- 1Immunoreceptors del Sistema Innat i Adaptatiu, Institut d'Investigacions Biomèdiques August Pi i Sunyer (IDIBAPS), Barcelona, Spain
- 2Department of Autoimmune Diseases, ICMiD, Hospital Clínic, University of Barcelona, Barcelona, Spain
- 3Primary Care Centre Les Corts, Consorci d'Atenció Primària de Salut Barcelona Esquerra (CAPSBE), Barcelona, Spain
- 4Primary Healthcare Transversal Research Group, Institut d'Investigacions Biomèdiques August Pi i Sunyer (IDIBAPS), Barcelona, Spain
- 5Department of Statistics and Operations Research, Universitat Politècnica de Catalunya (UPC), Barcelona, Spain
- 6Research and Innovation Group in Autoimmune Diseases, RGAD-Sanitas Digital Hospital, Barcelona, Spain
- 7Systemic Autoimmune Diseases Unit, Internal Medicine, Millenium Clinic, Sanitas, Barcelona, Spain
- 8Servei d'Immunologia, Centre de Diagnòstic Biomèdic, Hospital Clínic de Barcelona, Barcelona, Spain
- 9Departament de Biomedicina, Facultat de Medicina, Universitat de Barcelona, Barcelona, Spain
Primary Sjögren's syndrome (pSS) is an autoimmune disease triggered by a combination of environmental and host genetic factors, which results in the focal lymphocytic infiltration of exocrine glands causing eye and mouth dryness. Glandular infiltrates include T and B cell subsets positive for CD5 and/or CD6, two surface scavenger receptors involved in the fine-tuning of intracellular signals mediated by the antigen-specific receptor complex of T (TCR) and B (BCR) cells. Moreover, the epithelial cells of inflamed glands overexpress CD166/ALCAM, a CD6 ligand involved in homo and heterotypic cell adhesion interactions. All this, together with the reported association of functionally relevant single nucleotide polymorphisms (SNPs) of CD5, CD6, and CD166/ALCAM with the risk or prognosis of some immune-mediated inflammatory disorders, led us to investigate similar associations in a local cohort of patients with pSS. The logistic regression analyses of individual SNPs showed the association of CD5 rs2241002T with anti-Ro/La positivity, CD6 rs17824933C with neutropenia, and CD6 rs11230563T with increased leukopenia and neutropenia but decreased peripheral nervous system EULAR Sjögren's syndrome disease activity index (ESSDAI). Further analyses showed the association of haplotypes from CD5 (rs2241002T-rs2229177C) with anemia and thrombocytopenia, CD6 (rs17824933G-rs11230563C-rs12360861G) with cutaneous ESSDAI, and CD166/ALCAM (rs6437585C-rs579565A-rs1044243C and rs6437585C-rs579565G-rs1044243T) with disease susceptibility and several analytical parameters (anti-nuclear antibodies, neurological ESSDAI, and hematologic cytopenias). These results support the relevance of gene variation at loci coding for cell surface receptors involved in the modulation of T and B lymphocyte activation (CD5, CD6) and epithelial-immune cell adhesion (CD166/ALCAM) in modulating the clinical and analytical outcomes in patients with pSS.
Introduction
Primary Sjögren's syndrome (pSS) is a chronic, systemic rheumatic disease characterized by the lymphoplasmacytic infiltration of exocrine glands—mainly salivary and lacrimal glands—resulting in sicca syndrome and systemic manifestations (1). It is a common disorder (prevalence of 0.5–1% in the general population) with a female/male ratio of approximately 9:1 (2, 3). pSS is considered as a complex and multifactorial process whose pathogenesis involves environmental factors, such as viral infections, combined with sex hormonal, genetic and epigenetic factors, causing epithelial cell barrier disruption followed by an abnormal immune cell-mediated inflammatory response (4, 5).
Periductal immune cell infiltrates in the affected glands of patients with pSS include CD5- and/or CD6-positive T and B cells (6–9). CD5 and CD6 are two highly homologous lymphocyte surface receptors of the scavenger receptor cysteine-rich superfamily (SRCR-SF) (10). They are expressed on all T cells and a subset of B cells (B1a) involved in the production of polyreactive natural antibodies, and they are abnormally expanded in the peripheral blood of patients undergoing autoimmune disorders, such as pSS and systemic lupus erythematosus (SLE) (6, 10, 11). Both receptors are signal-transducing molecules that modulate intracellular activation and differentiation signals from the antigen-specific receptor complex of T (TCR) and B (BCR) cells to which CD5 and CD6 physically associate (12–14). In addition, CD5 and CD6 act as pattern recognition receptors (PRRs) by recognizing microbial-associated molecular patterns (MAMPs) from the bacterial, fungal, viral, and parasitic origin (15–17). Particularly, CD5 has been shown to interact with fungal β-glucans (18), hepatitis C virus (19), and tegumental structures of Echinococcus granulosus (20), while CD6 interacts with lipopolysaccharide, lipoteichoic acid, and peptidoglycan from Gram-negative and -positive bacteria (21), gp120 from human immunodeficiency virus 1 (22), and the tegumental components of E. granulosus (20).
A central phenomenon in the immunopathogenesis of pSS is the aberrant epithelial cell activation status (pSS has been described as an autoimmune epithelitis) (23, 24). This results in the increased expression of human leukocyte antigen (HLA)-DR, costimulatory, and adhesion molecules. Among the latter, overexpression of the well-known CD6 ligand CD166/ALCAM has been reported in pSS epithelial lesions (8, 9, 25). CD166/ALCAM (for activated leukocyte cell adhesion molecule) is an adhesion molecule of the immunoglobulin superfamily with a broad tissue distribution, such as epithelia, endothelia, neurons, myeloid progenitors, hematopoietic stem cells, mesenchymal stem cells, bone marrow stromal cells, and cancer cells (26). Interestingly, CD166/ALCAM establishes not only homophilic (ALCAM-ALCAM) but also higher affinity heterophilic (ALCAM-CD6) interactions with the CD6 lymphocyte receptor, which facilitate cell interactions of T or B1a lymphocytes with epithelial and endothelial cells (26–28).
Studies aimed at the genetic basis of pSS show the associations of both human leukocyte antigen (HLA) and non-HLA genes with pSS susceptibility. The HLA-DR and HLA-DQ alleles have shown the strongest associations across different ethnicities (29, 30). The long, though still incomplete, list of non-HLA genetic polymorphisms contributed by genome-wide (GWAS) and gene-driven association studies includes interferon regulatory factor 5 (IRF5), signal transducer and activator of transcription 4 (STAT4), B lymphocyte kinase (BLK), tumor necrosis factor-α (TNF-α), interleukin (IL)-4, IL-10, IL-12A, C-X-C chemokine receptor type 5 (CXCR5), surfactant protein-D (SP-D), and Mannan-binding lectin (MBL) (30–36).
Single nucleotide polymorphisms (SNPs) at the CD5, CD6, and CD166/ALCAM gene loci have been associated with different immune-mediated inflammatory diseases (IMID) (37). Specifically, CD5 variation has been associated with rheumatoid arthritis (RA) susceptibility (38) and the development of lupus nephritis (39). CD6 and CD166/ALCAM SNPs have been identified and validated as risk factors for the development and progression of multiple sclerosis (MS) (40–42), psoriasis severity (43), Behçet's disease risk (44), and inflammatory bowel disease (IBD) risk (45, 46).
Given the expression of CD5, CD6, and CD166/ALCAM in pSS inflamed tissue and the association of their SNPs with other IMIDs, we hypothesize that variation at CD5, CD6, and CD166/ALCAM loci may impact the pathology of pSS. The results of the present candidate gene-driven association analysis show that CD5, CD6, and CD166/ALCAM genetic polymorphisms are associated with the clinical and analytical parameters of the disease in a local cohort of pSS patients.
Materials and Methods
Subjects
Consecutive patients with pSS (n = 212) attending to the Hospital Clínic de Barcelona, Barcelona, Spain were included in the study (Table 1). Patients fulfilled the 2002/2016 criteria approved by the American-European Consensus Group (47). Exclusion criteria for considering SS as a primary disease were chronic HCV/HIV infection, previous lymphoproliferative processes, and associated systemic autoimmune diseases. Diagnostic tests for SS (ocular tests, parotid scintigraphy, and salivary gland biopsy) were performed according to the European Community Study Group recommendations (48).
Unrelated volunteers (n = 305) from the Banc de Sang i Teixits (BST) from Generalitat de Catalunya were included as controls (143 women and 162 men).
The study was approved by the local Hospital Ethics Committee, and written informed consent was obtained from all participants before inclusion and blood extraction.
Definition of Variables
Disease diagnosis was defined as the time when the attending physician confirmed the fulfillment of the 2002/2016 criteria (47). The main disease features were retrospectively collected and analyzed. The following clinical variables were selected for harmonization and further refinement: age, gender, ethnicity, country of residence, fulfillment of the 2002/2016 criteria items, antinuclear antibodies (ANA), rheumatoid factor (RF), C3 and C4 levels, and cryoglobulins. The epidemiological variables included in this study were age at diagnosis, gender, and ethnicity according to the Food and Drug Administration (FDA) definitions (49). Systemic involvement at diagnosis was retrospectively classified and scored according to the EULAR Sjögren's syndrome disease activity index (ESSDAI) (50), which evaluates 12 domains or organ systems, and the ClinESSDAI (51), which evaluates the same domains but excluding the last (biological) domain. Each domain is divided into 3–4 levels according to the degree of activity and scored as 0 (no activity), 1 (low activity), 2 (moderate activity), or 3 (high activity) (52). Disease activity states (DAS) were calculated as: no activity (global score = 0), low activity (global score 1–4), moderate activity (global score 5–13), and high activity (global score ≥14) (53).
Additionally, cumulative systemic involvement was classified and scored according to the ESSDAI. Cumulative systemic involvement was defined as the systemic activity present since the diagnosis of pSS to the last medical visit.
Genotyping
DNA was purified from ethylenediaminetetraacetic acid (EDTA)-treated peripheral blood using the QIAamp DNA Blood Mini Kit (Qiagen, Venio, The Netherlands) and subjected to real-time (RT)-PCR with the following TaqMan probes: CD5 rs2241002 (assay number: C__25472293_20), CD5 rs2229177 (assay number: C___3237272_10), CD6 rs17824933 (assay number: C__33967506_10), CD6 rs11230563 (assay number: C__31727142_10), CD6 rs12360861 (assay number: C__25922320_10), and CD166/ALCAM rs6437585 (assay number: C__29281365_20), all from ThermoFisher Scientific (Barcelona, Spain). Primers for PCR amplification and further sequence-base typing (PCR-SBT) of CD166/ALCAM rs579565 and rs1044243, which lie 2 bp apart from each other, were also from ThermoFisher Scientific (Hs00666884_CE assay). SNP genotyping and clinical data are available at a public repository (54).
Statistical Analyses
Statistical analyses were performed with R 3.6.0 (R Foundation for Statistical Computing, Vienna, Austria). Genotypic statistical associations among the SNPs and susceptibility or disease outcomes were tested by generalized linear models using the R package “SNPstats.” For each analysis, 4 models were generated (codominant, dominant, recessive, and log-additive), and the model with the lowest Akaike information criterion (AIC) was chosen. The p values were corrected for false discovery rate (FDR, q values). Haplotypic analyses were performed with generalized linear models by means of the R package “haplo.stats.”
Results
A total of 212 patients with pSS with a mean age of 54 years at diagnosis were included in the study, most of them were women (95.3%) and presented dry mouth (100%) and dry eyes (96.7%). The association of individual SNPs with susceptibility and the clinical parameters of pSS was first investigated (Supplementary Table 1). Sex is a major risk factor in pSS, so statistical models for subphenotypical analyses were generated with or without including sex as a covariant, and their goodness of fit compared with the AIC. The results presented here do not include sex as a covariant, as these models had lower AIC. Susceptibility analyses were performed only with female patient cases and controls. No significant association was found between any individual CD5, CD6, and CD166/ALCAM SNPs and pSS susceptibility, although the CD166/ALCAM rs579565A allele showed a trend for statistical association in women (q = 0.064) (Table 2).
Regarding association with pSS clinical parameters, the CD5 rs2241002C allele was found associated with a higher frequency of anti-Ro/La antibody positivity (Table 3). The CD6 rs17824933G allele was associated with decreased risk of neutropenia (Table 3), and the CD6 rs11230563T allele with increased leukopenia and neutropenia, but decreased ESSDAI peripheral nervous system (PNS) activity (Table 3).
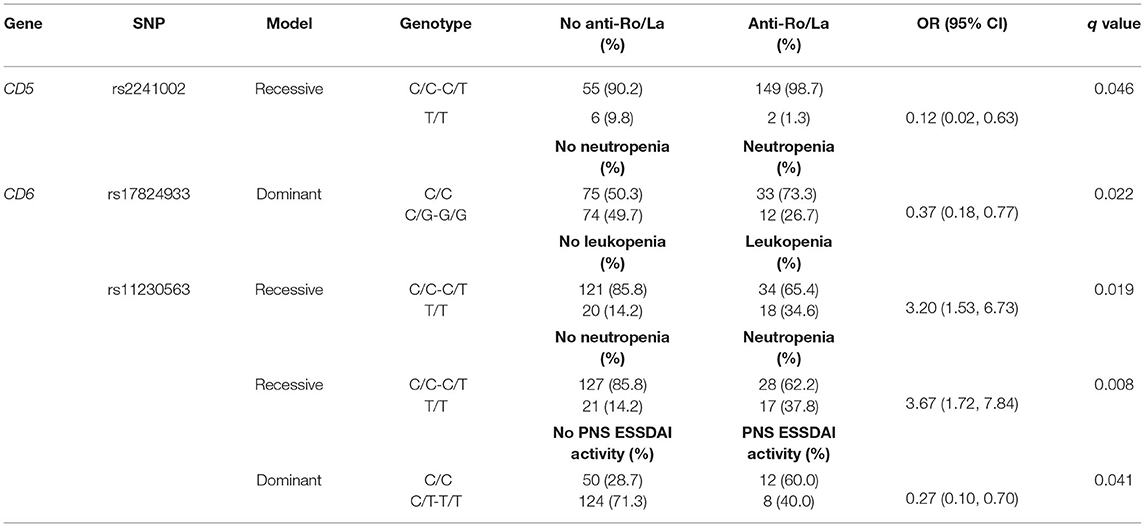
Table 3. Logistic regression analyses of CD5 and CD6 SNPs association with anti-Ro/La antibodies, neutropenia, leukopenia, and peripheral nervous system (PNS) EULAR Sjögren's syndrome disease activity index (ESSDAI) activity.
Haplotypic analyses showed the association of CD5 rs2241002T-rs2229177C haplotype with an increased risk of anemia and thrombocytopenia (Table 4). The CD6 rs17824933G-rs11230563C-rs12360861G haplotype was associated with an increased risk of ESSDAI cutaneous activity (Table 5). The CD166/ALCAM rs6437585C-rs579565G-rs1044243T haplotype was associated with increased ANA positivity, ESSDAI PNS activity, and hematologic cytopenias, such as anemia and lymphopenia (Table 6).
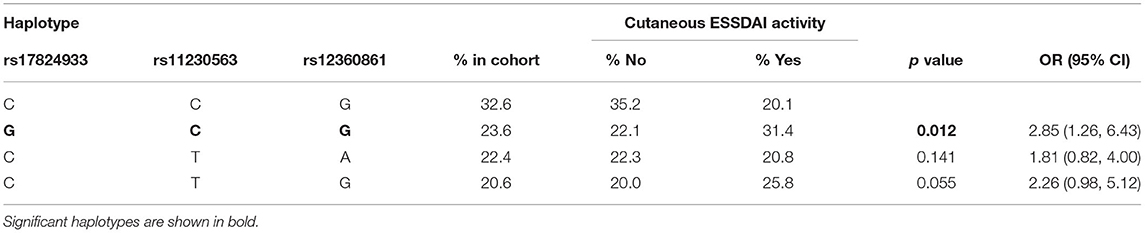
Table 5. Logistic regression analysis of CD6 haplotype association to cutaneous affectation. Only the 4 most common haplotypes are shown.
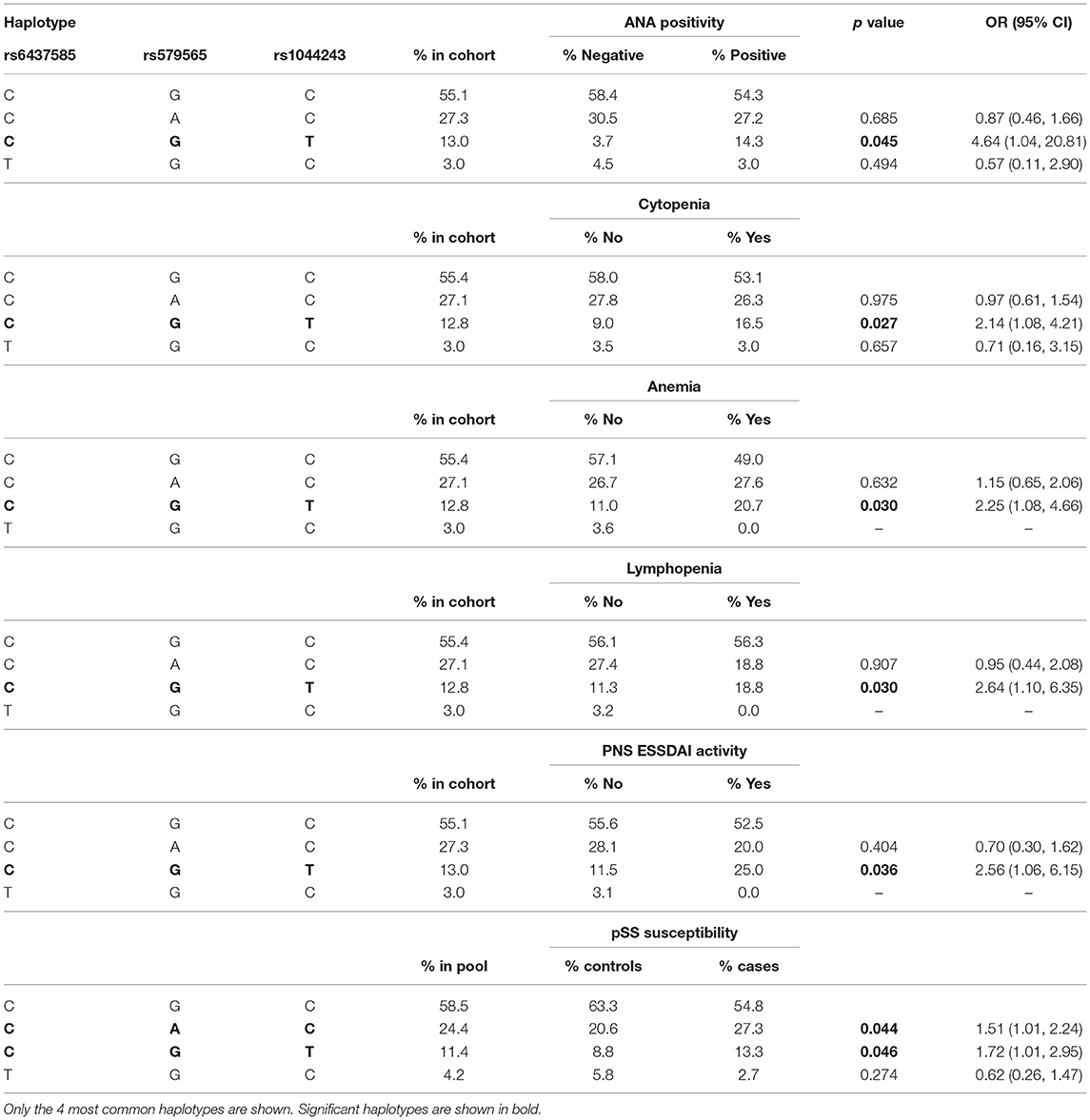
Table 6. Logistic regression analysis of CD166/ALCAM haplotype association with anti-nuclear antibodies (ANA), cytopenia, anemia, lymphopenia, peripheral nervous system (PNS) ESSDAI activity, and pSS susceptibility.
Case-control analyses to assess the influence of CD5, CD6, and CD166/ALCAM haplotypes on pSS risk were also performed. To account for the gender skew in pSS, only female cases and controls were included in this haplotypic analysis. The results showed that the only associations with pSS susceptibility were with the CD166/ALCAM rs643785C-rs579565A-rs1044243C (CAC) and rs643785C-rs579565G-rs1044243T (CGT) haplotypes (Table 6), which were over-represented in the case cohort, indicating the association of rs579565A and rs1044243T alleles with pSS susceptibility.
Discussion
The pathophysiology of pSS is complex and multifactorial. How the innate and adaptive immune responses are dysregulated through both cellular- and humoral-mediated processes (30) is still poorly understood. Identifying genetic factors associated with pSS may help in the better comprehension of pathogenic mechanisms leading to the overall pSS phenotype and clinically heterogeneous subsets of patients (55). By using a candidate gene-driven strategy, the present work shows evidence on the impact of CD5, CD6, and CD166/ALCAM gene variants in the susceptibility and clinical expression of pSS, thus supporting their involvement in pSS pathophysiology.
CD5, CD6, and CD166/ALCAM variation study in pSS responds to: first, the three genes encode functionally relevant and related cell surface receptors. CD5 and CD6 are highly homologous lymphocyte receptors of the ancient and highly conserved SRCR-SF and are encoded by contiguous genes likely resulting from a duplication event (56, 57). Both CD5 and CD6 are expressed by all T cell types and the B1a cell subset, with the lower levels of expression in other cell types (e.g., macrophages, dendritic cells, or natural killer cells) (10, 13), all found in pSS periductal immune cell infiltrates (6–9). From the functional point of view, CD5 and CD6 are considered relevant signaling immune receptors at the interphase of the innate and adaptive immune responses as a result from their involvement in (i) the recognition and sensing of bacterial, viral, and/or parasitic MAMPs (17) and (ii) the fine-tuning of lymphocyte activation signals delivered by clonotypic T and B antigen-specific receptors, which they are physically associated to (58–60). While the nature of the endogenous CD5 ligand is yet uncertain, one of the most-well studied CD6 ligands is CD166/ALCAM, a cell adhesion molecule overexpressed in pSS salivary gland epithelial cells (8, 9, 25), but also RA synovium (61), MS blood–brain barrier endothelium (62), and lupus nephritis kidneys (63), thus contributing to T and B cell migration and infiltration at inflamed tissues in autoimmune processes.
Second, several CD5, CD6, and/or CD166/ALCAM gene variants have been associated with different IMIDs, such as RA (38), lupus nephritis (39), MS (40–42), psoriasis (43), Behçet's disease (44), and IBD (45, 46) (Supplementary Table 2). The CD5, CD6, and CD166/ALCAM SNPs included in the present study were selected not only for being informative in the above-mentioned IMIDs but also for their putative functional relevance. Regarding CD5, the rs2241002 (C > T) and rs2229177 (C > T) SNPs result in amino acid substitutions at the extracellular SRCR2 domain (Pro224>Leu) and just next to a cytoplasmic ITAM-like motif (Ala471>Val), respectively (39, 64). Functional studies show that homozygous carriers for the ancestral rs2241002C-rs2229177C haplotype (Pro224-Ala471) present increased T-cell proliferation and cytokine release and a bias toward a Th2 profile, compared with the homozygous carriers of more recently derived rs2241002C-rs2229177T haplotype (Pro224-Val471) (39). Regarding CD6, the rs11230563 (C>T) and rs12360861 (G>A) SNPs result in amino acid substitutions at the extracellular SRCR2 (Arg225>Trp) and SRCR3 (Ala271>Thr) domains, respectively, and the intron 1 rs17824933 (C>G) SNP results in the skipping of exon 5 and expression of a CD6 isoform lacking the SRCR3 domain (CD6Δd3), in which the CD166/ALCAM-binding site locates (65). Functional studies show that the CD6 rs11230563C-rs2074225C haplotype (Arg225-Ala257) results in higher CD6 surface expression on CD4+ and CD8+ naïve T cells and NKT cells (41). The carriage of CD6 rs17824933G allele results in an increased CD6Δd3/full-length CD6 ratio driving to lower CD4+ T cell activation responses (66). Regarding CD166/ALCAM, the rs6437585 (C > T) SNP maps at the 5'-untranslated region (UTR) and is known to influence the transcriptional activity of CD166/ALCAM (42, 67), while the rs579565 (G > A) and rs1044243 (C > T) SNPs result in synonymous (Leu300>Leu) and non-synonymous (Thr301>Met) changes at the extracellular C1-like domain (42) with still unknown functional consequences.
Individual SNP and haplotypic analyses showed the association of CD5, CD6, and CD166/ALCAM SNPs with different pSS clinical parameters. Thus, the CD5 rs2241002C allele and the minor CD5 rs2241002T-rs2229177C haplotype, previously associated with a more aggressive form of SLE (lupus nephritis) (39), showed association with anti-Ro/anti-La antibody positivity, and with anemia and thrombocytopenia, respectively. This could be interpreted as a result of hyperactive autoantibody-producing B cells (most likely CD5+ B1a cells) in pSS carriers of such CD5 variants.
The individual CD6 rs11230563C allele was associated with the higher risk of PNS ESSDAI activity, and the CD6 rs17824933G-rs11230563C-rs12360861G haplotype with cutaneous ESSDAI activity. This is reminiscent of the increased MS risk and psoriasis severity previously reported for rs11230563C allele (40, 43, 68–70). It is noteworthy that both rs17824933G and rs11230563C alleles were associated with the reduced risk of neutropenia. Since both alleles impact the extracellular region of CD6 (an increased expression of CD6Δd3 isoform and Arg225 to Trp substitution at SRCR2, respectively), it remains to be analyzed whether this relates to the reported surface CD6 (and CD166/ALCAM) expression by hematopoietic cell progenitors present in the bone marrow and in mobilized blood (71, 72).
The CD166/ALCAM (rs6437585C-rs579565G-rs1044243T) haplotype was found associated with the increased incidences of ANA positivity, neurological affectation, and hematologic cytopenias. These results further support the damaging role of CD6 rs17824933G and rs11230563C alleles and of CD166/ALCAM rs1044243T allele by worsening some analytical and clinical parameters of pSS. Interestingly, haplotypic analyses showed the association of CD166/ALCAM rs6437585C-rs579565A-rs1044243C and rs6437585C-rs579565G-rs1044243T haplotypes with increased pSS susceptibility. This supports a role for minor rs579565A and rs1044243T alleles in pSS susceptibility, which is reminiscent of the earlier age of MS diagnosis reported for the rs579565A allele (42).
The association of CD5, CD6, and CD166/ALCAM SNPs with pSS phenotype highlights the relevance of genetic variation at loci related with immune activation in pSS pathophysiology. In addition, this is illustrated by the previously reported association of HLA-DR and HLA-DQ, IRF5, STAT4, BLK, TNF, IL4RA, IL10, IL12A, CXCR5, TNFAIP3, MTHFR, CD28, CTLA4, IKZF1, HIF1A, AKNA, SFTPD, and MBL2 loci with pSS (30–36, 73, 74) (Supplementary Table 3). Interestingly, CD5 and CD6 interact with microorganisms, such as SP-D and mannose-binding lectin (encoded by SFTPD and MBL, respectively). This brings out the relevance of microbial/pathogen recognition in pSS.
We are aware of some limitations in the present study regarding: first, the limited number of pSS cases and controls in this single-center study. Second, only a single patient cohort was available for the analysis in spite of our efforts to access validation cohorts with the necessary subphenotypical data for replicates. Therefore, validation in an independent cohort is pending for significant confirmation of the role of CD5, CD6, and CD166/ALCAM gene variants in pSS.
In summary, we identified the CD166/ALCAM rs579565 and rs1044243 SNPs as pSS risk markers, and the CD5 rs2241002, CD6 rs17824933 and rs11230563 and CD166/ALCAM rs1044243 SNPs as disease modifiers markers. Further studies in independent cohorts will be required to validate these results. Nevertheless, our observations are the first to support a role for CD5, CD6, and CD166/ALCAM variation in pSS, and they highlight the shared immunogenetic basis of different IMIDs (75). These results, along with the identification of other genetic factors involved in pSS etiopathogenesis, may also help to classify patients and allow better identification, management, and treatment of the disease.
Data Availability Statement
The datasets presented in this study can be found in online repositories. The names of the repository/repositories and accession number(s) can be found below: https://github.com/SergiCLl/CD5-CD6-ALCAM-pSS, CD5-CD6-ALCAM-pSS.
Ethics Statement
The studies involving human participants were reviewed and approved by Comitè d'Ètica de la Investigació amb medicaments (CEIm) Hospital Clínic de Barcelona. The patients/participants provided their written informed consent to participate in this study.
Author Contributions
FL, MR-C, and PB-Z conceptualized the study. SC-L, HG, SA, MC-F, and NA-B contributed to genetic studies. HG, MR-C, and PB-Z contributed to sample and clinical information collection. SC-L and BK contributed to statistical analyses. SC-L, PB-Z, and FL wrote the original draft. All authors read, critically revised, and approved the final version of the manuscript.
Funding
This work was supported by grants from the Spanish Ministerio de Economía y Competitividad (MINECO; SAF2016-80535-R) and the Ministerio de Ciencia e Innovación (MCIN/AEI/10.13039/501100011033, PID2019-106658RB-I00), co-financed by the European Development Regional Fund (A way to achieve Europe) ERDF, and Agència de Gestió d'Ajuts Universitaris i de Recerca (AGAUR; 2017/SGR/1582) from Generalitat de Catalunya. SC-L is recipient of a fellowship from the Spanish Ministerio de Educación, Cultura y Deporte (FPU15/02897) and Universitat de Barcelona (Convocatòria d'ajuts per a alumnes que estan finalitzant la tesi doctoral). Funders had no access to clinical data and did not participate in study design, data analysis, or manuscript redaction.
Conflict of Interest
FL is a founding partner at Sepsia Therapeutics SL.
The remaining authors declare that the research was conducted in the absence of any commercial or financial relationships that could be construed as a potential conflict of interest.
Publisher's Note
All claims expressed in this article are solely those of the authors and do not necessarily represent those of their affiliated organizations, or those of the publisher, the editors and the reviewers. Any product that may be evaluated in this article, or claim that may be made by its manufacturer, is not guaranteed or endorsed by the publisher.
Acknowledgments
We thank Belén Suárez and Maria Teresa Arias for technical support in genetic analyses, and Marcos Isamat for manuscript editing and critical comments.
Supplementary Material
The Supplementary Material for this article can be found online at: https://www.frontiersin.org/articles/10.3389/fmed.2022.822290/full#supplementary-material
References
1. Parisis D, Chivasso C, Perret J, Soyfoo MS, Delporte C. Current state of knowledge on primary sjögren's syndrome, an autoimmune exocrinopathy. J Clin Med. (2020) 9:2299. doi: 10.3390/jcm9072299
2. Haugen AJ, Peen E, Hultén B, Johannessen AC, Brun JG, Halse AK, et al. Estimation of the prevalence of primary Sjögren's syndrome in two age-different community-based populations using two sets of classification criteria: the Hordaland Health Study. Scand J Rheumatol. (2008) 37:30–4. doi: 10.1080/03009740701678712
3. Ramos-Casals M, Brito-Zerón P, Kostov B, Sisó-Almirall A, Bosch X, Buss D, et al. Google-driven search for big data in autoimmune geoepidemiology: analysis of 394,827 patients with systemic autoimmune diseases. Autoimmun Rev. (2015) 14:670–9. doi: 10.1016/j.autrev.2015.03.008
4. Sandhya P, Kurien B, Danda D, Scofield R. Update on pathogenesis of sjogren's syndrome. Curr Rheumatol Rev. (2016) 13:5–22. doi: 10.2174/1573397112666160714164149
5. Cafaro G, Bursi R, Chatzis LG, Fulvio G, Ferro F, Bartoloni E, et al. One year in review 2021: Sjögren's syndrome. Clin Exp Rheumatol. (2021) 39:3–13.
6. Youinou P, Mackenzie L, le Masson G, Papadopoulos NM, Jouquan J, Pennec YL, et al. CD5-expressing B lymphocytes in the blood and salivary glands of patients with primary Sjögren's syndrome. J Autoimmun. (1988) 1:185–194. doi: 10.1016/0896-8411(88)90025-X
7. Zeher M, Surányi P, Nagy G, Szegedi G. B cells expressing CD5 in minor labial salivary glands of patients with primary Sjögren's syndrome. Arthritis Rheum. (1990) 33:453–453. doi: 10.1002/art.1780330329
8. Alonso R, Buors C, Le Dantec C, Hillion S, Pers JO, Saraux A, et al. Aberrant expression of CD6 on B-cell subsets from patients with Sjögren's syndrome. J Autoimmun. (2010) 35:336–41. doi: 10.1016/j.jaut.2010.07.005
9. Le Dantec C, Alonso R, Fali T, Montero E, Devauchelle V, Saraux A, et al. Rationale for treating primary Sjögren's syndrome patients with an anti-CD6 monoclonal antibody (Itolizumab). Immunol Res. (2013) 56:341–7. doi: 10.1007/s12026-013-8423-x
10. Martínez VG, Moestrup SK, Holmskov U, Mollenhauer J, Lozano F. The conserved scavenger receptor cysteine-rich super family in therapy and diagnosis. Pharmacol Rev. (2011) 63:967–1000. doi: 10.1124/pr.111.004523
11. Hardy RR, Hayakawa K. CD5 B Cells, a fetal B cell lineage. Adv Immunol. (1993) 55:297–339. doi: 10.1016/S0065-2776(08)60512-X
12. Santos RF, Oliveira L, Carmo AM. Tuning T Cell Activation: The function of CD6 at the immunological synapse and in T cell responses. Curr Drug Targets. (2016) 17:630–9. doi: 10.2174/1389450116666150531152439
13. Burgueño-Bucio E, Mier-Aguilar CA, Soldevila G. The multiple faces of CD5. J Leukoc Biol. (2019) 105:891–904. doi: 10.1002/JLB.MR0618-226R
14. Mori D, Grégoire C, Voisinne G, Celis-Gutierrez J, Aussel R, Girard L, et al. The T cell CD6 receptor operates a multitask signalosome with opposite functions in T cell activation. J Exp Med. (2021) 218:e20201011. doi: 10.1084/jem.20201011
15. Consuegra-Fernández M, Aranda F, Simões I, Orta M, Sarukhan A, Lozano F. CD5 as a target for immune-based therapies. Crit Rev Immunol. (2015) 35:85–115. doi: 10.1615/CritRevImmunol.2015013532
16. Sarukhan A, Martinez-Florensa M, Escoda-Ferran C, Carrasco E, Carreras E, Lozano F. Pattern recognition by CD6: a scavenger-like lymphocyte receptor. Curr Drug Targets. (2016) 17:640–50. doi: 10.2174/1389450116666150316224308
17. Velasco-de Andrés M, Casadó-Llombart S, Català C, Leyton-Pereira A, Lozano F, Aranda F. Soluble CD5 and CD6: lymphocytic class I scavenger receptors as immunotherapeutic agents. Cells. (2020) 9:2589. doi: 10.3390/cells9122589
18. Vera J, Fenutria R, Canadas O, Figueras M, Mota R, Sarrias M-R, et al. The CD5 ectodomain interacts with conserved fungal cell wall components and protects from zymosan-induced septic shock-like syndrome. Proc Natl Acad Sci. (2009) 106:1506–11. doi: 10.1073/pnas.0805846106
19. Sarhan MA, Pham TNQ, Chen AY, Michalak TI. Hepatitis C virus infection of human T lymphocytes is mediated by CD5. J Virol. (2012) 86:3723–35. doi: 10.1128/JVI.06956-11
20. Mourglia-Ettlin G, Miles S, Velasco-De-Andrés M, Armiger-Borràs N, Cucher M, Dematteis S, et al. The ectodomains of the lymphocyte scavenger receptors CD5 and CD6 interact with tegumental antigens from Echinococcus granulosus sensu lato and protect mice against secondary cystic echinococcosis. PLoS Negl Trop Dis. (2018) 12:e0006891. doi: 10.1371/journal.pntd.0006891
21. Sarrias M-R, Farnós M, Mota R, Sánchez-Barbero F, Ibáñez A, Gimferrer I, et al. CD6 binds to pathogen-associated molecular patterns and protects from LPS-induced septic shock. Proc Natl Acad Sci U S A. (2007) 104:11724–9. doi: 10.1073/pnas.0702815104
22. Carrasco E, Escoda C, Alvarez-Fenrández C, Sanchez-Palomino S, Carreras E, Gatell JM, et al. A role for scavenger-like lymphocyte receptor CD6 in HIV-1 viral infection. AIDS Res Hum Retroviruses. (2014) 30:A49–50. doi: 10.1089/aid.2014.5085.abstract
23. Tsunawaki S, Nakamura S, Ohyama Y, Sasaki M, Ikebe-Hiroki A, Hiraki A, et al. Possible function of salivary gland epithelial cells as nonprofessional antigen-presenting cells in the development of Sjögren's syndrome. J Rheumatol. (2002) 29:1884–96.
24. Voulgarelis M, Tzioufas AG. Pathogenetic mechanisms in the initiation and perpetuation of Sjögren's syndrome. Nat Rev Rheumatol. (2010) 6:529–37. doi: 10.1038/nrrheum.2010.118
25. Abidi SMA, Saifullah MK, Zafiropulos MD, Kaput C, Bowen MA, Cotton C, et al. CD166 expression, characterization, and localization in salivary epithelium: Implications for function during sialoadenitis. J Clin Immunol. (2006) 26:12–21. doi: 10.1007/s10875-006-7119-6
26. Ferragut F, Vachetta VS, Troncoso MF, Rabinovich GA, Elola MT. ALCAM/CD166: A pleiotropic mediator of cell adhesion, stemness and cancer progression. Cytokine Growth Factor Rev. (2021) 61:27–37. doi: 10.1016/j.cytogfr.2021.07.001
27. Singer NG, Fox DA, Haqqi TM, Beretta L, Endres JS, Prohaska S, et al. CD6: expression during development, apoptosis and selection of human and mouse thymocytes. Int Immunol. (2002) 14:585–97. doi: 10.1093/intimm/dxf025
28. Te Riet J, Zimmerman AW, Cambi A, Joosten B, Speller S, Torensma R, et al. Distinct kinetic and mechanical properties govern ALCAM-mediated interactions as shown by single-molecule force spectroscopy. J Cell Sci. (2007) 120:3965–76. doi: 10.1242/jcs.004010
29. Cruz-Tapias P, Rojas-Villarraga A, Maier-Moore S, Anaya JM. HLA and Sjögren's syndrome susceptibility. A meta-analysis of worldwide studies. Autoimmun Rev. (2012) 11:281–7. doi: 10.1016/j.autrev.2011.10.002
30. Lessard CJ, Li H, Adrianto I, Ice JA, Rasmussen A, Grundahl KM, et al. Variants at multiple loci implicated in both innate and adaptive immune responses are associated with Sjögren's syndrome. Nat Genet. (2013) 45:1284–94. doi: 10.1038/ng.2792
31. Hulkkonen J, Pertovaara M, Antonen J, Lahdenpohja N, Pasternack A, Hurme M. Genetic association between interleukin-10 promoter region polymorphisms and primary Sjögren's syndrome. Arthritis Rheum. (2001) 44:176–9. doi: 10.1002/1529-0131(200101)44:1<176::AID-ANR23>3.0.CO;2-K
32. Ramos-Casals M, Font J, Brito-Zeron P, Trejo O, Garcia-Carrasco M, Lozano F. Interleukin-4 receptor alpha polymorphisms in primary Sjögren's syndrome. Clin Exp Rheumatol. (2004) 22:374.
33. Ramos-Casals M, Brito-Zerón P, Soria N, Nardi N, Vargas A, Muñoz S, et al. Mannose-binding lectin-low genotypes are associated with milder systemic and immunological disease expression in primary Sjögren's syndrome. Rheumatology. (2009) 48:65–9. doi: 10.1093/rheumatology/ken411
34. Qin B, Wang J, Liang Y, Yang Z, Zhong R. The association between TNF-α, IL-10 gene polymorphisms and primary sjögren's syndrome: a meta-analysis and systemic review. PLoS ONE. (2013) 8:e63401. doi: 10.1371/journal.pone.0063401
35. Soto-Cárdenas MJ, Gandía M, Brito-Zerón P, Arias MT, Armiger N, Bové A, et al. Etiopathogenic role of surfactant protein D in the clinical and immunological expression of primary sjögren syndrome. J Rheumatol. (2015) 42:111–8. doi: 10.3899/jrheum.140394
36. Ben-Eli H, Gomel N, Aframian DJ, Abu-Seir R, Perlman R, Ben-Chetrit E, et al. SNP variations in IL10, TNFα and TNFAIP3 genes in patients with dry eye syndrome and Sjogren's syndrome. J Inflamm (United Kingdom). (2019) 16:1–6. doi: 10.1186/s12950-019-0209-z
37. Casadó-Llombart S, Velasco-de Andrés M, Català C, Leyton-Pereira A, Lozano F, Bosch E. Contribution of evolutionary selected immune gene polymorphism to immune-related disorders: the case of lymphocyte scavenger receptors CD5 and CD6. Int J Mol Sci. (2021) 22:5315. doi: 10.3390/ijms22105315
38. Eyre S, Bowes J, Diogo D, Lee A, Barton A, Martin P, et al. High-density genetic mapping identifies new susceptibility loci for rheumatoid arthritis. Nat Genet. (2012) 44:1336–40. doi: 10.1038/ng.2462
39. Cenit MC, Martínez-Florensa M, Consuegra M, Bonet L, Carnero-Montoro E, Armiger N, et al. Analysis of ancestral and functionally relevant CD5 variants in systemic lupus erythematosus patients. PLoS ONE. (2014) 9:e113090. doi: 10.1371/journal.pone.0113090
40. De Jager PL, Jia X, Wang J, de Bakker PIW, Ottoboni L, Aggarwal NT, et al. Meta-analysis of genome scans and replication identify CD6, IRF8 and TNFRSF1A as new multiple sclerosis susceptibility loci. Nat Genet. (2009) 41:776–82. doi: 10.1038/ng.401
41. Swaminathan B, Cuapio A, Alloza I, Matesanz F, Alcina A, García-Barcina M, et al. Fine Mapping and Functional Analysis of the Multiple Sclerosis Risk Gene CD6. PLoS ONE. (2013) 8:e62376. doi: 10.1371/journal.pone.0062376
42. Wagner M, Bilinska M, Pokryszko-Dragan A, Sobczynski M, Cyrul M, Kusnierczyk P, et al. ALCAM and CD6 - multiple sclerosis risk factors. J Neuroimmunol. (2014) 276:98–103. doi: 10.1016/j.jneuroim.2014.08.621
43. Consuegra-Fernández M, Julià M, Martínez-Florensa M, Aranda F, Català C, Armiger-Borràs N, et al. Genetic and experimental evidence for the involvement of the CD6 lymphocyte receptor in psoriasis. Cell Mol Immunol. (2018) 15:898–906. doi: 10.1038/cmi.2017.119
44. Zheng M, Zhang L, Yu H, Hu J, Cao Q, Huang G, et al. Genetic polymorphisms of cell adhesion molecules in Behçet's disease in a Chinese Han population. Sci Rep. (2016) 6:24974. doi: 10.1038/srep24974
45. Jostins L, Ripke S, Weersma RK, Duerr RH, McGovern DP, Hui KY, et al. Host–microbe interactions have shaped the genetic architecture of inflammatory bowel disease. Nature. (2012) 491:119–24. doi: 10.1038/nature11582
46. Ellinghaus D, Jostins L, Spain SL, Cortes A, Bethune J, Han B, et al. Analysis of five chronic inflammatory diseases identifies 27 new associations and highlights disease-specific patterns at shared loci. Nat Genet. (2016) 48:510–8. doi: 10.1038/ng.3528
47. Vitali C, Bombardieri S, Jonsson R, Moutsopoulos HM, Alexander EL, Carsons SE, et al. Classification criteria for Sjögren's syndrome: A revised version of the European criteria proposed by the American-European Consensus Group. Ann Rheum Dis. (2002) 61:554–8. doi: 10.1136/ard.61.6.554
48. Vitali C, Bombardieri S, Moutsopoulos HM, Balestrieri G, Bencivelli W, Bernstein RM, et al. Preliminary criteria for the classification of Sjögren's syndrome. Results of a prospective concerted action supported by the European community. Arthritis Rheum. (1993) 36:340–7. doi: 10.1002/art.1780360309
49. Revisions to the Standards for the Classification of Federal Data on Race Ethnicity (49). Fed. Reg. 62. Available online at: https://www.federalregister.gov/documents/1997/10/30/97-28653/revisions-to-the-standards-for-the-classification-of-federal-data-on-race-and-ethnicity. (accessed June 15, 2021).
50. Seror R, Ravaud P, Bowman SJ, Baron G, Tzioufas A, Theander E, et al. EULAR Sjögren's syndrome disease activity index: Development of a consensus systemic disease activity index for primary Sjögren's syndrome. Ann Rheum Dis. (2010) 69:1103–9. doi: 10.1136/ard.2009.110619
51. Seror R, Meiners P, Baron G, Bootsma H, Bowman SJ, Vitali C, et al. Development of the ClinESSDAI: A clinical score without biological domain. A tool for biological studies. Ann Rheum Dis. (2016) 75:1945–50. doi: 10.1136/annrheumdis-2015-208504
52. Seror R, Bowman SJ, Brito-Zeron P, Theander E, Bootsma H, Tzioufas A, et al. EULAR Sjögren's syndrome disease activity index (ESSDAI): a user guide. RMD Open. (2015) 1:22. doi: 10.1136/rmdopen-2014-000022
53. Seror R, Bootsma H, Saraux A, Bowman SJ, Theander E, Brun JG, et al. Defining disease activity states and clinically meaningful improvement in primary Sjögren's syndrome with EULAR primary Sjögren's syndrome disease activity (ESSDAI) and patient-reported indexes (ESSPRI). Ann Rheum Dis. (2016) 75:382–9. doi: 10.1136/annrheumdis-2014-206008
54. Casadó-Llombart S, Gheitasi H, Ariño S, Consuegra-Fernández M, Armiger-Borràs N, Kostov B, et al. Data from: CD5, CD6 and CD166/ALCAM sequencing in pSS patients. (2021). Available online at: https://github.com/SergiCLl/CD5-CD6-ALCAM-pSS (accessed November 26, 2021).
55. Cobb BL, Lessard CJ, Harley JB, Moser KL. Genes and Sjögren's syndrome. Rheum Dis Clin North Am. (2008) 34:847–68. doi: 10.1016/j.rdc.2008.08.003
56. Lecomte O, Bock JB, Birren BW, Vollrath D, Parnes JR. Molecular linkage of the mouse CD5 and CD6 genes. Immunogenetics. (1996) 44:385–90. doi: 10.1007/BF02602784
57. Padilla O, Calvo J, Vila JM, Arman M, Gimferrer I, Places L, et al. Genomic organization of the human CD5 gene. Immunogenetics. (2000) 51:993–1001. doi: 10.1007/s002510000235
58. Lankester AC, van Schijndel GMW, Cordell JL, van Noesel CJM, van Lier RAW. CD5 is associated with the human B cell antigen receptor complex. Eur J Immunol. (1994) 24:812–6. doi: 10.1002/eji.1830240406
59. Gimferrer I, Calvo M, Mittelbrunn M, Farnós M, Sarrias MR, Enrich C, et al. Relevance of CD6-mediated interactions in T cell activation and proliferation. J Immunol. (2004) 173:2262–70. doi: 10.4049/jimmunol.173.4.2262
60. Cho J-H, Sprent J. TCR tuning of T cell subsets. Immunol Rev. (2018) 283:129–37. doi: 10.1111/imr.12646
61. Levesque MC, Heinly CS, Whichard LP, Patel DD. Cytokine-regulated expression of activated leukocyte cell adhesion molecule (CD166) on monocyte-lineage cells and in rheumatoid arthritis synovium. Arthritis Rheum. (1998) 41:2221–9. doi: 10.1002/1529-0131(199812)41:12<2221::AID-ART18>3.0.CO;2-I
62. Cayrol R, Wosik K, Berard JL, Dodelet-Devillers A, Ifergan I, Kebir H, et al. Activated leukocyte cell adhesion molecule promotes leukocyte trafficking into the central nervous system. Nat Immunol. (2008) 9:137–45. doi: 10.1038/ni1551
63. Chalmers SA, Ramachandran RA, Garcia SJ, Der E, Herlitz L, Ampudia J, et al. The CD6/ALCAM pathway promotes lupus nephritis via T cell–mediated responses. J Clin Invest. (2022) 132. doi: 10.1172/JCI147334
64. Carnero-Montoro E, Bonet L, Engelken J, Bielig T, Martínez-Florensa M, Lozano F, et al. Evolutionary and functional evidence for positive selection at the human CD5 immune receptor gene. Mol Biol Evol. (2012) 29:811–23. doi: 10.1093/molbev/msr251
65. Castro MAA, Oliveira MI, Nunes RJ, Fabre S, Barbosa R, Peixoto A, et al. Extracellular isoforms of CD6 generated by alternative splicing regulate targeting of CD6 to the immunological synapse. J Immunol. (2007) 178:4351–61. doi: 10.4049/jimmunol.178.7.4351
66. Kofler DM, Severson CA, Mousissian N, De Jager PL, Hafler DA. The CD6 multiple sclerosis susceptibility allele is associated with alterations in CD4+ T cell proliferation. J Immunol. (2011) 187:3286–91. doi: 10.4049/jimmunol.1100626
67. Zhou P, Du LF, Lv GQ, Yu XM, Gu YL, Li JP, et al. Functional polymorphisms in CD166/ALCAM gene associated with increased risk for breast cancer in a Chinese population. Breast Cancer Res Treat. (2011) 128:527–34. doi: 10.1007/s10549-011-1365-x
68. Johnson BA, Wang J, Taylor EM, Caillier SJ, Herbert J, Khan OA, et al. Multiple sclerosis susceptibility alleles in African Americans. Genes Immun. (2010) 11:343–50. doi: 10.1038/gene.2009.81
69. Swaminathan B, Matesanz F, Cavanillas ML, Alloza I, Otaegui D, Olascoaga J, et al. Validation of the CD6 and TNFRSF1A loci as risk factors for multiple sclerosis in Spain. J Neuroimmunol. (2010) 223:100–3. doi: 10.1016/j.jneuroim.2010.03.020
70. Leppä V, Surakka I, Tienari PJ, Elovaara I, Compston A, Sawcer S, et al. The genetic association of variants in CD6, TNFRSF1A and IRF8 to multiple sclerosis: a multicenter case-control study. PLoS ONE. (2011) 6:e18813. doi: 10.1371/journal.pone.0018813
71. Cortés F, Deschaseaux F, Uchida N, Labastie MC, Friera AM, He D, et al. HCA, an immunoglobulin-like adhesion molecule present on the earliest human hematopoietic precursor cells, is also expressed by stromal cells in blood-forming tissues. Blood. (1999) 93:826–37. doi: 10.1182/blood.V93.3.826
72. Ohneda O, Ohneda K, Arai F, Lee J, Miyamoto T, Fukushima Y, et al. ALCAM (CD166): Its role in hematopoietic and endothelial development. Blood. (2001) 98:2134–42. doi: 10.1182/blood.V98.7.2134
73. Song GG, Bae SC, Seo YH, Kim JH, Choi SJ, Ji JD, et al. Meta-analysis of functional MBL polymorphisms: Associations with rheumatoid arthritis and primary Sjögren's syndrome. Z Rheumatol. (2014) 73:657–64. doi: 10.1007/s00393-014-1408-x
74. Harris VM, Hal Scofield R, Sivils KL. Genetics in Sjögren's syndrome: where we are and where we go. Clin Exp Rheumatol. (2019) 37:S234–S239.
Keywords: Sjögren's syndrome, CD5, CD6, CD166/ALCAM, polymorphism, SNP
Citation: Casadó-Llombart S, Gheitasi H, Ariño S, Consuegra-Fernández M, Armiger-Borràs N, Kostov B, Ramos-Casals M, Brito-Zerón P and Lozano F (2022) Gene Variation at Immunomodulatory and Cell Adhesion Molecules Loci Impacts Primary Sjögren's Syndrome. Front. Med. 9:822290. doi: 10.3389/fmed.2022.822290
Received: 25 November 2021; Accepted: 16 February 2022;
Published: 18 March 2022.
Edited by:
Yiping Wang, Westmead Institute for Medical Research, AustraliaReviewed by:
Clio Mavragani, National and Kapodistrian University of Athens, GreeceStefano Bombardieri, University of Pisa, Italy
Copyright © 2022 Casadó-Llombart, Gheitasi, Ariño, Consuegra-Fernández, Armiger-Borràs, Kostov, Ramos-Casals, Brito-Zerón and Lozano. This is an open-access article distributed under the terms of the Creative Commons Attribution License (CC BY). The use, distribution or reproduction in other forums is permitted, provided the original author(s) and the copyright owner(s) are credited and that the original publication in this journal is cited, in accordance with accepted academic practice. No use, distribution or reproduction is permitted which does not comply with these terms.
*Correspondence: Pilar Brito-Zerón, bXBicml0b0BzYW5pdGFzLmVz; Francisco Lozano, ZmxvemFub0BjbGluaWMuY2F0
†These authors share first authorship