- Department of Hematology, Zhongnan Hospital of Wuhan University, Wuhan University, Wuhan, China
The relationship between newly diagnosed acute leukemia (AL) and heart-related lesions remains unclear. This study aimed to investigate baseline cardiac function and risk of cardiovascular diseases (CVDs) in patients with new-onset AL, and provide data on cardiac management strategies for patients with AL. We retrospectively collected data on baseline characteristics, echocardiography, and biochemical blood indicators (e.g., myocardial enzymes) from 408 patients, 200 with newly diagnosed AL, 103 with coronary artery disease (CAD), and 105 controls from January 1, 2015 to August 31, 2019. The creatine kinase isoenzyme myocardial band, lactate dehydrogenase, highly sensitive troponin-I, and B-type natriuretic peptide levels and left ventricular internal diameter (LVID) were significantly higher in patients with newly diagnosed AL than in the control group. The degree of cardiac damage was lower in newly diagnosed AL patients than in CAD patients. The best predictor of heart damage was LVID (AUC [area under the curve] = 0.709; 95% CI [confidence interval]: 0.637–0.781; p < 0.001), and independent prognostic risk factors were age and ejection fraction (HR [hazard ratio] = 1.636; 95% CI: 1.039–2.575; p = 0.033). The ratio of leukemia blasts among patients with AL was positively correlated with cardiac damage. Our data indicated that newly diagnosed AL patients had certain myocardial damage before treatment. Clinicians need to pay attention to these manifestations, which may be related to the prognosis.
Introduction
Heart disease and cancer are the two leading causes of global mortality (1). Worldwide, 17.9 million deaths were due to cardiovascular diseases (CVDs) in 2019, accounting for 32% of global deaths (2). Nearly 10 million deaths were due to cancer in 2020 globally, and approximately 70% occurred in low- and middle-income countries (3). Leukemia is a life-threatening malignant clonal disease of the hematopoietic system and plays an important part in the global cancer burden (4). According to the 2017 Global Burden of Disease study data, the number of newly diagnosed leukemia cases increased from 354,500 in 1990 to 518,500 in 2017. Globally, the number of acute myelocytic leukemia (AML) deaths rose to 92.98% from 1990 to 2017, while acute lymphoblastic leukemia (ALL) deaths increased by 40.12% (5).
In recent years, researchers have paid increasing attention to the new field of cardio-oncology (6, 7). Studies have found that cancer survivors have an increased risk of CVDs, mostly due to the cardiotoxicity of anti-cancer agents (8–10) or shared common lifestyle risk factors (11, 12). In addition, cancer and CVDs share common systemic pathogenic pathways and mechanisms: inflammation, metabolic modification, clonal hematopoiesis, and altered angiogenesis, as well as extracellular matrix (ECM) and stroboscopic cells (13). Therefore, cancer often coexists with cardiac-related lesions. Studies have found that secondary cardiac tumors (often malignant) with a high incidence most often metastasize from the respiratory system, followed by the hematopoietic system (leukemia/multiple myeloma) (14). The clinical manifestations of leukemic infiltration of the heart include congestive heart failure, pericardial effusion, infiltrative cardiac disease, and constrictive pericarditis (15, 16). Due to high cytokine release or direct leukemic myocardial infiltration, patients with AL may present cardiac dysfunction before starting anthracycline chemotherapy (17). Moreover, hyperleukocytic leukemia, associated with increased white blood cells, greatly increases the incidence of acute myocardial infarction (AMI) and stroke (18).
This study aimed to investigate baseline cardiac function and risk of CVDs in patients with new-onset AL, explore CVD risk factors present before treatment and their association with prognostic survival, and provide data on cardiac management strategies for patients with AL.
Materials and Methods
Study Design and Patients
This single-center retrospective observational study included patients with newly diagnosed AL, CAD and controls seen at Zhongnan Hospital, Wuhan University from January 1, 2015 to August 31, 2019. All study participants were Chinese and ≥ 18 years old. The AL group included patients seen at the department of hematology who were newly diagnosed (19) with AL and had not undergone chemotherapy. Patients with other previous or concurrent malignant tumors or a history of known cardiomyopathy or congestive heart failure were excluded from this study. The AL group was subdivided according to the white blood cell (WBC) count into hyperleukocytic acute leukemia (HAL) and non-hyperleukocytic acute leukemia (NHAL). In this study, HAL was defined as a total WBC count > 100 × 109/L (20). The CAD group included patients diagnosed (21) for the first time with no hematological diseases or other malignant tumors. The control group included healthy participants who had undergone a physical examination at the physical examination center, and none of them had heart-related diseases, leukemia, or other known cancers. Before starting the research, a written informed consent was signed by all study participants. All procedures followed the tenets of the Declaration of Helsinki and were approved by the Ethics in Research Committee of Wuhan University in Wuhan, China. This study was conducted and reported in accordance with the STROBE statement (22).
Measurements and Data
Collected the data in the medical records of patients at the first visit: age (years), sex, cardiovascular risk factors (hypertension, diabetes, and smoking history), AL type, routine blood tests: white blood cells (WBCs, 109/L), red blood cells (RBCs, 1012/L), hemoglobin (HGB, g/L), platelets (PLT, 109/L), alanine aminotransferase (ALT, U/L), aspartate transaminase (AST, U/L), blood urea nitrogen (BUN, mmol/L), creatinine (Cr, μmol/L), D-dimer (DD, ng/ml), biochemical blood indicators: creatine kinase (CK, U/L), creatine kinase myocardial band (CK-MB, U/L), hydroxybutyrate dehydrogenase (HBDH, U/L), lactate dehydrogenase (LDH, U/L), highly sensitive troponin-I (hs-cTnI, pg/ml), B-type natriuretic peptide (BNP, pg/mL) and echocardiography parameters: left atrial internal diameter (LAID, mm), left ventricular internal diameter (LVID, mm), interventricular septal (IVS, mm), left ventricular posterior wall (LVPW, mm), end-diastolic volume (EDV, ml), end-systolic volume (ESV, ml), stroke volume (SV, ml) and ejection fraction (EF, %).
Statistical Analysis
We performed linear regression analysis of biochemical blood indicators, myocardial enzymes, and echocardiographic indicators after logarithmic conversion. Normally distributed samples were compared using independent sample t-tests. The Mann-Whitney U rank-sum test was used for non-normally distributed samples and qualitative data were analyzed with the chi-square test. To reduce confounding in the AL group, such as anemia and cardiovascular risk factors, propensity score matching (PSM) analysis was conducted between the AL and control groups. The log-rank test was used to perform univariate analysis through Kaplan-Meier curves, and meaningful values were screened for multivariate Cox regression analysis. Odd ratios (ORs) and hazard ratios (HRs) were expressed with 95% confidence intervals (CIs). Statistical analysis was conducted using SPSS version 25.0 (IBM Corp., Armonk, NY, United States) and GraphPad Prism version 7.0 (GraphPad, San Diego, CA, United States). A value of p < 0.05 was considered statistically significant.
Results
Biochemical Blood Indicators and Echocardiography
A total of 408 individuals participated in this study, including 200 newly diagnosed AL patients (including 15 HAL patients), 103 patients with confirmed CAD, and 105 control patients. We eliminated patients who did not meet the study requirements. The screening flowchart is shown in Figure 1 and baseline patient characteristics are shown in Table 1. The mean age (range) of study participants in the control, AL, and CAD groups was 55 (44–63), 57 (41–66), and 62 (55–70) years, respectively. There were statistically significant differences in diabetes and routine blood indexes (WBC, RBC, HGB, PLT) between the AL and control groups. Meanwhile, there were significant differences in hypertension, diabetes, AST, BUN, and Cr between the CAD and control groups.
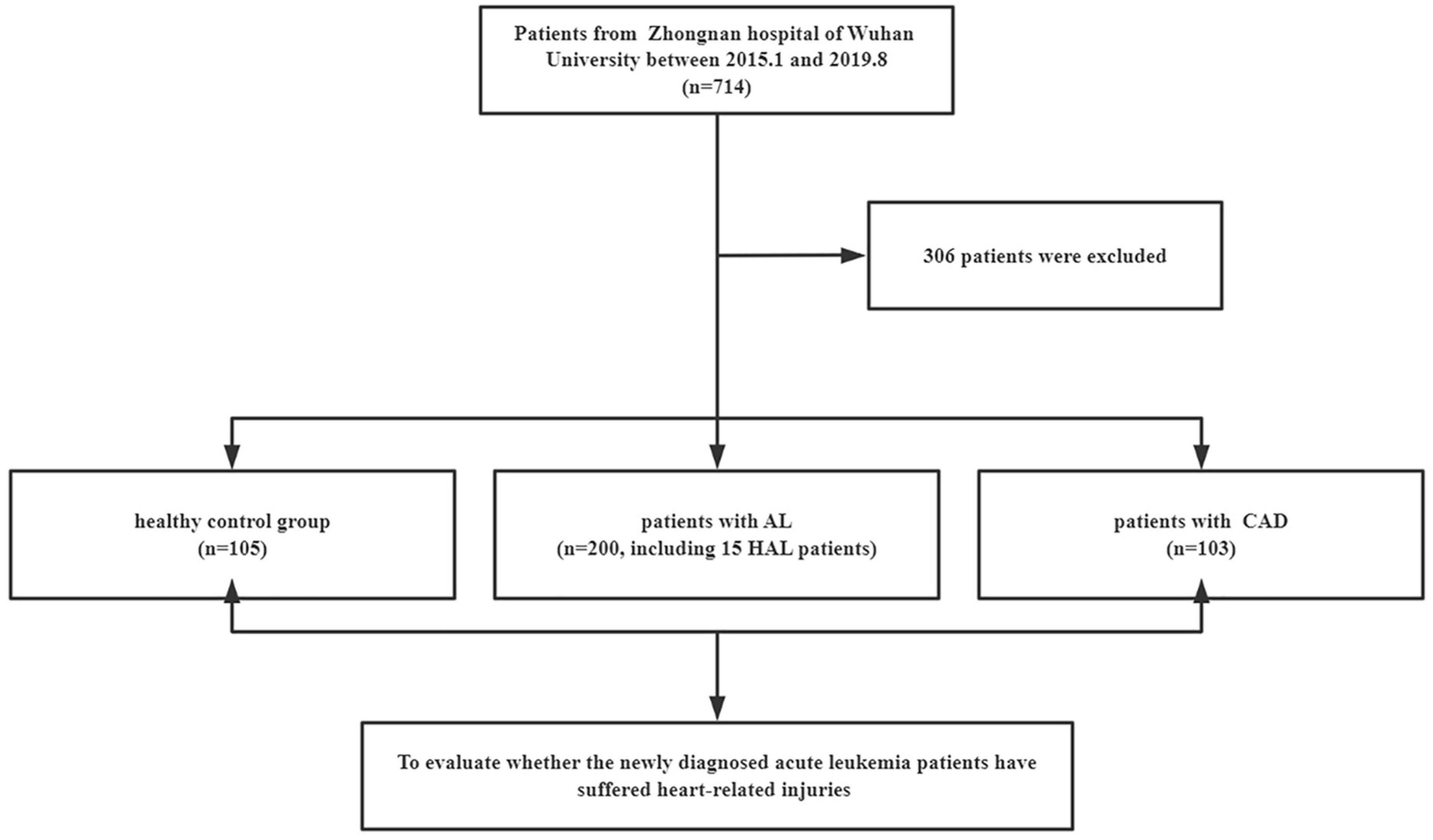
Figure 1. Selection of participants. AL, acute leukemia; CAD, coronary artery disease; HAL, hyperleukocytic acute leukemia.
A comparison of cardiac damage index results among the three groups is shown in Figure 2. The mean values of biochemical blood indicators, such as DD, CK-MB, HBDH, LDH, hs-cTnI, and BNP and echocardiography indexes such as LVID, EDV, ESV, and SV were significantly higher, while EF was significantly lower, in the AL group than in the control group (p < 0.05). In the comparison between the control and CAD groups, it could be found that the CAD group had more severe myocardial damage. The average values of echocardiography parameters LAID, LVID, EDV, and ESV were significantly higher, and EF value was significantly lower, in the CAD group compared with the control group; the average values of cardiac function and myocardial enzyme indexes increased significantly. Comparing the AL and the CAD groups, the average values of LAID, IVS, EDV, CK, CK-MB, and hs-cTnI in the CAD group were significantly higher, while only the values of DD and BNP were higher in the AL group.
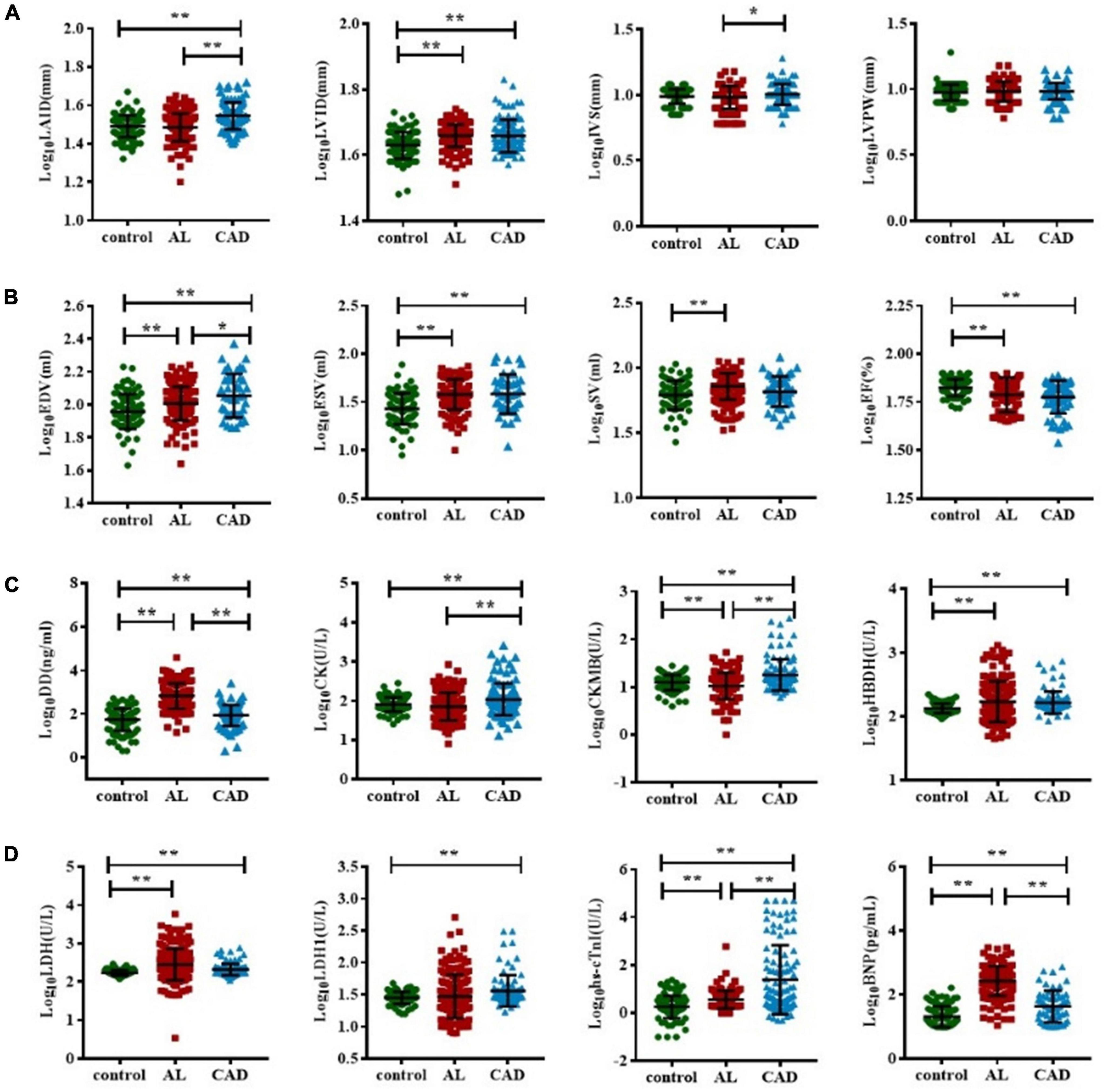
Figure 2. Comparison of cardiac function impairment indicators in acute leukemia, coronary artery disease, and control group patients. The T test was used to compare the myocardial enzyme and echocardiography indexes of the three group (A–D). (*represents p < 0.05, ** represents p < 0.01). BNP, B-type natriuretic peptide; CK, creatine kinase; CK-MB, creatine kinase myocardial band; DD, D-dimer; EDV, end-diastolic volume; ESV, end-systolic volume; EF, ejection fraction; hs-cTnI, highly sensitive troponin-I; HBDH, hydroxybutyrate dehydrogenase; IVS, interventricular septal; LVPW, left ventricular posterior wall; LDH, lactate dehydrogenase; LAID, left atrial internal diameter; LVID, left ventricular internal diameter; SV, stroke volume.
The indicators with significant differences between the AL and control group were included in the multivariate linear regression analysis, and the multiple linear regression equation was fitted. The model had 60.3% explanatory power (R2 = 0.603) for whether the AL group had damage from heart disease. Using multiple linear stepwise regression analysis, the final factors entering the equation were CK-MB, LDH, BNP, DD, and LVID (p < 0.05; Table 2).
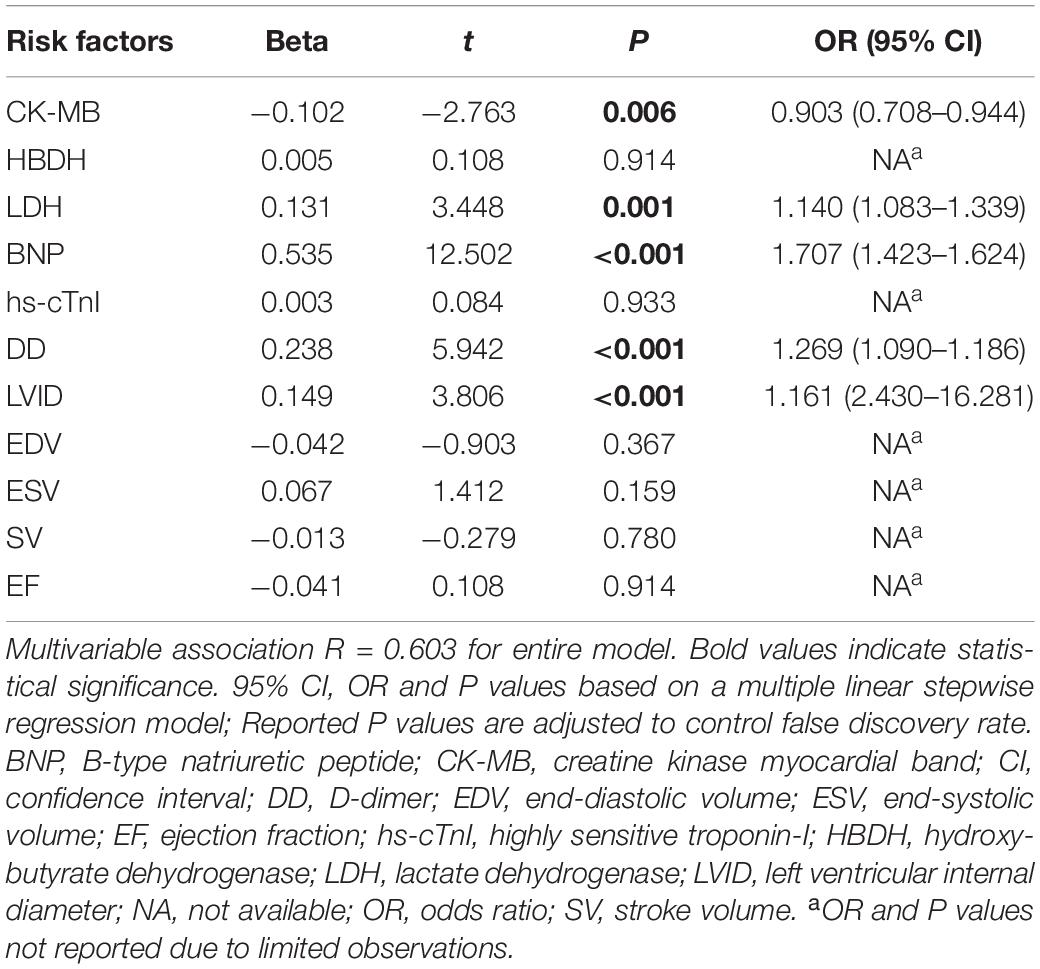
Table 2. Multivariate association of clinically relevant indicators between AL and control groups (related variables).
Propensity Score Matching Analysis
A total of 44 patients with AL and 44 control patients (1:1) were matched for age, sex, cardiovascular risk factors (hypertension, diabetes, and smoking history), and hemoglobin concentration. The indexes with significant differences between the matched AL and control group were included in the multiple linear regression analysis. Using multiple linear stepwise regression analysis, the final factors entering the equation were BNP, HBDH, and LVID (p < 0.05; Tables 3, 4).
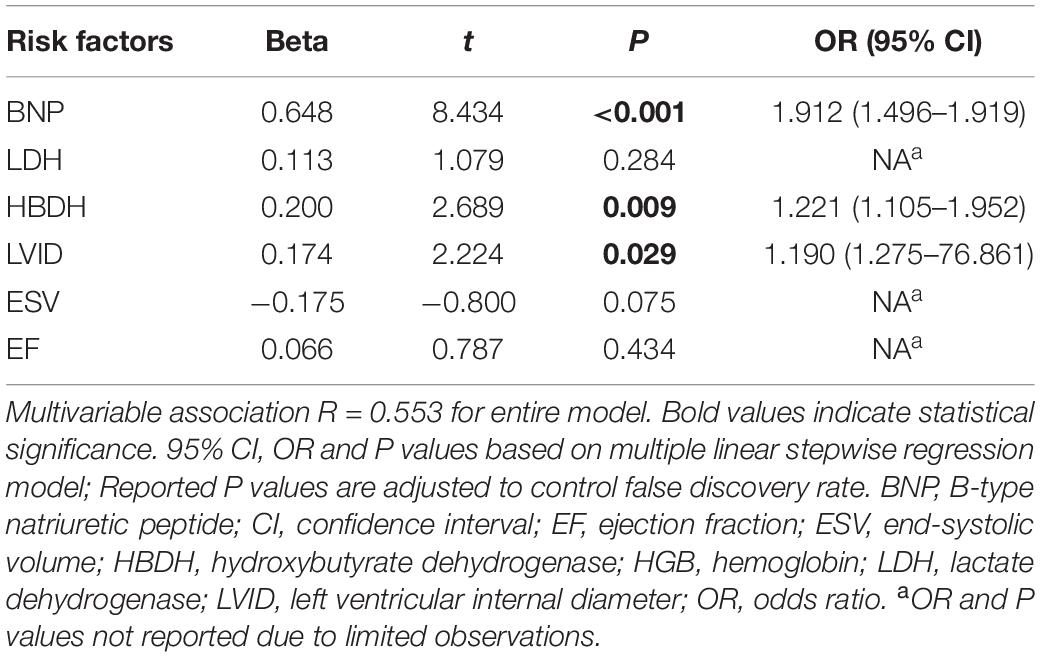
Table 4. Multivariate correlation of clinically relevant indicators between matched AL and control group patients (related variables).
Subgroup Analysis
Acute leukemia patients were subdivided into HAL or NHAL groups according to the WBC count. We found that AST, HBDH, and LDH were significantly higher in the HAL group than in the NHAL group (p < 0.05). There were no significant differences between the two groups in terms of other serological indicators or echocardiogram-related indicators (Figure 3).
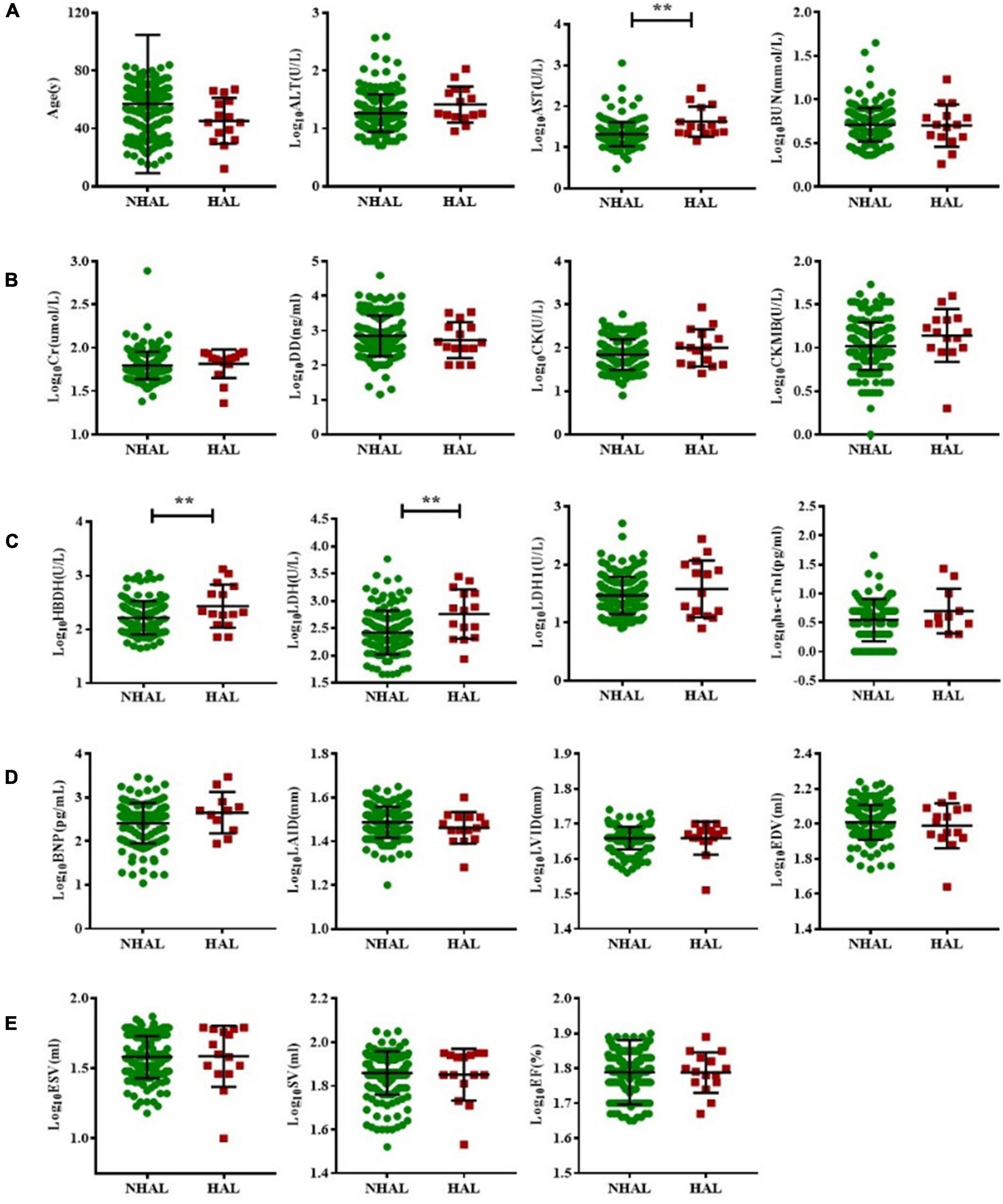
Figure 3. Subgroup analysis of non-hyperleukocytic and hyperleukocytic acute leukemia groups. The T test was used to compare BNP and myocardial enzyme and echocardiography indexes between the NHAL HAL groups (A–E). (** represents p < 0.01). AST, aspartate transaminase; ALT, alanine aminotransferase; BNP, B-type natriuretic peptide; BUN, blood urea nitrogen; Cr, creatinine; CK, creatine kinase; CK-MB, creatine kinase myocardial band; DD, D-dimer; EDV, end-diastolic volume; ESV, end-systolic volume; EF, ejection fraction; hs-cTnI, highly sensitive troponin-I; HBDH, hydroxybutyrate dehydrogenase; HAL, hyperleukocytic acute leukemia; IVS, interventricular septal; LVPW, left ventricular posterior wall; LDH, lactate dehydrogenase; LAID, left atrial internal diameter; LVID, left ventricular internal diameter; NHAL, non-hyperleukocytic acute leukemia; SV, stroke volume.
Prognostic Analysis
We followed all newly diagnosed AL patients for prognostic analysis. The median follow-up time was 35 months (range 1–55). We analyzed the relationship among age, sex, cardiovascular risk factors (hypertension, diabetes, and smoking history), biochemical blood indicators, myocardial enzyme indexes, echocardiography indexes, and the number of abnormal myocardial enzyme indexes and survival, to screen for risk factors related to the prognosis of the disease. Variables with statistically significant effect measurement values included age (p < 0.001), LDH (p = 0.038), EF (p = 0.025), and the number of myocardial enzyme abnormalities (p = 0.002). The meaningful survival curve is shown in Figure 4. In addition, the meaningful variables or close to meaningful values (p < 0.1) in the previous single-factor analysis were subjected to multi-factor Cox regression analysis. The results showed that age (HR = 0.540, 95%CI: 0.354–0.824, p = 0.004) and EF (HR = 1.636, 95% CI: 1.039–2.575, p = 0.033) were independent factors related to the prognosis of AL patients (Figure 5).
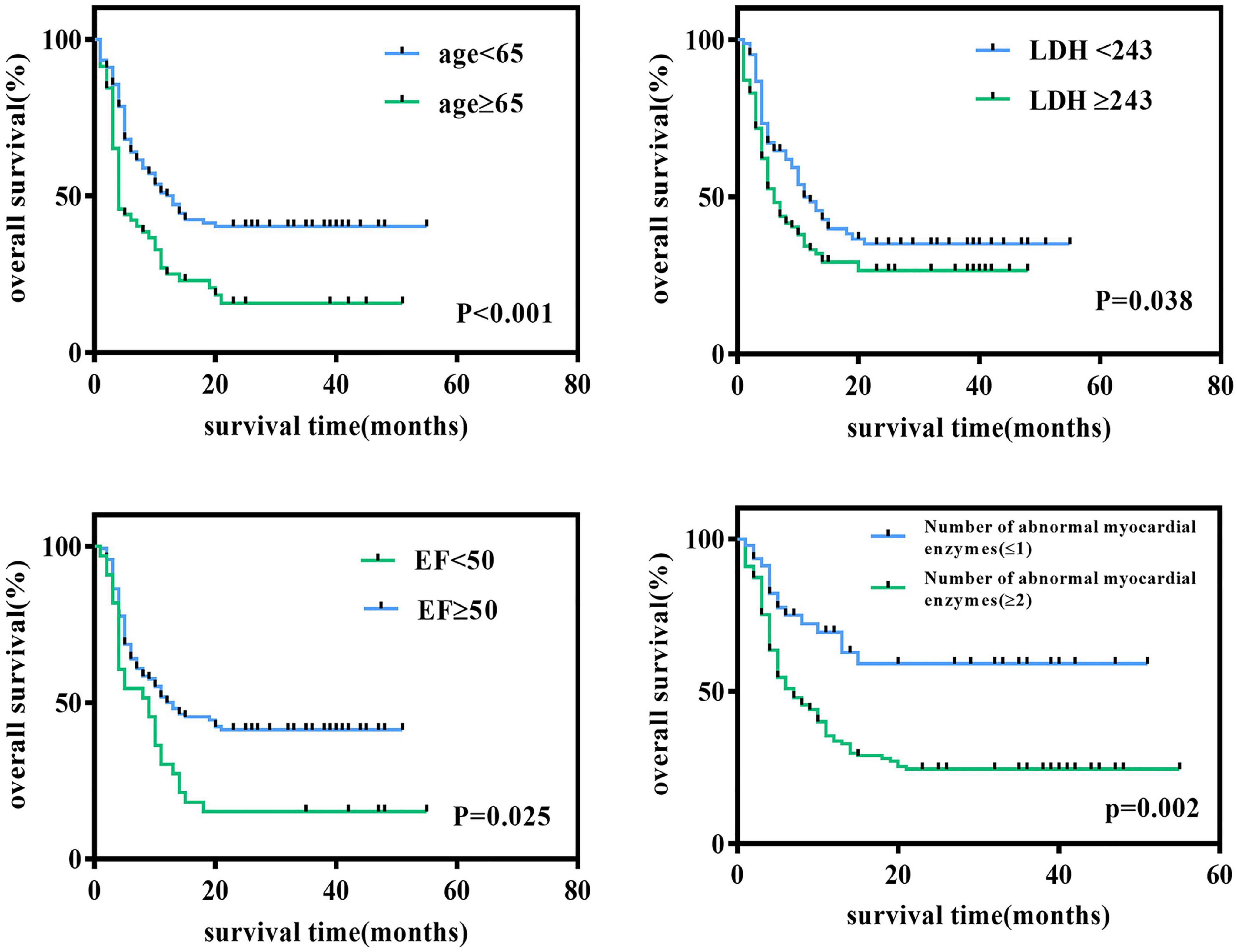
Figure 4. Survival curve of newly diagnosed acute leukemia patients. Log-rank test for univariate analysis of heart-related indicators in newly diagnosed acute leukemia patients through Kaplan-Meier curves. LDH, lactate dehydrogenase; EF, ejection fraction.
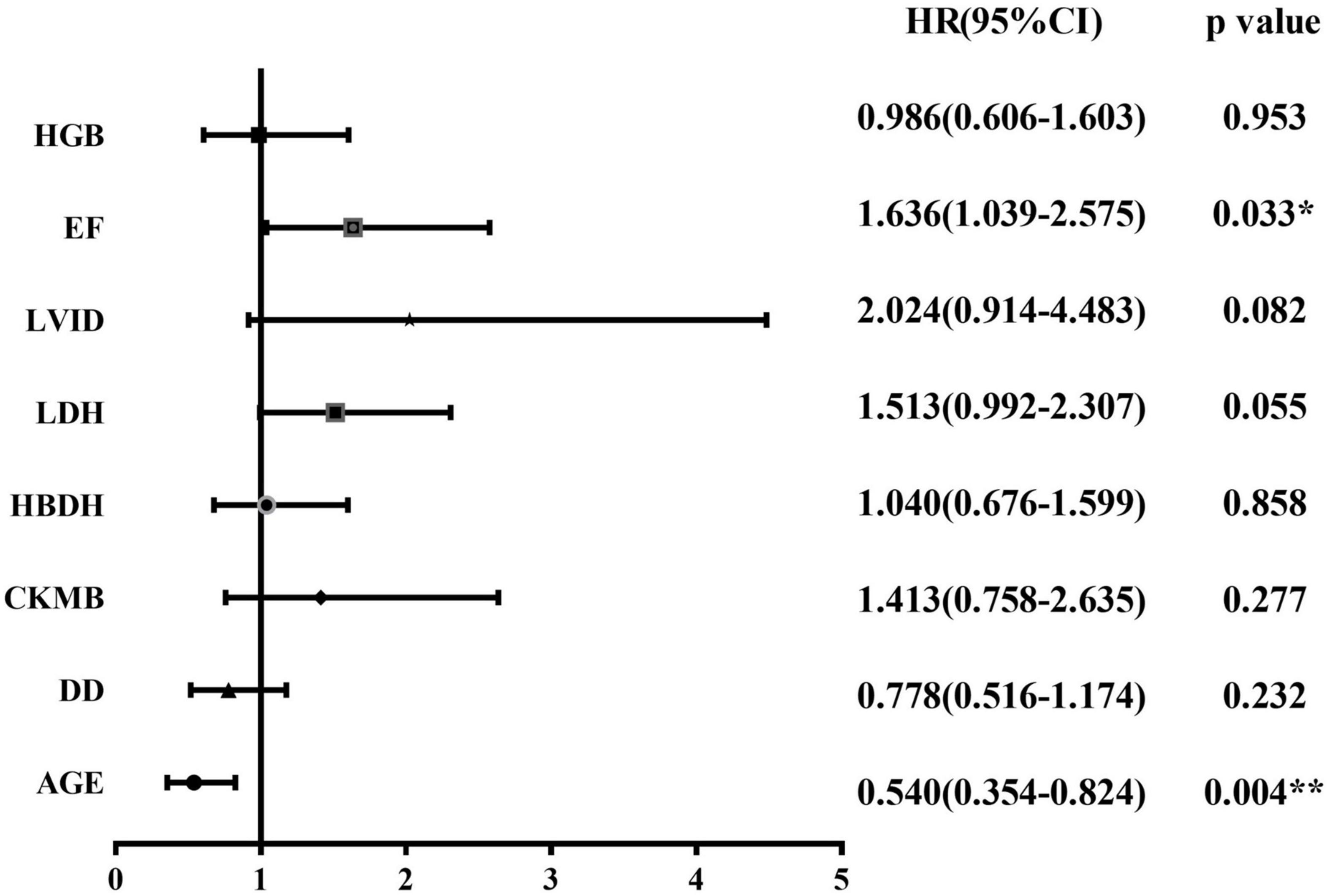
Figure 5. Forest plot for Cox regression analysis. Cox regression model for multivariate analysis. *p < 0.05, **p < 0.01. HRs and 95% CIs are estimated in Cox proportional hazards regression. CI, confidence interval; CK-MB, creatine kinase myocardial band; DD, D-dimer; EF, ejection fraction; HBDH, hydroxybutyrate dehydrogenase; HGB, hemoglobin; HRs, hazard ratios; LDH, lactate dehydrogenase; LVID, left ventricular internal diameter.
ROC Curve
Classification based on the echocardiography clinical diagnosis in newly diagnosed AL patients include significant differences in myocardial enzymes and related echocardiographic indicators; the three indicators with the highest results for the ROC area under the curve (AUC) were LVID (0.709), EDV (0.675), and HBDH (0.591). This result revealed that the best predictor of leukemic myocardial damage was LVID. It showed the predictive ability of LVID (≥43.5 cm) (AUC = 0.709, 95%CI: 0.637–0.781, p < 0.001; sensitivity = 95.4%; specificity = 32.2%). The ROC curve is shown in Figure 6.
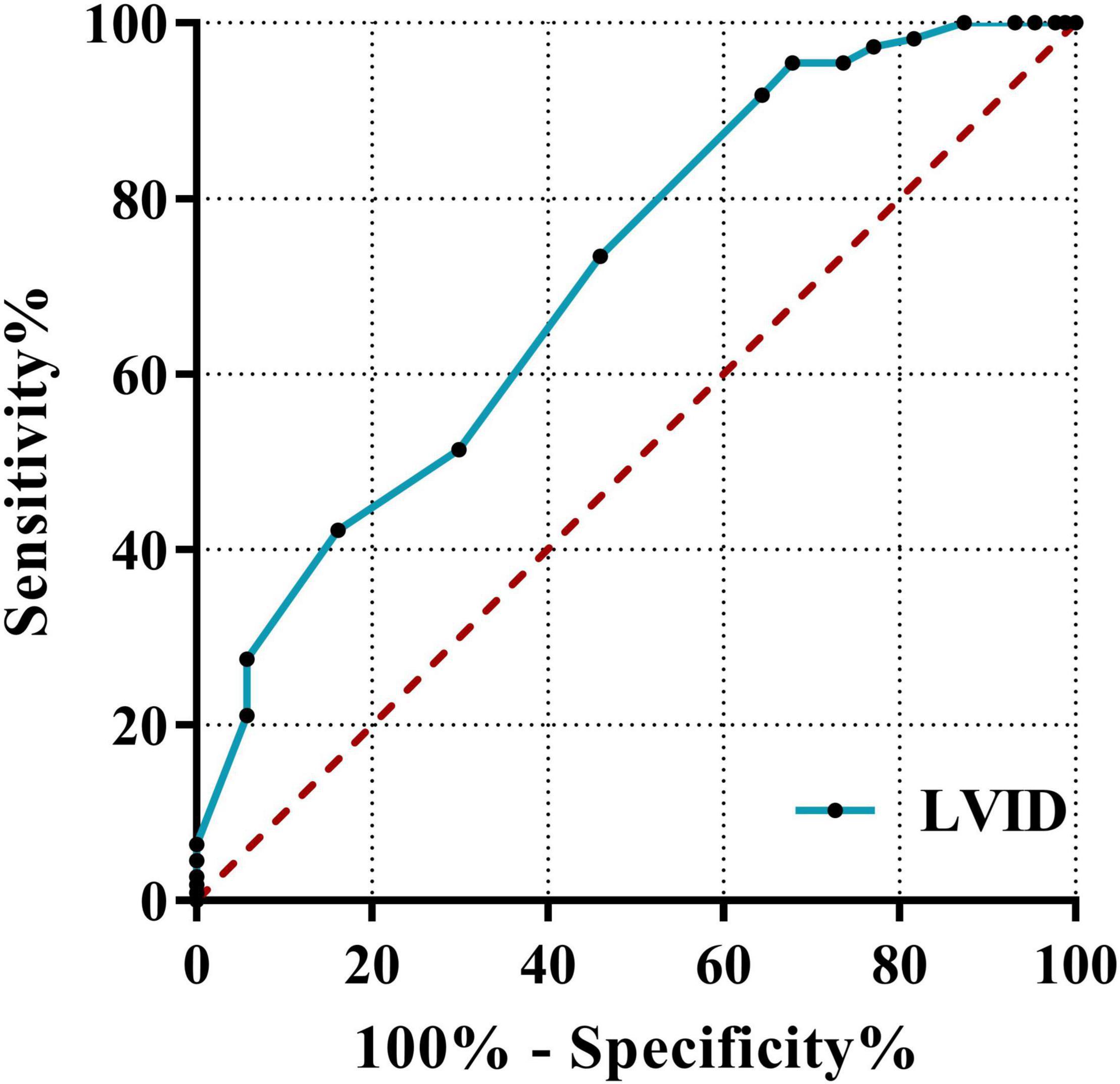
Figure 6. Receiver operating characteristic curves of LVID. The ROC curve using the clinical diagnosis of cardiac function as the dependent variable and myocardial enzymes, BNP, and echocardiography indicators as independent variables. LVID, left ventricular internal diameter; ROC, receiver operating characteristic; AUC, area under the curve.
Pearson Correlation Analysis
Pearson correlation analysis was performed for multiple variables related to the all-AL cases. The result of this analysis revealed that the percentage of bone marrow blasts was positively correlated with DD (r = 0.440), CK (r = 0.361), hs-cTnI (r = 0.433), and BNP (r = 0.379), while negatively correlated with EF (r = −0.257). Furthermore, WBC count was positively correlated with HBDH, LDH, and LDH1. LVID was positively correlated with age, CK, LDH, LDH1, EDV, ESV, and SV and negatively correlated with HGB and EF (Supplementary Table 1).
Discussion
This study examined the correlation between clinical characteristics, cardiac biomarkers, and cardiac imaging parameters in newly diagnosed AL patients. The results showed that compared with the control group, AL patients had already experienced some heart-related injuries, accompanied by a significant increase in CK-MB, LDH, hs-cTnI, BNP, and LVID, and a decrease in EF. However, the degree of cardiac damage was less than that of the CAD group. PSM analysis showed that cardiac damage in AL patients was significantly manifested in BNP, HBDH, and LVID. Subgroup analysis showed that cardiac damage was more severe in the HAL than NHAL subgroup. Furthermore, the best predictor of cardiac damage in AL patients was LVID, while age and EF were independent prognostic risk factors. The ratio of bone marrow blasts was positively correlated with the index of cardiac damage.
In recent years, cardiac tumors have been recognized as an important aspect of cardio-oncology practice where diagnosis and management are critical (23, 24). However, most scholars only focus on heart damage caused by anti-tumor therapy (25, 26). Cardiotoxicity during the treatment of AL patients has been extensively reported (27), including radiotherapy (28, 29), conventional chemotherapies (e.g., anthracyclines, antimetabolites, and cyclophosphamide) (30–32), immune checkpoint inhibitors (programmed cell death 1, programmed death ligand 1, or CTL-associated protein 4) (33, 34), and many targeted therapies, particularly monoclonal antibodies and tyrosine kinase inhibitors (35, 36), CART therapy (37–39), natural killer cell immunotherapy (40), and hematopoietic stem cell transplantation (41–43). Possible mechanisms are mediated by reactive oxygen species generated in iron-dependent chemical reactions (44), mitochondrial dysfunction (45), increased calcium flux in cardiomyocytes (46), and disorders of DNA topoisomerase 2-beta metabolism (47). However, only a small amount of research has been done on cardiac lesions directly attributed to leukemia in AL patients.
Patients with leukemia can have secondary cardiac tumors. It has been reported that cardiac tumors metastasize most frequently from the respiratory system, followed by the hematopoietic system (leukemia/multiple myeloma), and most commonly involve the pericardium, followed by myocardium, epicardium, and endocardium (14, 47, 48). Cardiac infiltration is frequently observed in leukemia patients at autopsy, with an incidence of 11–44% (48–52). However, most patients with cardiac infiltrates are clinically asymptomatic, and only a small number of cases presented pericardial effusion, hypertrophic cardiomyopathy, or AMI (15, 49, 53–59) as the first sign. There appears to be no significant difference in these microanatomical or clinical features between AML and ALL patients. Therefore, we have reason to speculate that AL itself will cause the heart lesions, and anti-cancer treatment may be an aggravating factor for cardiotoxicity. However, due to the concealment of clinical symptoms in patients with newly diagnosed AL, the incidence of cardiac dysfunction reported in the current study may only be the tip of the iceberg. Furthermore, as these cases occur more frequently in older patients with cardiac risk factors, the role of AL itself on heart disease may be overlooked.
Our analysis of cardiac-related parameters in newly diagnosed AL patients showed that compared with controls, AL patients did experience some cardiac-related lesions, both in myocardial enzymes and echocardiographic parameters. These clinical manifestations are similar to existing reports and recommendations for cardiac management monitoring in cancer patients (60–62). However, at the time of diagnosis, AL patients often present with anemia and increased WBC count that can cause a certain degree of myocardial damage, which introduces confounding factors to our research results. However, it should be noted that the WBCs of AL patients contain many leukemia cells, which differs from the WBCs of other diseases. Therefore, we matched the AL with the control group and tried to adjust for possible confounding factors, including age, sex, cardiovascular risk factors (hypertension, smoking), and hemoglobin, and found that cardiac injury in the AL group remained. In a subgroup comparison of HAL and NHAL, we found that HAL caused more severe myocardial enzymatic changes. This may be related to the stagnation and accumulation of leukemia cells (58, 63, 64). In addition, correlation analysis showed that the proportion of blast cells was positively correlated with heart damage. Although the correlation coefficient was not particularly high, it still suggested that leukemia itself may cause direct damage to the heart.
Acute leukemia disease itself may be associated with cardiac damage because it shares systemic pathogenic pathways and mechanisms with CVDs: inflammation, metabolic alterations, clonal hematopoiesis, angiogenesis alterations, and extracellular matrix and stroboscopic cells (13, 65). Cancer metabolic byproducts can also impair cardiac function. An increased amount of d-2-hydroxyglutaric acid produced by IDH2-mutant leukemia cells directly contributes to cardiac insufficiency by inhibiting α-KGDH (66). Furthermore, cancer cells can directly secrete factors that induce cardiomyocyte atrophy and metabolic changes, but the exact signaling pathways in cardiomyocytes remain poorly understood (67).
For the reasons described above, clinicians should comprehensively evaluate patients’ cardiac function before chemotherapy. Echocardiography can be used to observe changes in LVEF (68). Conditioned individuals can examine peak systolic global longitudinal strain by speckle-tracking echocardiography (69), which can detect cardiotoxicity at an early stage. In addition, cardiac biomarkers (26) such as troponin, high-sensitivity troponin, BNP, cardiac enzymes, and other potential biomarkers such as those for endothelial dysfunction, myocardial ischemia, oxidative stress and inflammation should be evaluated for a comprehensive assessment to achieve early real-time identification, assessment, and monitoring of cardiotoxicity, and timely targeted treatment. In addition, some studies (26, 70) suggest that early application of cardioprotective therapy to cancer patients may be considered, but its role has not been proven or recommended.
There were several limitations in this study. First, in order to eliminate confounding factors, we used PSM analysis, but the sample size after PSM analysis was small, which may cause some data bias and be different from the real-world situation. Second, we focused on the systolic function of the ventricle, and did not collect related indicators of ventricular diastolic function, thus possibly missing some correlations. Finally, this was a single-center observational study and the sample size was small. Larger sample size validation and prospective studies are needed to confirm the degree of cardiac damage in patients with leukemia. Nonetheless, our study provides important information about the relationship between cardiac damage and leukemia.
In summary, we identified significant differences in cardiac function between patients with primary AL and control patients, and these differences could have an impact on patient prognosis. Our findings provide new insights into the complex etiology and molecular events leading to cardiac injury in patients with AL, and how they can alert clinicians to the presence of cardiac injury in AL patients. In addition, more research is still needed to confirm and explore the specific mechanism between leukemia and cardiac lesions.
Data Availability Statement
The raw data supporting the conclusions of this article will be made available by the authors, without undue reservation.
Ethics Statement
The studies involving human participants were reviewed and approved by the Ethics Research Committee of Wuhan University in Wuhan, China. The patients/participants provided their written informed consent to participate in this study.
Author Contributions
WX, LM, YT, and FZ conceived and designed this research. LM, FY, GC, and JW were responsible for collecting the clinical outcome data of the patients included in the study. YL and TR re-checked the data. WX, YS, QW, and NZ performed the statistical analysis. WX, LM, and FZ wrote the manuscripts and created figures and tables. All authors strictly reviewed the manuscript and approved the final submitted manuscript.
Funding
This study was supported by the National Natural Science Foundation of China (81770179). The funder had no role in study design, data collection and analysis, decision to publish, or preparation of the manuscript.
Conflict of Interest
The authors declare that the research was conducted in the absence of any commercial or financial relationships that could be construed as a potential conflict of interest.
Publisher’s Note
All claims expressed in this article are solely those of the authors and do not necessarily represent those of their affiliated organizations, or those of the publisher, the editors and the reviewers. Any product that may be evaluated in this article, or claim that may be made by its manufacturer, is not guaranteed or endorsed by the publisher.
Supplementary Material
The Supplementary Material for this article can be found online at: https://www.frontiersin.org/articles/10.3389/fmed.2022.844350/full#supplementary-material
References
1. Foreman KJ, Marquez N, Dolgert A, Fukutaki K, Fullman N, McGaughey M, et al. Forecasting life expectancy, years of life lost, and all-cause and cause-specific mortality for 250 causes of death: reference and alternative scenarios for 2016-40 for 195 countries and territories. Lancet. (2018) 392:2052–90. doi: 10.1016/S0140-6736(18)31694-5
2. World Health Organization.Cardiovascular Diseases (CVDs). Geneva: World Health Organization (2021).
4. Miranda-Filho A, Pineros M, Ferlay J, Soerjomataram I, Monnereau A, Bray F. Epidemiological patterns of leukaemia in 184 countries: a population-based study. Lancet Haematol. (2018) 5:e14–24. doi: 10.1016/S2352-3026(17)30232-6
5. Ou Z, Yu D, Liang Y, He W, Li Y, Zhang M, et al. Analysis of the global burden of disease study highlights the trends in death and disability-adjusted life years of leukemia from 1990 to 2017. Cancer Commun (Lond). (2020) 40:598–610. doi: 10.1002/cac2.12094
6. de Wall C, Bauersachs J, Berliner D. Cardiooncology-dealing with modern drug treatment, long-term complications, and cancer survivorship. Clin Exp Metastasis. (2021) 38:361–71. doi: 10.1007/s10585-021-10106-x
7. Zaha VG, Hayek SS, Alexander KM, Beckie TM, Hundley WG, Kondapalli L, et al. Future perspectives of cardiovascular biomarker utilization in cancer survivors: a scientific statement from the american heart association. Circulation. (2021) 144:e551–63. doi: 10.1161/CIR.0000000000001032
8. Mulrooney DA, Yeazel MW, Kawashima T, Mertens AC, Mitby P, Stovall M, et al. Cardiac outcomes in a cohort of adult survivors of childhood and adolescent cancer: retrospective analysis of the childhood cancer survivor study cohort. BMJ. (2009) 339:b4606. doi: 10.1136/bmj.b4606
9. Haugnes HS, Wethal T, Aass N, Dahl O, Klepp O, Langberg CW, et al. Cardiovascular risk factors and morbidity in long-term survivors of testicular cancer: a 20-year follow-up study. J Clin Oncol. (2010) 28:4649–57. doi: 10.1200/JCO.2010.29.9362
10. Moslehi JJ. Cardiovascular toxic effects of targeted cancer therapies. N Engl J Med. (2016) 375:1457–67. doi: 10.1056/NEJMra1100265
11. Armenian SH, Xu L, Ky B, Sun C, Farol LT, Pal SK, et al. Cardiovascular disease among survivors of adult-onset cancer: a community-based retrospective cohort study. J Clin Oncol. (2016) 34:1122–30. doi: 10.1200/JCO.2015.64.0409
12. Sturgeon KM, Deng L, Bluethmann SM, Zhou S, Trifiletti DM, Jiang C, et al. A population-based study of cardiovascular disease mortality risk in US cancer patients. Eur Heart J. (2019) 40:3889–97. doi: 10.1093/eurheartj/ehz766
13. de Boer RA, Hulot JS, Tocchetti CG, Aboumsallem JP, Ameri P, Anker SD, et al. Common mechanistic pathways in cancer and heart failure. A scientific roadmap on behalf of the translational research committee of the heart failure association (HFA) of the European society of cardiology (ESC). Eur J Heart Fail. (2020) 22:2272–89. doi: 10.1002/ejhf.2029
14. Butany J, Leong SW, Carmichael K, Komeda M. A 30-year analysis of cardiac neoplasms at autopsy. Can J Cardiol. (2005) 21:675–80.
15. Chang K, Kim DY, Lee KH, Huh J, Kang JW, Shin DY, et al. An isolated cardiac relapse after allogeneic hematopoietic stem cell transplantation for acute lymphoblastic leukemia. Korean J Intern Med. (2017) 32:753–7. doi: 10.3904/kjim.2015.115
16. Zhang W, Azibani F, Okello E, Kayima J, Sinabulya I, Leeta J, et al. Clinical characterization, cardiovascular risk factor profile and cardiac strain analysis in a Uganda cancer population: the SATRACD study. PLoS One. (2021) 16:e249717. doi: 10.1371/journal.pone.0249717
17. Assuncao B, Handschumacher MD, Brunner AM, Yucel E, Bartko PE, Cheng KH, et al. Acute leukemia is associated with cardiac alterations before chemotherapy. J Am Soc Echocardiogr. (2017) 30:1111–8. doi: 10.1016/j.echo.2017.07.016
18. Ernst E, Hammerschmidt DE, Bagge U, Matrai A, Dormandy JA. Leukocytes and the risk of ischemic diseases. JAMA. (1987) 257:2318–24.
19. Arber DA, Orazi A, Hasserjian R, Thiele J, Borowitz MJ, Le Beau MM, et al. The 2016 revision to the world health organization classification of myeloid neoplasms and acute leukemia. Blood. (2016) 127:2391–405. doi: 10.1182/blood-2016-03-643544
20. Rollig C, Ehninger G. How I treat hyperleukocytosis in acute myeloid leukemia. Blood. (2015) 125:3246–52. doi: 10.1182/blood-2014-10-551507
21. Mendis S, Thygesen K, Kuulasmaa K, Giampaoli S, Mahonen M, Ngu BK, et al. World health organization definition of myocardial infarction: 2008-09 revision. Int J Epidemiol. (2011) 40:139–46. doi: 10.1093/ije/dyq165
22. Zeng X, Zhang Y, Kwong JS, Zhang C, Li S, Sun F, et al. The methodological quality assessment tools for preclinical and clinical studies, systematic review and meta-analysis, and clinical practice guideline: a systematic review. J Evid Based Med. (2015) 8:2–10. doi: 10.1111/jebm.12141
23. Tyebally S, Chen D, Bhattacharyya S, Mughrabi A, Hussain Z, Manisty C, et al. Cardiac tumors: JACC cardiooncology state-of-the-art review. JACC CardioOncol. (2020) 2:293–311. doi: 10.1016/j.jaccao.2020.05.009
24. Ghosh AK, Walker JM. Cardio-oncology. Br J Hosp Med (Lond). (2017) 78:C11–3. doi: 10.12968/hmed.2017.78.1.C11
25. Thavendiranathan P, Nolan MT. An emerging epidemic: cancer and heart failure. Clin Sci (Lond). (2017) 131:113–21. doi: 10.1042/CS20160412
26. Curigliano G, Cardinale D, Dent S, Criscitiello C, Aseyev O, Lenihan D, et al. Cardiotoxicity of anticancer treatments: epidemiology, detection, and management. CA Cancer J Clin. (2016) 66:309–25. doi: 10.3322/caac.21341
27. Ho G, Jonas BA, Li Q, Brunson A, Wun T, Keegan T. Early mortality and complications in hospitalized adult Californians with acute myeloid leukaemia. Br J Haematol. (2017) 177:791–9. doi: 10.1111/bjh.14631
28. Darby SC, Ewertz M, McGale P, Bennet AM, Blom-Goldman U, Bronnum D, et al. Risk of ischemic heart disease in women after radiotherapy for breast cancer. N Engl J Med. (2013) 368:987–98. doi: 10.1056/NEJMoa1209825
29. Raghunathan D, Khilji MI, Hassan SA, Yusuf SW. Radiation-induced cardiovascular disease. Curr Atheroscler Rep. (2017) 19:22. doi: 10.1007/s11883-017-0658-x
30. Anderlini P, Benjamin RS, Wong FC, Kantarjian HM, Andreeff M, Kornblau SM, et al. Idarubicin cardiotoxicity: a retrospective study in acute myeloid leukemia and myelodysplasia. J Clin Oncol. (1995) 13:2827–34. doi: 10.1200/JCO.1995.13.11.2827
31. Yeh ET, Bickford CL. Cardiovascular complications of cancer therapy: incidence, pathogenesis, diagnosis, and management. J Am Coll Cardiol. (2009) 53:2231–47. doi: 10.1016/j.jacc.2009.02.050
32. Swain SM, Whaley FS, Ewer MS. Congestive heart failure in patients treated with doxorubicin: a retrospective analysis of three trials. Cancer. (2003) 97:2869–79. doi: 10.1002/cncr.11407
33. Ji C, Roy MD, Golas J, Vitsky A, Ram S, Kumpf SW, et al. Myocarditis in cynomolgus monkeys following treatment with immune checkpoint inhibitors. Clin Cancer Res. (2019) 25:4735–48. doi: 10.1158/1078-0432.CCR-18-4083
34. Lyon AR, Yousaf N, Battisti N, Moslehi J, Larkin J. Immune checkpoint inhibitors and cardiovascular toxicity. Lancet Oncol. (2018) 19:e447–58. doi: 10.1016/S1470-2045(18)30457-1
35. Force T, Kolaja KL. Cardiotoxicity of kinase inhibitors: the prediction and translation of preclinical models to clinical outcomes. Nat Rev Drug Discov. (2011) 10:111–26. doi: 10.1038/nrd3252
36. Ewer MS, Ewer SM. Cardiotoxicity of anticancer treatments. Nat Rev Cardiol. (2015) 12:547–58. doi: 10.1038/nrcardio.2015.65
37. Lefebvre B, Kang Y, Smith AM, Frey NV, Carver JR, Scherrer-Crosbie M. Cardiovascular effects of CAR t cell therapy: a retrospective study. JACC CardioOncol. (2020) 2:193–203. doi: 10.1016/j.jaccao.2020.04.012
38. Alvi RM, Frigault MJ, Fradley MG, Jain MD, Mahmood SS, Awadalla M, et al. Cardiovascular events among adults treated with chimeric antigen receptor T-Cells (CAR-T). J Am Coll Cardiol. (2019) 74:3099–108. doi: 10.1016/j.jacc.2019.10.038
39. Goldman A, Maor E, Bomze D, Liu JE, Herrmann J, Fein J, et al. Adverse cardiovascular and pulmonary events associated with chimeric antigen receptor T-Cell therapy. J Am Coll Cardiol. (2021) 78:1800–13. doi: 10.1016/j.jacc.2021.08.044
40. Mamo T, Williams SM, Kinney S, Tessier KM, DeFor TE, Cooley S, et al. Infusion reactions in natural killer cell immunotherapy: a retrospective review. Cytotherapy. (2021) 23:627–34. doi: 10.1016/j.jcyt.2021.03.006
41. von Bonin M, Jambor HK, Teipel R, Stolzel F, Thiede C, Damm F, et al. Clonal hematopoiesis and its emerging effects on cellular therapies. Leukemia. (2021) 35:2752–8. doi: 10.1038/s41375-021-01337-8
42. Dulery R, Mohty R, Labopin M, Sestili S, Malard F, Brissot E, et al. Early cardiac toxicity associated with post-transplant cyclophosphamide in allogeneic stem cell transplantation. JACC CardioOncol. (2021) 3:250–9. doi: 10.1016/j.jaccao.2021.02.011
43. Ohmoto A, Fuji S. Cardiac complications associated with hematopoietic stem-cell transplantation. Bone Marrow Transplant. (2021) 56:2637–43. doi: 10.1038/s41409-021-01427-2
44. Zhang S, Liu X, Bawa-Khalfe T, Lu LS, Lyu YL, Liu LF, et al. Identification of the molecular basis of doxorubicin-induced cardiotoxicity. Nat Med. (2012) 18:1639–42. doi: 10.1038/nm.2919
45. Ichikawa Y, Ghanefar M, Bayeva M, Wu R, Khechaduri A, Naga PS, et al. Cardiotoxicity of doxorubicin is mediated through mitochondrial iron accumulation. J Clin Invest. (2014) 124:617–30. doi: 10.1172/JCI72931
46. Chintalgattu V, Ai D, Langley RR, Zhang J, Bankson JA, Shih TL, et al. Cardiomyocyte PDGFR-beta signaling is an essential component of the mouse cardiac response to load-induced stress. J Clin Invest. (2010) 120:472–84. doi: 10.1172/JCI39434
47. Lam KY, Dickens P, Chan AC. Tumors of the heart. A 20-year experience with a review of 12,485 consecutive autopsies. Arch Pathol Lab Med. (1993) 117:1027–31.
48. Blank N, Castellino RA. The intrathoracic manifestations of the malignant lymphomas and the leukemias. Semin Roentgenol. (1980) 15:227–45. doi: 10.1016/0037-198x(80)90049-8
49. Javier BV, Yount WJ, Crosby DJ, Hall TC. Cardiac metastasis in lymphoma and leukemia. Dis Chest. (1967) 52:481–4. doi: 10.1378/chest.52.4.481
50. Sumners JE, Johnson WW, Ainger LE. Childhood leukemic heart disease. A study of 116 hearts of children dying of leukemia. Circulation. (1969) 40:575–81. doi: 10.1161/01.cir.40.4.575
51. Kirshbaum JD, Preus FS. Leukemia: clinical and pathologic study of one hundred and twenty-three fatal cases in a series of 14,400 necropsies. Arch Intem Med. (1943) 71:777. doi: 10.1001/archinte.1943.00210060038003
52. Barcos M, Lane W, Gomez GA, Han T, Freeman A, Preisler H, et al. An autopsy study of 1206 acute and chronic leukemias (1958 to 1982). Cancer. (1987) 60:827–37. doi: 10.1002/1097-0142(19870815)60:43.0.co;2-a
53. Roberts WC, Bodey GP, Wertlake PT. The heart in acute leukemia. A study of 420 autopsy cases. Am J Cardiol. (1968) 21:388–412. doi: 10.1016/0002-9149(68)90143-4
54. Hori T, Suzuki N, Mizue N, Hatakeyama N, Takamuro M, Tsutsumi H. Relapse of T-cell all after stem cell transplant presenting as hypertrophic cardiomyopathy: the value of non-invasive diagnostic imaging in detecting cardiac leukemia. Pediatr Blood Cancer. (2006) 46:108–11. doi: 10.1002/pbc.20409
55. Butany J, Nair V, Naseemuddin A, Nair GM, Catton C, Yau T. Cardiac tumours: diagnosis and management. Lancet Oncol. (2005) 6:219–28. doi: 10.1016/S1470-2045(05)70093-0
56. Acibuca A, Yeral M, Kocer NE, Koc Z, Gullu H. Relapsed acute myeloid leukemia presenting with myocardial hypertrophy and constrictive pericardial physiology. Anatol J Cardiol. (2019) 21:287–9. doi: 10.14744/AnatolJCardiol.2019.64011
57. Arranto C, Tzankov A. Broken heart by T-prolymphocytic leukemia. Blood. (2017) 130:691. doi: 10.1182/blood-2017-05-784363
58. Cohen Y, Amir G, Da’As N, Gillis S, Rund D, Polliack A. Acute myocardial infarction as the presenting symptom of acute myeloblastic leukemia with extreme hyperleukocytosis. Am J Hematol. (2002) 71:47–9. doi: 10.1002/ajh.10155
59. Luo Z, Cheng J, Wang Y. Cardiac infiltration as the first manifestation of acute lymphoblastic leukemia: a systematic review. Front Oncol. (2022) 12:805981.
60. Pudil R, Mueller C, Celutkiene J, Henriksen PA, Lenihan D, Dent S, et al. Role of serum biomarkers in cancer patients receiving cardiotoxic cancer therapies: a position statement from the cardio-oncology study group of the heart failure association and the cardio-oncology council of the European society of cardiology. Eur J Heart Fail. (2020) 22:1966–83. doi: 10.1002/ejhf.2017
61. Curigliano G, Lenihan D, Fradley M, Ganatra S, Barac A, Blaes A, et al. Management of cardiac disease in cancer patients throughout oncological treatment: ESMO consensus recommendations. Ann Oncol. (2020) 31:171–90.
62. Alvarez-Cardona JA, Zhang KW, Mitchell JD, Zaha VG, Fisch MJ, Lenihan DJ. Cardiac biomarkers during cancer therapy: practical applications for cardio-oncology. JACC CardioOncol. (2020) 2:791–4.
63. McKee LJ, Collins RD. Intravascular leukocyte thrombi and aggregates as a cause of morbidity and mortality in leukemia. Medicine (Baltimore). (1974) 53:463–78. doi: 10.1097/00005792-197411000-00006
64. Shroff GS, Truong MT, Carter BW, Benveniste MF, Kanagal-Shamanna R, Rauch G, et al. Leukemic involvement in the thorax. Radiographics. (2019) 39:44–61. doi: 10.1148/rg.2019180069
65. Aboumsallem JP, Moslehi J, de Boer RA. Reverse cardio-oncology: cancer development in patients with cardiovascular disease. J Am Heart Assoc. (2020) 9:e13754. doi: 10.1161/JAHA.119.013754
66. Karlstaedt A, Zhang X, Vitrac H, Harmancey R, Vasquez H, Wang JH, et al. Oncometabolite d-2-hydroxyglutarate impairs alpha-ketoglutarate dehydrogenase and contractile function in rodent heart. Proc Natl Acad Sci USA. (2016) 113:10436–41. doi: 10.1073/pnas.1601650113
67. Schafer M, Oeing CU, Rohm M, Baysal-Temel E, Lehmann LH, Bauer R, et al. Ataxin-10 is part of a cachexokine cocktail triggering cardiac metabolic dysfunction in cancer cachexia. Mol Metab. (2016) 5:67–78. doi: 10.1016/j.molmet.2015.11.004
68. Plana JC, Galderisi M, Barac A, Ewer MS, Ky B, Scherrer-Crosbie M, et al. Expert consensus for multimodality imaging evaluation of adult patients during and after cancer therapy: a report from the American society of echocardiography and the European association of cardiovascular imaging. J Am Soc Echocardiogr. (2014) 27:911–39. doi: 10.1016/j.echo.2014.07.012
69. Negishi K, Negishi T, Haluska BA, Hare JL, Plana JC, Marwick TH. Use of speckle strain to assess left ventricular responses to cardiotoxic chemotherapy and cardioprotection. Eur Heart J Cardiovasc Imaging. (2014) 15:324–31. doi: 10.1093/ehjci/jet159
Keywords: acute leukemia, cardiac damage, hyperleukocytic leukemia, myocardial enzyme, echocardiography
Citation: Xiao W, Ma L, Shang Y, Yang F, Tan Y, Chen G, Wu J, Liang Y, Rouzi T, Wang Q, Zhang N and Zhou F (2022) Cardiac-Related Lesions in Newly Diagnosed Patients With Acute Leukemia: A Chinese Population-Based Real-World Study. Front. Med. 9:844350. doi: 10.3389/fmed.2022.844350
Received: 28 December 2021; Accepted: 16 May 2022;
Published: 09 June 2022.
Edited by:
Claudio Cerchione, Scientific Institute of Romagna for the Study and Treatment of Tumors (IRCCS), ItalyReviewed by:
Sinem Civriz Bozdaǧ, Ankara University, TurkeyMichael Diamantidis, University Hospital of Larissa, Greece
Copyright © 2022 Xiao, Ma, Shang, Yang, Tan, Chen, Wu, Liang, Rouzi, Wang, Zhang and Zhou. This is an open-access article distributed under the terms of the Creative Commons Attribution License (CC BY). The use, distribution or reproduction in other forums is permitted, provided the original author(s) and the copyright owner(s) are credited and that the original publication in this journal is cited, in accordance with accepted academic practice. No use, distribution or reproduction is permitted which does not comply with these terms.
*Correspondence: Fuling Zhou, zhoufuling@whu.edu.cn
†These authors have contributed equally to this work and share first authorship