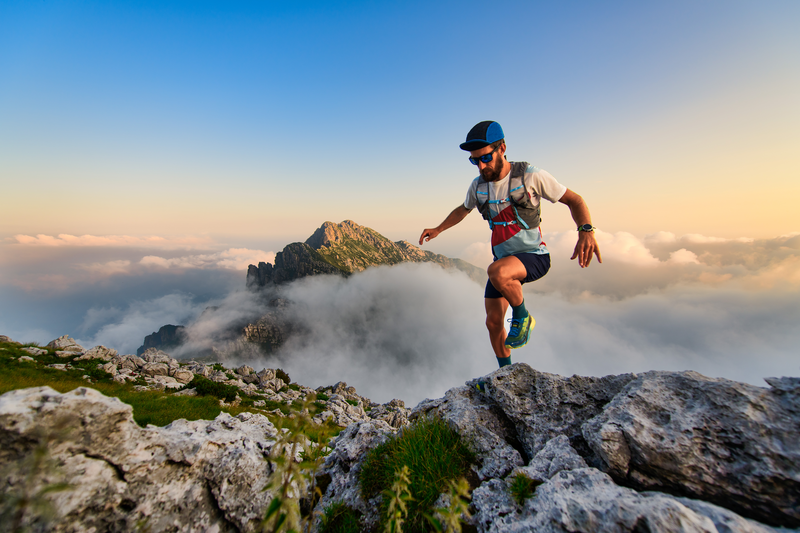
94% of researchers rate our articles as excellent or good
Learn more about the work of our research integrity team to safeguard the quality of each article we publish.
Find out more
MINI REVIEW article
Front. Med. , 16 May 2022
Sec. Pathology
Volume 9 - 2022 | https://doi.org/10.3389/fmed.2022.866746
This article is part of the Research Topic Organ Fibrosis: Pathogenesis, Biomarkers and Therapeutic Targets, Volume II View all 7 articles
Fibrosis, also known as organ scarring, describes a pathological stiffening of organs or tissues caused by increased synthesis of extracellular matrix (ECM) components. In the past decades, mounting evidence has accumulated showing that the coagulation cascade is directly associated with fibrotic development. Recent findings suggest that, under inflammatory conditions, various cell types (e.g., immune cells) participate in the coagulation process causing pathological outcomes, including fibrosis. These findings highlighted the potential of anticoagulation therapy as a strategy in organ fibrosis. Indeed, preclinical and clinical studies demonstrated that the inhibition of blood coagulation is a potential intervention for the treatment of fibrosis across all major organs (e.g., lung, liver, heart, and kidney). In this review, we aim to summarize our current knowledge on the impact of components of coagulation cascade on fibrosis of various organs and provide an update on the current development of anticoagulation therapy for fibrosis.
Fibrosis (organ scarring) denotes a pathological condition characterized by the elevation of interstitial fibrous tissue. The hallmarks of fibrosis include the presence and persistence of myofibroblasts and collagen deposits. Fibrosis is a consequence of failed tissue repair, often occurring due to a severe incurable, persisting, or repeated injury.
An injured tissue activates a tightly coordinated tissue repair program that is classically divided into four processes: (1) hemostasis, (2) inflammation, (3) proliferation, and (4) remodeling (1). Hemostasis induces vasodilation and platelet “plug” activation in response to an injury. Hemostasis activates blood coagulation, a complex cascade system that leads to fibrin clot formation in the damaged tissue. The fibrin clot minimizes bleeding as well as traps immune cells and pathogens facilitating immune response against infection (2). Hemostasis is accompanied by inflammation, a localized response to tissue damage. Hemostasis and inflammation affect each other: inflammation activates the hemostatic system that, in turn, controls inflammatory activity (3).
To date, studies have indicated that the persistence of fibrin in the matrix promotes fibrosis (4); increased levels of fibrinogen or impaired fibrinolysis increases collagen formation and subsequent fibrosis, while loss of fibrinogen or improved fibrinolysis ameliorates fibrosis (3–9). These findings led to the identification of coagulation factors as therapeutic targets in organ fibrosis. Indeed, growing evidence shows that the inhibition of blood coagulation is a promising intervention for the treatment of fibrosis across all major organs (5). However, the precise mechanistic link between fibrin and collagen formation still deserves further investigations.
Blood coagulation is a cascade system of enzymes that collectively produce fibrin clot in damaged tissues. The fibrin clot provides a provisional matrix for tissue repair: the fibrin matrix acts as a reservoir of growth factors and proinflammatory cytokines, promoting leukocyte migration, and the accumulation, activation, and proliferation of fibroblasts (6).
The coagulation cascade is divided into three pathways: the intrinsic, extrinsic, and common pathways (Figure 1) (10). The extrinsic pathway consists of the extravascular tissue factor (TF) and coagulation factor VII/VIIa (FVII/FVIIa) (11). TF is a protein constitutively expressed in tissues (e.g., by smooth muscle cells, pericytes, and fibroblasts) (7). Once TF binds to circulating FVIIa, the TF:FVIIa complex is formed. The TF:FVIIa complex then hydrolyses FX to its active form FXa. Under inflammatory conditions, TF is additionally expressed by monocytes, neutrophils, endothelial cells, and platelets leading to pathologic outcomes (e.g., thrombosis) (12). The intrinsic pathway consists of FIX, FXI, and FXII. The intrinsic pathway is also called the contact activation pathway because coagulation factors are activated by an externally charged surface (e.g., collagen). The intrinsic pathway begins with the binding of three plasma proteins (FXII, prekallikrein, high molecular weight kininogen) to a surface (13). The subsequent activation of FXII to FXIIa converts FXI to FXIa. FXIa, in turn, activates FIX, which forms a complex with its cofactor FVIIIa, converting FX to FXa. In the common pathway, FXa and its cofactor FV activate prothrombin to produce thrombin (FIIa). Thrombin subsequently converts fibrinogen to form fibrin, and activates FXIII to FXIIIa, covalently crosslinking the fibrin strands to form a more stable fibrin network. Once thrombin is activated, the amplification of coagulation begins. This occurs through thrombin, which catalysis the activation of FV, FVII, FIX, FX, and FXI (14).
During normal tissue repair, fibrin is removed by a process, called fibrinolysis, mediated by the serine protease plasmin, which is converted from plasminogen by the urokinase-type (uPA) and tissue-type plasminogen activator (tPA) and regulated by plasminogen activator inhibitor-1 (PAI-1) (15). PAI-1 is induced inflammatory cytokines and growth factors (e.g., EGF, IL-1β, TGF-β) (8). Normally, PAI-1 is significantly elevated in fibrotic tissues, and PAI-1 deficiency protects organs from fibrosis in response to injury-related profibrotic signals (8).
Recent studies on the coagulation cascade have revealed that the function of the coagulation cascade is not limited to fibrin clot formation and prevention of blood loss, but it is also crucial in regulating the inflammatory response, tissue repair, and wound healing process (16).
The tissue factor (TF)-FVII/VIIa complex has been studied as a potential mechanism by which the coagulation cascade contributes to pulmonary fibrosis development (17). TF expression is upregulated in the bronchoalveolar lavage fluid (BALF) of IPF patients and on type II pneumocytes of IPF patients (18, 19). Concerning IPF, the TF-FVII/VIIa complex has also been observed in the BALF obtained from patients with acute respiratory distress syndrome (ARDS); lung injury of which fluid leakage into the lungs may progress to a fibroproliferative response (20, 21). FVII has also been linked to intra-alveolar fibrosis, considered to be involved in the merging of alveolar walls observed in IPF, as it has been identified in intra-alveolar fibrosis patients (22, 23).
Increasing amounts of evidence supported that both FX/Xa and thrombin, other than their role in downstream coagulation cascade precursor activation, are involved in the fibro-proliferative response activation in IPF (23–27). Increased FXa expression has been reported in fibrotic lung tissue (28). The presence of FX activating procoagulant microparticles has been identified in the airways of IPF patients (29). Elevated thrombin levels in BALF samples obtained from pulmonary fibrosis patients have been reported as well (30, 31). It has been shown that FX/Xa and thrombin can cleave protease-activated receptors (PARs) for the activation of fibroblast proliferation and myofibroblast differentiation (32–35). FX/Xa and thrombin-mediated activation of PARs profibrotic effects also induce proinflammatory cytokine secretion and ECM deposition stimulation (26, 36).
The involvement of coagulation FVIII/VIIIc, FXIIa, and other prothrombotic factors may also play important roles in IPF. Elevated levels of FVIII/VIIIc were observed in IPF patients (35, 37). In one study, IPF patient lung fibroblasts were reported to have enhanced binding capacity for FXIIa, suggesting a potential role of FXIIa in fibrosis (38).
Several clinical studies have indicated that anticoagulation may be a promising therapeutic approach in hepatic fibrosis (39–43). Congruently, a meta-analysis of preclinical studies also provided strong evidence that anticoagulants alleviate hepatic fibrosis (44). Chronic liver diseases are often associated with portal vein thrombosis (PVT), and anticoagulation is a standard of care for treating PVT. Thus, studies have assessed the pharmacological safety of anticoagulants in patients with advanced chronic liver diseases (45–47).
Coagulation factors, FV, FVII, and FX are particularly prominent in hepatic fibrosis and cirrhosis (48–50). Due to the promotion of prothrombic state by these factors, fibrin(ogen) deposits may obstruct the hepatic sinusoids, which affect the blood flow and may set the base for fibrosis initiation or liver disease advancement (48). A connection between TF and hepatic fibrosis is suggested as fibrosis was reduced in transgenic mice lacking TF (51). In preclinical studies, FXa played an essential role in CCl4-induced hepatic fibrosis (52). Furthermore, FXa stimulated proinflammatory and profibrotic mediator production and thus activated hepatic stellate cells (HSCs) and the fibrogenic process (52). Also, TF signaling plays a direct role in CD68+ macrophage activation (53). Macrophages constitutively express and upregulate TF as macrophages mature (53). Kupffer cell polarization (M1) may be regulated and sustained by TF signaling (53).
A murine cardiac fibrosis model exhibited elevated levels of locally produced FXa compared to sham-operated mice. FXa is upregulated in the hypertrophic fibrotic cardiac interstitium (54). During cardiac fibrosis, PARs are activated by thrombin and FXa and induce cardiac fibroblast proliferation as well as myofibroblast activation (55). The infarction size of PAR-2 KO mice was significantly reduced after 30 min of ischemia on the heart, and the mRNA transcription of inflammatory genes, IL-1β, IL-6, and TNF-α, was attenuated in the heart of PAR-2 KO mice (56). However, transgenic mice that overexpressed PAR-1 on cardiomyocytes exhibited increased hypertrophy and cardiac remodeling, although whether PAR-1 directly affected myocardial infarction is unclear (57). Also, peptide agonists of PAR-1 and PAR-2 stimulated cardiac fibroblast proliferation. These collective data corroborated the role of PAR signaling mediated by coagulation factors, thrombin and FXa in cardiac inflammation, fibrotic response, and repair process.
The role of coagulation factors in kidney disease beyond normal hemostasis and thrombosis has been long suspected, and the increasing clinical utilization of anticoagulants targeting specific coagulation factors gain deeper insights into the mechanisms through which the coagulation system modulates renal health (58). Notably, increased TF expression has been detected in acute and chronic kidney diseases (59–64). Proinflammatory stimuli induce glomerular FV expression, whereas kidney IRI induces tubular fibrinogen expression (63, 65–67). Direct effects of coagulation proteases such as thrombin or FXa on glomerular cells have long been known, implying receptor-dependent modulation of intracellular signaling pathways (63, 68). The expression of PARs in renal cells provides a molecular link between coagulation factors and renal cell function (69–72).
Heparin and its low-molecular-weight derivatives (LMWHs, e.g., enoxaparin, nadroparin tinzaparin) are anticoagulants widely used in the prevention of blood clots (e.g., during hemodialysis, surgery) and in the prophylaxis and treatment of venous thromboembolism and myocardial infarction. Heparin inhibits blood coagulation by increasing the activity of antithrombin III, an enzymatic inhibitor of thrombin, FXa, and other proteases (Figure 1). Although heparin does not directly dissolve already formed clots, heparin may support the body’s natural clot lysis mechanisms. Therefore, several studies have assessed the anti-fibrotic activity of heparin and LMWHs using preclinical models. Consequently, researchers have found that heparin and LMWHs alleviate experimental pulmonary (73, 74) and hepatic (75–79), as well as endomysial fibrosis (80). In line with this, clinical studies corroborated the therapeutic potential of heparin and LMWHs in organ fibrosis by demonstrating that heparin and LMWHs inhibit collagen proliferation in the liver with chronic hepatitis B virus infection (39). Despite positive perspectives, clinical trials of heparin and LMWHs for organ fibrosis have been so far without success. Anti-fibrotic therapies with heparin and LMWHs appeared to pose more risks than benefits (81).
Warfarin is another widely used anticoagulant. As a vitamin K antagonist, warfarin inhibits vitamin K epoxide reductase, which is essential for the reactivation of vitamin K1 and, in turn, for ensuring the activity of vitamin K1-dependent coagulation factors such as thrombin, FVII, FIX, and FXa (82) (Figure 1). In animal studies, warfarin showed protective effects on hepatic fibrosis following congestive hepatopathy induced by partial ligation of the inferior vena cava (82) as well as following a CCl4 challenge (83). Clinical studies revealed that warfarin and LMWHs ameliorate portal vein thrombosis in patients with liver cirrhosis reducing de novo occurrence, improving new hepatic decompensation, and survival of patients with advanced disease (42). Other studies show that warfarin, heparin, and LMWHs reduce the proliferation and activation of HSCs limiting fibrotic areas (75). However, it remains to be clarified whether warfarin, heparin, and LMWHs have direct effects on HSCs, or the suppression of HSCs is rather a consequence of the anti-fibrotic activities of the anticoagulants. Warfarin does not appear to be effective in IPF. Warfarin was not beneficial in experimental model of IPF (24). Congruently, a clinical study of warfarin in IPF was suspended due to a low probability of benefit and an increase in mortality (84). However, this was contrary to the result of a precedent clinical study, where patients administered with warfarin in combination with prednisolone demonstrated significantly improved survival rates in acute IPF exacerbations as compared to the group treated with prednisolone alone (85).
Anti-platelet agents, e.g., aspirin, clopidogrel, ticagrelor, and prasugrel, serve as potential candidates for thrombosis treatment. Activated platelets, in combination with FVIIa, mark the initiation of the coagulation cascade (Figure 1). It is unclear whether TF is expressed by platelets (86). However, the formation of the TF-FVIIa complex may occur on platelets, potentially after absorbing microvesicles containing TF (86–88). Platelets are known for their role in accelerating the activation of coagulation cascade by setting the base for the prothrombinase complex to form (89). Platelets, by aggregating to the site of fibrosis, may worsen organ destruction (90). Research has shown that anti-platelet agents are also effective in attenuating organ fibrosis.
Aspirin inhibits platelets by acetylating serine529 in the active site of the platelet cyclooxygenase (COX) (91). By changing the structure of the active site, the platelet-dependent thromboxane formation is inhibited (91). Aspirin can reduce the severity of hepatic fibrosis and renal impairment (75, 92). Significant improvement in hepatic fibrosis was observed at both low- and high-dose levels of aspirin-treated groups, with a remarkable improvement in the high-dose aspirin-treated group (75). In the case of CKD, aspirin has beneficial effects in mild renal impairment conditions compared to moderate renal impairment conditions (92).
Clopidogrel is another anti-platelet drug used to treat thrombosis and has found applications in the treatment of certain types of fibrosis in mouse models (93). Clopidogrel works as an anti-platelet drug by preventing ADP from stimulating P2Y12, the purinergic receptor of platelets (94). Clopidogrel combats fibrosis through mechanisms related to angiotensin II, which has had reports of being a pro-fibrotic factor (93). Therapeutic effects of clopidogrel when combating fibrosis are assumed to occur by inhibiting angiotensin II-induced accumulation of myofibroblasts (93). As a treatment of hepatic fibrosis, clopidogrel shows a more promising effect compared to dabigatran, a direct oral anticoagulant, indicated by the difference between the hydroxyproline expressions: the main component of collagen and a potential fibrosis marker (95).
Other platelet inhibitors are currently under development for the treatment of fibrosis. Ticagrelor and prasugrel are P2Y12 antagonists and may also work as platelet antagonists. These drugs bind to P2Y12, the adenosine diphosphate (ADP) receptor of platelets, and prevent blood coagulation (93). A recent study has shown that ticagrelor may have a positive effect in ameliorating pulmonary fibrosis in rats (96). Likewise, prasugrel may have positive effects on relieving hepatic fibrosis (90). However, further research is necessary for these drugs to be used in human clinical trials as it is unclear, whether the effects in animal studies would be translatable to patients is unclear (96).
Direct oral anticoagulants (DOAC)—dabigatran, rivaroxaban, apixaban, edoxaban, and betrixaban are anticoagulation pharmacotherapies used for the prevention of thrombosis (Figure 1). DOACs are categorized into two main classes: direct FXa inhibitors (rivaroxaban, apixaban, edoxaban, and betrixaban) and direct thrombin inhibitors (dabigatran).
Rivaroxaban, apixaban, and edoxaban are direct FXa inhibitors that inhibit FXa by directly binding to the active site of FXa. Fondaparinux is an indirect FXa inhibitor that binds to antithrombin and changes its conformation which leads to inhibition of FXa (97). Dabigatran is a direct thrombin inhibitor that binds directly to active sites of thrombin and inhibits its activity (98). Recent studies revealed a correlation between thrombin and FXa with organ fibrosis, and the effectiveness of DOAC in treating organ fibrosis has emerged as a novel treatment of organ fibrosis. In an atrial fibrosis-induced model by transverse aortic constriction, the expression of TNF-α, IL-1β, and IL-6 was suppressed by treating rivaroxaban (99). In addition, collagen III, connective tissue growth factors, and TGF-β, which are upregulated during fibrosis development, were decreased by rivaroxaban treatment. Rivaroxaban treatment also inhibited FXa-mediated phosphorylation of NF-κB p65 and STAT3, which are important mediators of myofibroblast differentiation and fibrosis development (54). In the myocardial ischemia (MI)-induced fibrosis model, expression of collagen 1a1 and collagen 3a1 was significantly decreased after a high dosage of direct FXa inhibitor apixaban which indicates the efficacy of apixaban in attenuating MI-induced fibrosis (100). In unilateral ureteral obstruction (UUO) mice, which is the renal tubulointerstitial model, edoxaban treatment attenuated renal interstitial macrophage infiltration and release of inflammatory cytokines. Also, the expression of collagen II and III, TGF-β, and α-smooth muscle actin (SMA) was significantly attenuated with edoxaban treatment (101). Treatment of fondaparinux to kidney fibrosis, which was induced in mice by IRI, reduced macrophage infiltration. Also, proinflammatory IL-1β mRNA levels decreased in fondaparinux-treated models (67).
The efficacy of direct thrombin inhibitors in treating organ fibrosis has been revealed by dabigatran treatment. Both early and late treatment of dabigatran attenuated the development of bleomycin-induced pulmonary fibrosis by significantly reducing thrombin activity and inflammatory cells and protein concentrations in the bleomycin-induced pulmonary fibrosis model (102). Additionally, dabigatran inhibited thrombin-induced cell proliferation, α-SMA expression and organization, and the in vitro production of collagen and connective tissue growth factor (CTGF) by lung fibroblasts (103). Dabigatran attenuates cardiac fibrosis by improving coronary flow reserve and global cardiac function potentially by inhibiting thrombin activity and down-regulating PAR-1 expression (104). In the UUO model, dabigatran significantly inhibited UUO-induced type 1 collagen and tubulointerstitial fibrosis by inhibiting thrombin-activated PAR-1 expression in fibrotic kidneys (105). In hepatic IRI mice, dabigatran treatment significantly improved liver histological damage, induced sinusoidal protection, and provided both antiapoptotic and anti-inflammatory effects (106).
Rivaroxaban, co-administered with aspirin alone or with aspirin plus clopidogrel or ticlopidine, is indicated in the prevention of atherothrombotic events in patients with acute coronary syndrome (107). In clinical studies, drugs that pharmacologically target FX were effective in patients with cirrhosis of the liver. Rivaroxaban is currently in Phase III trials for treating patients with liver cirrhosis.
A large body of evidence has accumulated over the past decades to support the potential of anticoagulants as a promising therapeutic strategy for organ fibrosis. The literal meaning of the term “fibrosis” is “fibrous growth or development in an organ”; that of “fibrin” is “fibrous substance.” Although these terms appear to be semantically related, not much attention was previously paid to the biological link between fibrosis and coagulation. Preclinical and clinical studies have consistently demonstrated that the inhibition of coagulation may be beneficial in organ fibrosis. However, there have been so far only a few anticoagulation drug approvals/trials for the treatment of organ fibrosis per se, although anticoagulants are currently being used for complications, which are frequent in organ fibrosis. Anticoagulants are effective but have serious adverse effects making us extra cautious when expanding the application areas of these therapies. To date, our mechanistic understanding of how coagulation contributes to organ fibrosis is still largely unclear. Deeper insights into the precise mechanistic and functional role of coagulation in fibrotic development will allow us to better weigh the benefits and risks of anticoagulants in anti-fibrotic therapy.
All authors listed have made a substantial, direct, and intellectual contribution to the work, and approved it for publication.
This study was funded by the 2022 University Innovation Support Project at Handong Global University led by the Ministry of Education of the Republic of Korea.
The authors declare that the research was conducted in the absence of any commercial or financial relationships that could be construed as a potential conflict of interest.
All claims expressed in this article are solely those of the authors and do not necessarily represent those of their affiliated organizations, or those of the publisher, the editors and the reviewers. Any product that may be evaluated in this article, or claim that may be made by its manufacturer, is not guaranteed or endorsed by the publisher.
ARDS, acute respiratory distress syndrome; BALF, bronchoalveolar lavage fluid; ECM, extracellular matrix; IPF, idiopathic pulmonary fibrosis; IRI, ischemia-reperfusion injury; LMWH, low-molecular-weight heparin; PAI, plasminogen activator inhibitor; PAR, protease-activated receptor; SMA, smooth muscle actin; TF, tissue factor; MMP, matrix metalloprotease; TIMP, tissue inhibitor of metalloprotease.
1. Landén NX, Li D, Ståhle M. Transition from inflammation to proliferation: a critical step during wound healing. Cell Mol Life Sci. (2016) 73:3861–85. doi: 10.1007/s00018-016-2268-0
2. Barker TH, Engler AJ. The provisional matrix: setting the stage for tissue repair outcomes. Matrix Biol. (2017) 60-61:1–4. doi: 10.1016/j.matbio.2017.04.003
4. Loskutoff DJ, Quigley JP. PAI-1, fibrosis, and the elusive provisional fibrin matrix. J Clin Invest. (2000) 106:1441–3. doi: 10.1172/JCI11765
5. Lin C, Borensztajn K, Spek CA. Targeting coagulation factor receptors – protease-activated receptors in idiopathic pulmonary fibrosis. J Thromb Haemost. (2017) 15:597–607. doi: 10.1111/jth.13623
6. Mercer PF, Chambers RC. Coagulation and coagulation signalling in fibrosis. Biochim Biophys Acta. (2013) 1832:1018–27. doi: 10.1016/j.bbadis.2012.12.013
7. Mackman N, Tilley RE, Key NS. Role of the extrinsic pathway of blood coagulation in hemostasis and thrombosis. Arterioscler Thromb Vasc Biol. (2007) 27:1687–93. doi: 10.1161/ATVBAHA.107.141911
8. Ghosh AK, Vaughan DE. PAI-1 in tissue fibrosis. J Cell Physiol. (2012) 227:493–507. doi: 10.1002/jcp.22783
9. Baek JH, Zeng R, Weinmann-Menke J, Valerius MT, Wada Y, Ajay AK, et al. IL-34 mediates acute kidney injury and worsens subsequent chronic kidney disease. J Clin Invest. (2015) 125:3198–214. doi: 10.1172/JCI81166
10. Vojacek JF. Should we replace the terms intrinsic and extrinsic coagulation pathways with tissue factor pathway? Clin Appl Thromb Hemost. (2017) 23:922–7. doi: 10.1177/1076029616673733
11. Bernardi F, Mariani G. Biochemical, molecular and clinical aspects of coagulation factor VII and its role in hemostasis and thrombosis. Haematologica. (2021) 106:351–62. doi: 10.3324/haematol.2020.248542
12. Grover SP, Mackman N. Tissue factor: an essential mediator of hemostasis and trigger of thrombosis. Arterioscler Thromb Vasc Biol. (2018) 38:709–25. doi: 10.1161/ATVBAHA.117.309846
13. Wu Y. Contact pathway of coagulation and inflammation. Thromb J. (2015) 13:17. doi: 10.1186/s12959-015-0048-y
14. Sang Y, Roest M, de Laat B, de Groot PG, Huskens D. Interplay between platelets and coagulation. Blood Rev. (2021) 46:100733. doi: 10.1016/j.blre.2020.100733
15. Chapin JC, Hajjar KA. Fibrinolysis and the control of blood coagulation. Blood Rev. (2015) 29:17–24.
16. Chambers RC, Scotton CJ. Coagulation cascade proteinases in lung injury and fibrosis. Proc Am Thorac Soc. (2012) 9:96–101. doi: 10.1513/pats.201201-006AW
17. Wygrecka M, Kwapiszewska G, Jablonska E, von Gerlach S, Henneke I, Zakrzewicz D, et al. Role of protease-activated receptor-2 in idiopathic pulmonary fibrosis. Am J Respir Crit Care Med. (2011) 183:1703–14. doi: 10.1164/rccm.201009-1479OC
18. Fujii M, Hayakawa H, Urano T, Sato A, Chida K, Nakamura H, et al. Relevance of tissue factor and tissue factor pathway inhibitor for hypercoagulable state in the lungs of patients with idiopathic pulmonary fibrosis. Thromb Res. (2000) 99:111–7. doi: 10.1016/s0049-3848(00)00237-1
19. Imokawa S, Sato A, Hayakawa H, Kotani M, Urano T, Takada A. Tissue factor expression and fibrin deposition in the lungs of patients with idiopathic pulmonary fibrosis and systemic sclerosis. Am J Respir Crit Care Med. (1997) 156(2 Pt 1):631–6. doi: 10.1164/ajrccm.156.2.9608094
20. Marshall RP, Bellingan G, Webb S, Puddicombe A, Goldsack N, McAnulty RJ, et al. Fibroproliferation occurs early in the acute respiratory distress syndrome and impacts on outcome. Am J Respir Crit Care Med. (2000) 162:1783–8. doi: 10.1164/ajrccm.162.5.2001061
21. Idell S, James KK, Levin EG, Schwartz BS, Manchanda N, Maunder RJ, et al. Local abnormalities in coagulation and fibrinolytic pathways predispose to alveolar fibrin deposition in the adult respiratory distress syndrome. J Clin Invest. (1989) 84:695–705. doi: 10.1172/JCI114217
22. Fukuda Y, Basset F, Ferrans VJ, Yamanaka N. Significance of early intra-alveolar fibrotic lesions and integrin expression in lung biopsy specimens from patients with idiopathic pulmonary fibrosis. Hum Pathol. (1995) 26:53–61. doi: 10.1016/0046-8177(95)90114-0
23. Peyrol S, Cordier JF, Grimaud JA. Intra-alveolar fibrosis of idiopathic bronchiolitis obliterans-organizing pneumonia. Cell-matrix patterns. Am J Pathol. (1990) 137:155–70.
24. Shea BS, Probst CK, Brazee PL, Rotile NJ, Blasi F, Weinreb PH, et al. Uncoupling of the profibrotic and hemostatic effects of thrombin in lung fibrosis. JCI Insight. (2017) 2:e86608. doi: 10.1172/jci.insight.86608
25. Novelli F, Neri T, Tavanti L, Armani C, Noce C, Falaschi F, et al. Procoagulant, tissue factor-bearing microparticles in bronchoalveolar lavage of interstitial lung disease patients: an observational study. PLoS One. (2014) 9:e95013. doi: 10.1371/journal.pone.0095013
26. Blanc-Brude OP, Archer F, Leoni P, Derian C, Bolsover S, Laurent GJ, et al. Factor Xa stimulates fibroblast procollagen production, proliferation, and calcium signaling via PAR1 activation. Exp Cell Res. (2005) 304:16–27. doi: 10.1016/j.yexcr.2004.10.021
27. Blanc-Brude OP, Chambers RC, Leoni P, Dik WA, Laurent GJ. Factor Xa is a fibroblast mitogen via binding to effector-cell protease receptor-1 and autocrine release of PDGF. Am J Physiol Cell Physiol. (2001) 281:C681–9. doi: 10.1152/ajpcell.2001.281.2.C681
28. Scotton CJ, Krupiczojc MA, Königshoff M, Mercer PF, Lee YC, Kaminski N, et al. Increased local expression of coagulation factor X contributes to the fibrotic response in human and murine lung injury. J Clin Invest. (2009) 119:2550–63. doi: 10.1172/JCI33288
29. Neri T, Lombardi S, Faìta F, Petrini S, Balìa C, Scalise V, et al. Pirfenidone inhibits p38-mediated generation of procoagulant microparticles by human alveolar epithelial cells. Pulm Pharmacol Ther. (2016) 39:1–6. doi: 10.1016/j.pupt.2016.05.003
30. Hernández-Rodríguez NA, Cambrey AD, Harrison NK, Chambers RC, Gray AJ, Southcott AM, et al. Role of thrombin in pulmonary fibrosis. Lancet. (1995) 346:1071–3. doi: 10.1016/s0140-6736(95)91744-6
31. Dik WA, Zimmermann LJ, Naber BA, Janssen DJ, van Kaam AH, Versnel MA. Thrombin contributes to bronchoalveolar lavage fluid mitogenicity in lung disease of the premature infant. Pediatr Pulmonol. (2003) 35:34–41. doi: 10.1002/ppul.10219
32. Bogatkevich GS, Gustilo E, Oates JC, Feghali-Bostwick C, Harley RA, Silver RM, et al. Distinct PKC isoforms mediate cell survival and DNA synthesis in thrombin-induced myofibroblasts. Am J Physiol Lung Cell Mol Physiol. (2005) 288:L190–201. doi: 10.1152/ajplung.00448.2003
33. Howell DC, Goldsack NR, Marshall RP, McAnulty RJ, Starke R, Purdy G, et al. Direct thrombin inhibition reduces lung collagen, accumulation, and connective tissue growth factor mRNA levels in bleomycin-induced pulmonary fibrosis. Am J Pathol. (2001) 159:1383–95. doi: 10.1016/S0002-9440(10)62525-4
34. Chambers RC, Dabbagh K, McAnulty RJ, Gray AJ, Blanc-Brude OP, Laurent GJ. Thrombin stimulates fibroblast procollagen production via proteolytic activation of protease-activated receptor 1. Biochem J. (1998) 333(Pt 1):121–7. doi: 10.1042/bj3330121
35. Bargagli E, Madioni C, Bianchi N, Refini RM, Cappelli R, Rottoli P. Serum analysis of coagulation factors in IPF and NSIP. Inflammation. (2014) 37:10–6. doi: 10.1007/s10753-013-9706-z
36. Borensztajn K, Stiekema J, Nijmeijer S, Reitsma PH, Peppelenbosch MP, Spek CA. Factor Xa stimulates proinflammatory and profibrotic responses in fibroblasts via protease-activated receptor-2 activation. Am J Pathol. (2008) 172:309–20. doi: 10.2353/ajpath.2008.070347
37. Navaratnam V, Fogarty AW, McKeever T, Thompson N, Jenkins G, Johnson SR, et al. Presence of a prothrombotic state in people with idiopathic pulmonary fibrosis: a population-based case-control study. Thorax. (2014) 69:207–15. doi: 10.1136/thoraxjnl-2013-203740
38. Wujak L, Didiasova M, Zakrzewicz D, Frey H, Schaefer L, Wygrecka M. Heparan sulfate proteoglycans mediate factor XIIa binding to the cell surface. J Biol Chem. (2015) 290:7027–39. doi: 10.1074/jbc.M114.606343
39. Shi J, Hao JH, Ren WH, Zhu JR. Effects of heparin on liver fibrosis in patients with chronic hepatitis B. World J Gastroenterol. (2003) 9:1611–4. doi: 10.3748/wjg.v9.i7.1611
40. Dhar A, Tschotazis E, Brown R, Manousou P, Millson C, Aldersley M, et al. LP11 : warfarin anticoagulation for liver fibrosis in patients transplanted for hepatitis C (WAFT-C): results at one year. J Hepatol. (2015) 62:S268–9.
41. Cafolla A, Gentile G. Anticoagulant therapy with fondaparinux in a liver transplant patient with thrombosis and liver fibrosis: a case report. Clin Case Rep. (2017) 5:342–5. doi: 10.1002/ccr3.820
42. Villa E, Cammà C, Marietta M, Luongo M, Critelli R, Colopi S, et al. Enoxaparin prevents portal vein thrombosis and liver decompensation in patients with advanced cirrhosis. Gastroenterology. (2012) 143:1253–60.e4. doi: 10.1053/j.gastro.2012.07.018
43. Huang JS, Luo X, Yu JX, Liu W, Chen XW, Xie L, et al. [Indigenous and imported low molecular weight heparin in the treatment of chronic hepatitis B and cirrhosis with hepatitis B virus: a prospective randomized controlled clinical study]. Zhongguo Wei Zhong Bing Ji Jiu Yi Xue. (2007) 19:408–11.
44. Zhang R, Huang X, Jiang Y, Wang J, Chen S. Effects of anticoagulants on experimental models of established chronic liver diseases: a systematic review and meta-analysis. Can J Gastroenterol Hepatol. (2020) 2020:8887574. doi: 10.1155/2020/8887574
45. Basili S, Pastori D, Raparelli V, Violi F. Anticoagulant therapy in patients with liver cirrhosis and portal vein thrombosis: insights for the clinician. Therap Adv Gastroenterol. (2018) 11:1756284818793561. doi: 10.1177/1756284818793561
46. O’Leary JG, Greenberg CS, Patton HM, Caldwell SH. AGA clinical practice update: coagulation in cirrhosis. Gastroenterology. (2019) 157:34–43.e1. doi: 10.1053/j.gastro.2019.03.070
47. O’Shea RS, Davitkov P, Ko CW, Rajasekhar A, Su GL, Sultan S, et al. AGA clinical practice guideline on the management of coagulation disorders in patients with cirrhosis. Gastroenterology. (2021) 161:1615–27.e1. doi: 10.1053/j.gastro.2021.08.015
48. Pant A, Kopec AK, Luyendyk JP. Role of the blood coagulation cascade in hepatic fibrosis. Am J Physiol Gastrointest Liver Physiol. (2018) 315:G171–6. doi: 10.1152/ajpgi.00402.2017
49. Tripodi A, Anstee QM, Sogaard KK, Primignani M, Valla DC. Hypercoagulability in cirrhosis: causes and consequences. J Thromb Haemost. (2011) 9:1713–23. doi: 10.1111/j.1538-7836.2011.04429.x
50. Lisman T, Leebeek FW, de Groot PG. Haemostatic abnormalities in patients with liver disease. J Hepatol. (2002) 37:280–7. doi: 10.1016/s0168-8278(02)00199-x
51. Bitto N, Liguori E, La Mura V. Coagulation, microenvironment and liver fibrosis. Cells. (2018) 7:85. doi: 10.3390/cells7080085
52. Mahmoud NI, Messiha BAS, Abo-Saif AA, Abdel-Bakky MS. Inhibition of activated factor X; a new pathway in ameliorating carbon tetrachloride-induced liver fibrosis in rats. J Biochem Mol Toxicol. (2019) 33:e22287. doi: 10.1002/jbt.22287
53. Knight V, Lourensz D, Tchongue J, Correia J, Tipping P, Sievert W. Cytoplasmic domain of tissue factor promotes liver fibrosis in mice. World J Gastroenterol. (2017) 23:5692–9. doi: 10.3748/wjg.v23.i31.5692
54. Guo X, Kolpakov MA, Hooshdaran B, Schappell W, Wang T, Eguchi S, et al. Cardiac expression of factor X mediates cardiac hypertrophy and fibrosis in pressure overload. JACC Basic Transl Sci. (2020) 5:69–83. doi: 10.1016/j.jacbts.2019.10.006
55. Sabri A, Short J, Guo J, Steinberg SF. Protease-activated receptor-1-mediated DNA synthesis in cardiac fibroblast is via epidermal growth factor receptor transactivation: distinct PAR-1 signaling pathways in cardiac fibroblasts and cardiomyocytes. Circ Res. (2002) 91:532–9. doi: 10.1161/01.res.0000035242.96310.45
56. Antoniak S, Rojas M, Spring D, Bullard TA, Verrier ED, Blaxall BC, et al. Protease-activated receptor 2 deficiency reduces cardiac ischemia/reperfusion injury. Arterioscler Thromb Vasc Biol. (2010) 30:2136–42. doi: 10.1161/ATVBAHA.110.213280
57. Pawlinski R, Tencati M, Hampton CR, Shishido T, Bullard TA, Casey LM, et al. Protease-activated receptor-1 contributes to cardiac remodeling and hypertrophy. Circulation. (2007) 116:2298–306. doi: 10.1161/circulationaha.107.692764
58. Madhusudhan T, Kerlin BA, Isermann B. The emerging role of coagulation proteases in kidney disease. Nat Rev Nephrol. (2016) 12:94–109. doi: 10.1038/nrneph.2015.177
59. Wiggins RC, Njoku N, Sedor JR. Tissue factor production by cultured rat mesangial cells. Stimulation by TNF alpha and lipopolysaccharide. Kidney Int. (1990) 37:1281–5. doi: 10.1038/ki.1990.112
60. Ono T, Liu N, Kasuno K, Kusano H, Nogaki F, Kamata T, et al. Coagulation process proceeds on cultured human mesangial cells via expression of factor V. Kidney Int. (2001) 60:1009–17. doi: 10.1046/j.1523-1755.2001.0600031009.x
61. Sommeijer DW, Florquin S, Hoedemaker I, Timmerman JJ, Reitsma PH, Ten Cate H. Renal tissue factor expression is increased in streptozotocin-induced diabetic mice. Nephron Exp Nephrol. (2005) 101:e86–94. doi: 10.1159/000086646
62. Sevastos J, Kennedy SE, Davis DR, Sam M, Peake PW, Charlesworth JA, et al. Tissue factor deficiency and PAR-1 deficiency are protective against renal ischemia reperfusion injury. Blood. (2007) 109:577–83. doi: 10.1182/blood-2006-03-008870
63. Nomura K, Liu N, Nagai K, Hasegawa T, Kobayashi I, Nogaki F, et al. Roles of coagulation pathway and factor Xa in rat mesangioproliferative glomerulonephritis. Lab Invest. (2007) 87:150–60. doi: 10.1038/labinvest.3700502
64. Kurtulus S, Madi A, Escobar G, Klapholz M, Nyman J, Christian E, et al. Checkpoint blockade immunotherapy induces dynamic changes in PD-1- CD8+ tumor-infiltrating T cells. Immunity. (2019) 50:181–94.e6. doi: 10.1016/j.immuni.2018.11.014
65. Sörensen-Zender I, Rong S, Susnik N, Lange J, Gueler F, Degen JL, et al. Role of fibrinogen in acute ischemic kidney injury. Am J Physiol Renal Physiol. (2013) 305:F777–85. doi: 10.1152/ajprenal.00418.2012
66. Sumi A, Yamanaka-Hanada N, Bai F, Makino T, Mizukami H, Ono T. Roles of coagulation pathway and factor Xa in the progression of diabetic nephropathy in db/db mice. Biol Pharm Bull. (2011) 34:824–30. doi: 10.1248/bpb.34.824
67. Tillet S, Giraud S, Delpech PO, Thuillier R, Ameteau V, Goujon JM, et al. Kidney graft outcome using an anti-Xa therapeutic strategy in an experimental model of severe ischaemia-reperfusion injury. Br J Surg. (2015) 102:132–42; discussion 42. doi: 10.1002/bjs.9662
68. Xu Y, Zacharias U, Peraldi MN, He CJ, Lu C, Sraer JD, et al. Constitutive expression and modulation of the functional thrombin receptor in the human kidney. Am J Pathol. (1995) 146:101–10.
69. Adams MN, Ramachandran R, Yau MK, Suen JY, Fairlie DP, Hollenberg MD, et al. Structure, function and pathophysiology of protease activated receptors. Pharmacol Ther. (2011) 130:248–82. doi: 10.1016/j.pharmthera.2011.01.003
70. Hollenberg MD, Mihara K, Polley D, Suen JY, Han A, Fairlie DP, et al. Biased signalling and proteinase-activated receptors (PARs): targeting inflammatory disease. Br J Pharmacol. (2014) 171:1180–94. doi: 10.1111/bph.12544
71. Lin H, Liu AP, Smith TH, Trejo J. Cofactoring and dimerization of proteinase-activated receptors. Pharmacol Rev. (2013) 65:1198–213. doi: 10.1124/pr.111.004747
72. Gieseler F, Ungefroren H, Settmacher U, Hollenberg MD, Kaufmann R. Proteinase-activated receptors (PARs) - focus on receptor-receptor-interactions and their physiological and pathophysiological impact. Cell Commun Signal. (2013) 11:86. doi: 10.1186/1478-811X-11-86
73. Günther A, Lübke N, Ermert M, Schermuly RT, Weissmann N, Breithecker A, et al. Prevention of bleomycin-induced lung fibrosis by aerosolization of heparin or urokinase in rabbits. Am J Respir Crit Care Med. (2003) 168:1358–65. doi: 10.1164/rccm.2201082
74. Kijiyama N, Ueno H, Sugimoto I, Sasaguri Y, Yatera K, Kido M, et al. Intratracheal gene transfer of tissue factor pathway inhibitor attenuates pulmonary fibrosis. Biochem Biophys Res Commun. (2006) 339:1113–9. doi: 10.1016/j.bbrc.2005.11.127
75. Li CJ, Yang ZH, Shi XL, Liu DL. Effects of aspirin and enoxaparin in a rat model of liver fibrosis. World J Gastroenterol. (2017) 23:6412–9. doi: 10.3748/wjg.v23.i35.6412
76. Abe W, Ikejima K, Lang T, Okumura K, Enomoto N, Kitamura T, et al. Low molecular weight heparin prevents hepatic fibrogenesis caused by carbon tetrachloride in the rat. J Hepatol. (2007) 46:286–94. doi: 10.1016/j.jhep.2006.08.023
77. Busch S, Landberg G. CAF-specific markers: role of the TGFβ pathway. Oncoscience. (2015) 2:835–6. doi: 10.18632/oncoscience.209
78. Yan Y, Guan C, Du S, Zhu W, Ji Y, Su N, et al. Effects of enzymatically depolymerized low molecular weight heparins on CCl. Front Pharmacol. (2017) 8:514. doi: 10.3389/fphar.2017.00514
79. Abdel-Salam OM, Baiuomy AR, Ameen A, Hassan NS. A study of unfractionated and low molecular weight heparins in a model of cholestatic liver injury in the rat. Pharmacol Res. (2005) 51:59–67. doi: 10.1016/j.phrs.2004.04.009
80. Spronk HM, De Jong AM, Verheule S, De Boer HC, Maass AH, Lau DH, et al. Hypercoagulability causes atrial fibrosis and promotes atrial fibrillation. Eur Heart J. (2017) 38:38–50. doi: 10.1093/eurheartj/ehw119
81. Silver D, Kapsch DN, Tsoi EK. Heparin-induced thrombocytopenia, thrombosis, and hemorrhage. Ann Surg. (1983) 198:301–6.
82. Ageno W, Gallus AS, Wittkowsky A, Crowther M, Hylek EM, Palareti G. Oral anticoagulant therapy: antithrombotic therapy and prevention of thrombosis, 9th ed: American college of chest physicians evidence-based clinical practice guidelines. Chest. (2012) 141(2 Suppl.):e44S–88S. doi: 10.1378/chest.11-2292
83. Anstee QM, Goldin RD, Wright M, Martinelli A, Cox R, Thursz MR. Coagulation status modulates murine hepatic fibrogenesis: implications for the development of novel therapies. J Thromb Haemost. (2008) 6:1336–43. doi: 10.1111/j.1538-7836.2008.03015.x
84. Noth I, Anstrom KJ, Calvert SB, de Andrade J, Flaherty KR, Glazer C, et al. A placebo-controlled randomized trial of warfarin in idiopathic pulmonary fibrosis. Am J Respir Crit Care Med. (2012) 186:88–95. doi: 10.1164/rccm.201202-0314oc
85. Kubo H, Nakayama K, Yanai M, Suzuki T, Yamaya M, Watanabe M, et al. Anticoagulant therapy for idiopathic pulmonary fibrosis. Chest. (2005) 128:1475–82. doi: 10.1378/chest.128.3.1475
86. Butenas S. Tissue factor structure and function. Scientifica (Cairo). (2012) 2012:964862. doi: 10.6064/2012/964862
87. Siddiqui FA, Desai H, Amirkhosravi A, Amaya M, Francis JL. The presence and release of tissue factor from human platelets. Platelets. (2002) 13:247–53. doi: 10.1080/09537100220146398
88. Zillmann A, Luther T, Müller I, Kotzsch M, Spannagl M, Kauke T, et al. Platelet-associated tissue factor contributes to the collagen-triggered activation of blood coagulation. Biochem Biophys Res Commun. (2001) 281:603–9. doi: 10.1006/bbrc.2001.4399
89. Mackman N. Role of tissue factor in hemostasis, thrombosis, and vascular development. Arterioscler Thromb Vasc Biol. (2004) 24:1015–22. doi: 10.1161/01.ATV.0000130465.23430.74
90. Czajka P, Przybyłkowski A, Nowak A, Postula M, Wolska M, Mirowska-Guzel D, et al. Antiplatelet drugs and liver fibrosis. Platelets. (2021) 33:1–10. doi: 10.1080/09537104.2021.1883574
91. Schrör K. Aspirin and platelets: the antiplatelet action of aspirin and its role in thrombosis treatment and prophylaxis. Semin Thromb Hemost. (1997) 23:349–56. doi: 10.1055/s-2007-996108
92. Polzin A, Dannenberg L, Sansone R, Levkau B, Kelm M, Hohlfeld T, et al. Antiplatelet effects of aspirin in chronic kidney disease patients. J Thromb Haemost. (2016) 14:375–80. doi: 10.1111/jth.13211
93. Jia LX, Qi GM, Liu O, Li TT, Yang M, Cui W, et al. Inhibition of platelet activation by clopidogrel prevents hypertension-induced cardiac inflammation and fibrosis. Cardiovasc Drugs Ther. (2013) 27:521–30. doi: 10.1007/s10557-013-6471-z
94. Amin AM, Sheau Chin L, Azri Mohamed Noor D, Sk Abdul Kader MA, Kah Hay Y, Ibrahim B. The personalization of clopidogrel antiplatelet therapy: the role of integrative pharmacogenetics and pharmacometabolomics. Cardiol Res Pract. (2017) 2017:8062796. doi: 10.1155/2017/8062796
95. Mahmoud NI, Messiha BAS, Salehc IG, Abo-Saif AA, Abdel-Bakky MS. Interruption of platelets and thrombin function as a new approach against liver fibrosis induced experimentally in rats. Life Sci. (2019) 231:116522. doi: 10.1016/j.lfs.2019.05.078
96. Birnbaum Y, Tran D, Chen H, Nylander S, Sampaio LC, Ye Y. Ticagrelor improves remodeling, reduces apoptosis, inflammation and fibrosis and increases the number of progenitor stem cells after myocardial infarction in a rat model of ischemia reperfusion. Cell Physiol Biochem. (2019) 53:961–81. doi: 10.33594/000000189
97. Julia S, James U. Direct oral anticoagulants: a quick guide. Eur Cardiol. (2017) 12:40–5. doi: 10.15420/ecr.2017:11:2
98. Chen A, Stecker E, Warden BA. Direct oral anticoagulant use: a practical guide to common clinical challenges. J Am Heart Assoc. (2020) 9:e017559. doi: 10.1161/JAHA.120.017559
99. Kondo H, Abe I, Fukui A, Saito S, Miyoshi M, Aoki K, et al. Possible role of rivaroxaban in attenuating pressure-overload-induced atrial fibrosis and fibrillation. J Cardiol. (2018) 71:310–9. doi: 10.1016/j.jjcc.2017.08.007
100. Shi G, Yang X, Pan M, Sun J, Ke H, Zhang C, et al. Apixaban attenuates ischemia-induced myocardial fibrosis by inhibition of Gq/PKC signaling. Biochem Biophys Res Commun. (2018) 500:550–6. doi: 10.1016/j.bbrc.2018.04.071
101. Horinouchi Y, Ikeda Y, Fukushima K, Imanishi M, Hamano H, Izawa-Ishizawa Y, et al. Renoprotective effects of a factor Xa inhibitor: fusion of basic research and a database analysis. Sci Rep. (2018) 8:10858. doi: 10.1038/s41598-018-29008-2
102. Bogatkevich GS, Ludwicka-Bradley A, Nietert PJ, Akter T, van Ryn J, Silver RM. Antiinflammatory and antifibrotic effects of the oral direct thrombin inhibitor dabigatran etexilate in a murine model of interstitial lung disease. Arthritis Rheum. (2011) 63:1416–25. doi: 10.1002/art.30255
103. Bogatkevich GS, Ludwicka-Bradley A, Silver RM. Dabigatran, a direct thrombin inhibitor, demonstrates antifibrotic effects on lung fibroblasts. Arthritis Rheum. (2009) 60:3455–64. doi: 10.1002/art.24935
104. Dong A, Mueller P, Yang F, Yang L, Morris A, Smyth SS. Direct thrombin inhibition with dabigatran attenuates pressure overload-induced cardiac fibrosis and dysfunction in mice. Thromb Res. (2017) 159:58–64. doi: 10.1016/j.thromres.2017.09.016
105. Saifi MA, Annaldas S, Godugu C. A direct thrombin inhibitor, dabigatran etexilate protects from renal fibrosis by inhibiting protease activated receptor-1. Eur J Pharmacol. (2021) 893:173838. doi: 10.1016/j.ejphar.2020.173838
106. Noguchi D, Kuriyama N, Hibi T, Maeda K, Shinkai T, Gyoten K, et al. The impact of dabigatran treatment on sinusoidal protection against hepatic ischemia/reperfusion injury in mice. Liver Transpl. (2021) 27:363–84. doi: 10.1002/lt.25929
Keywords: fibrosis, coagulation, ECM, coagulation factors, hemostasis
Citation: Oh H, Park HE, Song MS, Kim H and Baek J-H (2022) The Therapeutic Potential of Anticoagulation in Organ Fibrosis. Front. Med. 9:866746. doi: 10.3389/fmed.2022.866746
Received: 31 January 2022; Accepted: 13 April 2022;
Published: 16 May 2022.
Edited by:
Peter Olinga, University of Groningen, NetherlandsReviewed by:
Eliane Pedra Dias, Fluminense Federal University, BrazilCopyright © 2022 Oh, Park, Song, Kim and Baek. This is an open-access article distributed under the terms of the Creative Commons Attribution License (CC BY). The use, distribution or reproduction in other forums is permitted, provided the original author(s) and the copyright owner(s) are credited and that the original publication in this journal is cited, in accordance with accepted academic practice. No use, distribution or reproduction is permitted which does not comply with these terms.
*Correspondence: Jea-Hyun Baek, amJhZWtAaGFuZG9uZy5lZHU=
†These authors have contributed equally to this work
Disclaimer: All claims expressed in this article are solely those of the authors and do not necessarily represent those of their affiliated organizations, or those of the publisher, the editors and the reviewers. Any product that may be evaluated in this article or claim that may be made by its manufacturer is not guaranteed or endorsed by the publisher.
Research integrity at Frontiers
Learn more about the work of our research integrity team to safeguard the quality of each article we publish.