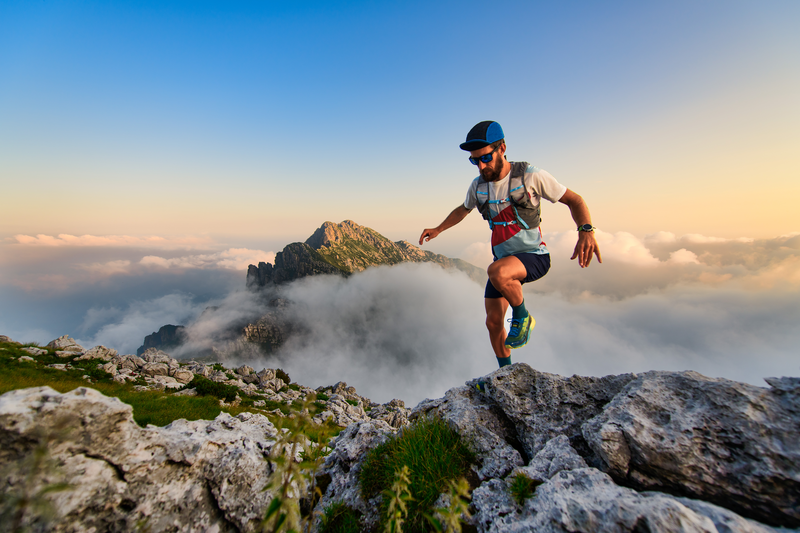
95% of researchers rate our articles as excellent or good
Learn more about the work of our research integrity team to safeguard the quality of each article we publish.
Find out more
OPINION article
Front. Med. , 17 March 2022
Sec. Intensive Care Medicine and Anesthesiology
Volume 9 - 2022 | https://doi.org/10.3389/fmed.2022.868514
This article is part of the Research Topic Multidisciplinary Critical Care Medicine – Getting Things Done Across Specialties View all 15 articles
Individuals commonly admitted to the intensive care unit (ICU) for acute respiratory failure, due to pulmonary or neuromuscular disease, shock and the need for airway protection or temporary respiratory support after major surgery, are generally placed on invasive mechanical ventilation support. The recovery processes of these patients is uncertain (the longer the duration of respiratory assistance, the more uncertain the vital prognosis) (1) and will take time even with the application of respiratory weaning process (i.e., spontaneous breathing periods alternated with respiratory assistance; enabling patients to gradually come back to unsupported spontaneous breathing) before leaving ICU (2). As these patients suffer from post-mechanical ventilation physical, cognitive, and mental sequelae, in addition to comorbidities (3), maximizing their recovery is therefore paramount to reduce any disabling experiences and to avoid the menace of relapse following discharge and consecutive risk of readmission (2).
It would be beneficial to adopt a multidisciplinary approach by pooling knowledge from the healthcare system—e.g., respiratory medicine and sport medicine—to advance the understanding of the (post-) ICU recovery process which will allow practitioners to set up the most beneficial rehabilitation program (4). In this view, based on neuroscience findings, we propose that Jeannerod's theory (5, 6) related motor simulation would be a useful complement to physiotherapy, motor rehabilitation and other accompanying interventions (e.g., speech therapy, nutritional, and psychological support) to optimize patients' return to autonomy.
Ventilation support for 2–4 weeks and respiratory weaning care (i.e., alternating spontaneous breathing periods with respiratory assistance) result in significant muscle atrophy and weakness (3, 7). It imposes great challenge for optimal recovery from respiratory muscle fatigue due to patients' low breathing capacity, inability to perform basic movements and fatigability. Interestingly, motor simulation offers a motor learning/relearning alternative process without performing any movement (8). Briefly, an individual is engaged in motor simulation when he/she is able to imagine, observe or verbally describe a movement (Figure 1) (8). According to Jeannerod (5, 6), motor imagery or action observation (called covert stage) and action execution (called overt stage) share a common activation of cortical motor systems. Of interest, overt stages could be replayed off-line through motor simulation that enables the brain to represent the sensorial consequences and future states of simulated actions. In the field of neuroscience, systematic scientific reviews and meta-analysis have shown, first, that the imagination or the observation of a movement activates a premotor-parietal and primary somatosensory network similarly to the one involved during “real” movement execution (9); second, that premotor and primary motor areas are activated during arm or leg movement verbalization and execution (10); third and last, as suggested almost two decades ago (11), that human brain is provided by internal motor efference copies between simulated (imagined, observed, verbalized) and “real” movements (12–14). While simulating or performing movements, these internal forward models are enrolled to predict movement-induced future states and sensorial consequences. Thus, simulated movements are movements except for the fact they do not generate motor output which is blocked by a motor command inhibitory mechanism (5, 6).
Figure 1. Principles to be considered and tailored to patients' individual needs for an efficient motor simulation process.
However, during a motor simulation, the absence of sensorimotor feedback (i.e., sensorimotor consequences of the motor command) could inactivate the somatosensory processes underlying movement representation, thereby restricting the impact of motor simulation on motor learning/relearning process (15). In the same vein, others have argued that peripheral (proprioceptive and haptic) information is essential for accessing and maintaining motor representations stored in the brain and that, in their absence, these representations fade and even cease to be accessible (16). For instance, the effects of transient sensorimotor deprivation following orthopedic trauma in patients with no prior neurological deficits revealed motor execution impairments and maladaptive plasticity in the somatosensory and motor cortices including shrinkage of somatosensory cortical maps, decreased cortical excitability, reduced cortical thickness in the sensorimotor cortex, decreased of fractional anisotropy in the corticospinal tract and decreased interhemispheric inhibition from the impacted to the non-impacted hemisphere (8). The impaired functioning of internal forward models (referring to the interactions between motor commands and environment, useful to predict the sensory consequences of an action) accounts for these alterations. Though, these models need to be updated through experience (i.e., training that can be translated into changes in synaptic weights which will improve future forward model prediction) to operate reliably (11). Because of the lack of sensorimotor input (proprioceptive, haptic) in the case of immobilization for instance, forward models remain available but are no longer updated, generating an inaccurate prediction of sensory consequences, and thus initiating unskilled and inefficient movements (11). Consequently, integrating afferent information (i.e., proprioceptive and haptic) during motor simulation should be encouraged (i.e., mimicking a movement and handling an object related to a specific movement) (8). It has been reported that mimicking a movement concomitantly to its motor/mental simulation increases its technical quality and efficacy (17).
Studies probing the effects of motor simulation interventions prescribed alongside motor rehabilitation to recover from detrimental effects of transient sensorimotor deprivation are scarce in individuals without any neurological history (8). Notably, in patients over 60 years who underwent total hip and knee arthroplasty surgery were instructed to feel the sensations of movements they mentally simulated, results indicated some benefits through an increased motor performance following observation of locomotor tasks (18) and greater quadriceps strength subsequent to the imagination of knee flexion-extension (19). These positive outcomes are deemed to be due to equivalences between simulated and executed movements (18, 19), to the integration of proprioceptive information during motor simulation (8, 17), to the combination of motor rehabilitation and simulation sessions (18, 19), and to cortical plasticity in response to internal or external constraints such as motor learning or training interventions (8, 18, 19). The scientific community hence agrees that cortical changes in healthy individuals exposed to motor learning/training are seen in brain areas and networks related to the physical execution of movements.
Despite the lack of scientific evidence promoting motor simulation benefits to ICU and/or post-ICU patients so far, its integration in the rehabilitation process could appear as a very promising adjunct for the following reasons.
First, delivering early active mobilization and rehabilitation in ICU, when the cardiovascular, respiratory and neurological states of patients are stable (20), has been shown to improve the rehabilitation outcomes (improved muscle strength, functional capacity and mobility; increased number of ventilator-free day and discharged-to-home rate) (21). In this view, motor simulation can be helpful in the early rehabilitation process (i.e., when the patient is temporarily completely or partially unable to move, too weak to exercise or subject to the use of supportive devices), as it offers a motor learning/relearning alternative without requiring patient to perform any movement (8). Whenever possible, it is important to alternate motor simulation and movement execution, whether active or assisted, within the same session, as the benefit of this approach has been demonstrated (18, 19).
Second, because ICU patients suffer from sensory deprivation increased by the interference of sedative and analgesic medication, it is essential to provide visual, proprioceptive, haptic information during motor simulation (e.g., watching a video of a movement, mimicking it, handling an object related to this movement). Interestingly, as observing an object automatically potentiates actions associated to it (22), showing objects that patient used in their daily lives will activate action representations (e.g., reaching or grasping actions when a mug is shown).
Third, depending on an individual's ability to use motor simulation techniques (e.g., patients with poor imagery ability), on his/her fatigability, motivation and individual needs, a variety of different simulation packages could be offered for an optimal motor simulation process. The Figure 1 summarizes the available motor simulation techniques that can be used alone or in combination (i.e., preferably associated with miming a movement and/or handling an object related to this movement to generate afferent information during (motor) simulation) and declined under varied modalities (8) to personalize the intervention in reference to the patient's requirements.
The present opinion aimed to promote motor simulation as a plausible non-invasive, safe, easy to implement and low cost complementary adjunct among the healthcare delivery provided during the (post-) ICU recovery process. Hopefully, the ICU practitioners and their multidisciplinary healthcare professionals will consider motor simulation as a practical, relevant and therapeutic option to maximize patient's return to autonomy.
The authors listed have made substantial, direct, and intellectual contribution to the work and approved it for publication.
The authors declare that the research was conducted in the absence of any commercial or financial relationships that could be construed as a potential conflict of interest.
All claims expressed in this article are solely those of the authors and do not necessarily represent those of their affiliated organizations, or those of the publisher, the editors and the reviewers. Any product that may be evaluated in this article, or claim that may be made by its manufacturer, is not guaranteed or endorsed by the publisher.
The French Institute of Sport is a partner of the French-speaking network ReFORM, recognized as a Research Center for the Prevention of Injury and Illness and the Protection of Athletes by the Olympic Committee (IOC). As a member of the IOC Medical Research Network, ReFORM has received funding from the IOC to establish long-term research programs on the prevention of injuries and illnesses in sport for the protection of athlete health.
1. Sun Y, Li S, Wang S, Li C, Li G, Xu J, et al. Predictors of 1-year mortality in patients on prolonged mechanical ventilation after surgery in intensive care unit: a multicenter, retrospective cohort study. BMC Anesthesiol. (2020) 20:44. doi: 10.1186/s12871-020-0942-0
2. Vincent JL. The post-ICU patient. ICU Manag Pract. (2020) 20:238. Available online at: https://healthmanagement.org/c/icu/issuearticle/the-post-icu-patient-1
3. Haviland K, Tan KS, Schwenk N, Pillai MV, Stover DE, Downey RJ. Outcomes after long-term mechanical ventilation of cancer patients. BMC Palliat Care. (2020) 19:42. doi: 10.1186/s12904-020-00544-x
4. Faghy MA, Ashton RE, Maden-Wilkinson TM, Copeland RJ, Bewick T, Smith A, et al. Integrated sports and respiratory medicine in the aftermath of COVID-19. Lancet Respir Med. (2020) 8:852. doi: 10.1016/S2213-2600(20)30307-6
5. Jeannerod M. Neural simulation of action: a unifying mechanism for motor cognition. Neuroimage. (2001) 14:S103–9. doi: 10.1006/nimg.2001.0832
6. Jeannerod M. The representing brain: neural correlates of motor intention and imagery. Behav Brain Sci. (1994) 17:187–202. doi: 10.1017/S0140525X00034026
7. MacIntyre NR, Epstein SK, Carson S, Scheinhorn D, Christopher K, Muldoon S, et al. Management of patients requiring prolonged mechanical ventilation: report of a NAMDRC consensus conference. Chest. (2005) 128:3937–54. doi: 10.1378/chest.128.6.3937
8. Calmels C. Beyond Jeannerod's motor simulation theory: an approach for improving post-traumatic motor rehabilitation. Neurophysiol Clin. (2019) 49:99–107. doi: 10.1016/j.neucli.2019.01.033
9. Hardwick RM, Caspers S, Eickhoff SB, Swinnen SP. Neural correlates of action: comparing meta-analyses of imagery, observation, and execution. Neurosci Biobehav Rev. (2018) 94:31–44. doi: 10.1016/j.neubiorev.2018.08.003
10. Hauk O, Johnsrude I, Pulvermuller F. Somatotopic representation of action words in human motor and premotor cortex. Neuron. (2004) 41:301–7. doi: 10.1016/S0896-6273(03)00838-9
11. Wolpert DM, Flanagan JR. Motor prediction. Curr Biol. (2001) 11:R729–32. doi: 10.1016/S0960-9822(01)00432-8
12. Gazzola V, Keysers C. The observation and execution of actions share motor and somatosensory voxels in all tested subjects: single-subject analyses of unsmoothed fMRI data. Cereb Cortex. (2009) 19:1239–55. doi: 10.1093/cercor/bhn181
13. Whitford TJ, Jack BN, Pearson D, Griffiths O, Luque D, Harris AW, et al. Neurophysiological evidence of efference copies to inner speech. Elife. (2017) 6:e28197. doi: 10.7554/eLife.28197
14. Kilteni K, Andersson BJ, Houborg C, Ehrsson HH. Motor imagery involves predicting the sensory consequences of the imagined movement. Nat Commun. (2018) 9:1617. doi: 10.1038/s41467-018-03989-0
15. Lacourse MG, Turner JA, Randolph-Orr E, Schandler SL, Cohen MJ. Cerebral and cerebellar sensorimotor plasticity following motor imagery-based mental practice of a sequential movement. J Rehabil Res Dev. (2004) 41:505–24. doi: 10.1682/JRRD.2004.04.0505
16. Bosbach S, Cole J, Prinz W, Knoblich G. Inferring another's expectation from action: the role of peripheral sensation. Nat Neurosci. (2005) 8:1295–7. doi: 10.1038/nn1535
17. Guillot A, Moschberger K, Collet C. Coupling movement with imagery as a new perspective for motor imagery practice. Behav Brain Funct. (2013) 9:8. doi: 10.1186/1744-9081-9-8
18. Marusic U, Grospretre S, Paravlic A, Kovac S, Pisot R, Taube W. Motor imagery during action observation of locomotor tasks improves rehabilitation outcome in older adults after total hip arthroplasty. Neural Plast. (2018) 2018:5651391. doi: 10.1155/2018/5651391
19. Moukarzel M, Di Rienzo F, Lahoud JC, Hoyek F, Collet C, Guillot A, et al. The therapeutic role of motor imagery during the acute phase after total knee arthroplasty: a pilot study. Disabil Rehabil. (2019) 41:926–33. doi: 10.1080/09638288.2017.1419289
20. Devlin JW, Skrobik Y, Gelinas C, Needham DM, Slooter AJC, Pandharipande PP, et al. Clinical practice guidelines for the prevention and management of pain, agitation/sedation, delirium, immobility, and sleep disruption in adult patients in the ICU. Crit Care Med. (2018) 46:e825–e73. doi: 10.1097/CCM.0000000000003299
21. Zhang L, Hu W, Cai Z, Liu J, Wu J, Deng Y, et al. Early mobilization of critically ill patients in the intensive care unit: a systematic review and meta-analysis. PLoS ONE. (2019) 14:e0223185. doi: 10.1371/journal.pone.0223185
Keywords: intensive care medicine, immobilization, sensorimotor deprivation, motor learning, public health
Citation: Calmels C, Le Garrec S and Brocherie F (2022) Motor Simulation as an Adjunct to Patient Recovery Process Following Intensive Care Unit Admission. Front. Med. 9:868514. doi: 10.3389/fmed.2022.868514
Received: 02 February 2022; Accepted: 23 February 2022;
Published: 17 March 2022.
Edited by:
Björn Tampe, University Medical Center Göttingen, GermanyReviewed by:
Jason Qu, Massachusetts General Hospital and Harvard Medical School, United StatesCopyright © 2022 Calmels, Le Garrec and Brocherie. This is an open-access article distributed under the terms of the Creative Commons Attribution License (CC BY). The use, distribution or reproduction in other forums is permitted, provided the original author(s) and the copyright owner(s) are credited and that the original publication in this journal is cited, in accordance with accepted academic practice. No use, distribution or reproduction is permitted which does not comply with these terms.
*Correspondence: Franck Brocherie, ZnJhbmNrLmJyb2NoZXJpZUBpbnNlcC5mcg==
Disclaimer: All claims expressed in this article are solely those of the authors and do not necessarily represent those of their affiliated organizations, or those of the publisher, the editors and the reviewers. Any product that may be evaluated in this article or claim that may be made by its manufacturer is not guaranteed or endorsed by the publisher.
Research integrity at Frontiers
Learn more about the work of our research integrity team to safeguard the quality of each article we publish.