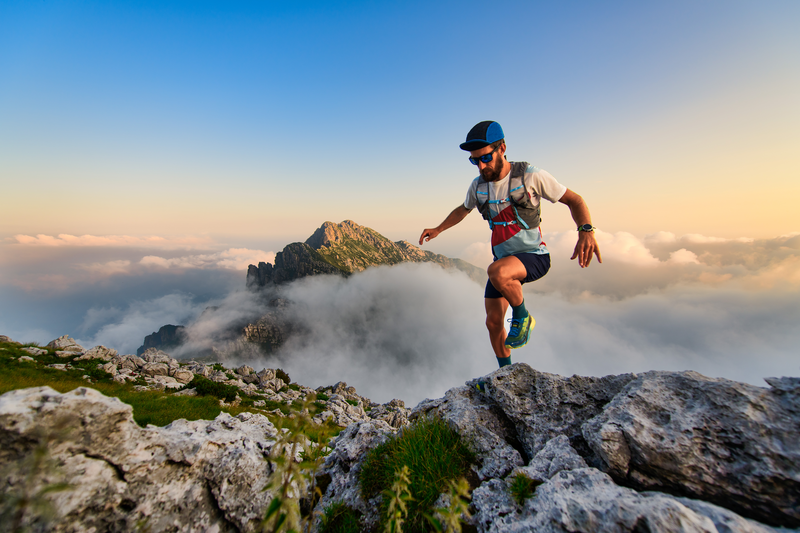
94% of researchers rate our articles as excellent or good
Learn more about the work of our research integrity team to safeguard the quality of each article we publish.
Find out more
ORIGINAL RESEARCH article
Front. Med. , 15 April 2022
Sec. Intensive Care Medicine and Anesthesiology
Volume 9 - 2022 | https://doi.org/10.3389/fmed.2022.839787
This article is part of the Research Topic Multidisciplinary Critical Care Medicine – Getting Things Done Across Specialties View all 15 articles
Background: The main aim of this study was to evaluate the effect of oxygen saturation on mortality in critically ill patients with mechanical ventilation according to obesity status.
Methods: We conducted an observational study in mechanically ventilated patients admitted to the ICU retrospectively. Demographic, arterial blood gas, ventilator setting, interventions, and peripheral oxygen saturation (Spo2) during the first 24 h were recorded and analyzed between non-obese and obese patients. The main exposure included Spo2, time-weighted mean Spo2 (TWM-Spo2), and proportion of time spent in different Spo2 (PTS-Spo2) levels. The primary outcome was hospital mortality. We used multivariable logistic regression models to assess the relationship between Spo2 and mortality, as well as the interaction between PTS-Spo2 and obesity status.
Results: A total of 25,100 patients were included, of which 10,564 (42%) were obese patients. After adjusting for confounders, compared with TWM-Spo2 of 94–98%, TWM-Spo2 of < =88% (OR 3.572; CI [2.343, 5.455]; p < 0.001) and of 89–93% (OR 1.514; CI [1.343, 1.706]; p < 0.001) were both associated with higher risk of mortality. PTS-Spo2 of 99–100% was associated with increased risk of mortality for obese patients (OR 1.028; 95% CI 1.010–1.046; p = 0.002; Pinteraction = 0.001), while PTS-Spo2 of 89–93% was associated with increased risk of mortality (OR 1.089; 95% CI 1.051–1.128; p < 0.001; Pinteraction = 0.001) for non-obese patients.
Conclusions: For obese and non-obese critically ill patients with mechanical ventilation, the impact of oxygen saturation on hospital mortality is different.
Obesity has emerged as one of the leading health concerns over the past century (1). In the United States, for the years 2013 and 2014, the overall obesity prevalence was 37.7% (2). Moreover, for adult obesity and severe obesity, the prevalence will continue to increase (3). Recent studies estimate by 2030 nearly 1 in 2 adults will have obesity (4, 5). At the same time, obese patients are over-represented in primary care (6). The similar circumstance is also reflected in critically ill patients where a recent study reported a prevalence of around 20% for the year 2012 (7, 8). Moreover, obese patients represent a specific population that seemed to be associated with high morbidity and increased resource utilization, who require an adapted ICU management (7–10).
The provision of supplemental oxygen is a ubiquitous intervention for mechanically ventilated patients. However, excessive oxygen could also be injurious (11, 12). British Thoracic Society (BTS) recommends, for morbidly obese patients who are at the risk of hypoventilation, even without evidence of coexistent obstructive sleep apnea, targeted oxygen saturation of 88–92% should be titrated (grade D) (13). Two small trials conducted in morbidly obese patients showed exposure to hyperoxia caused elevated partial pressure of carbon dioxide (Paco2) and hypoventilation (14, 15). However, for patients with milder obesity, the literature is really sparse, especially concerning the impact in the critical setting. Moreover, the BTS or Thoracic Society of Australia and New Zealand guidelines did not cover the area of ICU (13, 16). Given the epidemiologic and specific pathophysiologic changes observed in obese patients, one could hypothesize that the obesity status could be an important confounder in the relationship between supplemental oxygen and outcomes. To the best of our knowledge, no study has specifically evaluated the relationship between supplemental oxygen and hospital mortality outcome in obese critically ill patients with mechanical ventilation.
The main aim of this study was to analyze the influence of the obesity status on the relationship between oxygen saturation and the hospital mortality in mechanically ventilated patients. The hypothesis was the impact of the supplemental oxygen on mortality in non-obese and obese critically ill patients with mechanical ventilation may be different.
This study used data stored in the high-resolution eICU (eicu-crd.mit.edu) database, which comprises 200,859 admissions for 139,367 unique patients between 2014 and 2015 at 208 hospitals located throughout the United States. The database contains parameters that were available in the routine ICU clinical information system, including admission diagnosis, APACHE IV score and components, laboratory measurements, vital signs, medications, and special treatments. The elaborate description of eICU can be found elsewhere (17).
The eICU database was certified as meeting safe harbor standards by Privacert (Cambridge, MA) (Health Insurance Portability and Accountability Act Certification no. 1031219-2). The institutional review board (IRB) approval was exempt due to the retrospective and re-identification design. The author (certification number: 28795067) was approved to access the database for research aims after completing the National Institutes of Health web-based training course, which was “Protecting Human Research Participants.”
All recorded patients in the eICU database were eligible for inclusion. The first ICU stay was selected for those who were admitted to ICU for more than once. We selected adult patients with invasively mechanical ventilation during the first 24 h after admission to ICU. Patients were excluded for the following reasons: (1) ICU length of stay <24 h, (2) Incomplete hospital mortality recording, (3) Missing Spo2 data, (4) Percentage of recorded Spo2 < =50%, values of Spo2 <70% were excluded based on such values were likely to be not accurate, (5) Missing height or weight data, (6) Missing APACHE IV score, (7) Ventilation with ambient air, i.e., fraction of inspired oxygen (Fio2) = 21%.
The following information data during the first 24 h of admission were extracted: age, gender, height, weight, ethnicity, comorbidities, admission diagnosis, ICU types, Acute Physiology and Chronic Health Evaluation (APACHE) IV score and components, and sequential organ failure assessment (SOFA) score. The use of dialysis, vasopressors, mechanical ventilation setting during the first 24 h were also collected. However, we could not extract the pattern of mechanical ventilation. Patients with a body mass index (BMI) ≥30 kg/(m2) were defined as obese according to the international standards (18), where BMI was calculated as body weight /(height2).
We included all arterial blood gas (ABG) samples that were obtained during the first 24 h admitted to the ICU. The time-weighted mean partial pressure of arterial oxygen (Pao2) (TWM- Pao2), Paco2 (TWM-Paco2), pH (TWM-pH), and Fio2 (TWM- Fio2) were calculated for every patient. The time-weighted mean (TWM) data was calculated as an area under the curve (AUC) by integrating all time-sequence data divided by the whole time (24 h). The duration of the first measured value represented the time from admission (0 h) to the first measurement time. The duration for the last measured value was the measurement time to 24 h in the ICU. TWM- Pao2, TWM-Paco2, and TWM-pH were considered as categorical variables, and patients with missing ABG data of Pao2, Paco2, and pH were considered as a unique category, respectively.
The Spo2, which was obtained during the first 24 h after ICU admission, were generally recorded from bedside vital signs monitors as 5-min median values. According to the BTS guideline for oxygen use in adults in healthcare and emergency settings (13), four categories were generated, which were < =88%, 89–93%, 94–98%, and 99–100%. TWM-Spo2 was calculated for every patient, and patients were classified into different Spo2 categories. We separately calculated the proportion of time spent in Spo2 (PTS-Spo2) of four categories during the first 24 h, defined as the time in each Spo2 category divided by total time. For each patient, PTS-Spo2 in each of the four predefined categories ranged from 0 to 100%, and the total proportion was 100%. A similar method was used in other studies (19, 20).
We selected the hospital mortality, defined as dead at hospital discharge as the primary outcome.
Categorical variables were reported as numbers and percentages and were analyzed with Chi-square test or Fisher's exact test as appropriate. Continuous variables are shown as median and interquartile range (IQR) or mean and standard deviation (SD), which were compared using Wilcoxon rank-sum test or Student's t-test, respectively. As for outliers in the database, which were defined as the recording lies outside the 3 × IQR range, were re-checked and replaced by the 5th or 95th percentile. As for missing values, the number was clearly stated for each variable. Patients with missing data of Pao2, Paco2, and pH were considered as a categorical variable, and multiple imputation was performed for missing data of TWM-Fio2.
First, a descriptive analysis was performed in non-obese and obese patients, including baseline characteristics and clinical parameters. Then, a univariate analysis was done according to survival at hospital discharge in non-obese and obese patients. We used the multivariable logistic regression models to assess the relationship between TWM-Spo2 categories and hospital mortality after adjusting for covariates. The association between each PTS-Spo2 of different categories and hospital mortality was investigated with multivariable logistic regression. The variables that were considered clinically relevant or that showed a univariate relationship with the outcome (p < 0.10) were selected into the multivariable model. A stepwise backward elimination method with a significance level of 0.05 was used to build the final model. The variance inflation factor was used to examine the multicollinearity, and the Hosmer-Lemeshow goodness-of-fit test to assess the calibration of the models.
PTS-Spo2 of different categories was compared between non-obese and obese patients. The interaction between PTS-Spo2 (as a continuous variable) and obesity status (obese and non-obese, as a categorical variable) was explored by the multivariable logistic regression model.
As for the data extraction, PostgreSQL (version 10, www.postgresql.org) was used. A two-sided p-value of <0.05 was considered statistically significant. We used the R software (version 3.5.1, www.r-project.org) to conduct all the statistical analyses.
Figure 1 showed the flow chart of the study. After exclusion, a total of 25,100 patients met our inclusion criteria, of which 10,564 (42%) were obese patients and 14,534 (58%) were non-obese patients. The median BMI in the total population was 28.3 (IQR 23.9–34.4) kg/m2, of which the non-obese was 25 (IQR 22–27) kg/m2 and the obese was 36 (IQR 33–42) kg/m2. The median age was 65 (IQR 54–75) years, 13,632 (54%) was male. 4,242 (17%) patients were non-survivors at hospital discharge. Demographics and baseline characteristics between the obese and non-obese were presented in Table 1.
Figure 1. Flow chart of subject selection. eICU, telehealth intensive care unit; Spo2, peripheral oxygen saturation; Fio2, fraction of inspiration oxygen.
In non-obese patients, the median age was 67 (IQR 55–78) and the hospital mortality was 18%. BMI of non-obese patients was higher in survivors than in non-survivors (25 vs. 24, p < 0.001). Non-obese patients with comorbidities of hypertension or chronic renal insufficiency had higher hospital mortality (Table 1). Non-obese survivors had a higher percentage of TWM-Spo2 of 99–100% (Table 2).
In obese patients, the median age was 63 (IQR 53–72) and hospital mortality was 15%, which was lower than non-obese patients (p < 0.001). Obese survivors had higher BMI than non-survivors (36 vs. 35, p = 0.014). Obese patients had a higher percentage of hypertension, diabetes mellitus, chronic heart failure, chronic obstructive pulmonary disease, and chronic renal insufficiency than the non-obese. Interestingly, obese survivors had a higher percentage of diabetes mellitus (33% vs. 30%, p = 0.009). Obese survivors had lower percentage of TWM-Spo2 of 99–100% (11% vs. 14%) and higher percentage of TWM-Paco2 > 45 mmHg (30 vs. 26%) (Table 2). Obese patients had lower PTS-Spo2 of 99–100% than non-obese patients (39.8% vs. 54.8%, p < 0.001) (Table 3).
The relationship between TWM-Spo2 categories and hospital mortality, which was assessed by the multivariable logistic models after adjusting confounders, was comparing with 94–98% category, < =88% category (OR 3.572; CI [2.343, 5.455]; p < 0.001) and 89–93% category (OR 1.514; CI [1.343, 1.706]; p < 0.001) both had higher odds ratio for hospital mortality, while 99–100% category was not associated with hospital mortality (Figure 2A and Supplementary Table 1). After multivariable analysis, PTS-Spo2 of < =88% (OR 1.445; CI [1.356, 1.541]; p < 0.001; per 10% increase) and PTS-Spo2 of 89–93% (OR 1.080; CI [1.052, 1.108]; p < 0.001; per 10% increase) had higher odds ratio for hospital mortality, PTS-Spo2 of 94–98% (OR 0.968; CI [0.955, 0.981]; p < 0.001; per 10% increase) had lower odd ratio for hospital mortality, and PTS-Spo2 of 99–100% (OR 0.997; CI [0.986, 1.009]; p = 0.636; per 10% increase) was not associated with high risk of hospital mortality (Figure 2B and Supplementary Tables 2–5).
Figure 2. Left (A) Adjusted odds ratio for hospital mortality according to different TWM-Spo2 categories. The adjusted odds ratio for each Spo2 level and 95% confidence intervals (error bars) were calculated (TWM-Spo2 of 94–98% as reference). Right (B) Adjusted odds ratio for hospital mortality according to PTS-Spo2 categories separately. An odds ratio is calculated per 10% increase in time in each given category. All models (i.e., five models, one for “A” and four for “B”) were separately adjusted for age, BMI categories, admission diagnosis, diabetes mellitus, Sequential Organ Failure Assessment score (not including points for the respiratory part), TWM-Fio2, TWM-Paco2, and TWM-pH categories. TWM, time-weighted mean; Spo2, peripheral oxygen saturation; PTS, proportion of time spent; BMI, body mass index; Fio2, fraction of inspiration oxygen; Paco2, partial pressure of arterial carbon dioxide. Full multivariable models see Supplementary Tables 1–5.
The multivariable models including the interaction between PTS-Spo2 and obesity status (non-obese and obese) showed that there was a significant interaction between PTS-Spo2 and obesity status (Table 3). While the PTS-Spo2 of 99-100% was not significantly associated with hospital mortality outcome (OR 1.004; 95% CI 0.990-1.018; p = 0.597; per 10% increase) in non-obese patients, it was associated with increased risk of mortality in obese patients (OR 1.028; 95% CI 1.010-1.046; p = 0.002; per 10% increase) (Figure 3A). While the PTS-Spo2 of 89-93% was significantly associated with increased risk of hospital mortality outcome (OR 1.089; 95% CI 1.051-1.128; p <0.001; per 10% increase) in non-obese patients, it was not associated with hospital mortality in obese patients (OR 1.022; 95% CI 0.985-1.060; p = 0.235; per 10% increase) (Figure 3B). Supplementary Figure 3 showed the interaction between PTS-Spo2 of < = 88% and 94-98% and obesity status (obese and non-obese).
Figure 3. Interaction between PTS-Spo2 and obesity status (obese and non-obese). Upper (A) While the PTS-Spo2 of 99–100% was not significantly associated with hospital mortality outcome (OR 1.004; 95% CI 0.990–1.018; p = 0.597; per 10% increase) in non-obese patients, it was associated with increased risk of mortality in obese patients (OR 1.028; 95% CI 1.010–1.046; p = 0.002; per 10% increase). Lower (B) While the PTS-Spo2 of 89–93% was significantly associated with increased risk of hospital mortality outcome (OR 1.089; 95% CI 1.051–1.128; p < 0.001; per 10% increase) in non-obese patients, it was not associated with hospital mortality in obese patients (OR 1.022; 95% CI 0.985–1.060; p = 0.235; per 10% increase). PTS-Spo2, proportion of time spent in peripheral oxygen saturation, OR odds ratio, CI confidential interval.
The results of our study provide evidence to support our hypothesis. The impact of supplemental oxygen on hospital mortality was different in non-obese and obese critically ill patients with mechanical ventilation. Spo2 of 99–100% was associated with a higher hospital mortality for obese patients but was not statistically significant for non-obese patients. Spo2 of 94–98% was associated with decreased risk of hospital mortality outcome for both obese and non-obese patients. Spo2 of 89–93% was associated with higher mortality for non-obese patients, but not statistically significant for obese patients. Spo2 of < =88% was associated with an increased risk of hospital mortality outcome in both non-obese patients and obese patients. This is the first study to assess the relationship between supplemental oxygen and mortality in the specific population of obese critically ill patients with mechanical ventilation. The results of the present study showed that supplemental oxygen therapy should consider the obesity status into account.
The Oxygen-ICU randomized clinical trial showed among critically ill patients with an ICU length of stay of 72 h or longer, conservative therapy (Spo2 94–98%) vs. conventional therapy (Spo2 97–100%) resulted in lower ICU mortality (11). However, ICU-ROX trial found in adults undergoing mechanical ventilation in the ICU, conservative oxygen therapy (Spo2 91–96%), as compared with usual oxygen therapy (Spo2 ≥91%), did not significantly affect the number of ventilator-free days or mortality (21). In the present study, compared with the TWM-Spo2 94–98% category, TWM-Spo2 of 99–100% was not associated with hospital mortality. However, when considering the dose of Spo2, PTS-Spo2 of 94–98% was associated with lower hospital mortality in overall patients. The results of our study were consistent with the two RCTs.
There were some explanations of the differences observed between the non-obese and obese groups. First, obese patients can cause hypoxemia by decreasing lung volumes and may be at risk for hyperoxia-induced hypercapnia (22, 23). Two small trials conducted in morbidly obese patients with obesity hypoventilation syndrome (OHS) showed an elevated baseline Paco2. After a 20-min exposure to hyperoxia, the patients had a mean increase in Paco2 of about 4.4 mmHg (14, 15). However, whether hyperoxia-induced hypercapnia occurs in patients with milder obesity and without hypoventilation is unclear. Denault et al. found in obese patients with mean BMI 34 kg/m2 after cardiac surgery, there was no clinically important increase in Paco2 associated with higher Spo2 values (24). Second, obese patients had higher rate of comorbidities, like COPD, chronic heart failure, diabetes mellitus, of which hyperoxia could exacerbate the outcome (25, 26). In the present study, percentage of COPD or heart failure was higher in obese than non-obese patients. Interestingly, in obese patients, survivors had a higher percentage of diabetes mellitus than non-survivors, which was not the case in the non-obese patients. Vught LA et al. also found diabetes was not associated with adjusted 90-day mortality risk in critically ill patients admitted with sepsis (27). Third, hyperoxia may result in nitric oxide depletion and induction of oxidative stress on the adipose tissue, which may exacerbate the critical illness (28).
The results of the current study suggest that compared with non-obese patients, excessive oxygen may be injurious for obese patients. However, targeted oxygenation was still unknown. BTS guideline recommends for morbidly obese patients (BMI > 40 kg/m2), a target saturation of 88–92% should be titrated to maintain (grade D) (13). However, the literature was really sparse. In the present study, for obese patients with mechanical ventilation, Spo2 of < =88% may be associated with poor outcome, 89–93% was not associated with mortality, 94–98% was associated with lower mortality, and 99–100% was associated with higher mortality. The results suggested Spo2 99–100% was not appropriate for obese patients. On the other hand, in obese patients, non-survivors had a higher percentage of TWM-Pao2 of 120–300 mmHg and >300 mmHg, which also suggested hyperoxia may be detrimental for obese patients.
For overall and non-obese patients, PTS-Spo2 of 89–93% was associated with increased hospital mortality in the present study. The contribution of modest hypoxemia to mortality is unknown. The recommendation of oxygen supply by guidelines mainly based on the normal ranges of Spo2 (13, 16). A North American study found Spo2 at sea level for adults with two standard deviation range is 94–98% (29). However, the standard reference ranges obtained from healthy volunteers may differ from the analogous range generated from data of ICU patients (30). Another larger observational study of over 37,000 patients admitted to four acute medical admissions units found that median Spo2 was 98% (IQR 97–99%) for young adults and 96% (IQR 95–98%) for old adults (31). They also found for patients with initial Spo2 values of 89, 90, 91, 92, and 93% had higher mortality and the 95% confidential intervals did not overlap with those initial Spo2 values of ≥95% (31). However, clearly, there were limitations to the raw results without adjusting for confounders.
The present study has several other strengths. First, a total of 25,100 patients was extracted from ICUs in 166 hospitals, which allowed for the adjustment of multiple confounding factors, making the findings more generalizable. Second, the total observations of Spo2 were 6,925,863, the average observations of Spo2 were 276 for each patient, accounting for the time of 95.8% in the first 24 h, which made the results robust. Third, we calculated both the TWM-Spo2 and PTS-Spo2, which considered the dose of Spo2.
However, several obvious limitations should be mentioned. First, the retrospective design in nature was subjected to the inherent limitations. This study design may only show statistical associations and but not causality between PTS-Spo2 and hospital mortality. Second, although the multivariable logistic regression to was used to adjust for potential confounders, the potential confounding factors (e.g., altitude, smoking status et al.) which were not included in the analysis, could lead to biased results. Third, some data could be invalidated due to the acquisition directly from the bedside vital sign monitors. Some of the data had not been checked and verified by a bedside care provider, i.e., the measurements may be noisy and may not reflect the true status. However, the Spo2 values recorded by the nurse and observed by the monitor at the same time were highly correlated (Supplementary Figure 4). Fourth, we only extracted the data for the first 24 h, which could not represent the entire course of stay in ICU. Fifth, we arbitrarily classified the Spo2 into four categories, < =88%, 89–93, 94–98%, and 99–100%, the rationality may need further research. Sixth, although Spo2 is widely used for clinical practice for continuously assessing the oxygenation in critically ill patients, blood gasses analyses are still the golden standard, especially in assessing the severity of hypoxemia and other gas exchange anomalies. Spo2 may not display the real oxygenation status. Finally, we excluded patients without APACHE IV score, whose diagnoses were mainly burns and certain organ transplantation (17, 32), which may cause biases.
The impact of supplemental oxygen on hospital mortality was different in non-obese and obese critically ill patients with mechanical ventilation. Spo2 of 99–100% was associated with higher mortality for obese patients, while Spo2 of 89–93% was associated with higher mortality for non-obese patients.
Publicly available datasets were analyzed in this study. This data can be found here: eicu-crd.mit.edu.
The eICU was exempt from Institutional Review Board (IRB) approval due to the retrospective design, lack of direct patient intervention, and the security schema, for which the re-identification risk was certified as meeting safe harbor standards by Privacert (Cambridge, MA) (Health Insurance Portability and Accountability Act Certification no. 1031219-2). Due to the HIPAA compliant de-identification in this database, our institutional IRB requirement was waived.
DZ and TL conceived this study and reviewed and modified the final manuscript. DZ extracted the data. TL, DZ, DW, and CW designed and performed the statistical analyses. DZ, TL, QL, RZ, and DZ wrote the first draft of the manuscript. All authors read, critically reviewed, and approved the final manuscript.
This study was supported by Youth Top-notch Talent Program of Beijing Tongren Hospital.
The authors declare that the research was conducted in the absence of any commercial or financial relationships that could be construed as a potential conflict of interest.
All claims expressed in this article are solely those of the authors and do not necessarily represent those of their affiliated organizations, or those of the publisher, the editors and the reviewers. Any product that may be evaluated in this article, or claim that may be made by its manufacturer, is not guaranteed or endorsed by the publisher.
The Supplementary Material for this article can be found online at: https://www.frontiersin.org/articles/10.3389/fmed.2022.839787/full#supplementary-material
1. Heymsfield SB, Wadden TA. Mechanisms, pathophysiology, and management of obesity. N Engl J Med. (2017) 376:254–66. doi: 10.1056/NEJMra1514009
2. Flegal KM, Kruszon-Moran D, Carroll MD, Fryar CD, Ogden CL. Trends in obesity among adults in the United States, 2005 to 2014. JAMA. (2016) 315:2284–91. doi: 10.1001/jama.2016.6458
3. Hales CM, Fryar CD, Carroll MD, Freedman DS, Ogden CL. Trends in obesity and severe obesity prevalence in US youth and adults by sex and age, 2007-2008 to 2015-2016. JAMA. (2018) 319:1723–5. doi: 10.1001/jama.2018.3060
4. Ward ZJ, Bleich SN, Cradock AL, Barrett JL, Giles CM, Flax C, et al. Projected U.S. state-level prevalence of adult obesity and severe obesity. N Engl J Med. (2019) 381:2440–50. doi: 10.1056/NEJMsa1909301
5. Finkelstein EA, Khavjou OA, Thompson H, Trogdon JG, Pan L, Sherry B, et al. Obesity and severe obesity forecasts through 2030. Am J Prev Med. (2012) 42:563–70. doi: 10.1016/j.amepre.2011.10.026
6. Stecker T, Sparks S. Prevalence of obese patients in a primary care setting. Obesity. (2006) 14:373–6. doi: 10.1038/oby.2006.49
7. Sakr Y, Alhussami I, Nanchal R, Wunderink RG, Pellis T, Wittebole X, et al. Being overweight is associated with greater survival in ICU patients: results from the intensive care over nations audit. Crit Care Med. (2015) 43:2623–32. doi: 10.1097/CCM.0000000000001310
8. Schetz M, De Jong A, Deane AM, Druml W, Hemelaar P, Pelosi P, et al. Obesity in the critically ill: a narrative review. Intensive Care Med. (2019) 45:757–69. doi: 10.1007/s00134-019-05594-1
9. Rosvall BR, Forgie K, MacLeod JB, Yip AM, Aguiar C, Lutchmedial S, et al. Impact of obesity on intensive care unit resource utilization after cardiac operations. Ann Thorac Surg. (2017) 104:2009–15. doi: 10.1016/j.athoracsur.2017.05.047
10. De Jong A, Chanques G, Jaber S. Mechanical ventilation in obese ICU patients: from intubation to extubation. Crit Care. (2017) 21:63. doi: 10.1186/s13054-017-1641-1
11. Girardis M, Busani S, Damiani E, Donati A, Rinaldi L, Marudi A, et al. Effect of conservative vs conventional oxygen therapy on mortality among patients in an intensive care unit: the oxygen-ICU randomized clinical trial. JAMA. (2016) 316:1583–9. doi: 10.1001/jama.2016.11993
12. Chu DK, Kim LH, Young PJ, Zamiri N, Almenawer SA, Jaeschke R, et al. Mortality and morbidity in acutely ill adults treated with liberal versus conservative oxygen therapy (IOTA): a systematic review and meta-analysis. Lancet. (2018) 391:1693–705. doi: 10.1016/S0140-6736(18)30479-3
13. O'Driscoll BR, Howard LS, Earis J, Mak V. BTS guideline for oxygen use in adults in healthcare and emergency settings. Thorax. (2017) 72(Suppl. 1):ii1–90. doi: 10.1136/thoraxjnl-2016-209729
14. Hollier CA, Harmer AR, Maxwell LJ, Menadue C, Willson GN, Unger G, et al. Moderate concentrations of supplemental oxygen worsen hypercapnia in obesity hypoventilation syndrome: a randomised crossover study. Thorax. (2014) 69:346–53. doi: 10.1136/thoraxjnl-2013-204389
15. Wijesinghe M, Williams M, Perrin K, Weatherall M, Beasley R. The effect of supplemental oxygen on hypercapnia in subjects with obesity-associated hypoventilation: a randomized, crossover, clinical study. Chest. (2011) 139:1018–24. doi: 10.1378/chest.10-1280
16. Beasley R, Chien J, Douglas J, Eastlake L, Farah C, King G, et al. Thoracic Society of Australia and New Zealand oxygen guidelines for acute oxygen use in adults: 'Swimming between the flags'. Respirology. (2015) 20:1182–91. doi: 10.1111/resp.12620
17. Pollard TJ, Johnson AEW, Raffa JD, Celi LA, Mark RG, Badawi O. The eICU Collaborative Research Database, a freely available multi-center database for critical care research. Sci Data. (2018) 5:180178. doi: 10.1038/sdata.2018.178
18. Pi-Sunyer FX, Becker DM, Bouchard C, Carleton RA, Colditz GA, Dietz WH, et al. Executive summary of the clinical guidelines on the identification, evaluation, and treatment of overweight and obesity in adults. Arch Intern Med. (1998) 158:1855–67. doi: 10.1001/archinte.158.17.1855
19. Vaahersalo J, Bendel S, Reinikainen M, Kurola J, Tiainen M, Raj R, et al. Arterial blood gas tensions after resuscitation from out-of-hospital cardiac arrest: associations with long-term neurologic outcome. Crit Care Med. (2014) 42:1463–70. doi: 10.1097/CCM.0000000000000228
20. Elmer J, Scutella M, Pullalarevu R, Wang B, Vaghasia N, Trzeciak S, et al. The association between hyperoxia and patient outcomes after cardiac arrest: analysis of a high-resolution database. Intensive Care Med. (2015) 41:49–57. doi: 10.1007/s00134-014-3555-6
21. ICU-ROX Investigators and the Australian and New Zealand Intensive Care Society Clinical Trials Group Mackle D Bellomo R Bailey M . Conservative oxygen therapy during mechanical ventilation in the ICU. N Engl J Med. (2019) 382:989–98. doi: 10.1056/NEJMoa1903297
22. Littleton SW, Tulaimat A. The effects of obesity on lung volumes and oxygenation. Respir Med. (2017) 124:15–20. doi: 10.1016/j.rmed.2017.01.004
23. Nowbar S, Burkart KM, Gonzales R, Fedorowicz A, Gozansky WS, Gaudio JC, et al. Obesity-associated hypoventilation in hospitalized patients: prevalence, effects, and outcome. Am J Med. (2004) 116:1–7. doi: 10.1016/j.amjmed.2003.08.022
24. Denault MH, Ruel C, Simon M, Bouchard PA, Simard S, Lellouche F. Evaluation of hyperoxia-induced hypercapnia in obese patients after cardiac surgery: a randomized crossover comparison of conservative and liberal oxygen administration. Can J Anaesth. (2019) 67:194–202. doi: 10.1007/s12630-019-01500-x
25. Austin MA, Wills KE, Blizzard L, Walters EH, Wood-Baker R. Effect of high flow oxygen on mortality in chronic obstructive pulmonary disease patients in prehospital setting: randomised controlled trial. BMJ. (2010) 341:c5462. doi: 10.1136/bmj.c5462
26. Smit B, Smulders YM, van der Wouden JC, Oudemans-van Straaten HM, Spoelstra-de Man AME. Hemodynamic effects of acute hyperoxia: systematic review and meta-analysis. Crit Care. (2018) 22:45. doi: 10.1186/s13054-018-1968-2
27. van Vught LA, Holman R, de Jonge E, de Keizer NF, van der Poll T. Diabetes Is Not Associated With Increased 90-Day Mortality Risk in Critically Ill Patients With Sepsis. Crit Care Med. (2017) 45:e1026–e1035. doi: 10.1097/CCM.0000000000002590
28. Gonzalez-Muniesa P, Garcia-Gerique L, Quintero P, Arriaza S, Lopez-Pascual A, Martinez JA. Effects of hyperoxia on oxygen-related inflammation with a focus on obesity. Oxid Med Cell Longev. (2015) 2015:8957827. doi: 10.1155/2016/8957827
29. Crapo RO, Jensen RL, Hegewald M, Tashkin DP. Arterial blood gas reference values for sea level and an altitude of 1,400 meters. Am J Respir Crit Care Med. (1999) 160(5 Pt. 1):1525–31. doi: 10.1164/ajrccm.160.5.9806006
30. Tyler PD, Du H, Feng ML, Bai R, Xu ZL, Horowitz GL, et al. Assessment of intensive care unit laboratory values that differ from reference ranges and association with patient mortality and length of stay. JAMA Netw Open. (2018) 1:e184521. doi: 10.1001/jamanetworkopen.2018.4521
31. Smith GB, Prytherch DR, Watson D, Forde V, Windsor A, Schmidt PE, et al. S(p)O(2) values in acute medical admissions breathing air–implications for the British Thoracic Society guideline for emergency oxygen use in adult patients? Resuscitation. (2012) 83:1201–5. doi: 10.1016/j.resuscitation.2012.06.002
Keywords: obesity, intensive care unit, mechanical ventilation, oxygen saturation, mortality
Citation: Li T, Zhou D, Zhao D, Lin Q, Wang D, Wang C and Zhang R (2022) Impact of Oxygen Saturation on Mortality in Obese and Non-obese Critically Ill Patients With Mechanical Ventilation: A Retrospective Observational Study. Front. Med. 9:839787. doi: 10.3389/fmed.2022.839787
Received: 20 December 2021; Accepted: 25 March 2022;
Published: 15 April 2022.
Edited by:
Björn Tampe, University Medical Center Göttingen, GermanyReviewed by:
Yi Yang, Zhongda Hospital, Southeast University, ChinaCopyright © 2022 Li, Zhou, Zhao, Lin, Wang, Wang and Zhang. This is an open-access article distributed under the terms of the Creative Commons Attribution License (CC BY). The use, distribution or reproduction in other forums is permitted, provided the original author(s) and the copyright owner(s) are credited and that the original publication in this journal is cited, in accordance with accepted academic practice. No use, distribution or reproduction is permitted which does not comply with these terms.
*Correspondence: Tong Li, dHJpY3VtZEAxMjYuY29t
†These authors have contributed equally to this work
Disclaimer: All claims expressed in this article are solely those of the authors and do not necessarily represent those of their affiliated organizations, or those of the publisher, the editors and the reviewers. Any product that may be evaluated in this article or claim that may be made by its manufacturer is not guaranteed or endorsed by the publisher.
Research integrity at Frontiers
Learn more about the work of our research integrity team to safeguard the quality of each article we publish.