of recent and long-term malaria transmission in a population by antibody testing to multiple Plasmodium falciparum antigens. J Infect Dis. (2014) 210:1123–32. doi: 10.1093/infdis/jiu225
42. Rogier E, Moss DM, Chard AN, Trinies V, Doumbia S, Freeman MC, et al. Evaluation of immunoglobulin G responses to plasmodium falciparum and Plasmodium vivax in Malian school children using multiplex bead assay. Am J Trop Med Hyg. (2017) 96:312–8. doi: 10.4269/ajtmh.16-0476
43. Johnson AH, Leke RG, Mendell NR, Shon D, Suh YJ, Bomba-Nkolo D, et al. Human leukocyte antigen class II alleles influence levels of antibodies to the Plasmodium falciparum asexual-stage apical membrane antigen 1 but not to merozoite surface antigen 2 and merozoite surface protein 1. Infect Immun. (2004) 72:2762–71. doi: 10.1128/IAI.72.5.2762-2771.2004
44. Martins JF, Marques C, Nieto-Andrade B, Kelley J, Patel D, Nace D, et al. Malaria risk and prevention in Asian migrants to Angola. Am J Trop Med Hyg. (2020) 103:1918–26. doi: 10.4269/ajtmh.20-0706
45. Galatas B, Bassat Q, Mayor A. Malaria parasites in the asymptomatic: looking for the hay in the haystack. Trends Parasitol. (2016) 32:296–308. doi: 10.1016/j.pt.2015.11.015
46. Wu L, van den Hoogen LL, Slater H, Walker PG, Ghani AC, Drakeley CJ, et al. Comparison of diagnostics for the detection of asymptomatic Plasmodium falciparum infections to inform control and elimination strategies. Nature. (2015) 528:S86–93.
47. Fowkes FJ, McGready R, Cross NJ, Hommel M, Simpson JA, Elliott SR, et al. New insights into acquisition, boosting, and longevity of immunity to malaria in pregnant women. J Infect Dis. (2012) 206:1612–21. doi: 10.1093/infdis/jis566
48. Wu L, Mwesigwa J, Affara M, Bah M, Correa S, Hall T, et al. Antibody responses to a suite of novel serological markers for malaria surveillance demonstrate strong correlation with clinical and parasitological infection across seasons and transmission settings in The Gambia. BMC Med. (2020) 18:304. doi: 10.1186/s12916-020-01724-5
49. Ly A, Hansen DS. Development of B cell memory in malaria. Front Immunol. (2019) 10:559. doi: 10.3389/fimmu.2019.00559
50. Doritchamou J, Teo A, Morrison R, Arora G, Kwan J, Manzella-Lapeira J, et al. Functional antibodies against placental malaria parasites are variant dependent and differ by geographic region. Infect Immun. (2019) 87:e865–818.
51. Suau R, Vidal M, Aguilar R, Ruiz-Olalla G, Vazquez-Santiago M, Jairoce C, et al. RTS,S/AS01E malaria vaccine induces IgA responses against CSP and vaccine-unrelated antigens in African children in the phase 3 trial. Vaccine. (2021) 39:687–98. doi: 10.1016/j.vaccine.2020.12.038
Keywords: malaria, antibodies, isotypes, boosting, exposure
Citation: Rogier E, Nace D, Dimbu PR, Wakeman B, Beeson JG, Drakeley C, Tetteh K and Plucinski M (2022) Antibody dynamics in children with first or repeat Plasmodium falciparum infections. Front. Med. 9:869028. doi: 10.3389/fmed.2022.869028
Received: 03 February 2022; Accepted: 29 June 2022;
Published: 19 July 2022.
Edited by:
Gisely Melo, Fundação de Medicina Tropical Doutor Heitor Vieira Dourado (FMT-HVD), BrazilReviewed by:
Prashant Kumar Mallick, National Institute of Malaria Research (ICMR), IndiaAmre Nasr, King Saud bin Abdulaziz University for Health Sciences, Saudi Arabia
Copyright © 2022 Rogier, Nace, Dimbu, Wakeman, Beeson, Drakeley, Tetteh and Plucinski. This is an open-access article distributed under the terms of the Creative Commons Attribution License (CC BY). The use, distribution or reproduction in other forums is permitted, provided the original author(s) and the copyright owner(s) are credited and that the original publication in this journal is cited, in accordance with accepted academic practice. No use, distribution or reproduction is permitted which does not comply with these terms.
*Correspondence: Eric Rogier, ZXJvZ2llckBjZGMuZ292