- 1Shenzhen Eye Hospital, Shenzhen Eye Institute, Jinan University, Shenzhen, China
- 2Department of Ophthalmology, Hunan Key Laboratory, National Clinical Research Center for Geriatric Disorders, Xiangya Hospital, Central South University, Changsha, China
Proliferative vitreoretinopathy (PVR), an inflammatory and fibrotic blinding disease, is still a therapeutic challenge. Retinal pigment epithelial (RPE) cells dislodged in the vitreous play a central role in the PVR pathogenesis. To identify potential novel contributors to the pathogenesis of PVR, we investigated a profile of vitreous-induced changes in ARPE-19 cells by RNA sequencing. Bioinformatics analysis of the sequencing data showed that there were 258 genes up-regulated and 835 genes down-regulated in the ARPE-19 cells treated with human vitreous. Among these genes, there were three genes related to eye disease with more than threefold changes. In particular, quantitative PCR and western blot results showed that interleukin 13 receptor (IL13R)α2 that is over-expressed in a variety of cancers was up-regulated more than three times in the vitreous-treated ARPE-19 cells. Immunofluorescence analysis indicated that interleukin-13 receptor subunit α2 (IL13Rα2) was highly expressed in ARPE-19 cells within epiretinal membranes from patients with PVR. Importantly, blocking IL13Rα2 with its neutralizing antibody significantly inhibited vitreous-induced contraction of ARPE-19 cells, suggesting a novel role of IL13Rα2 in the PVR pathogenesis. These findings will improve our understanding of the molecular mechanisms by which PVR develops and provides potential targets for PVR therapeutics.
Introduction
Proliferative vitreoretinopathy (PVR) refers to the retinal re-detachment caused by extensive contraction and traction of the proliferative membrane on the surface of the retina and behind the vitreous after reattachment of rhegmatogenous retinal detachment (RRD) (1–4). It is the most common cause of failure to repair RRD (2). The growth and contraction of cell membranes in the vitreous cavity and on both sides of the surface of the retina, and intraretinal fibrosis are characteristics of PVR. PVR occurs in 8–10% of patients undergoing primary retinal detachment correction surgery, and in 40–60% of patients with open global injury (5, 6). At present, repeated surgery is the only option for patients with PVR (3, 7). But recurrent detachment and retinal damage caused by the PVR process itself lead to poor visual effects of the surgery. In the past period of continuous development of vitreous surgery technology, the incidence of PVR in prospective studies has remained unchanged (8, 9).
Fibrotic epiretinal membranes (ERMs) are composed of extracellular matrix such as collagen and fibronectin and cells such as retinal pigment epithelial (RPE) cells, fibroblasts, glial cells, and macrophages (3, 10–12). Almost all risk factors for PVR are related to the diffusion of RPE cells in the vitreous or the destruction of the blood–eye barrier (6). In the process of retinal tear in PVR patients, the detached RPE cells will contact the vitreous, causing the vitreous to stimulate the migration of RPE cells on the surface of the retina. At the same time, the inflammatory mediators and blood released by the retinal tear promote the production of collagen by RPE cells, which further lead to the formation and contraction of ERM (13). Due to the role of RPE cells and glial cells in the vitreous membrane in the pathogenesis of PVR, RPE cells have been widely used in the study of the pathogenesis of PVR (14, 15).
The purpose of this study was to investigate the differentially expressed genes (DEGs) of human RPE cells induced by vitreous and thereby to identify potential therapeutic targets.
Materials and Methods
Major Reagents
Primary antibodies against β-actin (Cat. 4970 1:2,000), pan Keratin (Cat. 4545S, 1:200), and interleukin13 receptor subunit α2 (IL13Rα2) (Cat. 85677S 1:1,000) were bought from the Cell Signaling Technology (Danvers, MA, United States), a neutralizing antibody for IL13Rα2 was purchased from the R&D Systems (Cat. AF146), and a primary antibody against KI67 (Cat. ab243878, 1:200) was purchased from the Abcam (Danvers, MA, United States). Horseradish peroxidase-conjugated goat anti-rabbit IgG (Cat. SA00001-2, 1:5,000) was ordered from the Proteintech (Danvers, MA, United States). Fluorescent labeled secondary antibodies of rabbit (Cat. A21206) or mouse (Cat. WA316324) were bought from the Thermo Fisher Scientific (Waltham, MA, United States).
Patient Vitreous and ARPE-19 Cells Culture
Before launching the project, the ethics approval of the clinical research ethics committee of Jinan University was obtained. A written informed consent was signed by each patient before vitreous samples were harvested from patients with or without PVR (HV).
ARPE-19 purchased from American Type Culture Collection (Manassas, VA, United States), and was cultured in the DMEM/F12 medium with 10% fetal bovine serum (FBS) and 1% penicillin G sodium (100 units/ml) and streptomycin (100 mg/ml) in a humidified incubator at 37°C with 5% CO2 (16).
Porcine Vitreous
Porcine vitreous was taken from fresh porcine eyes, frozen at a –80°C freezer. The eyeballs were dissected on ice and the isolated vitreous was diluted at 1:3 in DMEM/F12 and filtered for sterilization (17).
Cell Proliferation Assay
Cells were cultured a 6-well plate with slides in the DMEM/F12 supplemented with 10% FBS. After the cells were completely attached to the slide, the medium was changed to DMEM/F12 only. Then the cells were treated with vitreous with Ki67 for a proliferation assay. The Ki67 primary antibody was incubated at 4°C overnight, and the fluorescent secondary antibody was incubated for 1 h in the dark at room temperature. Finally, the cells were stained with 4’,6-diamidino-2-phenylindole (DAPI) for 10 min, and the slides were mounted for photographs in a NIKON Ti2-E fluorescence microscope (18, 19).
Cell Migration Assay
Cells were grown to confluence in a 24-well plate before the cell monolayer was scratched with a 200-μl-pipet tip to create a wound. After washing the cells twice with phosphate-buffered saline (PBS), either DMEM/F12, or vitreous (1:3 dilution in DMEM/F12) was added. Photographs were taken to record the width of the wounds at the beginning of the experiment, and then they were taken again 24 h later. After at least three independent experiments were conducted, Adobe Photoshop CC 2018 software was used to analyze the wound healing areas (20, 21).
Contraction Assay
Cells were trypsinized, counted, and centrifuged at 800 rpm for 5 min. The cell pellets were re-suspended in a collagen I solution (Cat. A10483, Gibco, United States) (pH 7.2) on ice, and the collected cells were diluted to 1 × 106 cells/ml in the collagen solution, which were transferred to the wells (300 μl/well) in a 24-well plate that had been incubated with 5 mg/ml bovine serum albumin (BSA)/PBS overnight. The plates were moved into a 37°C incubator for 90 min to allow the collagen to solidify, where upon the collagen gel was overlaid with 0.5 ml of either DMEM/F12, vitreous (1:3 dilution in DMEM/F12), or vitreous plus a neutralizing antibody against IL13Rα2 (1 μg/well). After 48 h, the diameter of the gel was measured and calculated. The data of three independent experiments were used for statistical analysis (21–23). Neutralizing the IL13RA2 receptor using neutralizing antibodies in collagen gel contraction is the same as the contraction assay.
RNA Sequencing
Serum starved ARPE-19 cells at 70–80% confluence were treated with human vitreous diluted at a ratio of 1:3 in the DMEM/F12 medium for 24 h. The treated cells were then harvested by Trizol for RNA isolation using an OMEGA kit (R6834). Subsequently, a Complementary DNA (cDNA) library was established and the quality and integrity of the RNA were examined by a NanoDrop analysis. RNA sequencing was performed by NovaSeq 6000. The differential genes were screened to satisfy | log2FC| ≥ 1 and p < 0.05, and genes were further screened to identify significantly differentially expressed genes related to eye diseases (24, 25).
Notably, Gene Ontology (GO) and Kyoto Encyclopedia of Genes and Genomes (KEGG) are two databases, and enrichment analysis is an integrated calculation of the functional information in the database. The full name of GO database analysis is Gene Ontology, and it divides the function of genes into three parts: cellular component (CC), molecular function (MF), and biological process (BP). Using the GO database, we can get the disease correlation of the target gene at the three levels of CC, MF, and BP. KEGG database analysis is a kind of pathway-related database to study the various pathways of the human body that genes participate in (24, 25).
Quantitative PCR (qPCR) Analysis
Total RNA in the RPE cells treated by vitreous was extracted using an OMEGA kit (R6834), and then reverse transcribed to construct a cDNA library using a kit (RR036A, Takara). The differential expression of eye disease-related genes was analyzed by qPCR using a kit (RR820A, Takara). Primer sequences: IL13RA2 forward (F): 5’-GGGCATTGAAGCGAAGATACA-3’; IL13RA2 reverse (R): 5′-GCCCAGGAACTTTGAACTTCTG-3′ (26); APCDD1 forward (F): 5′-TCCTGCTCAGATACCTGTTCC-3′; APCDD1 reverse (R): 5′- GTGATGGCACTGTGACTCCT-3′; CD180 forward (F): 5′-AACCTAAGCCTGAACTTCAATGG-3′; CD180 reverse (R): 5′-GCCAGAGAGACTGAGTAGTAGAG-3′.
Western Blot
When cells reached 70–80% confluence, they were serum-starved for 24 h, and then treated with or without vitreous from patients (diluted 1:3 in DMEM/F12) for 24 h. After two washes with ice-cold PBS, cells were lysed in a cell lysis buffer for 30 min. The cell lysates were then clarified by centrifugation at 13,000 rpm for 10 min at 4°C. The total proteins were quantitated using a BCA kit (Cat. KGP250, keyGen, China), and the samples were boiled for 5 min. Proteins were separated by 10% sodium dodecyl-sulfate-polyacrylamide gel electrophoresis (SDS-PAGE), transferred to polyvinylidene difluoride (PVDF) membranes, and then subjected to western blot analysis using desired antibodies. Signal intensity was determined by densitometry using the Image J Software (22).
Immunofluorescence
This experiment was performed as described previously (27, 28). Briefly, the tissues on slides were fixed with 4% paraformaldehyde (PFA) for 10 min at room temperature, blocked with 3% FBS for 30 min at room temperature, and then incubated with primary antibodies (anti-IL13Rα2 antibody or non-immune IgG at 1:200 dilution) at 4°C overnight. After thorough washes, the secondary antibody at a ratio of 1:1,000 was incubated at room temperature for 1 h in the dark. Next, the DAPI staining at a ratio of 1:1,000 was performed in the dark for additional 10 min. Finally, after the slides were washed thoroughly, they were mounted for photograph in a NIKON Ti2-E fluorescence microscope (27, 28).
Statistics
Data from three independent experiments were used for statistical analysis with ordinary one-way ANOVA. The p value less than 0.05 was considered to be a significant difference (29).
Results
RNA Sequencing Analysis Reveals That There Are 258 Genes Up-Regulated and 835 Genes Down-Regulated in Vitreous-Treated Retinal Pigment Epithelial Cells
Treatment of RPE cells with vitreous in vitro was to mimic PVR pathogenesis in vivo, and vitreous from human, bovine, and experimental rabbits is able to enhance cell proliferation, survival, and contraction (23, 30, 31). We then tested if vitreous from porcine could also have a similar bioactivity. Results showed that porcine vitreous was also capable of enhancing cell proliferation as shown in Supplementary Figures 1–3. These data are consistent with our previous findings that vitreous enhances PVR-related cellular events (13).
To discover novel genes contributing to PVR pathogenesis, we next treated ARPE-19 cells with human vitreous for transcriptional profiling. The quality of the RNA extracted from ARPE-19 cells treated with human vitreous reached the requirements for RNA sequencing (data not shown). Significant DEGs between the control and the vitreous-treated groups were identified based on the criteria of a log2-fold change of >2. The results showed that in the RPE cells treated with human vitreous there were 1,093 differential genes, of which there were 258 genes up-regulated (23.6%) and 835 genes were down-regulated (76.4%). Among them, there are three genes related to eye diseases (Figure 1). Cluster analysis was performed on 46 of the differentially expressed genes in the genomics data. In the cluster heatmap, the red and blue regions represent the up-regulated and down-regulated expression of genes, respectively (Figure 2); KEGG enrichment analysis revealed that nearly half of the differential genes were mainly concentrated in pathways related to cellular components such as the mitogen-activated protein kinase (MAPK) pathway (Figure 3).
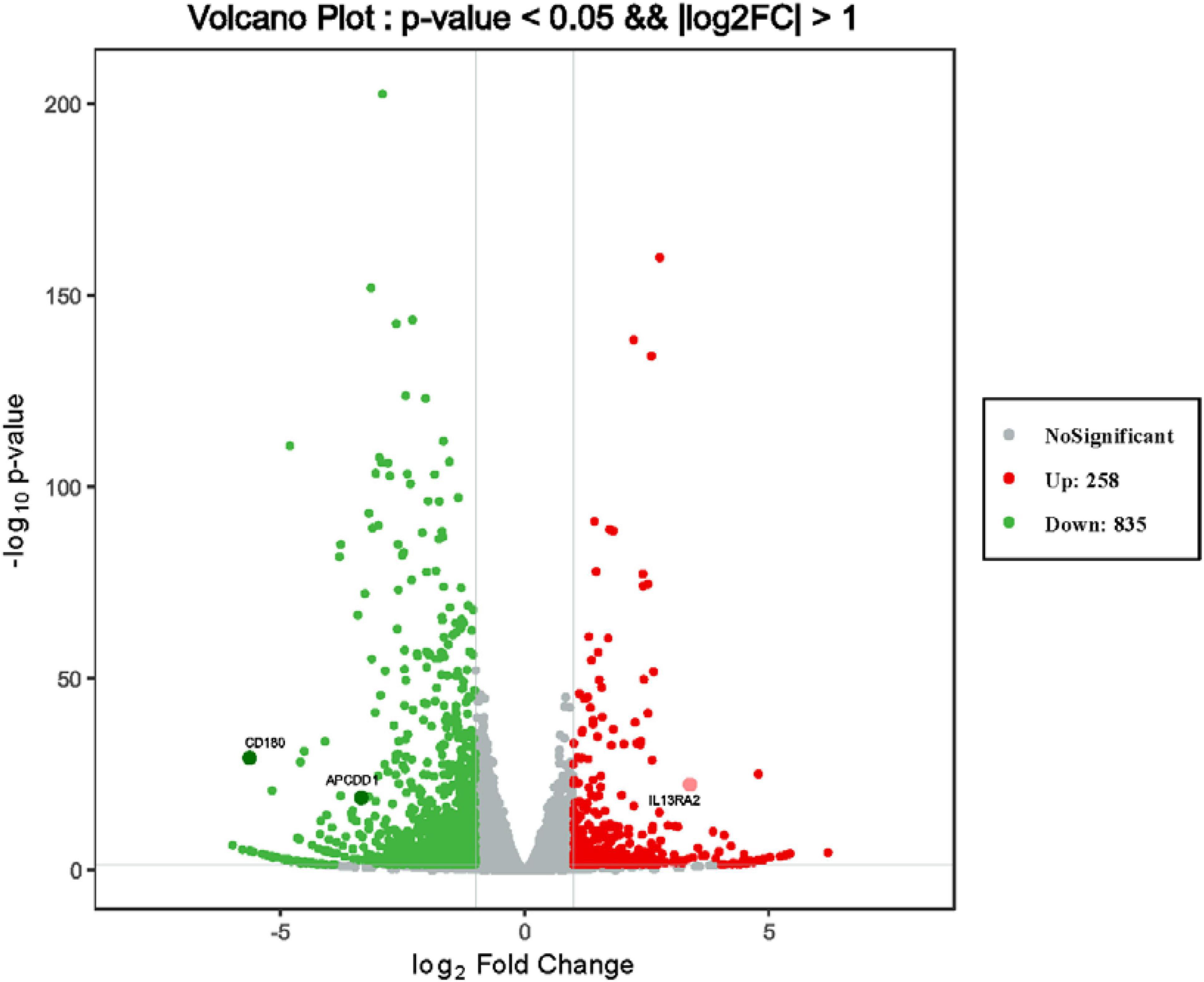
Figure 1. Differentially expressed genes (DEGs) of retinal pigment epithelial (RPE) cells induced by the control group and vitreous. Results of a volcano plot chart of the differentially expressed genes were identified from the control and vitreous-treated group.
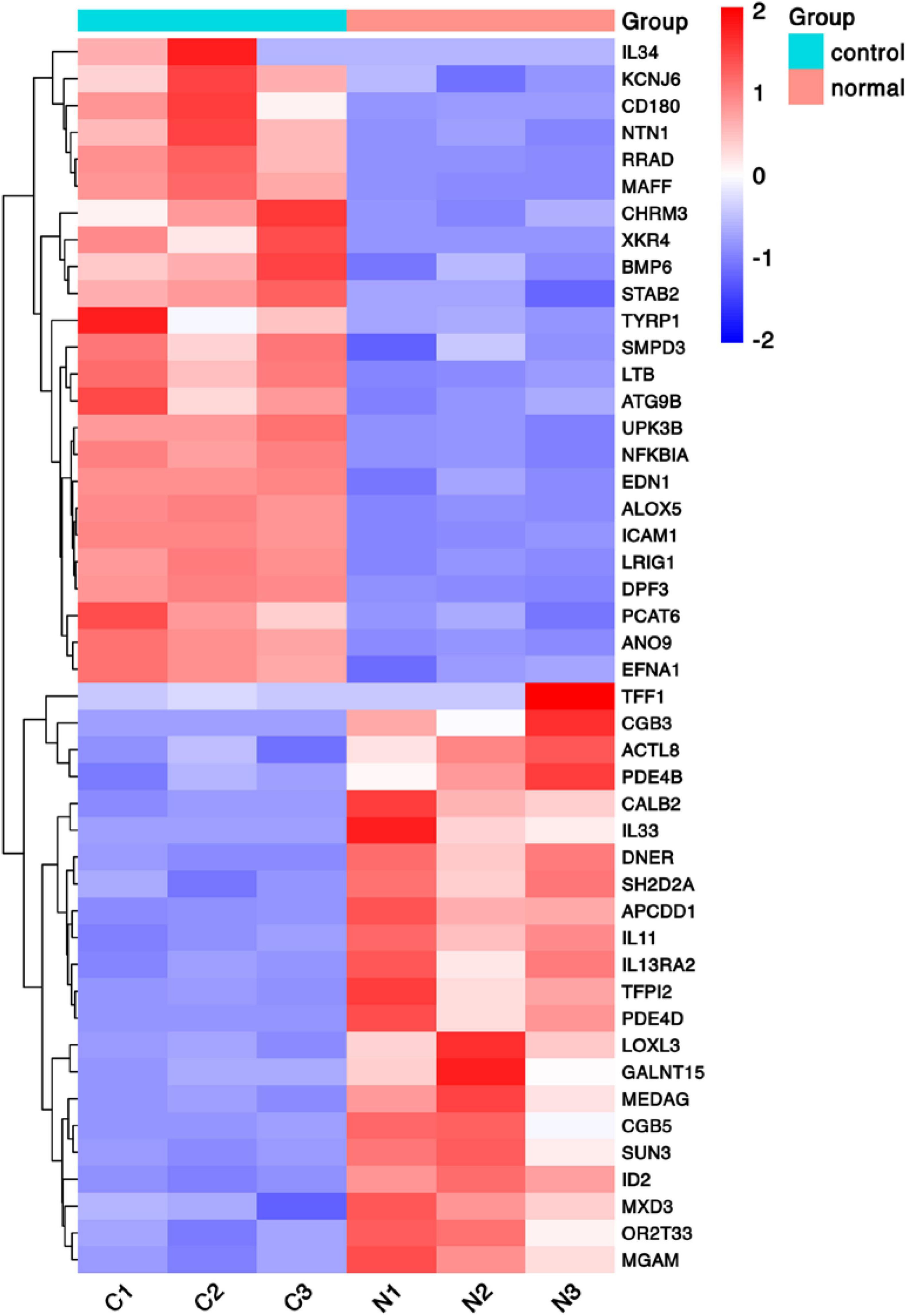
Figure 2. Heat map of hierarchical clustered genes. A heat map of hierarchical clustered genes. In total, there were 46 genes from the genomics data showing a significantly aberrant expression (at least two-fold change), p < 0.05. In the clustering analysis, red and blue regions indicate the up-regulated and down-regulated genes, respectively. C1, C2, C3, and N1, N2, N3 represent the three independent repetitions of the control group and the vitreous induction group, respectively.
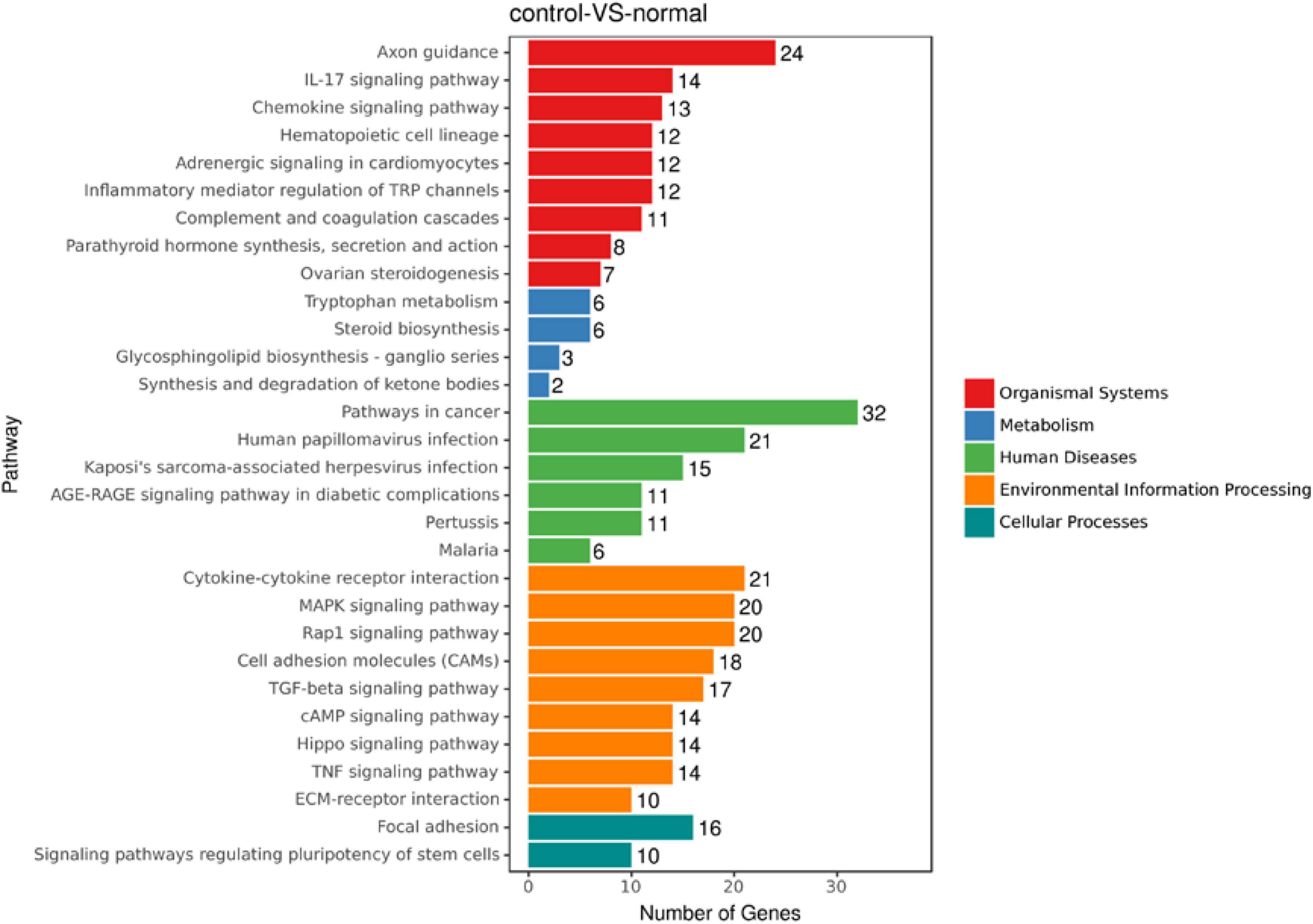
Figure 3. An enrich mean analysis of pathways for DEGs based on the KEGG database. An enrich mean analysis of pathways for DEGs based on the KEGG database. The horizontal and vertical axes represent the enrichment score of – log p and the pathway category, respectively.
Vitreous Induces Changes in IL13RA2 Expression of mRNA and Protein
Among the 1,093 differential genes induced by vitreous, there were three genes with more than threefold changes (Table 1), which were confirmed by qPCR analysis (Figure 4). Noticeably, PVR is an inflammatory eye disease (12), IL13 is present in the vitreous (32); in addition, IL13Rα2 can bind to IL13 with high affinity to enhance cell proliferation and migration in the carcinogenesis (33, 34), and these cellular events are related to PVR pathogenesis. Thereby, we next investigated whether a vitreous-induced change in IL13RA2 revealed by RNA sequencing was indeed the case. To this end, we treated ARPE-19 cells again with human vitreous and analyzed them with qPCR. The results showed that the vitreous treatments dramatically heightened the IL13RA2 expression in the RPE cells as shown in Figure 4.
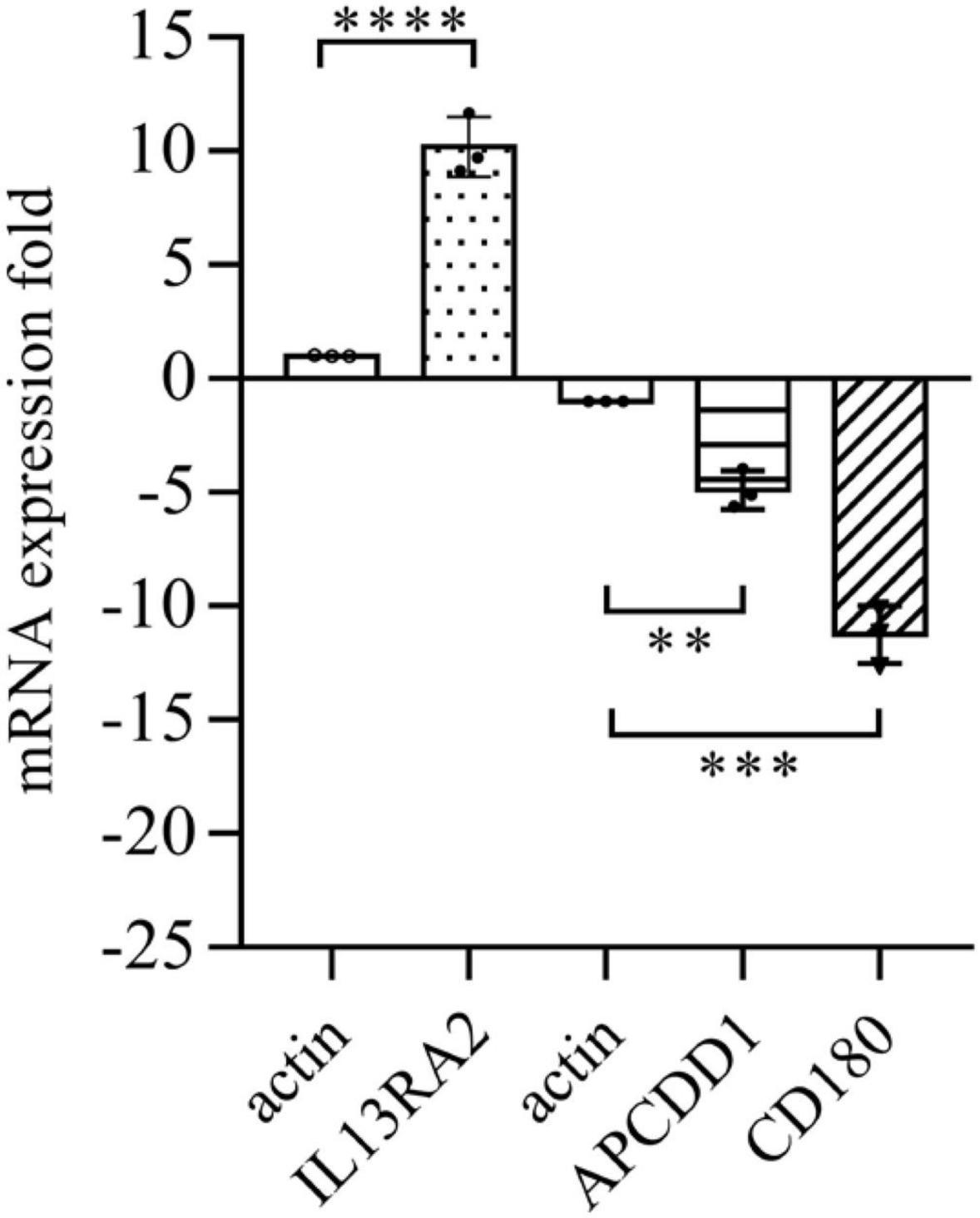
Figure 4. Vitreous induced changes in the mRNA expression. The qPCR analysis of mRNA expression in ARPE-19 cells induced by vitreous. The mean ± SD of three independent experiments is shown; **** denotes 0.0001, using paired t test; *** denotes 0.001, using paired t test; ** denotes 0.01, using paired t test. Positive values indicate a fold increase in expression of the target gene relative to the internal reference, and negative values are opposite.
Since vitreous induced changes in mRNA expression of IL13RA2, we next examined if its protein levels were also changed with the vitreous stimulation. As expected, Western blot analysis showed that vitreous augmented IL13Rα2 expression in the ARPE-19 cells (Figure 5). This Western blotting data are consistent with those obtained from RNA sequencing and qPCR.
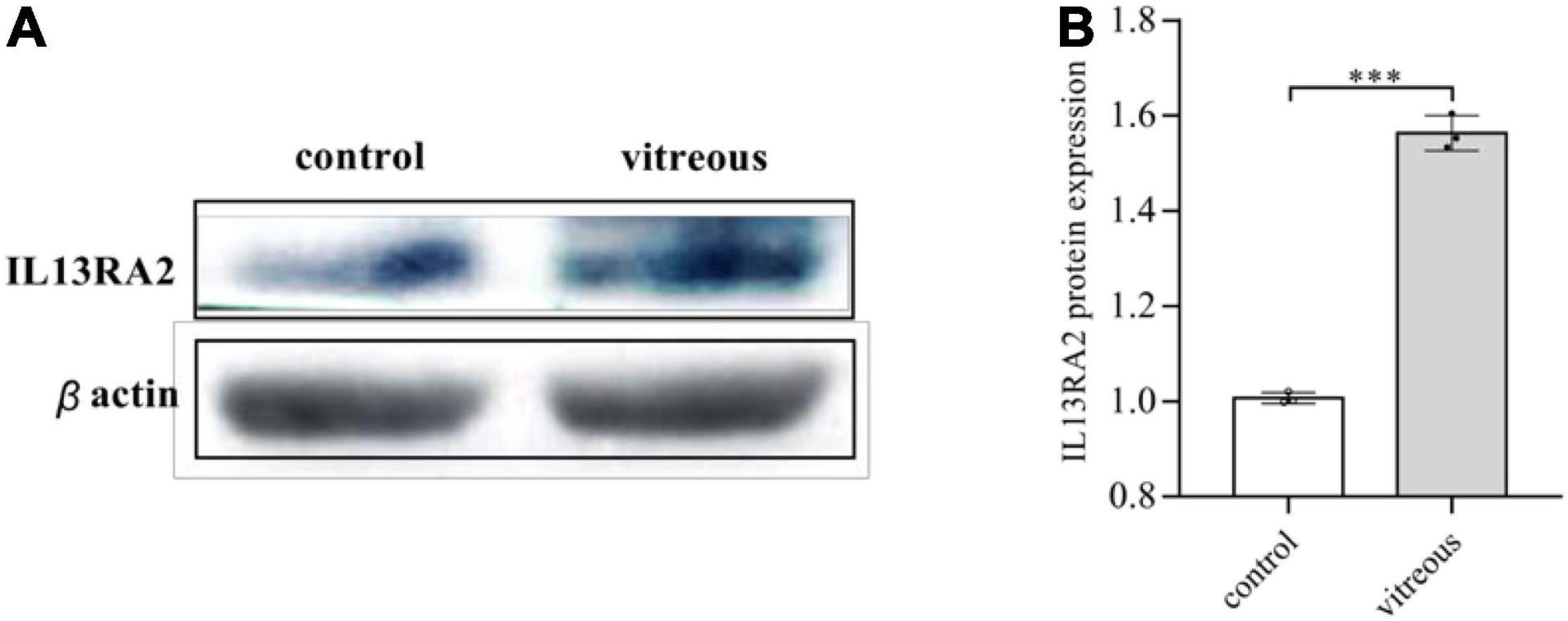
Figure 5. Vitreous induced the protein expression of IL13Rα2. Western blot analysis of the vitreous-induced protein expression of IL13RA2 in ARPE-19 cells. One of three representative experiments is shown. Bar graphs showed the Western blot band intensity, and the mean ± SD of three independent experiments is shown; *** denotes 0.001, using paired t test. (A) The protein expression of IL13RA2 in ARPE-19 cells. (B) Histogram of protein expression gray value. The mean ± SD of three independent experiments is shown; *** denotes 0.001, using paired t test. Control: ARPE-19 cells treated with DMEM/F12 only. Vitreous: ARPE-19 cells treated with vitreous diluted in DMEM/F12.
IL13Rα2 Is Highly Expressed in Epiretinal Membranes From Patients With Proliferative Vitreoretinopathy
We next investigated whether IL13Rα2 was expressed in RPE cells within the epiretinal membranes from PVR patients. To this end, we stained epiretinal membranes with antibodies against keratin specific for epithelial cells and IL13Rα2. The immunofluorescence results showed that IL13Rα2 was highly expressed in RPE cells in epiretinal membranes from PVR patients (Figure 6).
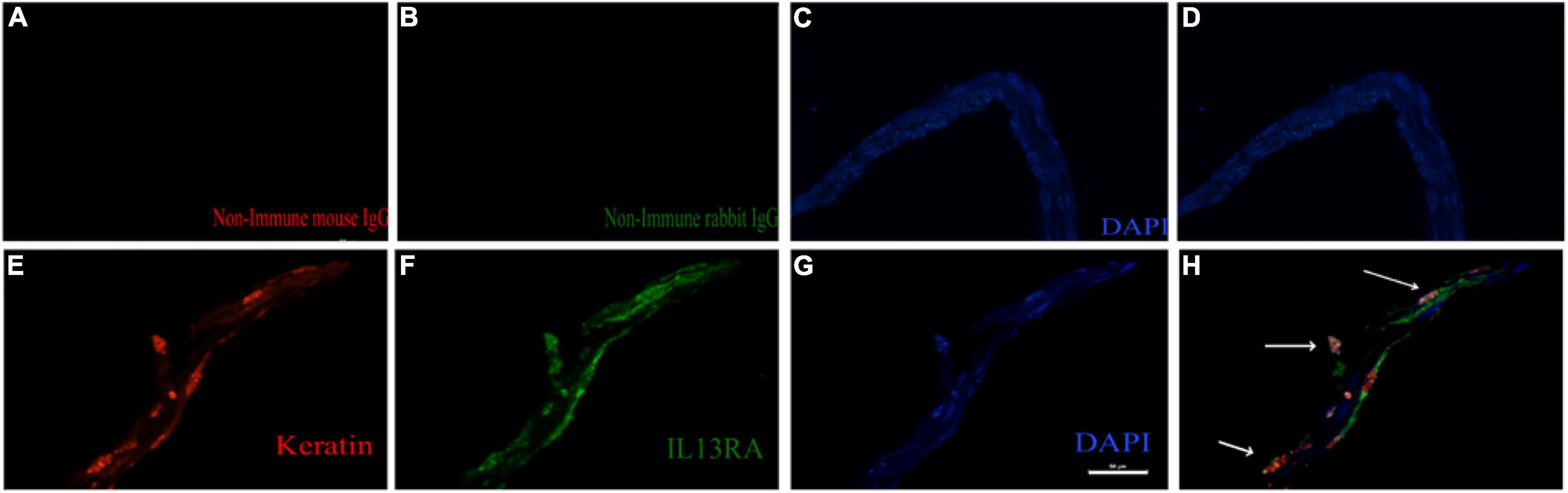
Figure 6. IL13Rα2 is highly expressed in RPE cells within epiretinal membranes from patients with proliferative vitreoretinopathy (PVR). Fibrotic epiretinal membranes from patients with PVR were first incubated with primary antibodies: a mixture of non-immune mouse and rabbit IgGs (A–D), or of anti-IL13Rα2 and pan keratin antibodies (E–H) at 4°C overnight, and then with fluorescently labeled secondary antibodies at 1 h in room temperature. Co-staining of IL13Rα2 with pan keratin in H indicates IL13Rα2 expression in RPE cells in the ERMs from patients with PVR. Scale bar: 50 μm.
Neutralization of IL13Rα2 Prevents Vitreous-Induced Contraction of Retinal Pigment Epithelial Cells
One of important events in the PVR pathogenesis is the contraction of epiretinal membranes, leading to the retinal detachment. Thereby we assessed if neutralization of IL13Rα2 could prevent vitreous-induced contraction of ARPE-19 cells in a collagen gel contraction assay, one of cellular models for PVR. As expected, vitreous stimulated the contraction of the collagen gel, and the antibody neutralizing IL13Rα2 significantly prevented the contraction induced by the vitreous (Figure 7), suggesting that this antibody is promising for PVR therapeutics.
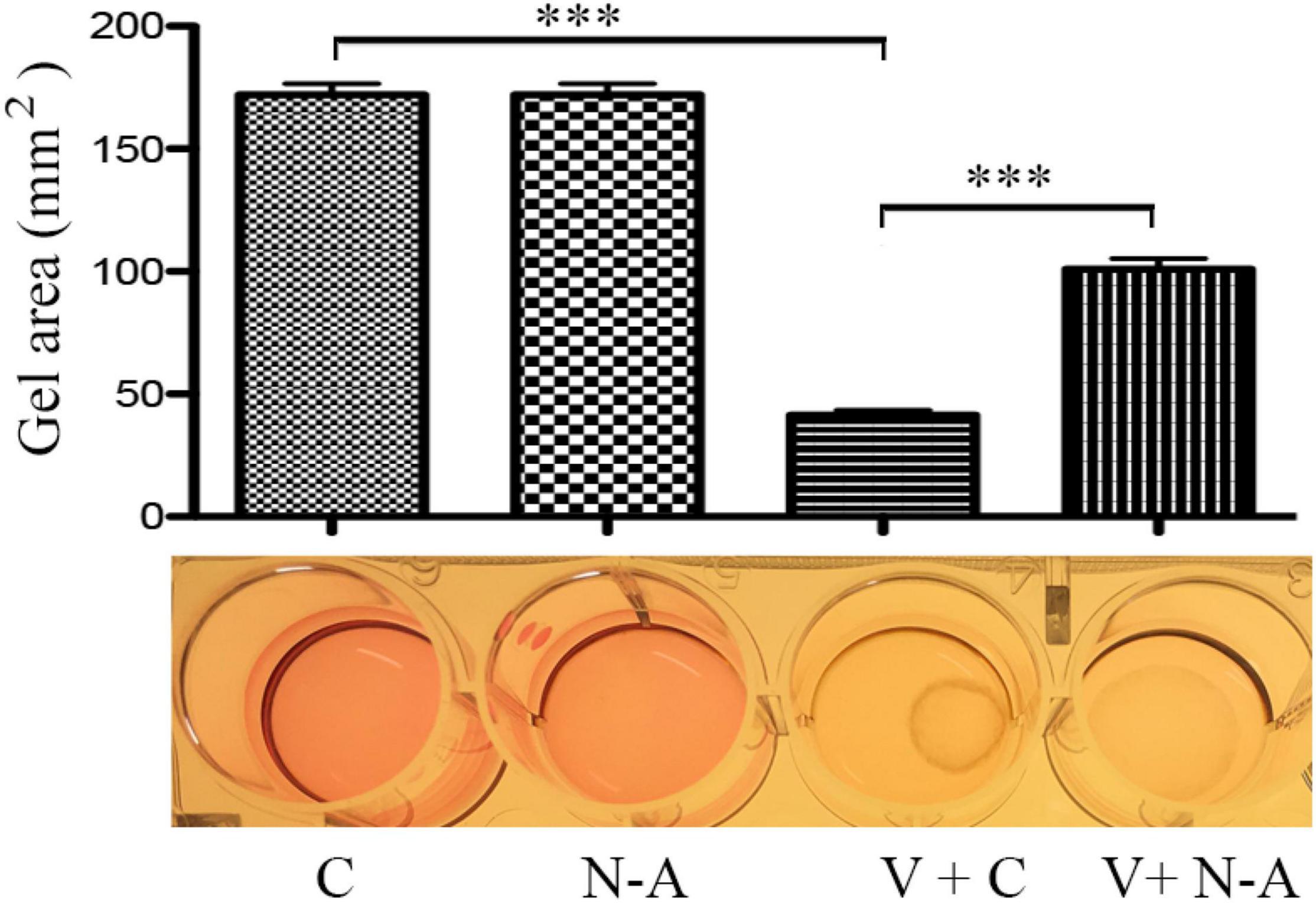
Figure 7. Blocking IL13Rα2 prevents vitreous-induced contraction of RPE cells. After the mixtures of ARPE-19 cells with collagen I solution formed a collagen gel, they were treated with DMEM/F12 + an antibody or vitreous + an antibody for 48 h. C, control antibody; N-A: neutralizing antibody against IL13Rα2; V + C, vitreous + control antibody; V + N-A, vitreous + neutralizing antibody against IL13Rα2. *** denotes 0.001, using paired t test.
Discussion
In this article, we report that vitreous induced a large increase in IL13Rα2 in ARPE-19 cells and blocking IL13Rα2 with its neutralizing antibody prevents contraction of ARPE-19 cells, implicating a novel role of IL13Rα2 in the PVR pathogenesis. The clinical manifestations of PVR are related to a series of inflammatory and fibrotic changes (12, 35–37). When the retina of a patient ruptures, RPE cells exposed in the vitreous cavity respond to the vitreal growth factors and cytokines, leading to forward feedback to secrete these factors more (38), mediating wound repair responses such as matrix synthesis, cell migration, proliferation, and epithelial–mesenchymal transition (EMT), leading to the formation of epiretinal membranes, an essential process of PVR (39).
So far, the pathogenesis of PVR has not been completely understood. Hypotheses for PVR formation have been proposed for the essential roles of multiple growth factors and cytokines, such as transforming growth factor beta (TGF-β), platelet-derived growth factor (PDGF), vascular endothelial growth factors (VEGF), interleukins (ILs), tumor necrosis factor alpha (TNF-a) (12, 38, 40). ILs can be secreted by a variety of cells in response to a variety of stimulation including tissue damage. In recent years, studies have revealed that ILs are closely related to the occurrence and development of PVR. For instance, IL-6, a marker of acute inflammation (41), is up-regulated in the vitreous and subretinal fluid of the PVR group, and is positively correlated with the degree and duration of RRD and PVR grades (42, 43). IL-8, playing a key role in regulating inflammation and mediating angiogenesis, is elevated in PVR patients (44).
We herein report that IL13RA2 is heightened in the vitreous-treated RPE cells. IL13RA2, one of the high-affinity membrane receptors of IL-13, is highly expressed in tumors, such as liver cancer (45), glioblastoma (46), colon cancer (47), and pancreatic cancer (48). IL13RA2 has two forms: transmembrane form and extracellular soluble form (49–51). The transmembrane form is related to the signal transduction of ligands and the binding of other membrane receptors to form different functional subunits; soluble IL13RA2 seems to inhibit the function of IL13 (51, 52). It is speculated that the functions of the two forms of IL13RA2 may be antagonistic to each other. The previous studies on tumors reported that the overexpression of IL13RA2 endows tumors with the ability to invade and metastasize. IL-13 plays a key role in many pathological processes, such as asthma, pulmonary fibrosis, and ulcerative colitis (53, 54), and IL13 as an inflammatory factor plays an important part in various inflammatory reactions, suggesting IL13RA2 may play an important role in PVR.
Noticeably, IL13Rα2 cooperates with epithermal growth factor receptor (EGFR) VIII signaling to promote glioblastoma multiforme (46), whereas PDGF receptor (PDGFR)β plays an essential role in vitreous-induced cellular responses related to PVR (13). Thereby we hypothesize that in ARPE-19 cells, IL13Rα2 interacts with PDGFRβ signaling to boost vitreous-stimulated cellular events intrinsic to PVR as illustrated in Figure 8, and this hypothesis is being tested in our research group.
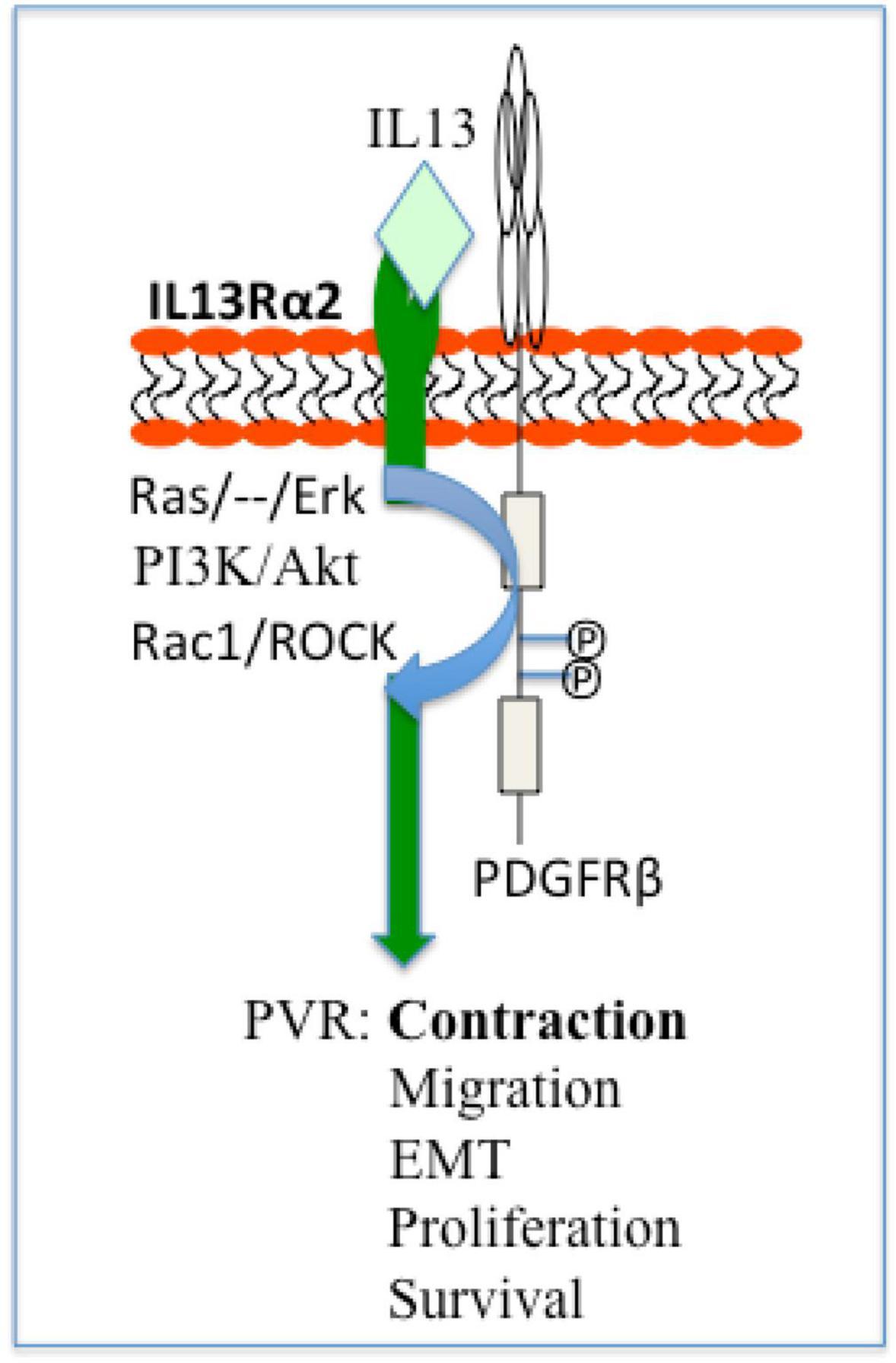
Figure 8. Schematic of a hypothesis: a role of IL13Rα2 cooperates with PDGFRβ in the PVR pathogenesis. Based on current literatures (13, 55) IL13Rα2 cooperates with PDGFRβ to initiate signaling pathways of Ras/Raf/MEK/ERK, PI3K/Akt, Rac1/ROCK, and thereby enhance cellular responses (survival, proliferation, EMT, migration, and contraction) intrinsic to PVR. ERK, extracellular signal-regulated kinases; PI3K, phosphoinositide 3 kinase; Rac1, Ras-related C3 botulinum toxin substrate 1; ROCK, Rho-associated protein kinase; EMT, epithelial–mesenchymal transition; PVR, proliferative vitreoretinopathy.
In this study, we employed RNA sequencing to discover changes in vitreous-treated ARPE-19 cells, leading to our findings that IL13Rα2 was up-regulated significantly, and blockade of IL13Rα2 prevented contraction of ARPE-19 cells, suggesting that IL13Rα2 be a novel therapeutic target for PVR.
Data Availability Statement
HL is the guarantor of this work, has full access to all the data in the study, and takes responsibility for the integrity of the data and the accuracy of the data analysis. The datasets generated during and/or analyzed during the current study are available from the corresponding author upon reasonable request.
Ethics Statement
The studies involving human participants were reviewed and approved by the Jinan University Research Ethics Board. The patients/participants provided their written informed consent to participate in this study.
Author Contributions
HQ, LD, and DF performed most of the experiments and analyzed the results. YW, NF, XM, GZ, and WW performed some experiments and managed projects. SZ and HL conceived the experiments, analyzed the data, and wrote the manuscript. All authors contributed to the article and approved the submitted version.
Funding
This work was supported by the National Natural Science Foundation of China (82070989) to HL, and Sanming Project of Medicine in Shenzhen (No. SZSM202011015) to XY, GZ, SZ, and HL. No funding bodies had any role in study design, data collection and analysis, and decision to publish, or preparation of the manuscript.
Conflict of Interest
The authors declare that the research was conducted in the absence of any commercial or financial relationships that could be construed as a potential conflict of interest.
Publisher’s Note
All claims expressed in this article are solely those of the authors and do not necessarily represent those of their affiliated organizations, or those of the publisher, the editors and the reviewers. Any product that may be evaluated in this article, or claim that may be made by its manufacturer, is not guaranteed or endorsed by the publisher.
Supplementary Material
The Supplementary Material for this article can be found online at: https://www.frontiersin.org/articles/10.3389/fmed.2022.831436/full#supplementary-material
Abbreviations
ARPE-19, a human retinal pigment epithelial cell line; CLL, chronic lymphocytic leukemia; DEG, differentially expressed genes; EGFR, epidermal growth factor receptor; EMT, epithelial–mesenchymal transition; ERM, epiretinal membranes; HPRRE, human primary RPE; IL13RA2, Interleukin 13 receptor alpha-2; MAPK, mitogen-activated protein kinase; PDGFR, platelet-derived growth factor receptor; PVR, proliferative vitreoretinopathy; RPE, retinal pigment epithelial cells; TGF- β, transforming growth factor beta; TNF- α, tumor necrosis factor alpha; VEGF, vascular endothelial growth factor.
References
1. Das V, Bhattacharya S, Chikkaputtaiah C, Hazra S, Pal M. The basics of epithelial-mesenchymal transition (EMT): a study from a structure, dynamics, and functional perspective. J Cell Physiol. (2019) 234: 14535–55. doi: 10.1002/jcp.28160
2. Lin YC, Shen ZR, Song XH, Liu X, Yao K. Comparative transcriptomic analysis reveals adriamycin-induced apoptosis via p53 signaling pathway in retinal pigment epithelial cells. J Zhejiang Univ Sci B. (2018) 19:895–909. doi: 10.1631/jzus.B1800408
3. Asato R, Yoshida S, Ogura A, Nakama T, Ishikawa K, Nakao S, et al. Comparison of gene expression profile of epiretinal membranes obtained from eyes with proliferative vitreoretinopathy to that of secondary epiretinal membranes. PLoS One. (2013) 8:e54191. doi: 10.1371/journal.pone.0054191
4. Roybal CN, Velez G, Toral MA, Tsang SH, Bassuk AG, Mahajan VB. Personalized proteomics in proliferative vitreoretinopathy implicate hematopoietic cell recruitment and mTOR as a therapeutic target. Am J Ophthalmol. (2018) 186:152–63. doi: 10.1016/j.ajo.2017.11.025
5. Andre F, Ciruelos E, Rubovszky G, Campone M, Loibl S, Rugo HS, et al. Alpelisib for PIK3CA-mutated, hormone receptor-positive advanced breast cancer. N Engl J Med. (2019) 380:1929–40.
6. Pastor JC, Rojas J, Pastor-Idoate S, Lauro S. Di, Gonzalez-Buendia L, Delgado-Tirado S. Proliferative vitreoretinopathy: a new concept of disease pathogenesis and practical consequences. Prog Retin Eye Res. (2016) 51:125–55. doi: 10.1016/j.preteyeres.2015.07.005
7. Schiff L, Boles C, Fernandes M, Nachmani B, Gentile R, Blenkinsop TA. P38 inhibition reverses TGFβ1 and TNFα-induced contraction in a model of proliferative vitreoretinopathy. Commun. Biol. (2019) 2:162.
8. Zhou RM, Wang XQ, Yao J, Shen Y, Chen SN, Yang H, et al. Identification and characterization of proliferative retinopathy-related long noncoding RNAs. Biochem Biophys Res Commun. (2015) 465:324–30. doi: 10.1016/j.bbrc.2015.07.120
9. Rojas JI, Fernandez JC, Pastor R, MacLaren E, Ramkissoon Y, Harsum S, et al. Predicting proliferative vitreoretinopathy: temporal and external validation of models based on genetic and clinical variables. Br J Ophthalmol. (2015) 99:41–8. doi: 10.1136/bjophthalmol-2014-305263
10. Bastiaans J, van Meurs JC, Mulder VC, Nagtzaam NM, Smits-te Nijenhuis M, Dufour-van den Goorbergh DC, et al. The role of thrombin in proliferative vitreoretinopathy. Invest Ophthalmol Vis Sci. (2014) 55:4659–66. doi: 10.1167/iovs.14-14818
11. Garweg JG, Tappeiner C, Halberstadt M. Pathophysiology of proliferative vitreoretinopathy in retinal detachment. Surv Ophthalmol. (2013) 58:321–9. doi: 10.1016/j.survophthal.2012.12.004
12. Moysidis SN, Thanos A, Vavvas DG. Mechanisms of inflammation in proliferative vitreoretinopathy: from bench to bedside. Mediat Inflamm. (2012) 2012:1–11. doi: 10.1155/2012/815937
13. Yang Y, Huang X, Ma G, Cui J, Matsubara JA, Kazlauskas A, et al. PDGFRbeta plays an essential role in patient vitreous-stimulated contraction of retinal pigment epithelial cells from epiretinal membranes. Exp Eye Res. (2020) 197:108116. doi: 10.1016/j.exer.2020.108116
14. Oberstein SY, Byun J, Herrera D, Chapin EA, Fisher SK, Lewis GP. Cell proliferation in human epiretinal membranes: characterization of cell types and correlation with disease condition and duration. Mol Vis. (2011) 17:1794–805.
15. Umazume K, Tsukahara R, Liu L, Fernandez de Castro JP, McDonald K, Kaplan HJ, et al. Role of retinal pigment epithelial cell beta-catenin signaling in experimental proliferative vitreoretinopathy. Am J Pathol. (2014) 184:1419–28. doi: 10.1016/j.ajpath.2014.01.022
16. Sant DW, Camarena V, Mustafi S, Li Y, Wilkes Z, Van Booven D, et al. Ascorbate Suppresses VEGF expression in retinal pigment epithelial cells. Invest Ophthalmol Vis Sci. (2018) 59:3608–18. doi: 10.1167/iovs.18-24101
17. Rimpela AK, Reunanen S, Hagstrom M, Kidron H, Urtti A. Binding of small molecule drugs to porcine vitreous humor. Mol Pharm. (2018) 15:2174–9. doi: 10.1021/acs.molpharmaceut.8b00038
18. Graefe C, Eichhorn L, Wurst P, Kleiner J, Heine A, Panetas I, et al. Optimized Ki-67 staining in murine cells: a tool to determine cell proliferation. Mol Biol Rep. (2019) 46:4631–43. doi: 10.1007/s11033-019-04851-2
19. Meng S, Zhu Y, Li J-F, Wang X. Apigenin inhibits renal cell carcinoma cell proliferation. Oncotarget. (2017) 8:19834–42.
20. Huang X, Zhou G, Wu W, Ma G, D’Amore PA, Mukai S, et al. Editing VEGFR2 Blocks VEGF-Induced Activation of Akt and Tube Formation. Invest Ophthalmol Vis Sci. (2017) 58:1228–36. doi: 10.1167/iovs.16-20537
21. Chen N, Hu Z, Yang Y, Han H, Lei H. Inactive Cas9 blocks vitreous-induced expression of Mdm2 and proliferation and survival of retinal pigment epithelial cells. Exp Eye Res. (2019) 186:107716. doi: 10.1016/j.exer.2019.107716
22. Lei H, Velez G, Hovland P, Hirose T, Gilbertson D, Kazlauskas A. Growth factors outside the PDGF family drive experimental PVR. Invest Ophthalmol Vis Sci. (2009) 50:3394–403. doi: 10.1167/iovs.08-3042
23. Lei H, Velez G, Cui J, Samad A, Maberley D, Matsubara J, et al. N-acetylcysteine suppresses retinal detachment in an experimental model of proliferative vitreoretinopathy. Am J Pathol. (2010) 177:132–40. doi: 10.2353/ajpath.2010.090604
25. Schumacker ST, Coppage KR, Enke RA. RNA sequencing analysis of the human retina and associated ocular tissues. Sci Data. (2020) 7:199. doi: 10.1038/s41597-020-0541-4
26. Wagner EM. Monitoring gene expression: quantitative real-time rt-PCR. Methods Mol Biol. (2013) 1027:19–45. doi: 10.1007/978-1-60327-369-5_2
27. Ding X, Bai Y, Zhu X, Li T, Jin E, Huang L, et al. The effects of pleiotrophin in proliferative vitreoretinopathy. Graefes Arch Clin Exp Ophthalmol. (2017) 255:873–84. doi: 10.1007/s00417-016-3582-9
28. Diercks GF, Pas HH, Jonkman MF. Immunofluorescence of autoimmune bullous diseases. Surg Pathol Clin. (2017) 10:505–12.
29. Huang X, Zhou G, Wu W, Duan Y, Ma G, Song J, et al. Genome editing abrogates angiogenesis in vivo. Nat Commun. (2017) 8:112. doi: 10.1038/s41467-017-00140-3
30. Lei H, Velez G, Kazlauskas A. Pathological signaling via platelet-derived growth factor receptor {alpha} involves chronic activation of Akt and suppression of p53. Mol Cell Biol. (2011) 31:1788–99.
31. Han H, Yang Y, Liu B, Tian J, Dong L, Qi H, et al. Chalcomoracin prevents vitreous-induced activation of AKT and migration of retinal pigment epithelial cells. J Cell Mol Med. (2021) 25:9102–11. doi: 10.1111/jcmm.16590
32. Yoshida S, Kobayashi Y, Nakama T, Zhou Y, Ishikawa K, Arita R, et al. Increased expression of M-CSF and IL-13 in vitreous of patients with proliferative diabetic retinopathy: implications for M2 macrophage-involving fibrovascular membrane formation. Br J Ophthalmol. (2015) 99:629–34. doi: 10.1136/bjophthalmol-2014-305860
33. Fujisawa T, Joshi B, Nakajima A, Puri RK. A novel role of interleukin-13 receptor alpha2 in pancreatic cancer invasion and metastasis. Cancer Res. (2009) 69:8678–85. doi: 10.1158/0008-5472.CAN-09-2100
34. Marquez-Ortiz RA, Contreras-Zarate MJ, Tesic V, Alvarez-Eraso KLF, Kwak G, Littrell Z, et al. IL13Ralpha2 promotes proliferation and outgrowth of breast cancer brain metastases. Clin Cancer Res. (2021) 27:6209–21. doi: 10.1158/1078-0432.CCR-21-0361
35. Chaudhary R, Scott RAH, Wallace G, Berry M, Logan A, Blanch RJ. Inflammatory and fibrogenic factors in proliferative vitreoretinopathy development. Transl Vis Sci Technol. (2020) 9:23. doi: 10.1167/tvst.9.3.23
36. Ni Y, Qin Y, Huang Z, Liu F, Zhang S, Zhang Z. Distinct serum and vitreous inflammation-related factor profiles in patients with proliferative vitreoretinopathy. Adv Ther. (2020) 37:2550–9. doi: 10.1007/s12325-020-01325-x
37. Dai Y, Dai C, Sun T. Inflammatory mediators of proliferative vitreoretinopathy: hypothesis and review. Int Ophthalmol. (2020) 40:1587–601. doi: 10.1007/s10792-020-01325-4
38. Pennock S, Haddock LJ, Eliott D, Mukai S, Kazlauskas A. Is neutralizing vitreal growth factors a viable strategy to prevent proliferative vitreoretinopathy? Prog Retin Eye Res. (2014) 40:16–34. doi: 10.1016/j.preteyeres.2013.12.006
39. Rouberol F, Chiquet C. [Proliferative vitreoretinopathy: pathophysiology and clinical diagnosis]. J Fr Ophtalmol. (2014) 37:557–65. doi: 10.1016/j.jfo.2014.04.001
40. Morescalchi F, Duse S, Gambicorti E, Romano MR, Costagliola C, Semeraro F. Proliferative vitreoretinopathy after eye injuries: an overexpression of growth factors and cytokines leading to a retinal keloid. Mediat Inflamm. (2013) 2013:269787. doi: 10.1155/2013/269787
41. Ricker LJ, Kijlstra A, Kessels AG, de Jager W, Liem AT, Hendrikse F, et al. Interleukin and growth factor levels in subretinal fluid in rhegmatogenous retinal detachment: a case-control study. PLoS One. (2011) 6:e19141. doi: 10.1371/journal.pone.0019141
42. Symeonidis C, Papakonstantinou E, Androudi S, Georgalas I, Rotsos T, Karakiulakis G, et al. Comparison of interleukin-6 and matrix metalloproteinase expression in the subretinal fluid and the vitreous during proliferative vitreoretinopathy: Correlations with extent, duration of RRD and PVR grade. Cytokine. (2014) 67:71–6. doi: 10.1016/j.cyto.2014.02.012
43. Kon CH, Occleston NL, Aylward GW, Khaw PT. Expression of vitreous cytokines in proliferative vitreoretinopathy: a prospective study. Invest Ophthalmol Vis. (1999) 40:705–12.
44. Rasier R, Gormus U, Artunay O, Yuzbasioglu E, Oncel M, Bahcecioglu H. Vitreous levels of VEGF, IL-8, and TNF-alpha in retinal detachment. Curr Eye Res. (2010) 35:505–9. doi: 10.3109/02713681003597248
45. Wang M, Yao R, Wang Y. Silencing of IL13RA2 promotes partial epithelial-mesenchymal transition in hepatocellular carcinoma via ERK signaling pathway activation. FEBS Open Bio. (2020) 10:229–36. doi: 10.1002/2211-5463.12774
46. Newman JP, Wang GY, Arima K, Guan SP, Waters MR, Cavenee WK, et al. Interleukin-13 receptor alpha 2 cooperates with EGFRvIII signaling to promote glioblastoma multiforme. Nat Commun. (2017) 8:1913.
47. Song X, Traub B, Shi J, Kornmann M. Possible roles of interleukin-4 and -13 and their receptors in gastric and colon cancer. Int J Mol Sci. (2021) 22: 727.
48. Barderas R, Bartolome RA, Fernandez-Acenero MJ, Torres S, Casal JI. High expression of IL-13 receptor alpha2 in colorectal cancer is associated with invasion, liver metastasis, and poor prognosis. Cancer Res. (2012) 72:2780–90. doi: 10.1158/0008-5472.CAN-11-4090
49. Tabata Y, Khurana HGK. IL-13 receptor isoforms: breaking through the complexity. Curr Allergy Asthma. (2007) 7:338–45. doi: 10.1007/s11882-007-0051-x
50. Wills-Karp M, Finkelman FD. Untangling the complex web of IL-4- and IL-13-mediated signaling pathways. Sci Signal. (2008) 1:e55. doi: 10.1126/scisignal.1.51.pe55
51. McKenzie AN, Fallon PG. Decoy receptors in the regulation of T helper cell type 2 responses. J Exp Med. (2003) 197:675–9. doi: 10.1084/jem.20030096
52. Mm M-K. Opposing roles for IL-13 and IL-13 receptor alpha 2 in health and disease. Immunol Rev. (2004) 202:191–202. doi: 10.1111/j.0105-2896.2004.00210.x
53. Chung SI, Horton JA, Ramalingam TR, White AO, Chung EJ, Hudak KE, et al. IL-13 is a therapeutic target in radiation lung injury. Sci Rep. (2016) 6:39714. doi: 10.1038/srep39714
54. Rosen MJ, Frey MR, Washington MK, Chaturvedi R, Kuhnhein LA, Matta P, et al. STAT6 activation in ulcerative colitis: a new target for prevention of IL-13-induced colon epithelial cell dysfunction. Inflamm Bowel Dis. (2011) 17:2224–34. doi: 10.1002/ibd.21628
Keywords: vitreous, retinal pigment epithelial cells, RNA sequencing, IL13Rα2, proliferative vitreoretinopathy
Citation: Qi H, Dong L, Fang D, Chen L, Wang Y, Fan N, Mao X, Wu W, Yan X, Zhang G, Zhang S and Lei H (2022) A Novel Role of IL13Rα2 in the Pathogenesis of Proliferative Vitreoretinopathy. Front. Med. 9:831436. doi: 10.3389/fmed.2022.831436
Received: 08 December 2021; Accepted: 28 April 2022;
Published: 13 June 2022.
Edited by:
Jorge M. Barcia, Catholic University of Valencia San Vicente Mártir, SpainReviewed by:
Xiaoqing Guo, Harvard Medical School, United StatesTeng Teng, Capital Medical University, China
Maryada Sharma, Postgraduate Institute of Medical Education and Research, India
Copyright © 2022 Qi, Dong, Fang, Chen, Wang, Fan, Mao, Wu, Yan, Zhang, Zhang and Lei. This is an open-access article distributed under the terms of the Creative Commons Attribution License (CC BY). The use, distribution or reproduction in other forums is permitted, provided the original author(s) and the copyright owner(s) are credited and that the original publication in this journal is cited, in accordance with accepted academic practice. No use, distribution or reproduction is permitted which does not comply with these terms.
*Correspondence: Shaochong Zhang, emhhbmdzaGFvY2hvbmdAZ3p6b2MuY29t; Hetian Lei, bGVpaGV0aWFuMThAaG90bWFpbC5jb20=