- 1Department of Intensive Care Unit, The Affiliated Hospital of Guizhou Medical University, Guiyang, China
- 2School of Clinical Medicine, Guizhou Medical University, Guiyang, China
The efficacy and safety of early renal replacement therapy (eRRT) for critically ill patients with acute kidney injury (AKI) remain controversial. Therefore, the purpose of our study was to perform an up-to-date meta-analysis with the trial sequential analysis (TSA) of randomized controlled trials (RCTs) to evaluate the therapeutic effect of eRRT on patients in an intensive care unit (ICU). We extensively searched MEDLINE, EMBASE, LILACS, the Cochrane Central Register of Controlled Trials and ClinicalTrials.gov, Gray Literature Report, and Bielefeld Academic Search Engine (BASE), and conducted an updated search on December 27, 2021. The included studies were RCTs, which compared the efficacy and safety of eRRT and delayed renal replacement therapy (dRRT) on critically ill patients with AKI. We adopted TSA and sensitivity analysis to strengthen the robustness of the results. About 12 RCTs with a total of 5,423 participants were included. Patients receiving eRRT and dRRT had the similar rate of all-cause mortality at day 28 (38.7% vs. 38.9%) [risk ratio (RR), 1.00; 95%CI, 0.93–1.07, p = 0.93, I2 = 0%, p = 0.93]. A sensitivity and subgroup analysis produced similar results for the primary outcome. TSA showed that the required information size was 5,034, and the cumulative Z-curve crossed trial sequential monitoring boundaries for futility. Patients receiving eRRT had a higher rate of renal replacement therapy (RRT) (RR, 1.50, 95% CI: 1.28–1.76, p < 0.00001, I2 = 96%), and experienced more adverse events comparing to those receiving dRRT (RR: 1.41, 95% CI: 1.22–1.63, p < 0.0001, heterogeneity not applied). The most remarkable and important experimental finding is that, to our knowledge, the current meta-analysis included the largest sample size from the RCTs, which were published in the past 10 years to date, show that eRRT had no significant survival benefit for ill patients with AKI compared with dRRT and TSA indicating that no more studies were needed to confirm it.
Trial Registration: INPLASY, INPLASY2020120030. Registered 04 December 2020.
Introduction
Acute kidney injury (AKI) is an important complication in patients who are admitted to an intensive care unit (ICU) and is associated with a high risk of death (1). AKI could result from prerenal azotemia, acute tubular necrosis, or post-renal obstructive disease (2). Renal replacement therapy (RRT) is an effective treatment for AKI when indicated (3), helping to optimize fluid administration and hemodynamic management, etc. (4–6). Therefore, RRT is often applied whenever patients suffered from fluid overload and/or other complications [7–9] (7, 8).
However, there is no optimal timing of RRT, and whether early RRT (eRRT) is superior to delayed RRT (dRRT) is also a matter of controversy (9–11). Some multicenter, randomized controlled trials (RCTs) indicated that eRRT has not shown clinical benefits (12, 13), but others demonstrated that eRRT significantly reduces the mortality of patients with AKI (6, 14).
In view of controversial results, recently, two high-quality RCTs were published in NEJM (15) and Lancet (16), which compared the effects of eRRT vs. dRRT on critically ill patients with AKI. So, we did an updated systematic review involving sufficient patients from RCTs to explore the uncertainty arising from conflicting results. Review evidence may provide contemporary evidence to guide clinical policies and practices.
In the traditional meta-analysis, the positive results are easy to be changed after more evidence is accumulated (17). The trial sequential analysis (TSA), however, has the potential to make conclusions more reliable than traditional meta-analyses (18, 19). TSA can also provide “futility boundaries” to identify invalid conclusions earlier, preventing researchers from spending a lot of resources on unneeded research. Here, we performed TSA to minimize random errors in a cumulative meta-analysis and strengthen the robustness of the results.
Methods
Overview
Our systematic review was registered at INPLASY (INPLASY2020120030), (https://inplasy.com/inplasy-2020-12-0030/), following a prespecified protocol and complying with the Preferred Reporting Items for Systematic Meta-Analyses (PRISMA) guideline (20).
Eligibility Criteria
We included studies, which met all the following criteria: (1) RCTs published from January 1, 2010, to October 11, 2020, and an updated search was conducted on December 27, 2021. (2) Subjects were critically ill patients (≥18 years) with AKI (KDIGO stage 2 or 3, or at the failure stage of RIFLE) (21). (3) With a clear criterion used for defining eRRT and dRRT.
Search Strategy
We did an extensive electronic search from January 1, 2010, to October 11, 2020, based on the recommendations (20, 22) from MEDLINE, EMBASE, LILACS, the Cochrane Central Register of Controlled Trials, and ClinicalTrials.gov, and conducted an updated search on December 27, 2021. Besides, we searched the two gray literature databases: The Grey Literature Report (www.greylit.org/) and Bielefeld Academic Search Engine (BASE) (http://www.base-search.net/about/en/). The detailed retrieval method was included as Supplementary Materials.
Selection of Studies
The two authors independently screened research titles and abstracts that were potentially relevant to this article according to the inclusion criteria. To determine studies that meet the inclusion criteria of this study, we retain potentially relevant data and research information and evaluate the full text when necessary. Any disagreements in the selection of studies were negotiated or consulted with a third review author if necessary.
Data Extraction and Effect Measure
Data were independently entered into an Excel spreadsheet by three reviewers. We collected the data including first author, recruitment period, the year of publication, sample size, mean age, gender, RRT modality, primary outcome: all-cause mortality at day 28, secondary outcomes: all-cause mortality at days 60 and 90, all-cause mortality in an ICU and a hospital, the length of stay (LOS)in an ICU and a hospital, ventilator-free days vasoactive agents free days/rate of RRT dependence at day 28, and the risk of total adverse events and specific adverse events (bleeding, arrhythmia, and hypotension). Any disagreements in data extraction were negotiated or consulted with a third review author if necessary. For binary classification results, the risk ratio (RR) with 95% CI was used to describe the binary classification results, and the mean difference (MD) with 95% CI was used to describe continuous variables.
Risk of Bias Assessment and Certainly Assessment
At least two review authors independently evaluated the methodological quality of each included trial and assessed the risk of bias according to the Cochrane Handbook for Systematic Reviews of Interventions (22). Included trials were judged for the risk of bias in all bias domains (23). Any disagreements concerning the assessment of the risk of bias were negotiated with a third review author if necessary. We assessed the certainty of the evidence for all-cause mortality at day 28, the LOS of survivors in an ICU and a hospital, the rate of RRT dependence at day 28, and the incidence of total adverse events by grading with Grading of Recommendations, Assessment, Development, and Evaluations (GRADE) pro GDT (24) available from gdt.gradepro.org.
Reporting Biases Assessment
Visual inspection of funnel plots and contour-enhanced funnel in the graphic was used to assess the potential existence of reporting bias. Besides, Begg's and Egger's tests were performed to quantitatively assess the publication bias.
Data Analysis
We undertook this systematic review following the recommendations of the Cochrane Handbook for Systematic Reviews of Interventions (22) and Meta package for the R language. We performed a meta-analysis for the outcomes with comparable methods in a similar population when more than one trial was included by using statistical software Review Manager 5. We set p < 0.05 as the statistical significance and represented individual trial and summary reports with 95% CIs. Moreover, we evaluated the statistical heterogeneity of each outcome through the I2 statistic measure and chi-squared test, in which p ≤ 0.1 was regarded as to be significant. We adopted a random-effects model in all analyses because of fears of that clinical heterogeneity and methodological heterogeneity. To explore the eventual sources of heterogeneity, we conducted the respective subgroup analyses based on these characteristics including study design, patient population, the modality of RRT, and the presence of sepsis at randomization for the primary outcome. We also performed sensitivity analyses for the primary outcome such that investigating the potential effect of excluding studies judged as being at unclear or a high risk of bias in any of the risk of bias domains. Furthermore, we implemented the two important analyses, the “best-worst-case” scenario and the “worst-best-case” scenario (25) to assess the possible impact of missing data on the primary outcome.
Trial Sequential Analyses
Recent studies showed that TSA has the potential to make conclusions more reliable than those traditional meta-analyses (18, 26). Also in traditional meta-analyses, it is sometimes to get positive conclusions resulting from “random error” rather than a “real” intervention effect (27). In this situation, the TSA software provides methods to minimize the incidences of these false positive results (28). TSA is also able to provide “futility boundaries” that could draw invalid conclusions early (27, 29). In our analysis, we conducted TSA using the TSA software (version 0.9.5.10 Beta) (19), of which the information size was calculated according to an anticipated—relative risk reduction (RRR) of 15% with a power of 90% (15, 30) and a type-I error value of 1% (two-sided).
Results
Research Results
We totally screened 2,108 titles and abstracts of which 504 full-text reports were assessed for eligibility and 492 references were excluded due to some reasons (Figure 1). About 12 records were finally included for a meta-analysis and 9 of them were selected in quantitative synthesis for the primary outcome in our meta-analysis (Figure 1).
Characteristics of the Studies
About 12 RCTs fulfilled the planned inclusion criteria. The baseline characteristics of the included studies are presented in Table 1. Around 9 of 12 studies came from a multi-center (12, 13, 15, 16, 30–34), while the other 3 came from a single-center (6, 35, 36). Severity scores of diseases reported mostly were Sequential Organ Failure Assessment (SOFA) and Acute Physiology and Chronic Health Evaluation (APACHE II).
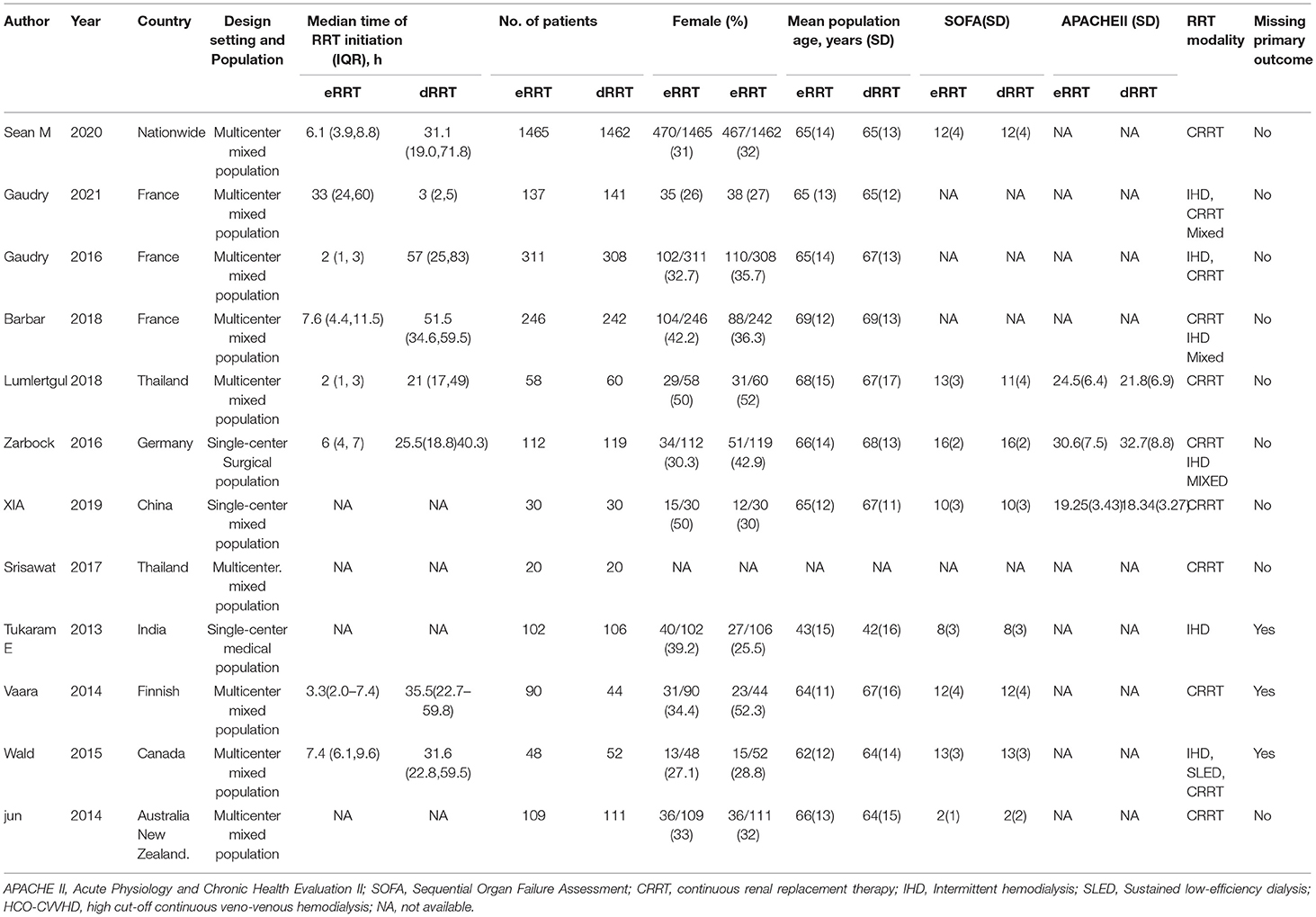
Table 1. Baseline characteristics of the randomized controlled clinical trials included in the meta-analysis.
The criteria for eRRT and dRRT of the included trials are depicted in Table 2. In the RENAL study by Jun et al. (31), as 439 patients were assigned to four groups according to variant initiating time of CRRT other than to early and delay initiation, we only selected the patients from the earliest (within 7.1 h of AKI diagnosis) and latest group (46 h after AKI diagnosis). Therefore, only 220 of 439 patients were included for analysis. In a trial by Lumlertgul et al. (13), the furosemide stress test (FST) was performed for all patients with AKI, FST-nonresponsive patients were then randomized to eRRT or dRRT, and 118 of 162 patients were included for analysis. In the trials by Srisawat et al. (34) and Xia et al. (36), patients were grouped based on the level of plasma neutrophil gelatinase-associated lipocalin (pNGAL), and only patients with a high level of pNGAL were assigned to receive either eRRT or dRRT, resulting in the inclusion of 40 of 60 patients from Srisawat's trial and of 60 of 100 patients from Xia's trial in our analysis.
About 12 RCTs with a total of 5,423 patients were included in a meta-analysis, of whom 2,728 received eRRT and other 2,695 received dRRT. The basic characteristics of 5,423 patients were detailed in Table 3. These patients had similar age, sex ratio, serum creatinine, blood urea nitrogen, and SOFA score in eRRT and dRRT groups.
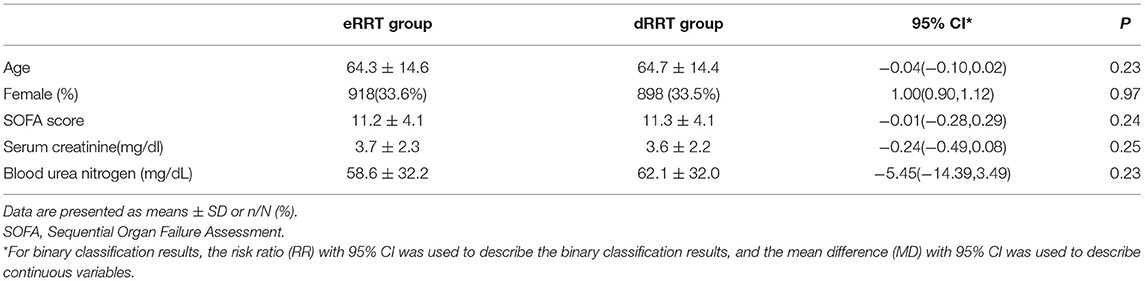
Table 3. Characteristics of patients from the randomized controlled trials (RCTs) included in the meta-analysis.
Quality of Studies
We evaluated the risk of bias for each included article following the Cochrane ROB (risk of bias) tool (Figure 2A) and graphed the degrees of risk of bias (Figure 2B). Of the included studies, 8 (67%) studies were judged to have a low risk of bias, and 3 (25%) were judged to have a high risk of bias, while 1 study (8%) was unclear.
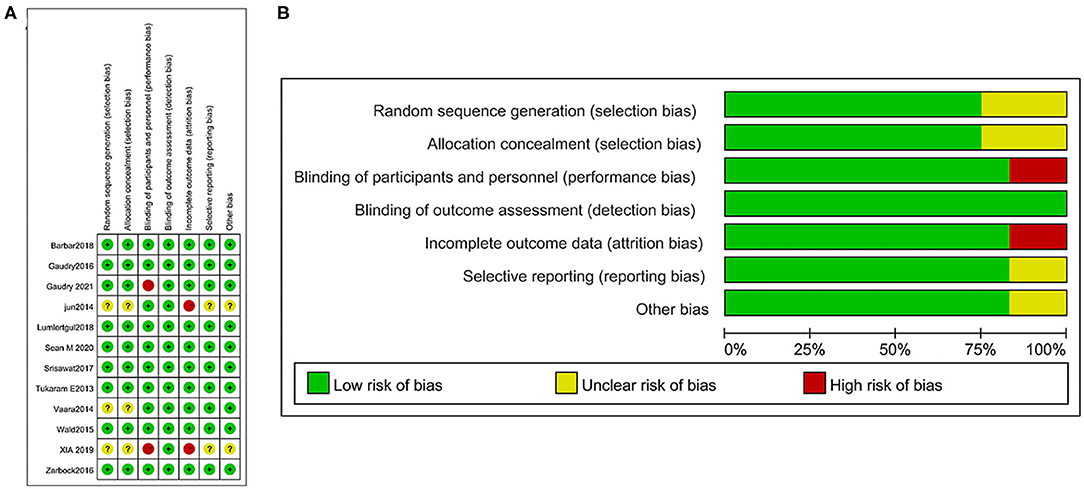
Figure 2. Risk of bias summary: a review of authors' judgments concerning the risk of bias in the included studies (A) and risk of bias graph: a review of authors' judgments about each risk of bias item presented as percentages across all included studies (B).
Certainty of the Evidence
Six main outcomes were graded by the GRADE for the quality of evidence (Table 4). The results of the analysis showed that the certainty of the evidence was high for the mortality at day 28, the rate of RRT, moderate for the LOS of survivors in an ICU, the LOS survivors in a hospital, and total adverse events. Meanwhile, the evidence for RRT dependence at day 28 was of low certainty due to serious inconsistency and imprecision.
Quantitative Data Synthesis
Primary Outcomes
About 9 of 12 trials with a total of 4,981 participants and a mean follow-up of 28 days reported all-cause mortality (6, 12, 13, 15, 16, 30, 34, 36). About 442 of 5,423 (8.1%) patients did not have complete data on the prespecified primary outcome. For the remaining 4,981 patients, the rates of all-cause mortality at day 28 (38.7% in eRRT and 38.9% in dRRT) were not significantly different between the two groups (RR 1.00, 95% CI: 0.93–1.07, p = 0.93) (Figure 3A). There was no inconsistency factor across trials, indicating a statistical heterogeneity (I2 = 0%, p = 0.69).
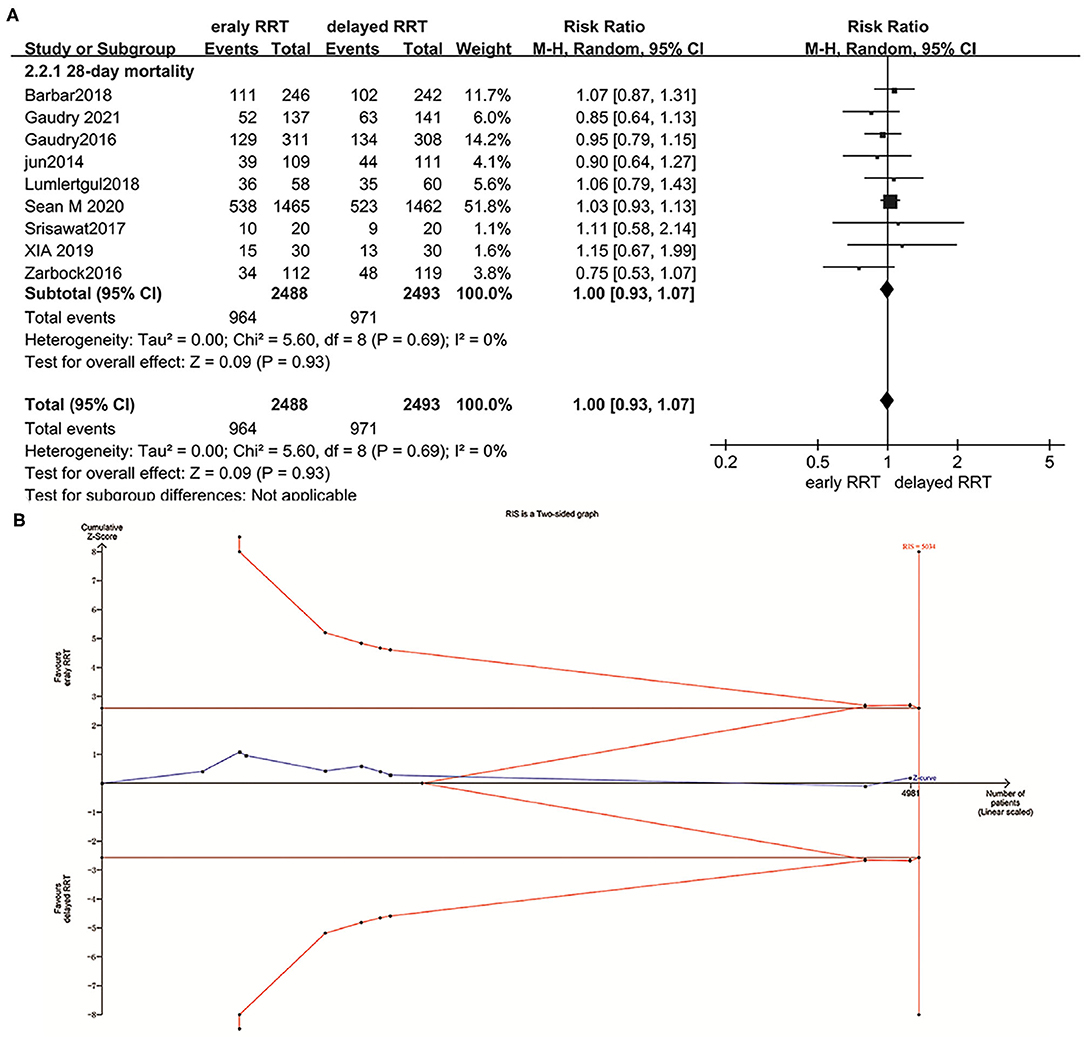
Figure 3. Forest plot for all-cause mortality at day 28 (A) and trial sequential analysis (TSA) for all-cause mortality at day 28 (B).
Reporting Biases for the Primary Outcome
The visual assessment showed that there was a partial asymmetry in the funnel plot and contour-enhanced funnel (Figures 4A,B), while p-values (0.51 and 0.75) were found in the Egger's test and Begg's test in a dominant model, respectively, which indicated that no statistical significance was found (Table 5).
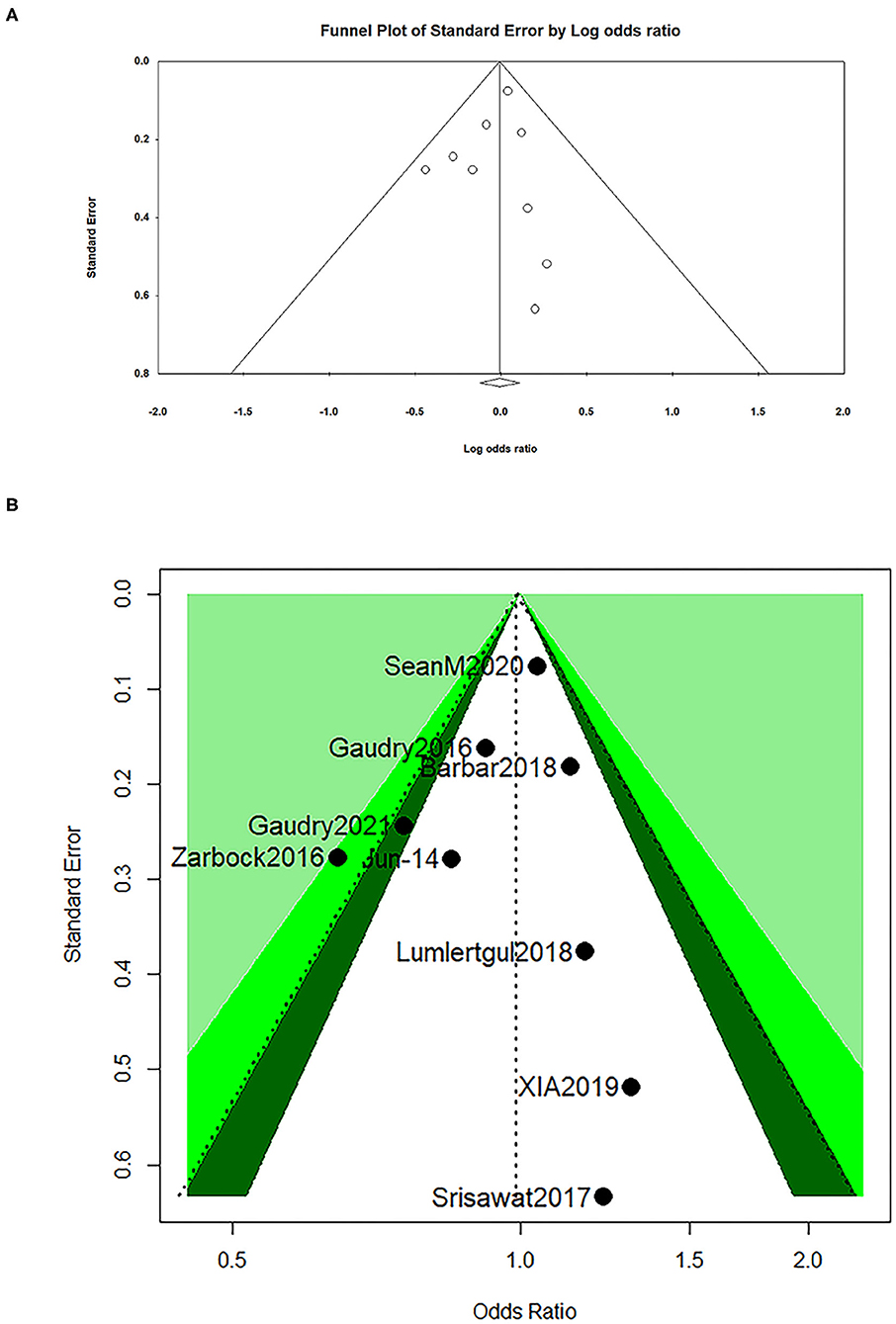
Figure 4. Funnel plot for all-cause mortality at day 28 (A) and contour-enhanced funnel for all-cause mortality at day 28 (B).
A Subgroup Analysis for the Primary Outcome
To investigate heterogeneity, we performed a subgroup analysis for the primary outcome. As depicted in Table 6, there was no substantial difference between the groups regarding mortality at day 28 across prespecified subgroups based on study design, patient population, the modality of RRT, and the presence of sepsis at randomization for the primary outcome.
TSA for the Primary Outcome
The trial sequential analysis showed that the required information size was 5,034 and the cumulative Z-curve crossed trial sequential monitoring boundaries for futility (Figure 3B), indicating that there is no difference between the eRRT and dRRT in terms of mortality at day 28 and no more studies were needed.
A Sensitivity Analysis for the Primary Outcome
To evaluate the potential effect of non-low bias studies on the robustness of data analysis, we performed a sensitivity analysis for the primary outcome by removing individual trials at a time with non-low bias in each domain. This sensitivity analysis produced similar results for the primary outcome (Figure 5A). Furthermore, we also conducted a sensitivity analysis by using the best-worst-case scenario analysis and worst-best-case scenario analysis to explore the influence of incomplete primary outcome data, which suggested that incomplete outcome data did not influence the results, either confirmed by the best-worst-case scenario analysis (RR 0.87, 95% CI: 0.68–1.11, p = 0.26, I2 = 85%) (Figure 5B) or by worst-best-case scenario analysis (RR 1.08, 95% CI: 0.85–1.36, p = 0.54, I2 = 84%) (Figure 5C), respectively.
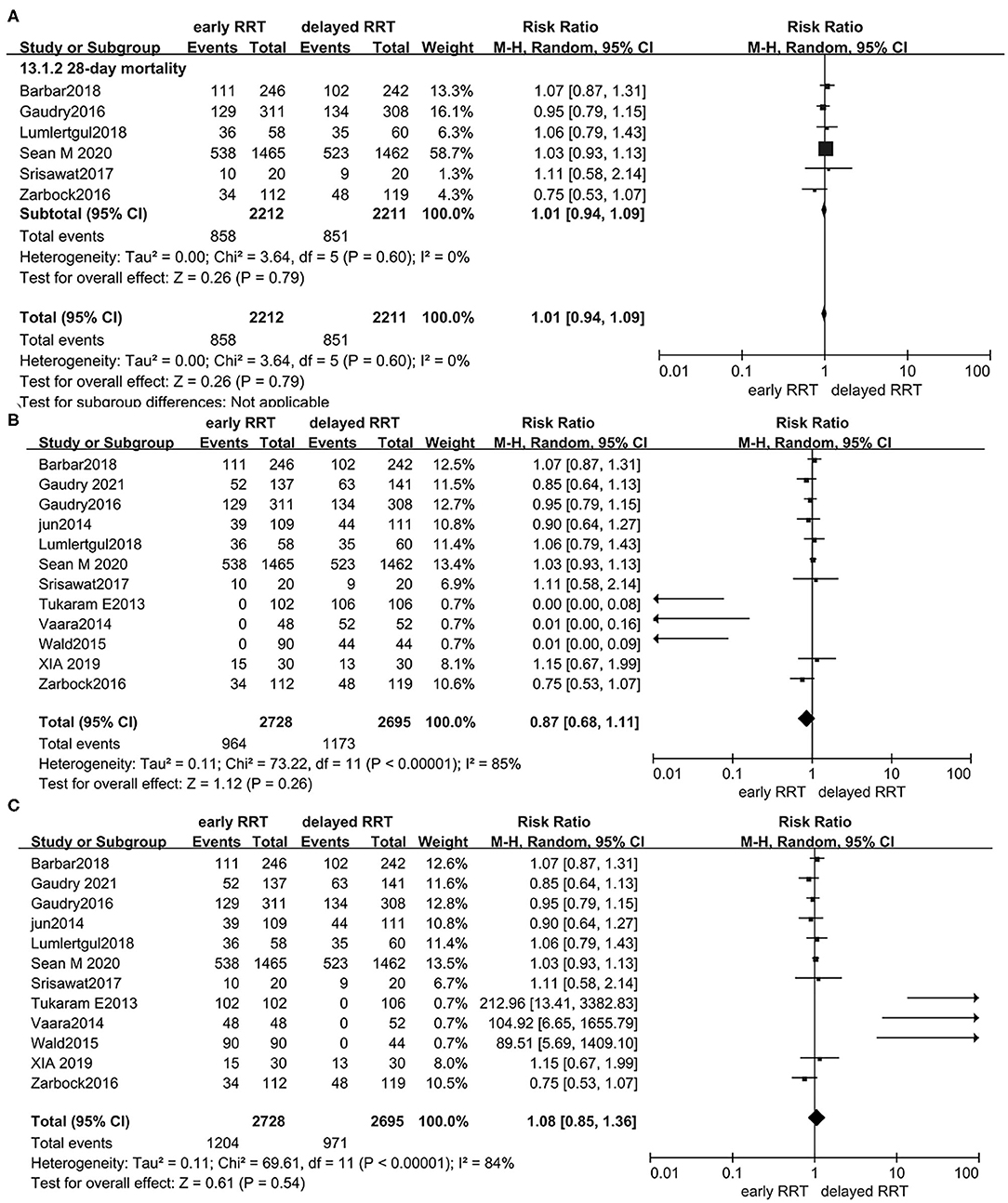
Figure 5. Forest plot for all-cause mortality at day 28 of sensitivity analysis by removing individual trials at a time with non-low bias in each domain (A); best-worst-case scenario random-effects meta-analysis for the rate of all-cause mortality at day 28 (B); and worst-best-case scenario random-effects meta-analysis for the rate of all-cause mortality at day 28 (C).
Secondary Outcomes
All-Cause Mortality (at Days 60 and 90, in ICU and in Hospital)
Definitions for all-cause mortality included all-cause mortality at day 60, day 90, in an ICU, and in a hospital. The conventional meta-analysis showed that all mortality outcomes did not differ between eRRT and dRRT (Supplementary Digital Content—Supplementary Figures S1A–D), TSA for all-cause mortality at day 60 showed that the cumulative Z-curve touched neither a traditional boundary nor trial sequential monitoring boundaries, indicating that further studies with larger sample size are needed to confirm this result. TSA for all-cause mortality at day 90, in an ICU, and in a hospital were consistent with those reported in mortality at day 28 (Supplementary Digital Content—Supplementary Figures S2A–D).
The Rate of RRT
The rate of RRT was reported in 10 trials, including 5,069 participants and 4,064 events (6, 12, 13, 15, 16, 30, 33–36). The result demonstrated that the rate of RRT significantly increased in eRRT (RR, 1.50, 95% CI: 1.28–1.76, p < 0.00001, I2 = 96%) (Supplementary Digital Content—Supplementary Figure S3A). TSA showed that the cumulative Z-curve touched the traditional boundary, but not for the sequential monitoring boundary, suggesting that further studies with a larger sample size are needed to rule out possible false positives (Supplementary Digital Content—Supplementary Figure S3B).
Ventilator-Free Days, Vasoactive Agent-Free Days, and the Rate of RRT Dependence at Day 28
There was also no significant difference in ventilator-free and vasoactive agents-free days between the two groups at day 28 (Supplementary Digital Content—Supplementary Figures S4A,B). About 7 of 12 trials (6, 12, 13, 16, 30, 34, 36) reported the rate of RRT dependence at day 28. The pooled analysis showed that the risk of RRT dependence at day 28 was not significantly different between the two groups (RR, 0.80, 95% CI: 0.50–1.29, p = 0.36, I2 = 58%) (Supplementary Digital Content—Supplementary Figure S5A), and the cumulative Z-curve did not touch a traditional boundary or trial sequential monitoring boundaries, indicating that further studies with a larger sample size are needed to confirm the result (Supplementary Digital Content—Supplementary Figure S5B).
The LOS of Survivors in ICU and Hospital
The meta-analysis from five of the included articles (6, 12, 15, 30, 33) showed that the LOS of survivors in an ICU (MD: −1.55 days; 95% CI: −3.08 to −0.02, p = 0.05, I2 =29%) (Supplementary Digital Content—Supplementary Figure S6A) and the LOS of survivors in a hospital were significantly decreased in eRRT (MD: −2.74 days; 95% CI: −4.93 to −0.55, p = 0.01, I2=14%) (Supplementary Digital Content—Supplementary Figure S6B).
Adverse Events
Sean et al. (15) and Wald et al. (33) reported the total adverse events during the first 14 days, but the analysis was only relied on Sean's study because of Wald's too small trial. Sean et al. (15) reported that the adverse events included an association with renal-replacement therapy (hypotension, arrhythmia, seizure, bleeding, allergic reaction, decreased phosphate, decreased potassium, and decreased ionized calcium) and an association with the use of a dialysis catheter (pneumothorax or hemothorax, bleeding, thrombus, arterial puncture). The total adverse events of Wald et al. (33) include arrhythmia, hypotension, hemorrhage, hypocalcemia, ischemic bowel, and sepsis. Adverse events were significantly increased in eRRT (RR, 1.41, 95%CI: 1.22–1.63, p < 0.00001. Heterogeneity not applied) (Supplementary Digital Content—Supplementary Figure S7A), and it was confirmed by TSA (Supplementary Digital Content—Supplementary Figure S7B). However, the risk of bleeding, arrhythmia, and hypotension had no difference between the two groups (Supplementary Digital Content—Supplementary Figures S7C–E).
Discussion
Conflicting results about the effect of eRRT vs. dRRT on patients with AKI exited for a long time, resulting in the optimal timing of RRT, which has been undecidable (37–39). Given the lack of quality evidence, more recently, two high-quality RCTs were published in NEJM (15) and Lancet (16), which compared the effects of eRRT vs. dRRT on critically ill patients with AKI. So, we did an updated systematic review with TSA.
In our meta-analysis, we found that there was no evidence to support the beneficial effects of eRRT on all-cause mortality at days 28 and 90, as well as in a hospital and in an ICU. These results were further confirmed by a sensitivity analysis or the TSA. Our result was consistent with some previous analyses (40, 41). In the analysis by Fayad et al. (42), the proportion of patients who died on day 28 did not significantly differ between the two groups. And, the other meta-analyses (40, 43) had the same results as did in Fayad et al. (42). However, some other meta-analyses have drawn the opposite conclusion. Zou et al. (44) did a meta-analysis, which included 15 studies with 1,479 patients and found that eRRT decreased 28-day mortality, especially when it was started within 24 h after cardiac surgery in patients with AKI. Wang et al. (45) selected 51 studies (including 10 RCTs) with a total of 8,179 patients with AKI showed that patients receiving eRRT had a 25% reduction in all-cause mortality compared to those receiving dRRT. These inconsistent results could be explained by several factors as follows. First, the sample sizes enrolled were quite large in the meta-analysis by Zou et al. and Wang et al., but a significant heterogeneity of the included studies could not be ignored, which may affect the robustness of the results. Second, study by Zou et al. was conducted in patients with AKI who had undergone cardiac surgery only. As we all know that most patients undergoing cardiac surgery were associated with cardiac insufficiency, and RRT can reduce generalized congestion and decrease cardiac load resulting in proving cardiac dysfunction.
These inconsistencies added further uncertainty about the efficacy of eRRT in critically ill patients with AKI. In a traditional meta-analysis, the positive results were easy to be changed with more evidence being accumulated (17, 46). Therefore, we performed TSA, of which the results were able to verify our traditional meta-analysis results: all-cause mortality at days 28 and 90, as well as in a hospital and in an ICU, and most importantly, to say no more studies were needed. While TSA for all-cause mortality at day 60 indicated that further studies with a larger sample size are needed to confirm this result, this may be related to the small sample size. In this respect, our analytic results of all-cause mortality at day 28, day 90, in a hospital, and in an ICU should be considered as convincing.
During our analysis, low degrees of heterogeneity were found in all-cause mortality at day 28 (I2 = 0%, p = 0.69). As we all know, in addition to statistical heterogeneity, it also includes clinical heterogeneity and methodological heterogeneity. Therefore, we conducted subgroup analyses based on study design, patient population, the modality of RRT, and the presence of sepsis at randomization, and found no substantial difference between the groups regarding mortality at day 28. Sensitivity analyses also showed that missing data did not change the primary outcome. However, the definitions of eRRT and dRRT in each included study were different, which prevents us from performing relevant heterogeneity analysis. In addition, we could not evaluate all possible causes of heterogeneity, which may affect the accuracy of our results.
In our analysis, an indicator of safety—the risk of total adverse events increased in eRRT and TSA also confirmed the result, but there was no significant difference in the risk of bleeding, arrhythmia, hypotension, and hyperkalemia. The TSA result required further studies are needed to confirm these results. The inconsistency may be due to the inclusion of different studies for the analysis of these two results. Therefore, it is expected that the same studies are used simultaneously for these two indicators.
Our meta-analysis has the following strengths: (1) We prespecified our analytical plan and registered the study protocol with INPLASY to ensure the methodological quality of the review. (2) We selected RCTs published only in the last 10 years and included the latest published high-quality RCTs (15, 16). (3) Considering clinical heterogeneity especially due to differences in the definition of eRRT and dRRT, we performed a sensitivity analysis with the “best-worst-case” scenario and “worst-best-case” scenario to assess the possible impact of missing data on the robustness of results and performed subgroup analyses to explore eventual heterogeneity. (4) We also conducted TSA to ascertain the trend of uncertain outcomes and provide “futility boundaries” that can draw invalid conclusions as soon as possible, preventing researchers from spending a lot of resources on unneeded research.
There were several limitations in our analysis. First, the heterogeneity of studies, particularly the definition of eRRT and dRRT was quite various and might affect the accuracy of our results. Second, there is an inability to assess all possible causes of heterogeneity, the decision regarding the optimal timing of RRT lack of objective basis. Third, the total adverse events and other specific adverse events showed contradictory results, the inconsistency possibly due to the inclusion of different studies for analysis.
Conclusion
Early RRT strategy provided no significant survival benefit for ill patients with AKI compared with the dRRT strategy, and TSA indicated that no more studies were needed to confirm it.
Data Availability Statement
All data generated or analyzed during this study are included in this published article (and its Supplementary Material), further inquiries can be directed to the corresponding author
Author Contributions
CX contributed to the study concept and design, drafting of the manuscript. CX, JX, and YC contributed to the acquisition, analysis, and interpretation of data, and statistical analysis. QL, WL, TH, SL, and DG helped in data arrangement. FS contributed to study concept, supervision, and organizing the final manuscript. All authors contributed to the article and approved the submitted version.
Conflict of Interest
The authors declare that the research was conducted in the absence of any commercial or financial relationships that could be construed as a potential conflict of interest.
Publisher's Note
All claims expressed in this article are solely those of the authors and do not necessarily represent those of their affiliated organizations, or those of the publisher, the editors and the reviewers. Any product that may be evaluated in this article, or claim that may be made by its manufacturer, is not guaranteed or endorsed by the publisher.
Acknowledgments
We thank Ph.D. Wei Qiang, the librarian of Guizhou Medical University for her help with the search strategies. We thank the associate editor and the reviewers for their useful feedback to improve this paper.
Supplementary Material
The Supplementary Material for this article can be found online at: https://www.frontiersin.org/articles/10.3389/fmed.2022.820624/full#supplementary-material
Supplementary Figure S1. Forest plot for all-cause mortality at day 60. The size of each square represents the proportion of information provided by each study (A); forest plot for all-cause mortality at day 90 (B); forest plot for all-cause mortality in an intensive care unit (ICU) (C); and forest plot for all-cause mortality in a hospital (D).
Supplementary Figure S2. Trial sequential analysis (TSA) for all-cause mortality at day 60 (A); TSA for all-cause mortality at day 90 (B); TSA for all-cause mortality in an ICU (C); and TSA for all-cause mortality in a hospital (D).
Supplementary Figure S3. Forest plot for the rate of renal replacement therapy (RRT) during the study period (A) and TSA for the rate of RRT during the study period (B).
Supplementary Figure S4. Forest plot for ventilator-free days at day 28 (A) and forest plot for vasoactive agent-free days at day 28 (B).
Supplementary Figure S5. Forest plot for the rate of RRT dependence at day 28 (A) and TSA for the rate of RRT dependence at day 28 (B).
Supplementary Figure S6. Forest plot for the length of stay (LOS) of survivors in ICU (A) and forest plot for the LOS of the survivors in a hospital (B).
Supplementary Figure S7. Forest plot for the rate of total adverse events (A); TSA for the rate of total adverse events (B); forest plot for the rate of bleeding (C); forest plot for the rate of arrhythmia (D); and forest plot for the rate of hypotension (E).
Abbreviations
eRRT, early renal replacement therapy; AKI, acute kidney injury; TSA, trial sequential analysis; RCTs, randomized controlled trials; dRRT, delayed renal replacement therapy; RR, risk ratio; CI, confidence interval; GRADE, Grading of Recommendations, Assessment, Development and Evaluations; ICU, intensive care unit; RRT, renal replacement therapy; LOS, the length of stay; RRR, relative risk reduction; SOFA, Sequential Organ Failure Assessment; APACHE II, Acute Physiology and Chronic Health Evaluation; FST, furosemide stress test; pNGAL, plasma neutrophil gelatinase associated lipocalin.
References
1. Hoste EA, Bagshaw SM, Bellomo R, Cely CM, Colman R, Cruz DN, et al. Epidemiology of acute kidney injury in critically ill patients: the multinational AKI-EPI study. Intensive Care Med. (2015) 41:1411–23. doi: 10.1007/s00134-015-3934-7
2. Kellum JA, Lameire N. Diagnosis, evaluation, and management of acute kidney injury: a KDIGO summary (Part 1). Critical Care. (2013) 17:204. doi: 10.1186/cc11454
3. Shiao C-C, Huang T-M, Spapen HD, Honore PM, Wu V-C. Optimal timing of renal replacement therapy initiation in acute kidney injury: the elephant felt by the blindmen? Critical Care. (2017) 21:146. doi: 10.1186/s13054-017-1713-2
4. Zarbock A, Mehta RL. Timing of kidney replacement therapy in acute kidney injury. Clin J Am Soc Nephrol. (2019) 14:147–9. doi: 10.2215/CJN.08810718
5. Wald R, Bagshaw SM. The timing of renal replacement therapy initiation in acute kidney injury. Semin Nephrol. (2016) 36:78–84. doi: 10.1016/j.semnephrol.2016.01.009
6. Zarbock A, Kellum JA, Schmidt C, Van Aken H, Wempe C, Pavenstädt H, et al. Effect of early vs delayed initiation of renal replacement therapy on mortality in critically Ill patients with acute kidney injury: the ELAIN randomized clinical trial. JAMA. (2016) 315:2190–9. doi: 10.1001/jama.2016.5828
7. Vanmassenhove J, Vanholder R, Van Biesen W, Lameire N. Haste makes waste-Should current guideline recommendations for initiation of renal replacement therapy for acute kidney injury be changed? Semin Dial. (2018) 31:204–8. doi: 10.1111/sdi.12693
8. Lin WT, Lai CC, Chang SP, Wang JJ. Effects of early dialysis on the outcomes of critically ill patients with acute kidney injury: a systematic review and meta-analysis of randomized controlled trials. Sci Rep. (2019) 9:18283. doi: 10.1038/s41598-019-54777-9
9. Komaru Y, Doi K. Early or delayed initiation of renal replacement therapy for critically ill patients-do we know the right time? J Thoracic Dis. (2016) 8:E1006–E9. doi: 10.21037/jtd.2016.08.22
10. Karakala N, Tolwani AJ. Timing of renal replacement therapy for acute kidney injury. J Intensive Care Med. (2019) 34:94–103. doi: 10.1177/0885066618774257
11. Schiffl H. Timing of renal replacement therapy in acute kidney injury: shedding new light on an old controversy. Indian J Crit Care Med. (2018) 22:730–2. doi: 10.4103/ijccm.IJCCM_295_18
12. Barbar SD, Clere-Jehl R, Bourredjem A, Hernu R, Montini F, Bruyère R, et al. Timing of renal-replacement therapy in patients with acute kidney injury and sepsis. N Engl J Med. (2018) 379:1431–42. doi: 10.1056/NEJMoa1803213
13. Lumlertgul N, Peerapornratana S, Trakarnvanich T, Pongsittisak W, Surasit K, Chuasuwan A, et al. Early versus standard initiation of renal replacement therapy in furosemide stress test non-responsive acute kidney injury patients (the FST trial). Critical Care. (2018) 22:101. doi: 10.1186/s13054-018-2021-1
14. Yang XM, Tu GW, Gao J, Wang CS, Zhu DM, Shen B, et al. A comparison of preemptive versus standard renal replacement therapy for acute kidney injury after cardiac surgery. J Surg Res. (2016) 204:205–12. doi: 10.1016/j.jss.2016.04.073
15. Bagshaw SM, Wald R, Adhikari NKJ, Bellomo R, da Costa BR, Dreyfuss D, et al. Timing of initiation of renal-replacement therapy in acute kidney injury. N Engl J Med. (2020) 383:240–51. doi: 10.1056/NEJMoa2000741
16. Stéphane Gaudry DH, Martin-Lefevre L, Lebbah S, Louis G. Comparison of two delayed strategies for renal replacement therapy initiation for severe acute kidney injury (AKIKI 2): a multicentre, open-label, randomised, controlled trial. Lancet. (2021) 397:1293–300. doi: 10.1016/S0140-6736(21)00350-0
17. Ioannidis JPA, Lau J. Evolution of treatment effects over time: Empirical insight from recursive cumulative metaanalyses. Proc Natl Acad Sci USA. (2001) 98:831–6. doi: 10.1073/pnas.98.3.831
18. Zhang S, Tang Q, Wu W, Yuan B, Lu C, Xia Y, et al. Association between DAZL polymorphisms and susceptibility to male infertility: systematic review with meta-analysis and trial sequential analysis. Sci Rep. (2014) 4:4642. doi: 10.1038/srep04642
19. Wetterslev J, Jakobsen JC, Gluud C. Trial Sequential Analysis in systematic reviews with meta-analysis. BMC Med Res Methodol. (2017) 17:39. doi: 10.1186/s12874-017-0315-7
20. Stewart LA, Clarke M, Rovers M, Riley RD, Simmonds M, Stewart G, et al. Preferred Reporting Items for Systematic Review and Meta-Analyses of individual participant data: the PRISMA-IPD Statement. JAMA. (2015) 313:1657–65. doi: 10.1001/jama.2015.3656
22. Higgins JPT TJ, Chandler J. Cochrane Handbook for Systematic Reviews of Interventions version 6.1. Cochrane (2020). Available from cochrane.org/handbook. Cochrane Database of Systematic Reviews. doi: 10.1002/9781119536604 (updated September 2020).
23. Sterne JAC, Savovic J, Page MJ, Elbers RG, Blencowe NS, Boutron I, et al. RoB 2: a revised tool for assessing risk of bias in randomised trials. BMJ. (2019) 366:l4898. doi: 10.1136/bmj.l4898
24. Gordon H. Guyatt ADo, Gunn e Vist, Regina Kunz. GrADE: an emerging consensus on rating quality of evidence and strength of recommendations. Br Med J. (2008) 336:924–6. doi: 10.1136/bmj.39489.470347.AD
25. Jakobsen JC WJ, Winkel P. Thresholds for statistical and clinical significance in systematic reviews with meta-analytic methods. BMC Med Res Methodol. (2014) 14:120. doi: 10.1186/1471-2288-14-120
26. Bjelakovic G, Nikolova D, Gluud C. Meta-regression analyses, meta-analyses, and trial sequential analyses of the effects of supplementation with beta-carotene, vitamin A, and vitamin E singly or in different combinations on all-cause mortality: do we have evidence for lack of harm? PLoS ONE. (2013) 8:e74558. doi: 10.1371/journal.pone.0074558
27. Copenhagen Trial Unit, User manual for Trial Sequential Analysis.0.9. Beta. Copenhagen: Copenhagen Trial Unit (2017).
28. Brok J, Thorlund K, Gluud C, Wetterslev J. Trial sequential analysis reveals insufficient information size and potentially false positive results in many meta-analyses. J Clin Epidemiol. (2008) 61:763–9. doi: 10.1016/j.jclinepi.2007.10.007
29. Thorlund K, Devereaux PJ, Wetterslev J, Guyatt G, Ioannidis JP, Thabane L, et al. Can trial sequential monitoring boundaries reduce spurious inferences from meta-analyses? Int J Epidemiol. (2009) 38:276–86. doi: 10.1093/ije/dyn179
30. Gaudry S, Hajage D, Schortgen F, Martin-Lefevre L, Pons B, Boulet E, et al. Initiation strategies for renal-replacement therapy in the intensive care unit. N Engl J Med. (2016) 375:122–33. doi: 10.1056/NEJMoa1603017
31. Jun M, Bellomo R, Cass A, Gallagher M, Lo S, Lee J. Timing of renal replacement therapy and patient outcomes in the randomized evaluation of normal versus augmented level of replacement therapy study. Crit Care Med. (2014) 42:1756–65. doi: 10.1097/CCM.0000000000000343
32. Vaara ST, Reinikainen M, Wald R, Bagshaw SM, Pettilä V. Timing of RRT based on the presence of conventional indications. Clin J Am Soc Nephrol. (2014) 9:1577–85. doi: 10.2215/CJN.12691213
33. Wald R, Adhikari NK, Smith OM, Weir MA, Pope K, Cohen A, et al. Comparison of standard and accelerated initiation of renal replacement therapy in acute kidney injury. Kidney Int. (2015) 88:897–904. doi: 10.1038/ki.2015.184
34. Srisawata N, Laoveeravat P, Limphunudom P, Lumlertgul N. The effect of early renal replacement therapy guided by plasma neutrophil gelatinase associated lipocalin on outcome of acute kidney injury: a feasibility study. J Critical Care. (2018) 43:36–41. doi: 10.1016/j.jcrc.2017.08.029
35. Jamale TE, Hase NK, Kulkarni M, Pradeep KJ, Keskar V, Jawale S, et al. Earlier-start versus usual-start dialysis in patients with community-acquired acute kidney injury: a randomized controlled trial. Am J Kidney Dis. (2013) 62:1116–21. doi: 10.1053/j.ajkd.2013.06.012
36. Yan-mei XIA H-pS, Wei-dong WU, Xiu-zhe WANG. Effect of urinary NGAL on the timing of renal replacement therapy in patients with acute renal injury associated with sepsis. Med J Chin People's Liber Army. (2019) 44:605–10.
37. Bagshaw SM, Wald R. Strategies for the optimal timing to start renal replacement therapy in critically ill patients with acute kidney injury. Kidney Int. (2017) 91:1022–32. doi: 10.1016/j.kint.2016.09.053
38. Kidney Disease Improving Global Outcome. KDIGO Clinical Practice Guideline for Acute Kidney Injury. (2012) Available at: http://kdigo.org/home/guidelines/acute-kidney-injury/~
39. Prowle JR, Davenport A. Does early-start renal replacement therapy improve outcomes for patients with acute kidney injury? Kidney Int. (2015) 88:670–3. doi: 10.1038/ki.2015.225
40. Pasin L, Boraso S, Tiberio I. Early initiation of renal replacement therapy in critically ill patients: a meta-analysis of randomized clinical trials. BMC Anesthesiol. (2019) 19:62. doi: 10.1186/s12871-019-0733-7
41. Andonovic M, Shemilt R, Sim M, Traynor JP, Shaw M, Mark PB, et al. Timing of renal replacement therapy for patients with acute kidney injury: A systematic review and meta-analysis. J Intens Care Soc. (2021) 22:67–77. doi: 10.1177/1751143720901688
42. Fayad AII, Buamscha DG, Ciapponi A. Timing of renal replacement therapy initiation for acute kidney injury. Cochr Database Syst Rev. (2018) 12:CD010612. doi: 10.1002/14651858.CD010612.pub2
43. Xiaoming Li CL, Mao Z, Li Q, Zhou F. Timing of renal replacement therapy initiation for acute kidney injury in critically ill patients: a systematic review of randomized clinical trials with meta-analysis and trial sequential analysis. Critical Care. (2021) 25.
44. Zou H, Hong Q, Xu G. Early versus late initiation of renal replacement therapy impacts mortality in patients with acute kidney injury post cardiac surgery: a meta-analysis. Critical Care. (2017) 21:150. doi: 10.1186/s13054-017-1707-0
45. Wang C, Lv LS, Huang H, Guan J, Ye Z, Li S, et al. Initiation time of renal replacement therapy on patients with acute kidney injury: a systematic review and meta-analysis of 8179 participants. Nephrology. (2017) 22:7–18. doi: 10.1111/nep.12890
Keywords: mortality, early renal replacement therapy, delayed renal replacement therapy, acute kidney injury, trial sequential analysis (TSA)
Citation: Xiao C, Xiao J, Cheng Y, Li Q, Li W, He T, Li S, Gao D and Shen F (2022) The Efficacy and Safety of Early Renal Replacement Therapy in Critically Ill Patients With Acute Kidney Injury: A Meta-Analysis With Trial Sequential Analysis of Randomized Controlled Trials. Front. Med. 9:820624. doi: 10.3389/fmed.2022.820624
Received: 23 November 2021; Accepted: 11 January 2022;
Published: 21 February 2022.
Edited by:
Nattachai Srisawat, Chulalongkorn University, ThailandReviewed by:
Wisit Kaewput, Phramongkutklao Hospital, ThailandKonlawij Trongtrakul, Chiang Mai University, Thailand
Copyright © 2022 Xiao, Xiao, Cheng, Li, Li, He, Li, Gao and Shen. This is an open-access article distributed under the terms of the Creative Commons Attribution License (CC BY). The use, distribution or reproduction in other forums is permitted, provided the original author(s) and the copyright owner(s) are credited and that the original publication in this journal is cited, in accordance with accepted academic practice. No use, distribution or reproduction is permitted which does not comply with these terms.
*Correspondence: Feng Shen, doctorshenfeng@163.com
†These authors have contributed equally to this work and share first authorship