- 1Faculty of Chemical-Biological Sciences, Universidad Autónoma de Guerrero, Chilpancingo, Mexico
- 2Department of Parasitology, Escuela Nacional de Ciencias Biológicas, Instituto Politécnico Nacional, Mexico City, Mexico
- 3Department of Internal Medicine, Hospital General Dr. Raymundo Abarca Alarcón, Chilpancingo, Mexico
- 4Center for Research in Infectious Diseases, Instituto Nacional de Enfermedades Respiratorias Ismael Cosío Villegas, Mexico City, Mexico
- 5HLA Laboratory, Instituto Nacional de Enfermedades Respiratorias Ismael Cosío Villegas, Mexico City, Mexico
Protozoa, nematodes, and platyhelminths are of clinical interest due to their role on the modulation of the immune responses. To determine the frequency of infection by intestinal parasites as well as the status of single or mixed infection (coinfection) and its relation with inflammation and intestinal permeability markers in patients with rheumatoid arthritis (RA), a cross-sectional study was conducted in 18 women diagnosed with RA. A fecal sample of each participant was analyzed for parasitic identification. The DAS28-erythrocyte sedimentation rate score, as well as the serum levels of TNF-α, IL-10, IL-17A, and the intestinal fatty-acid binding protein 2 (IFABP2), was determined through the ELISA technique. The T CD4+ and CD8+ lymphocytes' proportions were determined by flow cytometry. In this study, 50% (n = 9) of the total sample tested were positive to the presence of intestinal protozoa (27% by single infection and 22.2% by coinfection). Blastocystis sp. and Endolimax nana were the most frequently identified protozoa. The serum levels of IFABP2 were increased in patients with infection by protozoa, mainly in those individuals with coinfection and a larger abundance of Blastocystis sp. We found that coinfection by protozoa was related to higher levels of TNF-α and higher frequency of T CD4+ lymphocytes, mainly in patients under antirheumatic treatment. Infection by intestinal protozoa is associated with increased intestinal permeability in patients with RA; thus, infection, coinfection, and abundance of intestinal protozoa should be clinically screened because they could be an associated factor to the clinical variability of the disease.
Introduction
Elements of the intestinal microbiota such as protozoa, nematodes, and platyhelminths are of great clinical interest due to their role in modulating the immune response and as potential triggers of the autoimmune process in rheumatoid arthritis (RA) (1–3). In the last few years, it has been revealed that intestinal dysbiosis has an important physiopathological function in the development of RA, as well as in the clinical manifestation of the disease and, potentially, in the response to antirheumatic treatment (4–7). Interestingly, it has been previously described that the composition of gut microbiota could influence the modulation and progression of parasitic infections (8), and at the same time, infection by intestinal parasites could promote intestinal dysbiosis (9, 10).
In patients with RA, several studies have reported that infections by protozoa (11–20) and by nematodes (16, 21, 22) are related to the development of inflammatory syndromes. The infection by enteric pathogens such as protozoa has an effect on the regulation of genes that are related to the stress response and cell proliferation, thus promoting the chemokines' synthesis and inflammation (23). On the other hand, some helminth products such as glycoprotein ES-62 decrease the damage of intestinal barrier in RA (24) and have anti-inflammatory effects by modulating the Th1 and Th17 immune responses (25), as well as the cell infiltration in joints, the reactivity of effector B cells, and the restoration of IL-10-producing B cells (26).
It has been demonstrated that the infection by parasites such as Blastocystis sp., Giardia sp., Cryptosporidium sp., Trichuris sp., Entamoeba histolytica, Nippostrongylus brasiliensis, and Heligmosomoides polygyrus could modulate the intrinsic and extrinsic apoptosis pathways in intestinal epithelial cells in the host (27), increasing the intestinal epithelial permeability and the bacterial translocation due to the loss of adherents junctions (E-cadherin and α-catenin) (28) and tight junctions [occludin, claudin 3, claudin 4, and zonula occludens-1 (ZO-1)] that maintain the enterocytes' paracellular integrity (28–32). Different molecules have been considered useful during the evaluation of intestinal permeability. ZO-1 has been considered an intestinal barrier functionality and integrity marker, mainly in the early stages of autoimmune diseases' development (33) and in the progression of chronic inflammatory disorders (34). Other markers such as D-dimer, a-glutathione S-transferase, and the intestinal fatty-acid binding protein 2 (IFABP2) have been related to the presence of intestinal mucosa's damage (35). IFABP2 is a 15-kDa cytosolic protein present in the epithelial cells of the small and large intestines, particularly in the duodenum, jejunum, ileum, and colon (36). IFABP2 function in the intestinal enterocytes is promoting their energetic homeostasis (37) and transporting long-chain fatty acids (38). It has been reported that the levels of expression of IFABP2 represents a marker for intestinal lesions (39, 40). Cascais-Figueiredo et al. (41) reported that the levels of IFABP2 are increased during Giardiasis, showing the potential relation between infections by protozoa with increased intestinal permeability. The present study has the objective to determine the frequency of intestinal parasitosis in its single or mixed infection status, and its relationship with serum levels of IFABP2 and inflammation markers in a group of women from southern Mexico who have been diagnosed with RA.
Materials and Methods
Patient's Selection
A cross-sectional pilot study was conducted on 18 women diagnosed with RA according to the 2010 American College of Rheumatology (ACR) and the European League Against Rheumatism (EULAR) criteria (42). They were diagnosed and treated at the Rheumatology Department of the General Hospital in Chilpancingo, Mexico. The recruitment of the patients was performed from March to November of 2019. All patients were surveyed to obtain sociodemographic data. Patients diagnosed with peptic ulcer and inflammatory bowel diseases were also excluded, as well as those with gastrointestinal surgeries (e.g., gastrectomy, bariatric surgery, and colectomy). Moreover, we considered as an exclusion criteria having had antiparasitic treatment at least 6 months before taking the sample. The clinical and treatment characteristics were evaluated during the consultation and from the clinical file. All patients agreed to participate and gave their informed consent in writing. This study was approved by the Ethics and Research Committee of the University Autonomous of Guerrero (Project identification code: CB-004/2017).
Patients with an overlapping syndrome (characteristics of two autoimmune connective tissue diseases present in the same patient), chronic viral infection (hepatitis C or B virus or human immunodeficiency virus), or acute bacterial, viral, or fungal infections were excluded from the study.
Clinical Assessment and Laboratory Measurements
Disease activity and functional disability were evaluated through the Disease Activity Score 28 (DAS28) and a Spanish version of the Health Assessment Questionnaire (HAQ-DI) by a rheumatologist. The blood samples were collected after 8 h of overnight fast. The samples in EDTA tubes were used to evaluate hematologic parameters (Coulter AcT 5diff Hematologic Analyzer, Beckman Coulter, USA) and the erythrocyte sedimentation rate (ESR). Levels of C-reactive protein (CRP) and rheumatoid factor (RF) (Othoclinical Diagnostics, VITROS®5600, USA) were also assessed according to the manufacturer's instructions.
Parasitological Assessment
The stool samples were collected from patients and placed into plastic vials without preservatives. The stool samples were microscopically analyzed for the detection of parasitic elements by directs fecal smears: saline and iodine wet-mount preparations and the concentration by sedimentation method according to the procedures recommended by Pan American Health Organization/World Health Organization (PAHO/WHO) (43) and Clinical and Laboratory Standards Institute (CLSI) (44), the abundance scale was defined according to quantitate protozoa as follows: Number/10 Oil Immersion Fields (1000×); scarce ≤2; moderate 3–9, and abundant, ≥10. The parasitic elements were identified using the atlas of human parasitology (45) and the bench aids for the diagnosis of intestinal parasites by PAHO/WHO (43).
Coprological Analysis
The pH was measured following the technique described by Manzano and Nogueda (46). Briefly, the samples diluted 1:2 with physiological saline were centrifuged at 500 ×g for 10 min. The pH test strips, 0–14 pH (Merck®), were introduced into the supernatant and compared, as soon as possible, with the supplier's reference color table.
The presence of mucus, Charcot–Leyden crystals, fatty acids, soaps, and undigested foods (starches, meat, and vegetable fibers) was determined by observing fecal smears stained with Lugol. The presence of neutral fats was determined with the Sudan III stain (46, 47).
Serum Quantification of IFABP2 and Cytokines (TNF-α, IL-10, and IL-17A)
The IFABP2 serum levels were determined using ELISA kits (Sigma-Aldrich, USA), following the manufacturer's instructions. The results were expressed as ng/ml, and the detection limit was 0.025 ng/ml. Similarly, TNF-α levels were quantified using ELISA kits (Invitrogen, Thermo Fisher Scientific, Vienna, Austria). IL-10 and IL-17A were quantified using a ProcartaPlex assay (Invitrogen, Thermo Fisher Scientific, Vienna, Austria), following the specifications from the manufacturers. Cytokine levels are expressed in pg/ml.
Separation of PBMCs and Flow Cytometry
Peripheral blood mononuclear cells (PBMCs) were separated by Ficoll density gradient (Axis-Shield, Oslo, Norway) and cryopreserved in freezing medium (10% DMSO, 90% fetal calf serum; Lonza, CA, USA) in liquid nitrogen until used. PBMCs were thawed, washed twice with phosphate-buffered saline (PBS, Lonza, CA, USA), and stained at room temperature for 15 min with the following antibodies: BV570-CD3 (clone UCHT1), APC-Cy7-CD4 (clone A161A1), and Alexa Fluor 700-CD8 (clone RPA-T8) (BD Biosciences, San Jose, CA, USA). Viability was determined using live/dead aqua fluorescent reactive dye (Thermo Fisher Scientific, CA, USA). Cells were washed and fixed in 300 μl of 1% paraformaldehyde (Sigma-Aldrich, St. Louis, MO, USA) and acquired immediately in a FACS Symphony cytometer (BD, Biosciences). Fluorescence minus one stained tubes were used as gating controls. Data were analyzed using FlowJo v.10 software (FlowJo LLC, Ashland, OR, USA) (Supplementary Figure S1).
Statistical Analysis
Statistical analysis was carried out using Stata v.14.0 (StataCorp, College Station, TX, USA) and GraphPad Prism v.8.0 (GraphPad Software, San Diego, CA, USA) for Windows. Categorical data were expressed as numbers and proportions, and they were compared using the Chi-squared test. Continuous variables were compared among groups by Mann–Whitney U Test. The p <0.05 indicated statistical significance.
Results
Demographic Data and Clinical Features According to Protozoa Infection Status
Eighteen women diagnosed with RA were included in this study. The median age of the participants was 46.5 years old. According to the DAS28-ESR score, the population presents moderate clinical activity. The 66.7% (n = 12) of the individuals had pharmacological prescriptions with disease-modifying antirheumatic drugs (DMARDs), and 33.3% (n = 6) were recently diagnosed and provided biological samples before the beginning of their prescribed treatment. When compared to the clinical characteristics and laboratory parameters according to the presence of protozoa, there were no significant differences identified (Table 1). We found that none of the fecal samples were positive for nematodes or platyhelminths; only protozoa were identified (Figure 1).
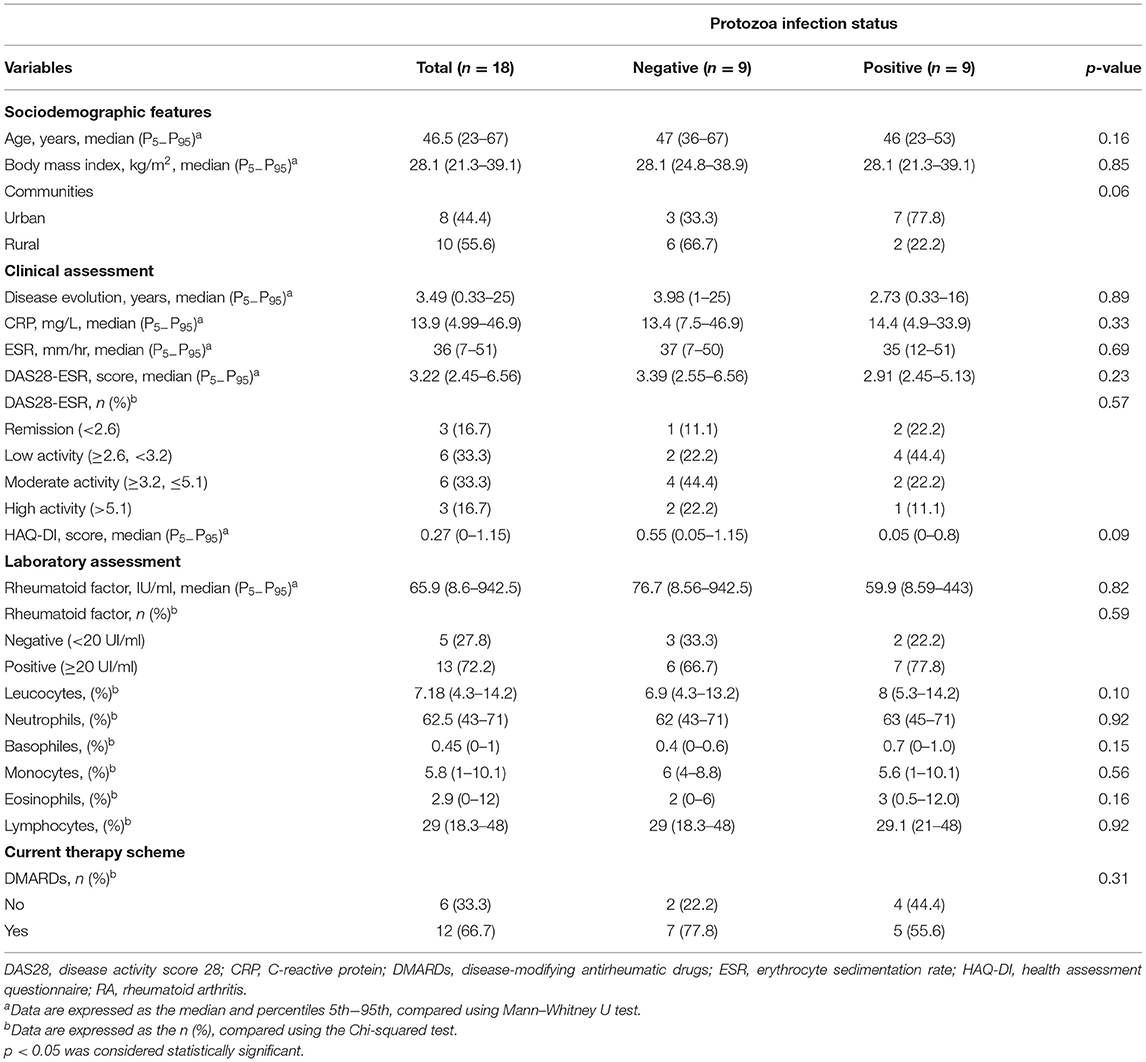
Table 1. Demographic, clinical, and inflammatory parameters according to the infection status by intestinal protozoa in patients with RA.
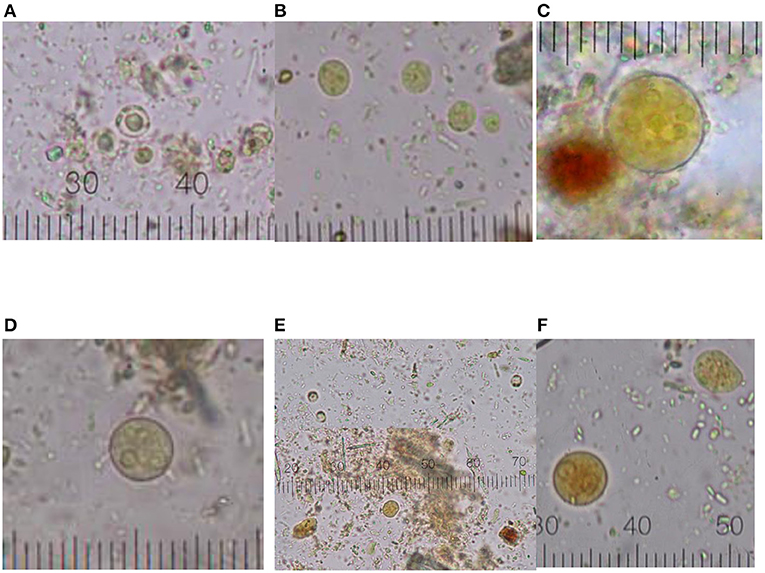
Figure 1. Graphical distribution of intestinal protozoa cysts. (A) Blastocystis sp.; (B) End. nana; (C) Ent. coli; (D) Ent. hystolytica/dispar; (E) coinfection (Blastocystis sp. and End. nana); (F) coinfection multiple (Ent. hystolytica/dispar, End. nana, Blastocystis sp.). Micrometric coefficient: 2.5 μm (A,C–F); 1 μm (B).
A total of 9 patients (50%) (four patients newly diagnosed and five under a therapeutic scheme with DMARDs) were positive for intestinal protozoa. Of those nine patients, 22.2% had a status of single infection with Blastocystis sp., and 27.8% had coinfection with Blastocystis sp. co-existing with one or more of the following protozoa: End. nana, Entamoeba coli, Ent. histolytica, or Entamoeba dispar (Figure 2).
Within the positive samples for Blastocystis sp., the abundance scale was distributed as follows: scarce on 18.2%, moderate on 9.1%, and abundant on 13.6%. For End. nana, the abundance scale was scarce on 4.5%, moderate on 4.5%, and abundant on 9.1% within the positive population, while for Ent. coli and Ent. histolytica/Ent. dispar, the identified scale was scarce on 4.5% of the positive population. On the other hand, the coprological analysis revealed higher quantities of neutral fat in patients who were positive for protozoa (p < 0.001), whereas starches, nondigestible fibers, and fatty-acid crystals were present similar between groups. The characteristics of the fecal analysis of each patient that were positive for protozoa are summarized in Supplementary Table S1.
Association Between IFABP2 Serum Levels in Infection Status in RA
In the present study, we observed the IFABP2 serum levels were significantly higher in patients positive for infection with intestinal protozoa (p = 0.010), particularly in those with coinfection (p = 0.019) (Figure 3A) and with a higher abundance of Blastocystis sp. (p = 0.019) (Figure 3B).
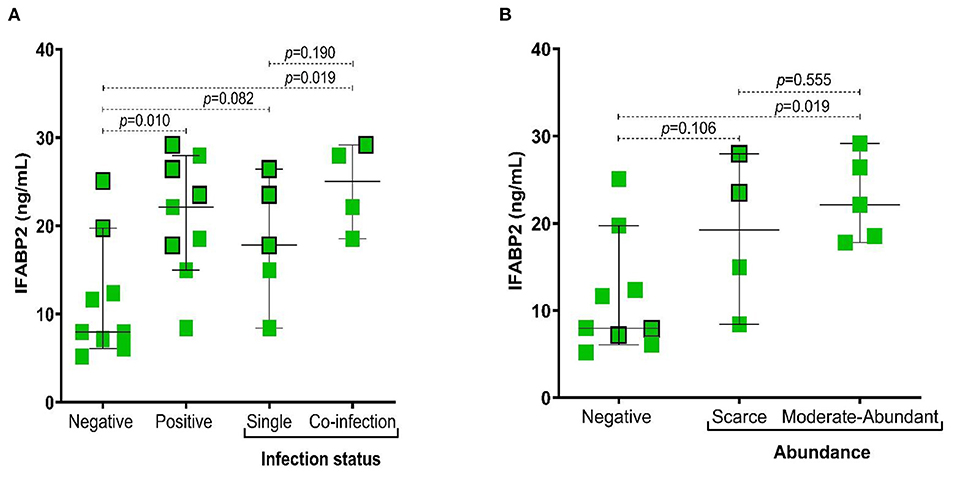
Figure 3. Association of IFABP2 levels according to infection status by intestinal protozoa and abundance of Blastocystis sp. (A) IFABP2 serum levels according to infection status; (B) IFABP2 serum levels according to the abundance of Blastocystis sp. Bars represent the median with the P5-P95 range. The boxes with borders represent patients without pharmacological therapy. Statistical analyses were performed by Mann–Whitney U test. Significance was set at p < 0.05.
When the cytokines' levels were compared between groups of patients according to therapy and protozoa infection status, we found that the levels of TNF-α were higher in patients with coinfection (p = 0.016), particularly in the group of patients under antirheumatic treatment (Figure 4). Additionally, we observed that in subjects with coinfection with intestinal protozoa, the levels of TNF-α (Supplementary Figure S2) and the frequency of CD4+ T cells were higher in participants with coinfection by protozoa, although not significantly (Figure 5A), while CD8+ T cells were similar between groups (Figure 5B).
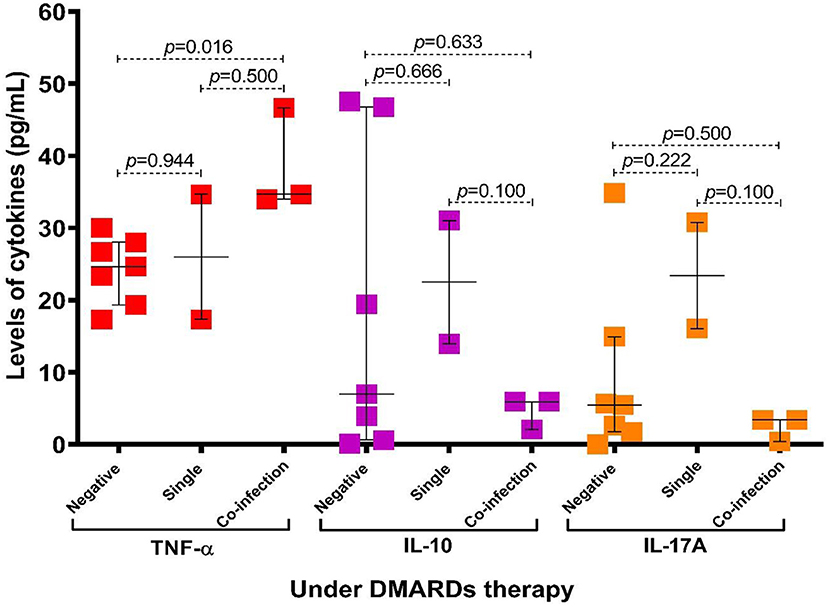
Figure 4. Association of levels of cytokines according to infection status by intestinal protozoa and patients with RA under DMARDs therapy. Bars represent the median with P5-P95 range. Statistical analyses were performed by Mann–Whitney U test. Significance was set at p < 0.05.
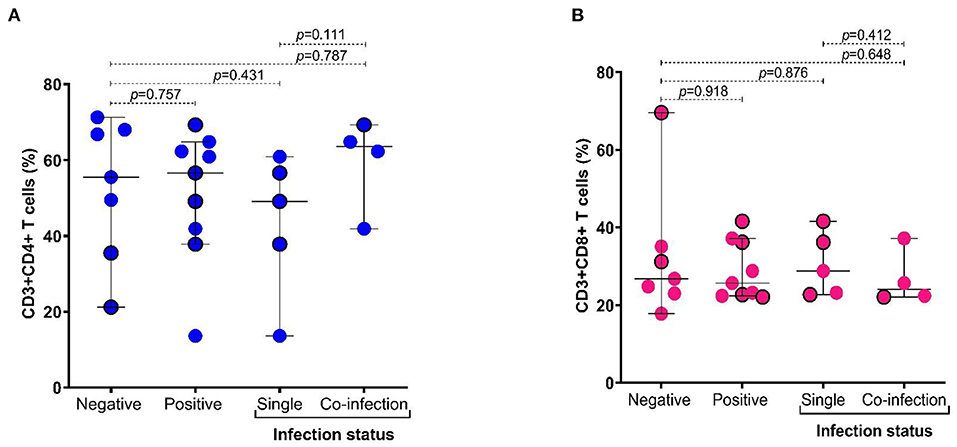
Figure 5. Distribution of frequency of CD4+ and CD8+ T cells according to infection status by intestinal protozoa. (A) Frequency of CD3+ and CD4+ T cells (%); (B) frequency of CD3+ and CD8+ T cells (%). Bars represent the median with the P5-P95 range. The circles with borders represent patients without pharmacological therapy. Statistical analyses were performed by Mann–Whitney U test. Significance was set at p < 0.05.
Discussion
The main findings in this study show that (i) infection by Blastocystis sp. is frequent in patients with RA in southern Mexico, (ii) serum levels of IFABP2 were higher in individuals with infection and coinfection of protozoa, (iii) the abundance of Blastocystis sp. is associated with higher serum levels of IFABP2, and (iv) infection by protozoa promotes the increase of TNF-α in patients with RA, mostly in those under DMARDs pharmacological treatment.
The prevalence of infection by intestinal protozoa in subjects with rheumatic diseases has been previously reported to be particularly high (25–30%) (48), when compared to that reported in Mexican population (49) and asymptomatic (50). In the present study, we found that 50% (9/18) of the individuals with RA recruited were positive for intestinal protozoa, similarly reported in an Egyptian population with RA (51), and higher than the report of Jimenez-Balderas et al. (48) for patients with RA in Mexico City [25%, (3/12)], and also the one reported for patients with RA in Brazil [11.9%, (8/67)] (20). These differences could be explained by sociodemographic factors that influence risk of parasitic infection, such as living in rural areas, lacking basic services (drinking water, for example), and the number of inhabitants per household (52), poor personal hygiene (50), and living with pets (48, 53). Another important factor for increased susceptibility for protozoa coinfection in patients with RA could be caused by pharmacological DMARDs treatment.
In our study, the number of positive cases for protozoa infections among patients with or without treatment did not show significant differences. However, patients under DMARDs treatment presented a higher frequency of coinfection (4/5) when compared to patients without treatment (1/4). Treatment with conventional DMARDs has been associated with the development of severe and moderate parasitic infections in RA (54).
Parasite's infection by Microsporidio (18), Endo. nana (20), and Cryptosporidium (51) has been previously reported in patients with RA. Hussein et al. (51) reported up to 46.72% (50/107) of positive cases of parasitic infection in the Egyptian population, identifying mainly the presence of Cryptosporidium (48%), Cyclospora cayetanensis (32%), Giardia lamblia (24%), Blastocystis hominis (20%), and Ent. histolytica (8%). In addition, Jimenez-Balderas et al. (48) reported single infections by Ent. coli and B. hominis, and one case of mixed infection by B. hominis and Ent. histolytica in Mexican patients. In the present study, we also found infection by End. nana. Also, we observed that the frequency of coinfection was 6 times higher than in others reports in the asymptomatic population, in which a percentage of 2% (50) and 3.4% of coinfection was identified (55).
Reactivation of RA has been associated with infection by intestinal parasites, and it is suggested that parasitic coinfection could compromise the immune response of susceptible hosts (16). Additionally, it has been reported that parasitic infection is accompanied by joint pain and elevated levels of ESR and CRP (51). In patients with RA, infection with End. nana, Ent. histolytica, and Ent. coli has been related to poor health scales and fatigue (20), but not with the parameters of disease's clinical activity (18), similar to what was found in this study. Nevertheless, B. hominis has been particularly associated with reactive RA in four clinical cases (13, 17, 19). Our study found infection by Blastocystis sp. in every positive case. We found that Blastocystis sp. abundance and its coexistence with other intestinal protozoa were associated with increase of IFABP2 and TNF-α serum levels, thus suggesting a relation between infection by intestinal protozoa and the potential damage of the intestinal epithelium and inflammation in patients with RA.
In this sense, it has been reported that B. hominis increases intestinal permeability by promoting the damage of epithelial cells through apoptosis and the degradation of tight junctions such as occludin and ZO-1 by two cysteine proteases (legumain and capthesin B). Furthermore, B. hominis has an immunomodulatory effect on the degradation of IgA, the inhibition of iNOS, and the increase of cytokines such as IL-8, GM-CSF, IL-1β, IL-6, and TNF-α (32, 56), and this could explains the increase of TNF-α in our patients with coinfection with Blastocystis sp. Also, it has been described that coinfection by Blastocystis sp. and Ent. histolytica modulates the apoptosis of intestinal epithelial cells (27); therefore, the coinfection status could strengthen the intestinal lesion. In our study, one case of coinfection by Blastocystis sp., Ent. histolytica, and End. nana was identified, and it was observed that even though it was in a patient under DMARDs triple-therapy treatment, the patient presented moderate clinical activity (DAS28-ESR = 3.07), a higher proportion of eosinophils (9%), and the presence of Charcot–Leyden crystals.
This study establishes a relation between the infection by Blastocystis sp., its abundance, and the serum levels of IFABP2. Previously, it has been shown that increased intestinal permeability happens during the infection by intestinal protozoa, and that the damage of the intestinal epithelium is enhanced during mixed infection by G. lamblia and B. hominis (57). It has been proved that subtype 7 of B. hominis promotes the apoptosis of enterocytes in vitro by activating the caspases 3 and 9 (intrinsic pathway) (31). Particularly, capthesin B virulence factor of subtype 7 increases the intestinal permeability in vitro (58), and it promotes in vivo the decrease of Bifidobacterium and Lactobacillus (10). Even though we did not investigate the Blastocystis subtypes, it is important to identify the presence of subtypes with higher virulence and its effects on the intestinal barrier (59), the gut microbiota, and the variability of the clinical phenotype of RA disease.
We observed in this study that the serum levels of TNF-α and the frequency of CD4+ T cells in peripheral blood were higher in patients with coinfection with protozoa; particularly in patients under DMARDs therapy and coinfection with protozoa, the TNF-α levels were significantly higher. The TNF-α serum levels are associated with prognostic response to DMARDs (60). Therefore, the presence of elevated levels of TNF-α and the increase of lymphocytes T CD4+ in patients under DMARDs treatment could suggest a relation between the poor response to treatment and the infection by protozoa, even though more robust studies are required to delve into this aspect.
We demonstrated the relation between the infection by protozoa and intestinal permeability, and potentially with inflammation in patients with RA. Nevertheless, our study presents some limitations worthy of consideration: (1) the design of the study was cross-sectional, and only one fecal sample of each participant was analyzed, (2) the sample size was small, and (3) individuals with and without treatment were included, which could represent a limitation for proving the relationship between the presence of intestinal protozoa and inflammation markers.
In conclusion, Blastocystis sp. was the most common intestinal protozoa found in women with RA from southern Mexico. Elevated levels of IFABP2 were found in individuals with coinfection by intestinal protozoa, mainly, in whom the abundance of Blastocystis sp. was higher. The coinfection with protozoa was more frequent in patients under DMARDs treatment, associated with higher levels of serum TNF-α.
Therefore, our results suggest that the infection with protozoa is associated with intestinal barrier damage and with the modulation of pro-inflammatory conditions in patients with RA; thus, our findings evidence the need to conduct a screening for intestinal parasites in patients with RA mainly during the first year of antirheumatic treatment to prevent epithelial barrier damage and side effects associated with pro-inflammatory status.
Data Availability Statement
The original contributions presented in the study are included in the article/Supplementary Material, further inquiries can be directed to the corresponding author.
Ethics Statement
The studies involving human participants were reviewed and approved by Ethics and Research Committee of the University Autonomous of Guerrero (Project identification code: CB-004/2017). The patients/participants provided their written informed consent to participate in this study.
Author Contributions
IG-G: conceptualization and design of the study and drafted the final manuscript. OZ-G and IG-P: data collection and population sampling. BN-T: performed the stool analysis. JN-Z: performed the clinical evaluation of patients. OB: performed the determination and analysis of T cells. OZ-G, RF-V, GP-R, IG-P, and IP-R: performed the proteins' determination. IG-G and OZ-G: statistical analysis. All authors contributed to manuscript revision, read, and approved the submitted version.
Conflict of Interest
The authors declare that the research was conducted in the absence of any commercial or financial relationships that could be construed as a potential conflict of interest.
Publisher's Note
All claims expressed in this article are solely those of the authors and do not necessarily represent those of their affiliated organizations, or those of the publisher, the editors and the reviewers. Any product that may be evaluated in this article, or claim that may be made by its manufacturer, is not guaranteed or endorsed by the publisher.
Acknowledgments
We especially thank the patients for participating in the study. Also, thanks go to the group of collaborators in the multidisciplinary team who contributed their knowledge and support for the management of and integral approach to these patients.
Supplementary Material
The Supplementary Material for this article can be found online at: https://www.frontiersin.org/articles/10.3389/fmed.2022.846934/full#supplementary-material
References
1. Bocanegra TS, Espinoza LR, Bridgeford PH, Vasey FB, Germain BF. Reactive arthritis induced by parasitic infestation. Ann Intern Med. (1981) 94:207–9. doi: 10.7326/0003-4819-94-2-207
2. Peng SL. Rheumatic manifestations of parasitic diseases. Semin Arthritis Rheum. (2002) 31:228–47. doi: 10.1053/sarh.2002.30441
3. Doury P. [Is there a role for parasites in the etiology of inflammatory rheumatism?]. Bull Acad Natl Med. (1990) 174:743–51.
4. Maeda Y, Takeda K. Host-microbiota interactions in rheumatoid arthritis. Exp Mol Med. (2019) 51:1–6. doi: 10.1038/s12276-019-0283-6
5. Chiang HI, Li JR, Liu CC, Liu PY, Chen HH, Chen YM, et al. An Association of gut microbiota with different phenotypes in chinese patients with rheumatoid arthritis. J Clin Med. (2019) 8:1770. doi: 10.3390/jcm8111770
6. Scher JU, Nayack RR, Ubeda C, Turnbaugh PJ, Abramson SB. Pharmacomicrobiomics in inflammatory arthritis: gut microbiome as modulator of therapeutic response. Nat Rev Rheumatol. (2020) 16:282–92. doi: 10.1038/s41584-020-0395-3
7. Zaragoza-García O, Castro-Alarcón N, Pérez-Rubio G, Guzmán-Guzmán IP. DMARDs-gut microbiota feedback: implications in the response to therapy. Biomolecules. (2020) 10:1479. doi: 10.3390/biom10111479
8. Partida-Rodríguez O, Serrano-Vázquez A, Nieves-Ramírez M, Moran P, Rojas L, Portillo T, et al. Human intestinal microbiota: interaction between parasites and the host immune response. Arch Med Res. (2017) 48:690–700. doi: 10.1016/j.arcmed.2017.11.015
9. Wang S, El-Fahmawi A, Christian DA, Fang Q, Radaelli E, Chen L, et al. Infection-induced intestinal dysbiosis is mediated by macrophage activation and nitrate production. MBio. (2019) 10:e00935–19. doi: 10.1128/mBio.00935-19
10. Yason JA, Liang YR, Png CW, Zhang Y, Tan K. Interactions between a pathogenic Blastocystis subtype and gut microbiota: in vitro and in vivo studies. Microbiome. (2019) 7:30. doi: 10.1186/s40168-019-0644-3
11. Burnstein SL, Liakos S. Parasitic rheumatism presenting as rheumatoid arthritis. J Rheumatol. (1983) 10:514–5.
12. Lee MG, Rawlins SC, Didier M, DeCeulaer K. Infective arthritis due to Blastocystis hominis. Ann Rheum Dis. (1990) 49:192–3. doi: 10.1136/ard.49.3.192
13. Lakhanpal S, Cohen SB, Fleischmann RM. Reactive arthritis from Blastocystis hominis. Arthritis Rheum. (1991) 34:251–3. doi: 10.1002/art.1780340227
14. Krüger K, Kamilli I, Schattenkirchner M. [Blastocystis hominis as a rare arthritogenic pathogen. A case report]. Z Rheumatol. (1994) 53:83–5.
15. Di Prieto A, Proverbio MR, Coletta M, Siani P, Zeoli L, Tammaro V. [Parasitic arthritis: a case report]. Pediatr Med Chir. (1996) 18:211–2.
16. Quiroz OF. Artritis Reactiva por Parásitos Intestinales en el Hospital Militar Central de Lima. Ann Fac Med. (1999) 60:49–54. doi: 10.15381/anales.v60i1.4511
17. González I, Pons M, Carrasco I. Artritis reactivas y Blastocystis hominis:¿una asociación casual? Rev Esp Reumatol. (2000) 27:74–5.
18. Aikawa NE, Twardowsky A, Carvalho JF, Silva CA, Silva IL, Ribeiro AC, et al. Intestinal microsporidiosis: a hidden risk in rheumatic disease patients undergoing anti-tumor necrosis factor therapy combined with disease modifying anti-rheumatic drugs? Clinics. (2011) 66:1171–75. doi: 10.1590/S1807-59322011000700008
19. Tejera B, Grados D, Martínez-Morillo M, Roure S. [Reactive arthritis caused by Blastocystis hominis]. Rheumatol Clin. (2012) 8:50–1. doi: 10.1016/j.reumae.2011.07.005
20. Gomides A, Pires A, Albuquerque C, Souza T, Muniz L, Lima C, et al. Enteroparasitoses in patients with rheumatoid arthritis. Advances Inf Dis. (2017) 7:143–52. doi: 10.4236/aid.2017.74015
21. Ashiri A, Beiromvad M, Khanzadeh A. Strongyloides stercoralis infection in patient with rheumatoid arthritis and type 2 diabetes mellitus: a case-based review. Clin Rheumatol. (2019) 38:3093–98. doi: 10.1007/s10067-019-04611-4
22. Nazik S, Yildiz F. A case of rheumatoid arthritis with Strongiloides stercoralis hyperinfection. Turkiye Parasitol Derg. (2020) 44:112–4. doi: 10.4274/tpd.galenos.2020.6222
23. Roxström-Lindquist K, Ringqvist E, Palm D, Svärd S. Giardia lamblia-induced changes in gene expression in differentiation Caco-2 human intestinal epithelial cells. Infect Immun. (2005) 73:8204–8. doi: 10.1128/IAI.73.12.8204-8208.2005
24. Doonan J, Tarafdar A, Pineda MA, Lumb FE, Crowe J, Khan AM, et al. The parasitic worm product ES-62 normalises the gut microbiota bone marrow axis in inflammatory arthritis. Nat Commun. (2019) 10:1554. doi: 10.1038/s41467-019-09361-0
25. Pineda MA, Al-Riyami L, Harnett W, Harnett MM. Lessons from helminth infections: ES-62 highlights new interventional approaches in rheumatoid arthritis. Clin Exp Immunol. (2014) 177:13–23. doi: 10.1111/cei.12252
26. Rodgers DT, Pineda MA, McGrath MA, Al-Riyami L, Harnett W, Harnett MM. Protection against collagen-induced arthritis in mice afforded by the parasitic worm product, ES-62, is associated with restoration of the levels of interleukin-10-producing B cells and reduced plasma cell infiltration of the joints. Immunology. (2014) 141:457–66. doi: 10.1111/imm.12208
27. Kapczuk P, Kosik-Bogacka D, Kupnicka P, Metryka E, Simińska D, Rogulska K, et al. The influence of select gastrointestinal parasites on apoptosis in intestinal epithelial cells. Biomolecules. (2020) 10:674. doi: 10.3390/biom10050674
28. Kumar A, Chatterjee I, Anbazhagan AN, Jayawardena D, Priyamvada S, Alrefai WA, et al. Cryptosporidium parvum disrupts intestinal epithelial barrier function via altering expression of key tight junction and adherens junction proteins. Cell Microbiol. (2018) 20:e12830. doi: 10.1111/cmi.12830
29. Buret AG, Mitchell K, Muench DG, Scott KG. Giardia lamblia disrupts tight junctional ZO-1 and increases permeability in non-transformed human small intestinal epithelial monolayers: effects of epidermal grow factor. Parasitology. (2002) 125:11–9. doi: 10.1017/S0031182002001853
30. Buret AG, Chin AC, Scott KG. Infection of human and bovine epithelial cells with Cryptosporidium andersoni induces apoptosis and disrupts tight junctional ZO-1: effects of epidermal grow factor. Int J Parasitol. (2003) 33:1363–71. doi: 10.1016/S0020-7519(03)00138-3
31. Wu Z, Mirza H, Teo JD, Tan KS. Strain-dependent induction of human enterocyte apoptosis by blastocystis disrupts epithelial barrier and ZO-1 organization in a caspase 3-and 9-dependent manner. Biomed Res Int. (2014) 2014:209163. doi: 10.1155/2014/209163
32. Ajjampur SS, Tan KS. Pathogenic mechanism in Blastocystis spp.-interpreting results from in vitro and in vivo studies. Parasitol Int. (2016) 65:772–9. doi: 10.1016/j.parint.2016.05.007
33. Fasano A. Zonulin, regulation of tight junctions, and autoimmune diseases. Ann N Y Acad Sci. (2012) 1258:25–33. doi: 10.1111/j.1749-6632.2012.06538.x
34. Sturgeon C, Fasano A. Zonulin, a regulator of epithelial and endothelial barrier functions, and its involvement in chronic inflammatory diseases. Tissue Barriers. (2016) 4:e1251384. doi: 10.1080/21688370.2016.1251384
35. Derikx JP, Schellekens DH, Acosta S. Serological markers for human intestinal ischemia: A systematic review. Best Pract Res Clin Gastroenterol. (2017) 31:69–74. doi: 10.1016/j.bpg.2017.01.004
36. Pelsers MM, Hermens WT, Glatz JF. Fatty acid-binding proteins as plasma markers of tissue injury. Clin Chim Acta. (2005) 352:15–35. doi: 10.1016/j.cccn.2004.09.001
37. Gajda A, Storch J. Enterocyte fatty acid binding proteins (FABPs): different functions of liver- and intestinal-FABPs in the intestine Prostaglandins. Leukot Essent Fatty Acids. (2015) 93:9–16. doi: 10.1016/j.plefa.2014.10.001
38. Ockner RK, Manning JA. Fatty acid-binding protein in small intestine. Identification, isolation, and evidence for its role in cellular fatty acid transport. J Clin Invest. (1974) 54:326–38. doi: 10.1172/JCI107768
39. Stevens BR, Goel R, Seugbum K, Richards EM, Holbert RC, Pepine CJ, et al. Increased human intestinal barrier permeability plasma biomarkers zonulin and FABP2 correlated with plasma LPS and altered gut microbiome in anxiety of depression. Gut. (2018) 67:1555–7. doi: 10.1136/gutjnl-2017-314759
40. Blaser A, Padar M, Tang J, Dulton J, Forbes A. Citrulline and intestinal fatty acid-binding protein as biomarkers for gastrointestinal dysfunction in the critically ill. Anaesthesiol Intensive Ther. (2019) 51:230–9. doi: 10.5114/ait.2019.86049
41. Cascais-Figueiredo T, Austriaco-Teixeira P, Fantinatti M, Silva-Freitas ML, Santos-Oliveira JR, Coelho CH, et al. Giardiasis alters intestinal fatty acid binding protein (I-FABP) and plasma cytokines levels in children in Brazil. Pathogens. (2019) 9:7. doi: 10.3390/pathogens9010007
42. Aletaha D, Neogi T, Silman AJ, Funovits J, Felson DT, Bingham CO 3rd, et al. 2010 Rheumatoid arthritis classification criteria: an American College of Rheumatology/European League Against Rheumatism collaborative initiative. Arthritis Rheum. (2010) 62:2569–81. doi: 10.1002/art.27584
43. Bench Bench aids for the diagnosis of intestinal parasites Second edition. Geneve: World Health Organization (2019).
44. CLSI. Procedures for the Recovery and Identification of Parasites From the Intestinal Tract; Approved Guideline—Second Edition. CLSI document M28-A2. Wayne, PA: Clinical and Laboratory Standards Institute (2005).
46. Sánchez-Manzano RM, Nogueda-Torres B. El examen general de las heces fecales. Metodología y atlas de enteroparásitos y del sedimento microscópico. México (2020).
48. Jimenez-Balderas FJ, Camargo-Coronel A, Gargia-Jaimes J, Zonana-Nacach A, Alcantara-Anguianoi I, Carrillo-Becerril L, et al. A study on parasites in Mexican rheumatic disease patients. J Egypt Soc Parasitol. (2012) 42:271–80. doi: 10.12816/0006316
49. Díaz D, Vazquez-Polanco AM, Argueta-Donohue J, Stephens C, Jimenez-Trejo F, Ceballos-Liceaga SE, et al. Incidence of intestinal infectious diseases due to protozoa and bacteria in Mexico: analysis of national surveillance records from 2003 to 2012. Biomed Res Int. (2018) 2018:2893012. doi: 10.1155/2018/2893012
50. Yoda T, Suzue T, Hirao T, Yoshioka A, Escobar-Jimenez JJ, Yanagi T, et al. Asymptomatic intestinal protozoan infections among inhabitans in Mexico city, Mexico. Int J Med Med Sci. (2011) 3:223–6. doi: 10.5897/IJMMS.9000039
51. Hussein EM, El-Hamshary EM, Osmar AS, Mokhtar AB. Parasitism rheumatism among patients infected with intestinal parasites. Act Sci Gastrl Dis. (2019) 2:19–23. doi: 10.31080/ASGIS.2019.02.0059
52. Ramos F, Morán P, González E, García G, Ramiro M, Gómez A, et al. High prevalence rate of Entamoeba histolytica asymptomatic infection in a rural Mexican community. Am J Trop Med Hyg. (2005) 73:87–91. doi: 10.4269/ajtmh.2005.73.87
53. Tiang AL, Gu YL, Zhou N, Cong W, Li GX, Elsheikha HM, et al. Seroprevalence of Toxoplasma gondii infection in arthritis patients in Eastern China. Infect Dis Poverty. (2017) 6:153. doi: 10.1186/s40249-017-0367-2
54. Boerbooms AM, Kerstens PJ, Loenhout JW, Mulder J, van de Putte LB. Infections during low-dose methotrexate treatment in rheumatoid arthritis. Semin Arthritis Rheum. (1995) 24:411–21. doi: 10.1016/S0049-0172(95)80009-3
55. Ramirez-Miranda ME, Hernandez-Castellanos R, Lopez-Escamilla E, Moncada D, Rodriguez-Magallan A, Pagaza-Melero C, et al. Parasites in Mexican patients with irritable bowel syndrome: a case-control study. Parasit Vectors. (2010) 3:96. doi: 10.1186/1756-3305-3-96
56. Lu YY, Pei P, Zhang LL, Xye S, Han X, Bi XL, et al. [Study on the mechanism of the intestinal tight-junction destruction caused by Blastocystis hominis infection in rats]. Zhongguo Xue Xi Chong Bing Fang Zhi Za Zhi. (2021) 33:28–34. doi: 10.16250/j.32.1374.2020295
57. Dagci H, Ustun S, Taner MS, Ersoz G, Karacasu F, Budak S. Protozoon infections and intestinal permeability. Acta Trop. (2002) 81:1–5. doi: 10.1016/S0001-706X(01)00191-7
58. Nourrison C, Wawrzyniak I, Cian A, Livrelli V, Viscogliosi E, Delbac F, et al. On Blastocystis secreted cysteine proteases: a legumain-activated cathepsin B increases paracellular permeability of intestinal Caco-2 cell monolayers. Parasitology. (2016) 143:1713–22. doi: 10.1017/S0031182016001396
59. Tito RY, Chaffron S, Caenepeel C, Lima-Mendez G, Wang J, Viera-Silva S, et al. Population-level analysis of Blastocystis subtype prevalence and variation in the gut microbiota. Gut. (2019) 68:1180–9. doi: 10.1136/gutjnl-2018-316106
Keywords: intestinal protozoa, infection status, IFABP2, inflammation, rheumatoid arthritis
Citation: Guzmán-Guzmán IP, Nogueda-Torres B, Zaragoza-García O, Navarro-Zarza JE, Briceño O, Pérez-Rubio G, Falfán-Valencia R, Gutiérrez-Pérez IA and Parra-Rojas I (2022) The Infection, Coinfection, and Abundance of Intestinal Protozoa Increase the Serum Levels of IFABP2 and TNF-α in Patients With Rheumatoid Arthritis. Front. Med. 9:846934. doi: 10.3389/fmed.2022.846934
Received: 12 January 2022; Accepted: 15 March 2022;
Published: 12 April 2022.
Edited by:
Mary Canavan, Trinity College Dublin, IrelandReviewed by:
Jean-Claude Marie, INSERM U1149 Centre de Recherche sur l'Inflammation, FrancePham My-Chan Dang, INSERM U1149 Centre de Recherche sur l'Inflammation, France
Copyright © 2022 Guzmán-Guzmán, Nogueda-Torres, Zaragoza-García, Navarro-Zarza, Briceño, Pérez-Rubio, Falfán-Valencia, Gutiérrez-Pérez and Parra-Rojas. This is an open-access article distributed under the terms of the Creative Commons Attribution License (CC BY). The use, distribution or reproduction in other forums is permitted, provided the original author(s) and the copyright owner(s) are credited and that the original publication in this journal is cited, in accordance with accepted academic practice. No use, distribution or reproduction is permitted which does not comply with these terms.
*Correspondence: Iris Paola Guzmán-Guzmán, pao_nkiller@yahoo.com.mx; orcid.org/0000-0003-1535-4226