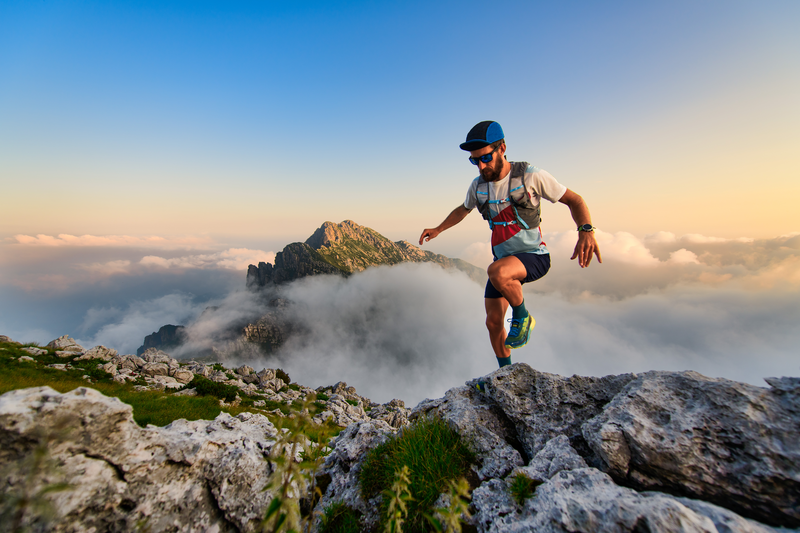
95% of researchers rate our articles as excellent or good
Learn more about the work of our research integrity team to safeguard the quality of each article we publish.
Find out more
ORIGINAL RESEARCH article
Front. Med. , 14 April 2022
Sec. Obstetrics and Gynecological Surgery
Volume 9 - 2022 | https://doi.org/10.3389/fmed.2022.857591
Context: Fetal Autonomic Nervous sysTem Evaluation (FANTE) is a non-invasive tool that evaluates the autonomic nervous system activity in a fetus. Autonomic nervous system maturation and development during prenatal life are pivotal for the survival and neuropsychiatric development of the baby.
Objective: Aim of the study is to evaluate the effect of music stimulation on fetal heart rate and specific parameters linked to ANS activity, in particular fetal heart rate variability.
Methods: Thirty-two women between the 32nd and 38th week with a singleton uncomplicated pregnancy were recruited. All FANTE data collections were acquired using a 10-derivation electrocardiograph placed on the maternal abdomen. In each session (5 min basal, 10 min with music stimulus, and 5 min post-stimulus), FANTE was registered. The music stimulus was “Clair de lune” Debussy, played through headphones on the mother’s abdomen (CTR: 31927).
Results: Music does not change the mean value of fetal heart rate. However, indices of total fetal heart rate variability statistically increase (RRsd p = 0.037, ANNsd p = 0.039, SD2 p = 0.019) during music stimulation in comparison to the basal phase. Heart rate variability increase depends mainly on the activation of parasympathetic branches (CVI p = 0.013), meanwhile, no significant changes from basal to stimulation phase were observed for indices of sympathetic activity. All the parameters of heart rate variability and parasympathetic activity remained activated in the post-stimulus phase compared to the stimulus phase. In the post-stimulus phase, sympathetic activity resulted in a significant reduction (LFn p = 0.037).
Conclusion: Music can influence the basal activity of the fetal autonomic nervous system, enhancing heart rate variability, without changing fetal heart rate mean value. Music is enabled to induce a relaxation state in a near-to-term fetus, mediated by parasympathetic activation and by a parallel sympathetic inhibition.
The autonomic nervous system (ANS) is a key homeostatic regulator both in prenatal and post-natal life (1). The main functions are regulated by the ANS: heart and flow rate, breathing rate, kidney, and gastroenteric function, and the secretion of the main endocrine glands (1, 2). These physiological functions depend on the degree of activation and maturation of the two main components of the ANS, namely the sympathetic and parasympathetic systems. The sympathetic system is responsible for the “fight-or-flight” response. Meanwhile, the parasympathetic nervous system is responsible for relaxation and moderating control over the sympathetic system, hypothalamic-pituitary-adrenal axis, and, when previous adaptive responses fail, it mediates the death-frightening behaviors as described by Porges in the “polyvagal theory” (2–4).
Fetal and neonatal wellbeing, as like fetal outcomes at birth, is strongly linked with ANS function. During fetal adaptation to postnatal life, ANS acts as the main regulator of the new-born cardiovascular and respiratory systems (2). At birth, both sympathetic activation and parasympathetic modulation play a fundamental role in increasing and stabilization of arterial blood pressure, heart rate, respiratory rate, and catecholamine level (2, 3, 5). Some of the most serious complications affecting new-born present an altered development of the ANS (6–8). When ANS activity is impaired, the risk of fetal death and sudden infant death outcomes increases (2, 8). In addition, children with such complications have a higher risk of developing chronic diseases (such as diabetes, hypertension, renal failure) (2, 9). Many studies underlined that ANS maturation, and, in particular, parasympathetic activation, seems to have a critical role in behavior, social communication, recognition, memory, mood, and adaptive responses to stress (2, 4, 10, 11). Then, we know that some autism spectrum disorders, rather than other severe diseases that afflict infants are often linked to impaired ANS development (12). This concept is of greater importance in preterm infants where altered ANS function may be compounded by immature development of the ANS itself and of the target organs (7).
For these reasons, it is important to identify markers and tools useful to evaluate and activate fetal ANS activity, to assess the neural maturity of a fetus, and to project clinical intervention about abnormal maturation of ANS management.
Several techniques have been studied to asset the quality of ANS activity in fetal life. Since the autonomic capacity is mirrored in cardiovascular regulation, a marker widely used for evaluating ANS activity is Heart Rate Variability (HRV) (1, 3, 9, 10, 13, 14). Analysis of HRV is used as a standard method for assessing ANS functions in adults. The association between HRV and autonomic function was first reported in the 1970s (15). HRV is an index of neurovisceral integration and organism self-regulation ability (16). Heart rate (HR) is mainly controlled by sinus node pacing. HRV represents the amount of HR fluctuation around the mean value of HR and is caused by continuous changes in the sympathetic/parasympathetic balance. In adulthood, HRV evaluation can be performed by statistical analysis (time-domain parameters), and spectral analysis (frequency-domain parameters) of R-R intervals obtained by ECG (17–20). Time-domain parameters quantify the amount of variability in R-R intervals, meanwhile, frequency-domain parameters estimate the distribution of absolute or relative power into the four specific frequency bands. HRV-related parameters can be extracted effectively from short-term electrocardiographic collection (5 min) (21, 22). N-N interval is another way of saying R-R interval, the letter N is used to distinguish that these statistics are intended to derive from “normal” R-R intervals, or R-R intervals which represent normal cardiac timing and are free from artifacts (23).
The evaluation of HRV is used as a predictor in a wide variety of disorders, like stroke, multiple sclerosis, end-stage renal disease, diabetes mellitus, ischemic heart disease, particularly myocardial infarction, cardiomyopathy, patients awaiting cardiac transplantation, valvular heart disease, and congestive heart failure (17–20).
Recently, some authors proposed fetal cardiomagnetography (fCMG) for the study of fetal ANS activity. The fMCG analysis allows obtaining time-domain and frequencies-domain parameters that reflect general maturation, adaptation, and organization of fetal ANS. Although, this method is quite expensive, not very usable in maternity care, and potentially dangerous if used routinely (1, 2, 24–26).
Our research group developed a non-invasive method of data collection of the fetal heart activity which could be considered equal to fCMG in providing useful data on ANS activity, called Fetal Autonomic Nervous sysTem Evaluation (FANTE) (27, 28). This technique consists of applying electrodes on the maternal abdomen to record joint maternal and fetal ECG signals. After data collection, fetal ECG signals are extracted and re-elaborated. This analysis allows the evaluation of time-domain and frequency-domain parameters related to ANS branches activity (27–29). With FANTE, we are able to assess the development of the ANS during fetal life, through the maternal abdomen, in an absolutely non-invasive, safe, and repeatable way. At the same time, it is possible to evaluate any changes in ANS activity after specific stimulations.
Acoustic stimulation and music have been used over the years in order to evaluate fetal and new-born well-being (30–34). Goodlin and Schimidt (35) first showed that fetuses with poor HR patterns that failed to respond to external sound were at increased risk for perinatal mortality or neurological impairment. Previous studies on anencephalic fetuses suggested that HR response to vibratory and acoustic stimulation is related to ANS activity (36, 37). Animal studies had revealed that a developed auditory apparatus is a necessary condition to obtain a fetal response (38–41). The human fetal auditory response begins about 26 weeks of gestation (30, 31, 34). Music played in the external environment is recognizable in utero (42). The ability of processing music involves a complex network of cortical and subcortical areas, and it has proved to be innate (34). Only around 32–33 weeks the maturation of the olivocochlear system allows relaying the information from the periphery to the cortex, for the elaboration of complex auditory stimuli such as music (43, 44).
Aim of this study was to determine the effect of music stimulation in normal fetuses from 32 to 38 weeks of gestation, through the analysis of temporal and frequency parameters related to ANS activity extracted from HR and HRV, recorded with the use of FANTE.
This study was carried out at the Gynecology and Obstetrics Department of the University of Pisa. From February to April 2021, we enrolled 32 pregnant women between the 32nd and 38th week of gestation. Women were enrolled during prenatal screening ultrasounds, and they carried a singleton and uncomplicated pregnancy. Exclusion criteria were multiple pregnancies, fetal malformations, drug abuse. Exit criteria were intrauterine fetal death, intrauterine restriction, and withdrawal of consent.
All participants received a FANTE data collection every week from the 32nd to the 38th week of gestation.
All FANTE data collections were acquired using a standard 10 derivation electrocardiograph (Cardioline S.p.A.) suitably modified. We used ten standard electrodes. The peripheral electrodes (Left, Right, and Foot) were placed on the maternal abdomen in a triangle around the navel (orange) with lower apex, while the precordial ones (C1, C2, C3, C4, C5, C6) were put on an external circle (Figure 1). The Neutral electrode was on the right side of the patient’s abdomen. The software was configured so that the system acquired and wrote on disk 8 signals (2 bipolar and 6 referenced to the centroid of the three L, R, and F leads) at 1,000 sps with a resolution of 0.763 uV. This high resolution is needed to resolve the very small voltage changes related to fetal cardiac activity.
Consistently with previous studies, the effect of the auditory stimulus on fetuses and newborns was verified using classic music (44–46). For this reason, we choose as music stimulus “Clair de lune,” Debussy from London Symphony Orchestra.” The music stimulus was played through headphones (stereo Headphones MDR-ZX110, Sony Corporation, Tokyo, Japan) placed on the mother’s abdomen to avoid maternal acoustic interferences (Figure 1).
In our study, we decided to apply a music stimulus with a sound intensity under the level consider detrimental (85 dB) to avoid any possible harmful effect on the fetuses (47).
Moreover, in a previous study by Gagnon et al. (36), the authors attribute part of the elicitation effect of acoustic stimulation on HR to the contextual activation of cutaneous vibratory and tactile nervous pathways mediated by Meissner’s corpuscles (80 dB). To avoid this confounding factor and we used an average sound intensity of the music stimulus of 50.8 dB (range 39–84 dB).
To all patients, FANTE multiple data collections were proposed. The data collections were performed in the same room, in supine position, without other acoustic interference.
In every session, we obtain a basal FANTE data collection (5 min), a stimulus FANTE data collection (10 min), and a subsequent post-stimulus FANTE data collection (5 min).
Fetal ECG extraction and subsequent analysis from FANTE were achieved with a dedicated analysis software based on an algorithm developed and tested by the group of IFC-CNR (28, 48, 49). The algorithm automatically extracts fetal ECG, starting from maternal multichannel abdominal signals even in critical conditions (noise, movement of the mother). This algorithm consists of finding the linear combination of the acquired ECGs, which maximizes a quality index that summarizes the pseudo-periodicity and temporal characteristics of the fetal QRS complex. From the extracted fetal ECG, the RR series of the fetus was calculated as the difference among adjacent QRSs. In this study, we applied a procedure to remove heartbeats of non-sinus origin and artifacts in the RR series as previously described by Billeci et al. (23).
From the initial sample of 32 mothers, 19 were excluded because their FANTE data collections were too noisy, and our method was unable to extract the fetal ECG and the obtained fetal RR series was meaningless. To exclude these cases from the analysis, we define a quality index. The Bad Index (BI) consider:
- the trimmed mean of the absolute successive differences of RR.
- the trimmed mean of the absolute successive second differences of RR.
- the ratio between the number of matched positions (of fetal QRS and maternal QRS) and the total number of QRS.
- a non-linear correction factor that takes accounts for the probability distribution of the mean fetal RR.
For this analysis, we consider FANTE data collection suitable for the analysis with a BI < 0.1 (threshold selected empirically). It is worth explaining that, at this gestational age, the execution of FANTE is achievable in 100% of cases. However, given the limited number of patients recruited, to obtain the best possible data quality, we included only FANTE data collection session in which BI < 0.1 in all three phases. This preliminary assessment led to the final sample of 13 mothers. Some of these mothers had multiple data collections in different gestational ages, leading to a sample of 20 signals.
The selected RR series was first screened for artifacts, uterine contractions, arrhythmias and then processed to compute temporal and frequency indices.
The following indices were extracted from the RR series:
- Time-domain features of HRV:
• fHRm: fetal HR mean.
• RRsd (ms): standard deviation of RR intervals, index of total HRV;
• ANNsd (ms): standard deviation of the Average NN intervals for each 5 min segment of a 24 h HRV registration, index of total HRV;
• SD1 (ms): standard deviation perpendicular to the line of the identity of Poincairè Plot; short term variability, mainly controlled by parasympathetic tone;
• SD2 (ms): standard deviation along the line of the identity of Poincairè Plot; long term variability, index of total HRV;
• CVI (ms2): log10 (SD2xSD1); Cardiac Vagal Index, the contribution of the parasympathetic nervous system to cardiac regulation.
- Frequency-domain features of HRV:
• Ptot (ms2): total power of fetal RR series, index of total HRV, mainly controlled by sympathetic tone;
• LFn: spectral power in the low-frequency band (0.04–0.15 Hz) normalized for the total power; low-frequency band mainly controlled by sympathetic tone;
• HFn: spectral power in the low-frequency band (0.15–0.4 Hz) normalized for the total power; low-frequency band mainly controlled by parasympathetic tone.
GraphPad Prism 7 (GraphPad Software Inc., San Diego, CA, United States) program was used for data analysis and graphic presentations.
The parameters assessed in women have been included in the table. Category variables have been represented as frequencies and percentages, while continuous variables were represented as averages, standard deviations (SD), and interquartile ranges.
The normality of the variables was determined using the Shapiro–Wilk normality test. In accordance, ordinary or repeated measures (RM) one-way ANOVA, followed by paired sample Tukey’s multiple comparisons test, was performed when comparing features extracted from fetal RR series in every session for each patient.
For all comparisons, the values of p < 0.05 were considered statistically significant.
This study was carried out in agreement with the recommendations of the Good Clinical Practice (ICH/GCP), Ministerial Decree of 1997. The protocol was approved by the Regional Ethics Committee for Clinical Trials, Tuscan North West Wide Area. All subjects gave written informed consent by the Declaration of Helsinki (clinical trial number 31927).
The demographic and clinical characteristics of the population are summarized in Table 1.
All women had a full-term delivery. No newborn needed neonatal resuscitation.
Music presents different effects on fetuses. The value of fHRm does not change during the stimulus phase and in the post-stimulus. However, fetal HRV statistically increases during the stimulus phase and this modification persists in the post-stimulus FANTE data collection (Figures 2A,B; Table 2).
Figure 2. Fetal heart rate mean (A) and heart rate variability, expressed ad RRsd (B) during FANTE data collection. pre, basal FANTE data collection; stimulus, stimulus FANTE data collection; post, post-stimulus FANTE data collection. *significant at the alpha level p < 0.05; **significant at the alpha level p < 0.01.
All the indices of the fetal heart variability significantly increase during the stimulus phase data collection compared to basal phase: RRsd (F = 6.71, p = 0.037, R2 = 0.26) (Figure 2B), ANNsd (F = 5.55, p = 0.036, R2 = 0.23), SD2 (F = 6.40, p = 0.039, R2 = 0.25), Ptot (F = 4.91, p = 0.019, R2 = 0.25) (Figures 3A–C). CVI, marker of parasympathetic activation, also statistically increases (F = 0.94, p = 0.013, R2 = 0.29) (Figure 3D) while other indices of parasympathetic activity (SD1 and HFn) do not change (Table 2).
Figure 3. The impact of musical stimulation on index-linked to ANS activity. (A) ANNsd (ms): standard deviation of the Average NN; (B) SD (ms): standard deviation perpendicular to the line of the identity of Poincairè Plot; short term variability, mainly controlled by parasympathetic tone; (C) Ptot (ms2): total power of fetal RR series, index of total HRV, mainly controlled by sympathetic tone; (D) CVI (ms2): Cardiac Vagal Index, the contribution of the parasympathetic nervous system to cardiac regulation; (E) LFn: low-frequency band (0.04–0.15 Hz) normalized for the total power; low-frequency band mainly controlled by sympathetic tone; pre: basal FANTE data collection; stimulus: stimulus FANTE data collection; post; post-stimulus FANTE data collection. *significant at the alpha level p < 0.05; **significant at the alpha level p < 0.01.
LFn, the low-frequency band mainly controlled by sympathetic tone, does not modify in the stimulus phases (F = 5.16, p = 0.88, R2 = 0.21) (Figure 3E).
All the parameters, which express ANS modulation of HRV, remain unchanged during the post-stimulus phase compared to stimulation phase: RRsd (p = 0.99) (Figure 2B), ANNsd (p = 0.832), SD2 (p = 0.997), Ptot (p = 0.346) (Figures 4A–C), but LFn. Instead, LFn (which expresses mainly the sympathetic tone) results in a significant reduction in the post-stimulus phase, in comparison to the stimulus phase (p = 0.037) (Figure 4E). CVI, controlled by parasympathetic tone, keeps being activated in the post-stimulus phase (p = 0.695) (Table 2).
Figure 4. Persistent short-term musical modulation of ANS activity. (A) ANNsd (ms): standard deviation of the Average NN; (B) SD (ms): standard deviation perpendicular to the line of the identity of Poincairè Plot; short term variability, mainly controlled by parasympathetic tone; (C) Ptot (ms2): total power of fetal RR series, index of total HRV, mainly controlled by sympathetic tone; (D) CVI (ms2): Cardiac Vagal Index, the contribution of the parasympathetic nervous system to cardiac regulation; (E) LFn: low-frequency band (0.04–0.15 Hz) normalized for the total power; low-frequency band mainly controlled by sympathetic tone; pre: basal FANTE data collection; stimulus: stimulus FANTE data collection; post; post-stimulus FANTE data collection. *significant at the alpha level p < 0.05; **significant at the alpha level p < 0.01.
Considering ANS signaling before and after music stimulation, we can note an important role of music as a stimulus of ANS activation. When the music stops, ANS modification persists in the following 5 min. Comparing ANS activity in the basal phase and the post-stimulus phase, the parameters showed a significant increase of the HRV (RRsd, ANNsd, Ptot) (Figures 2B, 4A–C) modulated by the increasing of parasympathetic activity (CVI) (Figure 4D) and by the parallel reduction of sympathetic tone (LFn) (Figure 4E; Table 2).
In this paper, we presented the effects of a short musical stimulus (10 min) on the activity of the fetal ANS, through the modulation of sympathetic and parasympathetic signaling. We registered this modulation through external sensors placed on the maternal abdomen as already shown in a previous work (24).
Music induces a modification of the parameters which regulate HRV (RRsd, ANNsd, Ptot), without a significant change in fetal heart rate.
This modulation persists as a short-term effect even after the music stops, for the entire length of the post-stimulus FANTE data collection (about 5 min). In our opinion, this demonstrates that music, at the intensity we used (mean 50.8 dB), determinates the activation of ANS without inducing a fight-or-flight reaction, sympathetically mediated, as a simple sound stimulus does. The music-dependent relaxation-state of the fetus is achieved by the increase of CVI through the activation parasympathetic system (2, 3).
It has been widely demonstrated that acoustic stimulation can influence HR activity (30–32, 36). Various external stimuli, and in particular vibratory and acoustic stimulation have been used over the years to evaluate fetal well-being (30–34). In the study conducted by Kisilevsky et al. (44), fetuses exposed to vibroacoustic stimulation at least 95 dB, showed a gradual heart rate acceleration. The same effect was observed by Al-Qahtani et al. (50) on fetuses between 37 and 40 weeks exposed to human voices higher than 105 dB. Those data were confident with the ones by Lecanuet et al. (51), in which term-fetuses responded to acoustic stimulus by increasing the ratios and amplitudes of accelerations and reducing deceleration ratios. Notably, the authors highlight that acoustic stimulus with lower sound intensity could elicit cardiac decelerations in near-to-term fetuses. This bimodal effect of acoustic stimulation has been considered by the authors as an expression of the defense and orientating response described in Sokolov’s model of information processing influenced by autonomic feedback.
Defense response is typically elicited by high-intensity stimulus and it determinates a sympathetic activation, meanwhile orienting response is activated by low-intensity stimulus and it reflexes a parasympathetic activation. Based on Sokolov’s model, those two responses were related to cardiac change (52).
In our study, the application of a music stimulus did not change the value of fetal heart rate. In accord with our results, D’Elia et al. (30) did not observe a change in fetal heart rate between 36 and 42 weeks after a vibroacoustic stimulation using an artificial larynx with an averaged sound intensity level of 68 dB. However, the low intensity of the music we used is not irrelevant. This stimulus is decoded by the fetal ANS and it determines a re-modulation of parasympathetic tone, in terms of increase, and of sympathetic tone, in terms of decrease. In particular, other studies using classic music showed a distressed effect of this type of stimuli on the ANS in the fetus, by using cardiotocography (45), or in newborns (46, 53). Therefore, this type of music seems to have a relaxing role for the fetal ANS, mediated both by a parasympathetic and sympathetic system, without determining a modification of the fHRm.
In the study conducted by Lecanuet et al. (54), the author observed that the magnitude of fetal cardiac and motor response to an acoustic stimulus was related to the basal HRV pattern. In our data, we did not observe any relation between the basal value of HRV indices (RRsd, ANNsd, SD2, Ptot), parasympathetic index (CVI), and their increase during the stimulus phase. We can only hypothesize that the effect of music on fetal ANS is not influenced by fetal behavioral state and does not depend on ANS basal tone.
From a translational point of view, the demonstration of the efficacy of an external stimulus in modulating the ANS signal is innovative and very promising, especially in those cases of incomplete development of the ANS.
For the first time, we demonstrated that, with music, it is possible to modulate fetal ANS activity in the short term, opening potentially significant scenarios from a therapeutic point of view. For example, in pregnant women with threatened preterm labor (where it is expected the birth of infants with delayed development of the ANS) rather than in term fetuses with potential risks of associated diseases (fetal growth restriction, preeclampsia, etc.), the use of music in the prenatal period, through ad hoc stimulation, could help the activation of the ANS and therefore its development.
On the other hand, we demonstrated music determines an early modulation of the whole autonomic activity, although, with our data, it is still not possible to verify if these modulating effects determine a long-term modification of fetal ANS basal activity.
The missing data about the possible long-term effect of music on the fetus is the real point of the weakness of our study. We were not able to demonstrate if this stimulus could have a real and positive effect on fetal ANS. This needs further investigation to clarify if we can long-term modulate fetal development and wellness with acoustic stimulus, perhaps repeated several times, at different time points of the pregnancy.
Another limitation of the study is the small sample size. In our previous article, we have been able to retrieve fetal ECG signals in the 62.5% of data collections. In the present study, the efficiency of the FANTE data collection was a little bit lower, since from 32 weeks of gestation to term, the presence of vernix caseosa can limit the possibility of fetal ECG signal extraction (27). Moreover, our decision to consider only FANTE data collection sessions in which BI < 0.1 in all three phases to obtain high-quality data further reduced the sample size. Finally, as described, our study aimed to verify the effect of music on near to term fetuses, hypothetically healthy. This was decided to avoid possible interfering factors related to fetal or maternal diseases which could alter ANS response in some way. Therefore our data are strictly linked to the wellness of the fetus. We have no data on “complicated” pregnancies and it is not possible to hypothesize beneficial effects in this type of patient.
This study concludes that music can modulate fetal ANS basal tone in the short term, enhancing HRV, without changing fHRm. In particular, music enables to induce a relaxation state in a near-to-term fetus, mostly mediated by parasympathetic activation.
The raw data supporting the conclusions of this article will be made available by the authors, without undue reservation.
The studies involving human participants were reviewed and approved by the Regional Ethics Committee for Clinical Trials, Tuscan North West Wide Area. The patients/participants provided their written informed consent to participate in this study.
PM, LB, and MV conceived and designed the study and coordinated the project. FM, AC, and GI performed the study and collected the data. LB and MV were responsible for the formal analysis. FM and MM-G were responsible for the data curation and provided the support in data analysis and visualization of results. PM, LB, and FM were involved in the interpretation of the data. FM prepared the first draft of the manuscript. PM, LB, MV, and TS revised it critically. All authors have given their approval for this version to be published.
The authors declare that the research was conducted in the absence of any commercial or financial relationships that could be construed as a potential conflict of interest.
All claims expressed in this article are solely those of the authors and do not necessarily represent those of their affiliated organizations, or those of the publisher, the editors and the reviewers. Any product that may be evaluated in this article, or claim that may be made by its manufacturer, is not guaranteed or endorsed by the publisher.
1. Schneider U, Bode F, Schmidt A, Nowack S, Rudolph A, Doelcker EM, et al. Developmental milestones of the autonomic nervous system revealed via longitudinal monitoring of fetal heart rate variability. PLoS One. (2018) 13:e0200799. doi: 10.1371/journal.pone.0200799
2. Mulkey SB, Plessis AD. The critical role of the central autonomic nervous system in fe-tal-neonatal transition. Semin Pediatr Neurol. (2018) 28:29–37. doi: 10.1016/j.spen.2018.05.004
3. Schlatterer SD, Plessis AJ. Exposures influencing the developing central autonomic nervous system. Birth Defect Res. (2020) 113:845–63. doi: 10.1002/bdr2.1847
4. Porges SW. The polyvagal theory: new insights into adaptive reactions of the autonomic nervous system. CCJM. (2009) 76(Suppl. 2):S86–90. doi: 10.3949/ccjm.76.s2.17
5. Segar JL. Ontogeny of the arterial and cardiopulmonary baroreflex during fetal and postnatal life. Am J Physiol. (1997) 273:R457–71. doi: 10.1152/ajpregu.1997.273.2.R457
6. Meister AL, Doheny KK, Travagli RA. Necrotizing enterocolitis: it’s not all in the gut. Exp Biol Med. (2020) 245:85–95. doi: 10.1177/1535370219891971
7. Gauda EB, McLemore GL. Premature birth, homeostatic plasticity and respiratory conse-quences of inflammation. Respir Physiol Neurobiol. (2020) 274:103337. doi: 10.1016/j.resp.2019.103337
8. Myers MM, Burtchen N, Retamar MO, Lucchini M, Fifer WP. Neonatal monitoring: predic-tion of autonomic regulation at 1 month from newborn assessments. In: Duncan JR, Byard RW editors. SIDS Sudden Infant and Early Childhood Death: The Past, the Present and the Future. (Adelaide, SA: University of Adelaide Press) (2018).
9. Lees T, Shad-Kaneez F, Simpson AM, Nassif NT, Lin Y, Lal S. Heart rate variability as a biomarker for predicting stroke, post-stroke complications and functionality. Biomark Insights. (2018) 13:117727191878693. doi: 10.1177/1177271918786931
10. Mulkey SB, du Plessis AJ. Autonomic nervous system development and its impact on neuro-psychiatric outcome. Pediatr Res. (2019) 85:120–6. doi: 10.1038/s41390-018-0155-0
11. Porges SW, Furman SA. The early development of the autonomic nervous system provides a neural platform for social behaviour: a polyvagal perspective. Infant Child Dev. (2011) 20:106–18. doi: 10.1002/icd.688
12. Billeci L, Tonacci A, Narzisi A, Manigrasso Z, Varanini M, Fulceri F, et al. Heart rate varia-bility during a joint attention task in toddlers with autism spectrum disorders. Front Physiol. (2018) 9:467. doi: 10.3389/fphys.2018.00467
13. Lange S, Van Leeuwen P, Geue D, Hatzmann W, Grönemeyer D. Influence of gestational age, heart rate, gender and time of day on fetal heart rate variability. Med Biol Eng Comput. (2005) 43:481–6. doi: 10.1007/BF02344729
14. Ernst G. Heart rate variability-more than heart beats? Front Public Health. (2017) 5:240. doi: 10.3389/fpubh.2017.00240
16. Thayer JF, Lane RD. A model of neurovisceral integration in emotion regulation and dysregu-lation. J Affect Disord. (2000) 61:201–16. doi: 10.1016/s0165-0327(00)00338-4
19. Kleiger RE, Stein PK, Bigger JT. Heart rate variability: measurement and clinical utility. Ann Noninvasive Electrocardiol. (2005) 10:88–101. doi: 10.1111/j.1542-474X.2005.10101.x
20. Drury R, Ginsberg JP, Porges SW, Thayer JF. Heart rate variability, health and well-being: a systems perspective. Front Public Health. (2020) 7:323. doi: 10.3389/fpubh.2019.00323
21. Shaffer F, Ginsberg JP. An overview of heart rate variability metrics and norms. Front Public Health. (2017) 5:258. doi: 10.3389/fpubh.2017.0025
22. Malik M. Heart rate variability: standards of measurement, physiological interpretation, and clinical use. Circulation. (1996) 93:1043–65. doi: 10.1161/01.cir.93.5.1043
23. Billeci L, Marino D, Insana L, Vatti G, Varanini M. Patient-specific seizure prediction based on heart rate variability and recurrence quantification analysis. PLoS One. (2018) 13:e0204339. doi: 10.1371/journal.pone.0204339
24. Seliger G, Stenzel A, Kowalski EM, Hoyer D, Nowack S, Seeger S, et al. Evaluation of standardized, computerized Dawes/Redman heart-rate analysis based on different recording methods and in relation to fetal beat-to-beat heart rate variability. J Perinat Med. (2016) 44:785–92. doi: 10.1515/jpm-2015-016
25. Haraldsdottir K, Watson AM, Goss KN, Beshish AG, Pegelow DF, Palta M, et al. Impaired autonomic function in adolescents born preterm. Physiol Rep. (2018) 6:e13620. doi: 10.14814/phy2.13620
26. Neilson JP. Fetal electrocardiogram (ECG) for fetal monitoring during labour. Cochrane Database Syst Rev. (2015) 2015:CD000116.
27. Mannella P, Billeci L, Giannini A, Canu A, Pancetti F, Simoncini T, et al. A feasibility study on non-invasive fetal ECG to evaluate prenatal autonomic nervous system activity. Eur J Obstet Gynecol Reprod Biol. (2020) 246:60–6. doi: 10.1016/j.ejogrb.2020.01.015
28. Varanini M, Tartarisco G, Billeci L, Macerata A, Pioggia G, Balocchi R. An efficient unsu-pervised fetal QRS complex detection from abdominal maternal ECG. Physiol Meas. (2014) 35:1607–19. doi: 10.1088/0967-3334/35/8/1607
29. Li R, Frasch MG, Wu HT. Efficient fetal-maternal ECG signal separation from two chan-nel maternal abdominal ECG via diffusion-based channel selection. Front Physiol. (2017) 8:277. doi: 10.3389/fphys.2017.00277
30. D’Elia A, Pighetti M, Vanacore F, Fabbrocini G, Arpaia L. Vibroacoustic stimulation in normal term human pregnancy. Early Hum Dev. (2005) 81:449–53. doi: 10.1016/j.earlhumdev.2004.04.017
31. Kisilevsky BS, Low JA. Human fetal behavior: 100 years of study. Dev Rev. (1998) 18:1–29. doi: 10.1006/drev.1998.0452
32. Gerhardt KJ, Abrams RM. Fetal exposures to sound and vibroacoustic stimulation. J Perinatol. (2000) 20:S21–30. doi: 10.1038/sj.jp.7200446
33. Pinette MG, Blackstone J, Wax JR, Cartin A. Using fetal acoustic stimulation to shorten the biophysical profile. J Clin Ultrasound. (2005) 33:223–5. doi: 10.1002/jcu.20116
34. Chorna O, Filippa M, De Almeida JS, Lordier L, Monaci MG, Hüppi P, et al. Neuroprocessing mechanisms of music during fetal and neonatal development: a role in neuroplasticity and neurodevelopment. Neural Plast. (2019) 2019:3972918. doi: 10.1155/2019/3972918
35. Goodlin RC, Schmidt W. Human fetal arousal as indicated by heart rate recordings. Am J Obstet Gynecol. (1972) 114:613–21. doi: 10.1016/0002-9378(72)90838-1
36. Gagnon R, Hunse C, Carmichael L, Fellows F, Patrick J. Human fetal responses to vibrator acoustic stimulation from twenty-six weeks to term. Am J Obstet Gynecol. (1987) 157:1375–81. doi: 10.1016/s0002-9378(87)80227-2
37. Ohel G, Simon A, Linder N, Mor-Yosef S. Anencephaly and the nature of fetal response to vibroacoustic stimulation. Am J Perinatol. (1986) 3:345–6. doi: 10.1055/s-2007-999894
38. Parkes MJ, Moore PJ, Moore DR, Fisk NM, Hanson MA. Behavioral changes in fetal sheep caused by vibroacoustic stimulation: the effects of cochlear ablation. Am J Obstet Gynecol. (1991) 164:1336–43. doi: 10.1016/0002-9378(91)90710-9
39. Gerhardt KJ. Characteristics of the fetal sheep sound environment. Semin Perinatol. (1989) 13:362–70.
40. Lecanuet JP, Graniere-Deferre C, Jacquet AY, DeCasper AJ. Fetal discrimination of low-pitched musical notes. Dev Psychobiol. (2000) 36:29–39. doi: 10.1002/(sici)1098-2302(200001)36:1<29::aid-dev4>3.0.co;2-j
41. Kisilevsky BS, Muir DW, Low JA. Human fetal responses to sound as a function of stimu-lus intensity. Am J Obstet Gynecol. (1989) 73:971–6. doi: 10.1097/00006250-198906000-00013
42. Querleu D, Renard X, Boutteville C, Crepin G. Hearing by the human fetus? Semin Perinatol. (1989) 13:409–20.
43. Jardri R, Pins D, Houfflin-Debarge V, Chaffiotte C, Rocourt N, Pruvo JP, et al. Fetal cortical activation to sound at 33 weeks of gestation: a functional MRI study. Neuroimage. (2008) 42:10–8. doi: 10.1016/j.neuroimage.2008.04.247
44. Kisilevsky BS, Hains SMJ, Jacquet AY, Granier-Deferre C, Lecanuet JP. Maturation of fetal responses to music. Dev Sci. (2004) 7:550–9. doi: 10.1111/j.1467-7687.2004.00379.x
45. Gebuza G, Zaleska M, Kaźmierczak M, Mieczkowska E, Gierszewska M. The effect of music on the cardiac activity of a fetus in a cardiotocographic examination. Adv Clin Exp Med. (2018) 27:615–21. doi: 10.17219/acem/68693
46. Amini E, Rafiei P, Zarei K, Gohari M, Hamidi M. Effect of lullaby and classical music on physiologic stability of hospitalized preterm infants: a randomized trial. J Neonatal Perinat Med. (2013) 6:295–301. doi: 10.3233/NPM-1371313
47. Lalande NM, Hétu R, Lambert J. Is occupational noise exposure during pregnancy a risk factor of damage to the auditory system of the fetus? Am J Ind Med. (1986) 10:427–35. doi: 10.1002/ajim.4700100410
48. Billeci L, Varanini MA. Combined independent source separation and quality index optimi-zation method for fetal ECG extraction from abdominal maternal leads. Sensors. (2017) 17:1135. doi: 10.3390/s17051135
49. Varanini M, Tartarisco G, Balocchi R, Macerata A, Pioggia G, Billeci L. A new method for QRS complex detection in multichannel ECG: application to self-monitoring of fetal health. Comput Biol Med. (2017) 85:125–34. doi: 10.1016/j.compbiomed.2016.04.008
50. Al-Qahtani NH. Foetal response to music and voice. Aust N Z J Obstet Gynecol. (2005) 45:414–7. doi: 10.1111/j.1479-828X.2005.00458.x
51. Lecanuet JP, Granier-Deferre C, Busnel MC. Fetal cardiac and motor responses to octave-band noises as a function of central frequency, intensity and heart rate variability. Early Hum Dev. (1988) 18:81–93. doi: 10.1016/0378-3782(88)90045-x
52. Graham FK, Clifton RK. Heart-rate change as a component of the orienting response. Psychol Bull. (1966) 65:305–20. doi: 10.1037/h0023258
53. Loewy J, Stewart K, Dassler AM, Telsey A, Homel P. The effects of music therapy on vital signs, feeding, and sleep in premature infants. Pediatrics. (2013) 131:902–18. doi: 10.1542/peds.2012-1367
Keywords: fetal autonomic nervous system, autonomic nervous system evaluation, fetal nervous system, heart rate variability (HRV), musical stimulation, non-invasive evaluation
Citation: Massimello F, Billeci L, Canu A, Montt-Guevara MM, Impastato G, Varanini M, Giannini A, Simoncini T and Mannella P (2022) Music Modulates Autonomic Nervous System Activity in Human Fetuses. Front. Med. 9:857591. doi: 10.3389/fmed.2022.857591
Received: 18 January 2022; Accepted: 30 March 2022;
Published: 14 April 2022.
Edited by:
Gabriele Saccone, Federico II University Hospital, ItalyReviewed by:
Manuela Filippa, Université de Genève, SwitzerlandCopyright © 2022 Massimello, Billeci, Canu, Montt-Guevara, Impastato, Varanini, Giannini, Simoncini and Mannella. This is an open-access article distributed under the terms of the Creative Commons Attribution License (CC BY). The use, distribution or reproduction in other forums is permitted, provided the original author(s) and the copyright owner(s) are credited and that the original publication in this journal is cited, in accordance with accepted academic practice. No use, distribution or reproduction is permitted which does not comply with these terms.
*Correspondence: Paolo Mannella, cGFvbG8ubWFubmVsbGFAdW5pcGkuaXQ=
†These authors share first authorship
Disclaimer: All claims expressed in this article are solely those of the authors and do not necessarily represent those of their affiliated organizations, or those of the publisher, the editors and the reviewers. Any product that may be evaluated in this article or claim that may be made by its manufacturer is not guaranteed or endorsed by the publisher.
Research integrity at Frontiers
Learn more about the work of our research integrity team to safeguard the quality of each article we publish.