- 1VA Informatics and Computing Infrastructure, VA Salt Lake City Health Care System, Salt Lake City, UT, United States
- 2Division of Epidemiology, Department of Internal Medicine, University of Utah School of Medicine, Salt Lake City, UT, United States
- 3VA Palo Alto Healthcare System, Palo Alto, CA, United States
- 4Department of Medicine, Stanford University School of Medicine, Palo Alto, CA, United States
- 5VA New York Harbor Healthcare System, New York, NY, United States
- 6Department of Medicine, Perlmutter Cancer Center, New York University Langone Health, New York, NY, United States
- 7Department of Urology, Perlmutter Cancer Center, New York University Langone Health, New York, NY, United States
- 8Cooperative Studies Program Clinical Epidemiology Research Center (CSP CERC), VA Connecticut Healthcare System, West Haven, CT, United States
- 9Department of Internal Medicine, Yale School of Medicine, Yale University, New Haven, CT, United States
- 10Department of Biomedical Data Science, Stanford University School of Medicine, Palo Alto, CA, United States
- 11Center of Excellence for Stress and Mental Health (CESAMH), VA San Diego Healthcare System, San Diego, CA, United States
- 12Department of Psychiatry, Center for Behavior Genetics of Aging, School of Medicine, University of California, San Diego, La Jolla, CA, United States
- 13VA Greater Los Angeles Healthcare System, Los Angeles, CA, United States
- 14School of Medicine, University of California, Los Angeles, Los Angeles, CA, United States
Background: The incidence and severity of coronavirus disease 19 (COVID-19) is substantially higher in men. Sex hormones may be a potential mechanism for differences in COVID-19 outcome in men and women. We hypothesized that men treated with androgen deprivation therapy (ADT) have lower incidence and severity of COVID-19.
Methods: We conducted an observational study of male Veterans treated in the Veterans Health Administration from February 15th to July 15th, 2020. We developed a propensity score model to predict the likelihood to undergo Severe Acute Respiratory Syndrome Coronavirus 2 (SARS-CoV-2) testing. We performed multivariable logistic regression modeling adjusted with inverse probability weighting to examine the relationship between ADT and COVID-19 incidence. We conducted logistic regression analysis among COVID-19 patients to test the association between ADT and COVID-19 severity.
Results: We identified a large cohort of 246,087 VA male patients who had been tested for SARS-CoV-2, of whom 3,057 men were exposed to ADT, and 36,096 men with cancer without ADT. Of these, 295 ADT patients and 2,427 cancer patients not on ADT had severe COVID-19 illness. In the primary, propensity-weighted comparison of ADT patients to cancer patients not on ADT, ADT was associated with decreased likelihood of testing positive for SARS-CoV-2 (adjusted OR, 0.88 [95% CI, 0.81–0.95]; p = 0.001). Furthermore, ADT was associated with fewer severe COVID-19 outcomes (OR 0.72 [95% CI 0.53–0.96]; p = 0.03).
Conclusion: ADT is associated with reduced incidence and severity of COVID-19 amongst male Veterans. Testosterone and androgen receptor signaling may confer increased risk for SARS-CoV-2 infection and contribute to severe COVID-19 pathophysiology in men.
Introduction
Severe acute respiratory syndrome coronavirus 2 (SARS-CoV-2) infection and coronavirus disease 2019 (COVID-19) have rapidly disseminated across the globe, accelerated with the delta variant surge, and caused more than 4.6 million deaths (1). The incidence and severity of COVID-19 have been related to multiple factors, including age, comorbid conditions, immunosuppression, smoking, race, and sex (2–4). Whereas men and women manifest a similar incidence of COVID-19, male sex is a risk factor for more severe illness. The severity of COVID-19 as indicated by admission to an intensive care unit (ICU), requirement for mechanical ventilation, or mortality is approximately twofold higher in men (5, 6). This sex disparity has been observed in Asia, North America, Europe, and across individual countries on each of the continents.
Explanations for sex differences in outcome include difference in health behaviors (e.g., smoking history), incidence of comorbidities such as lung and cardiovascular disease, genetics, and biology, including variations in sex hormones. Specifically, androgens including testosterone and dihydrotestosterone may worsen COVID-19 severity through two non-mutually exclusive physiologic effects. First, androgens are known to suppress both innate and adaptive immunity, which may increase susceptibility to the SARS-CoV-2 virus and disease severity (7, 8). Second, androgens induce the expression of the two plasma membrane proteins, transmembrane protease serine 2 (TMPRSS2) via an androgen response element in the promoter and angiotensin converting enzyme 2 (ACE2), which are required for entry of SARS-CoV-2 into epithelial cells (9–12). Co-expression of the androgen receptor (AR), TMPRSS2, and ACE2 has been observed in human pulmonary epithelial cells, alveolar type 2 pneumocytes, and nasal mucosal cells which are critical sites for SARS-CoV-2 infection and disease severity (12, 13). Androgen-driven overexpression of these viral co-receptors may augment cellular entry and replication of SARS-CoV-2, thereby mediating increased infection rate and COVID-19 severity in men. Furthermore, men who are carriers of TMPRSS2 gene mutations, which potentiate androgen-induced cellular expression of TMPRSS2, may be especially at risk for COVID-19 morbidity and mortality (14, 15). TMPRSS2 gene variants has also been found to confer a twofold increase in H1N1 influenza severity supporting the hypothesis that androgen regulation of TMPRSS2 may be a critical host factor for respiratory disease (16).
These observations suggest that suppression of androgen levels may have a salutary impact on COVID-19 illness. Two retrospective studies that evaluated the impact of androgen deprivation therapy (ADT) on COVID-19 illness yielded conflicting results. A study of 4,532 men in the Veneto region of Italy reported a reduced incidence of COVID-19 among patients with prostate cancer on ADT, whereas a study of patients in the United States (U.S.) failed to detect a protective effect of ADT on SARS-CoV-2 infection, but did not have sufficient statistical power to assess the effect of ADT on COVID-19 severity (17, 18). There have also been three additional investigations but with small sample sizes with one report finding that hospitalization with severe COVID-19 was significantly less in prostate cancer patients treated with ADT compared to those without ADT while two other reports observed no differences in severe COVID-19 between ADT and non-ADT groups (19–21). Our objective was to perform a nationwide, population-based study of the relationship of ADT to the incidence and outcomes of COVID-19. Using data from the U.S. Veterans Health Administration (VHA), we report the largest study to date evaluating the hypothesis that ADT is associated with a reduced incidence and severity of COVID-19.
Materials and Methods
Data Sources
We used the Corporate Data Warehouse (CDW) of the VHA, a national data repository that provides access to the electronic health records of all individuals who received care in the VHA. In addition, we drew from the VA COVID-19 Shared Data Resource (CSDR), a newly developed data domain that consists of a wide range of information related to COVID-19 available for all patients who received a COVID-19 laboratory test within VHA or whose positive test result outside VHA was recorded in VHA clinical notes (22). This study was approved by the VA Central Institutional Review Board.
Study Sample
The study sample consisted of all male Veterans who were alive as of February 15, 2020. The earliest testing date reported in the CSDR is February 16, 2020. Therefore, we considered anyone alive as of February 15, 2020 as having been eligible to be tested for SARS-CoV-2. From this base sample, we assembled two sub-samples to examine incidence of COVID-19 and severity of COVID-19. For incidence analysis, we derived a sample consisting of patients who underwent SARS-CoV-2 testing matched to patients who did not undergo SARS-CoV-2 testing on age, race, and VHA facility. Separately, we used an unmatched sample of patients who tested positive for SARS-CoV-2 to investigate ADT use and COVID-19 severity. The index date for the incidence analysis and the severity analysis was February 15, 2020 and the date of first positive SARS-CoV-2 test result, respectively.
Exposure
Exposure to ADT was defined as having any prescription for a luteinizing hormone releasing hormone analog (LHRH) (buserelin, degarelix, goserelin, historelin, leuprolide, or triptorelin) or an antiandrogen (abiraterone, apalutamide, bicalutamide, cyproterone, darolutamide, enzalutamide, flutamide, ketoconazole, or nilutamide) in the 6 months prior to the index date. A small number of patients were prescribed only antiandrogens. These patients were presumed to be receiving their LHRH analogs outside the VHA because it is highly unlikely to be prescribed antiandrogens as a monotherapy.
Endpoints
We investigated the relationship between ADT use and COVID-19 incidence and severity. To assess incidence, we used a binary variable indicating any positive reverse transcriptase polymerase chain reaction (RT-PCR) SARS-CoV-2 test result through July 15, 2020. To measure severity, we constructed a binary variable indicating whether a patient was admitted to the intensive care unit (ICU), placed on mechanical ventilation, or dead in the 60 days following a positive test up to July 15, 2020. Death was attributed to COVID-19 if it occurred within 60 days of a positive RT-PCR test for SARS-CoV-2. The primary comparison for both incidence and severity was patients with ADT exposure (ADT patients) vs. patients with non-prostate cancer who had no ADT exposure (non-ADT, other cancer patients). We selected this as the primary comparison because the incidence and severity of COVID-19 is adversely affected by a cancer diagnosis and virtually all ADT patients have high risk, recurrent, locally advanced, or metastatic prostate cancer. Secondary comparisons included all patients exposed to ADT vs. all who were not, and patients with prostate cancer with vs. without ADT exposure.
Covariates
Age, race, marital status, body mass index, smoking status, the Charlson Comorbidity Index categories (23) (excluding localized and metastatic solid tumors) in the prior 2 years, use of selected antihypertensives—angiotensin-converting enzyme inhibitors (ACEs), angiotensin II receptor blockers (ARBs), and spironolactone—in the prior 6 months, VHA utilization in the prior year as measured by total numbers of visits and hospital days, and the number of days to testing or first SARS-CoV-2 positivity were assessed at the index date. Lastly, the VHA facility of COVID-19 testing was included as a fixed effect in the COVID-19 incidence models and as a random effect in the COVID-19 severity models.
Statistical Analysis
Multivariate logistic regression models were used to determine the association of ADT exposure with SARS-CoV-2 positivity, weighted by the inverse of the predicted probabilities of being tested. Due to the limited availability of SARS-CoV-2 tests, receipt of test was prioritized based on a wide range of patient characteristics including demographics, comorbid conditions, and severity of symptoms. Such targeted testing results in a highly selected group of patients who are tested for SARS-CoV-2 (24). To adjust for unequal probabilities of being tested, we used the inverse probability weighting method, where the weight is based on the predicted probabilities (propensity score) of being tested, estimated by a logistic regression model using ADT exposure, selected patient covariates, and VHA facility (25, 26). For this model, we implemented a nested case-control design with incidence density sampling to match each tested patient (case) to five patients who were eligible to be tested (controls) at the time of the case’s testing on age, race, and VHA facility (27).
Generalized linear mixed models were used to determine associations between ADT exposure and severity of COVID-19 while controlling for patients’ demographic and clinical covariates, and the number of days from February 15 to the date of first SARS-CoV-2 positivity (as fixed effects) and VHA facility (as a random effect). All male Veterans in the study sample with a positive COVID-19 test were included in this analysis. We used SAS 9.2 (Cary, NC) for data preparation and all statistical analyses. Two-tailed hypothesis testing was performed using the significance level of 5%.
Results
Patient Characteristics
We identified 246,087 male patients tested for SARS-CoV-2 through July 15, 2020 (Table 1). Among the 246,087 tested patients, 3,057 patients were prescribed ADT and 243,030 were not. Compared to non-ADT patients, ADT patients were more likely to be Black (ADT 37%, no ADT 24%), older (mean age 73.9 vs. 62.9; p < 0.001), and had more comorbidities (Charlson Comorbidity Index ≥ 5 in 72% of ADT patients and 28% of non-ADT patients).
Severe Acute Respiratory Syndrome Coronavirus 2 Positivity
The strategy for selecting patients to be included in the SARS-CoV-2 testing propensity score model is shown in Figure 1, and the baseline characteristics of all SARS-CoV-2-tested patients (cases) and the matched controls are shown in Supplementary Table 1. ADT patients were more likely to be tested for SARS-CoV-2 (adjusted odds ratio (OR), 1.59 [95% confidence interval (CI), 1.52–1.68]; Supplementary Table 2). In the primary, propensity-weighted comparison of ADT vs. cancer patients not on ADT, ADT was associated with decreased likelihood of testing positive for SARS-CoV-2 after adjusting for the covariates listed in Table 1 (OR, 0.88 [95% CI, 0.81–0.95]; p = 0.001; Table 2). Similar results were observed in secondary comparisons of ADT patients vs. all non-ADT patients (OR, 0.75 [95% CI, 0.70–0.81]; p < 0.001; Table 2) and patients with prostate cancer on ADT vs. patients with prostate cancer not on ADT (OR, 0.85 [95% CI, 0.77–0.94]; p = 0.002; Supplementary Table 3).
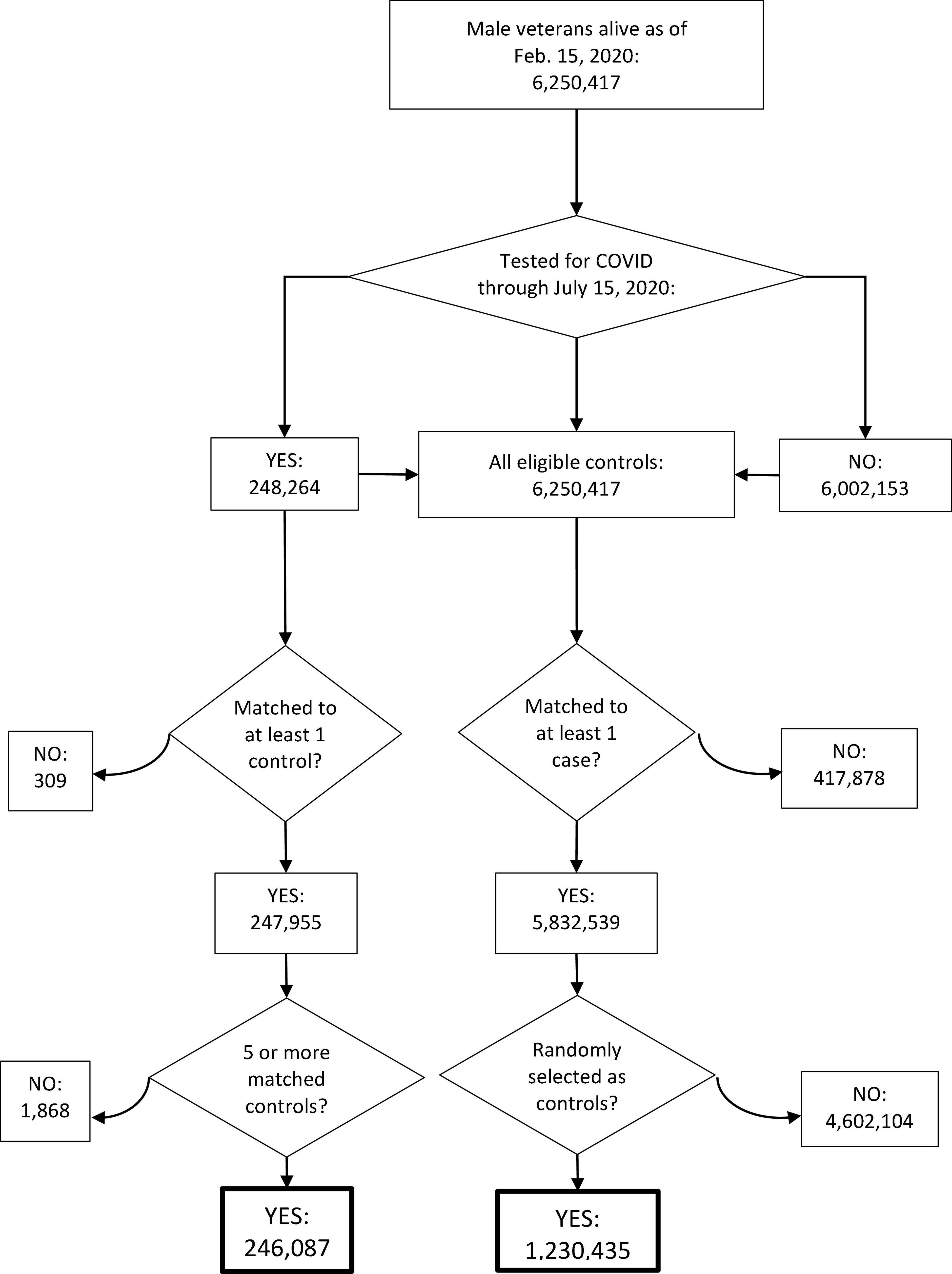
Figure 1. Consort diagram for the selection of patients for the model for the propensity to be tested for SARS-CoV-2. Curved arrows indicate excluded patients.
Coronavirus Disease 19 Severity
Our primary comparison of COVID-19 severity included 295 ADT patients with COVID-19 (including 189 with prostate cancer only and 97 with prostate cancer and a second cancer diagnosis), and 2,427 non-ADT, other cancer patients with COVID-19. Significant differences between the two groups included age, race, smoking status, and comorbidities (Table 3). Severe COVID-19 outcomes were observed in 76/295 (25%) of the ADT group and 727/2427 (30%) of the non-ADT group (Table 4). After controlling for clinical and demographic factors, ADT was associated with fewer severe COVID-19 outcomes (OR 0.72 [95% CI 0.53–0.96]; p = 0.03; Table 4). Comparisons between ADT patients vs. all patients not on ADT and prostate cancer patients on ADT vs. prostate cancer patients not on ADT did not demonstrate a statistically significant protective effect of ADT (Table 4 and Supplementary Table 3).
Discussion
In our analysis of male U.S. Veterans tested for SARS-CoV-2, ADT use was associated with a reduced incidence and severity of COVID-19 compared to Veterans with non-prostate cancers who were not on ADT. The reduction in the incidence of COVID-19 was modest (OR 0.88) after correcting for baseline characteristics (e.g., smoking, cardiovascular disease and other comorbidities, and race) and the likelihood for undergoing SARS-CoV-2 testing. A reduced incidence of COVID-19 was also observed among ADT patients compared to all SARS-CoV-2-tested Veterans not on ADT as well as Veterans with prostate cancer not on ADT.
The effects of ADT on the incidence of COVID-19 has been reported in two studies. Montopoli et al. (17) evaluated SARS-CoV-2 positive patients in the Veneto region of Italy and reported that COVID-19 infection was significantly higher in patients with a cancer diagnosis other than prostate cancer as well as patients with prostate cancer not on ADT compared to prostate cancer patients treated with ADT (OR 4.86 [95% CI 1.88–12.56]; p = 0.001, and OR 4.05 [95% CI 1.55–10.59]; p = 0.004, respectively). These results were based on COVID-19 incidence among all patients with cancer in the Veneto region, irrespective of whether these patients had been tested for SARS-CoV-2. Whereas the results from the Italian study are consistent with the protective effect of ADT on COVID-19 incidence in the analogous populations in the Veteran population, the magnitude of the effect was far greater in the Veneto population. In contrast, Klein et al. (18), reporting on a cohort of patients from Cleveland Clinic healthcare system, observed no protective effect of ADT on incidence of COVID-19 in prostate cancer patients on ADT vs. prostate cancer patients not on ADT.
The association of ADT with the severity of COVID-19 has been reported in three studies. In the Italian study, ADT was associated with a reduced likelihood of severe disease (ICU admission and death), but this was premised on only four COVID-19 positive patients on ADT (only 1 of whom developed severe disease), 13 COVID-19 positive patients with prostate cancer not on ADT, and 89 COVID-19 positive patients with other cancers not on ADT (17). By contrast, the Ohio study did not identify a protective effect of ADT on the severity of COVID-19 outcomes, but the authors recognized that robust statistics were not feasible due to limited sample size, despite being significantly larger than the Italian cohort (18). Based on a small cohort of ADT and non-ADT patients (n = 22 and 36, respectively), Patel et al. (19) reported that ADT was associated with a reduced risk of hospitalization (OR 0.23 [95% CI 0.06–0.79]; p < 0.02) and supplemental oxygen use (OR 0.26 [95% CI 0.07–0.92]; p = 0.04), and a non-statistically significant trend toward a reduction in mechanical ventilation and death after correcting for age, cardiac disease, and pulmonary disease (19). Two other reports with small sample sizes did not observe a reduction in COVID-19 severity with ADT (20, 21). By comparison, our study demonstrated ADT exposure was associated with a reduction in COVID-19 severity (OR 0.72) compared to cancer patients without ADT exposure, based on a composite endpoint of ICU admission, mechanical ventilation, and death.
Strengths of this study include a multivariate regression analysis to account for differences in baseline characteristics, which was not performed in the Italian study, as well as a much larger cohort of patients in comparison to the Italian study, though still an order of magnitude smaller than the current VHA cohort (e.g., n = 304 and n = 3,057 patients on ADT in the Ohio and Veteran cohorts, respectively). Importantly, neither the Italian nor the Ohio study accounted for differences in the likelihood to be tested for SARS-CoV-2 among the respective populations, which can bias the COVID-19 incidence results, especially if ADT patients are more likely to be tested for SARS-CoV-2. Indeed, in our Veteran population, the adjusted odds ratio for SARS-CoV-2 testing was 1.59 for ADT patients, which we incorporated as a propensity score in our analyses of COVID-19 incidence. In addition, our study is less subject to ascertainment bias, since Veterans largely seek care within the VA healthcare system. Weaknesses include the retrospective nature of the analysis and uncertainty about the underlying cause for ICU admission, mechanical ventilation, and death. While we treated VHA facility as a random effect in our severity analysis, the rapid change in treatment decisions and ability for hospitals to handle capacity at different times in the pandemic may leave confounding for patients who develop severe disease.
The role of androgens in SARS-CoV-2 cell entry and replication and in the clinical course and outcome of COVID-19 is likely to be complicated. Testosterone and dihydrotestosterone (DHT) are regulators of ACE-2, the membrane receptor binding SARS-CoV-2, and TMPRSS2, which promotes cellular entry of the virus following proteolytic processing of its spike protein (9–12). Downregulation of the expression and function of ACE-2 and TMPRSS2 in response to androgen deprivation may contribute to our data showing that ADT decreases COVID-19 infection and severity.
Androgen regulation of cellular immunity, however, may be a more important mechanism mediating our observation of ADT-induced reduction in COVID-19 infection and improvement of COVID-19 outcome. In general, androgens are immunosuppressive dampening many aspects of adaptive humoral and cell-mediated immune responses to pathogens including viruses (7, 8). Testosterone and DHT bind to androgen receptors on T lymphocytes, impair T lymphocyte activation, and inhibit Th1 differentiation and interferon-gamma (IFN-g) generation (28). Early T cell activation and interferon response are critical during the initial phase of COVID-19 infection for rapid clearance of SARS-CoV-2. Women have been found to rapidly activate T lymphocytes and increase interferon levels at the onset of COVID-19 infection, which has been shown to result in a good outcome (29). In contrast, men with low T lymphocyte activation and insufficient early interferon response impairs SARS-CoV-2 clearance and results in severe COVID-19 (29). Normal function of the JAK kinase TYK2 expressed in T lymphocytes has a critical role in T cell differentiation and type I interferon signaling (30). Testosterone and DHT bind to the promoter of the Ptnpn1 and increase the expression and function of this phosphatase (31). Ptpn1 then dephosphorylates the TYK2 protein thereby impeding TYK2-mediated T helper-1 (Th1) differentiation and interferon signaling essential for innate and acquired immune responses to viruses. Recently, a TYK2 missense mutation has been reported to confer high risk for COVID-19 severity (32). Consequently, androgen suppression of TYK2 signaling in T lymphocytes may be an important determinant of COVID-19 outcome. These findings suggest that ADT may lessen COVID-19 severity by abrogating androgen immunosuppression and promoting T cell differentiation and interferon signaling. In addition to research on androgens and cellular immunity, our hypothesis is also supported by clinical studies showing that ADT improves the response to immunotherapy of prostate cancer by counteracting androgen immunosuppression.
Alternatively, the susceptibility to COVID-19 infection and severity in men may be mediated by the interaction of androgens with the renin-angiotensin-aldosterone system (RAAS). The canonical RAAS pathway includes two angiotensin converting enzymes: (a) ACE that converts angiotensin I to octapeptide angiotensin II that binds to the Gq-coupled AT1a receptor; (b) ACE2, encoded by a gene located on the X chromosome at Xp22, that cleaves a single hydrophobic amino acid, Phe, from the C-terminus of angiotensin II to form angiotensin-(1–7), which binds to the Mas receptor, another G protein-coupled receptor in the RAAS system (33, 34). The actions of RAAS are homeostatically controlled by the AT1a receptor being counterregulated by the Mas receptor. Angiotensin II activates AT1a receptor signaling to drive pro-inflammatory pathways, promote abnormal ventricular remodeling and cardiac fibrosis, and trigger vasoconstriction, sympathetic activation, and hypertension (33, 34). In opposition, angiotensin-(1–7) activates vasoprotective Mas receptor signaling that exerts anti-inflammatory, anti-fibrotic, and anti-apoptotic actions in the heart (33, 34). Importantly, RAAS also regulates the pulmonary system. ACE, angiotensin II, and the AT1a receptor induce pro-inflammatory and fibrotic pathology in the lung, while ACE2, angiotensin-(1–7), and the Mas receptor protect the lung from inflammatory-induced injury and fibrosis during severe infection from pathogens including SARS-CoV-2 (35). Androgens testosterone and dihydrotestosterone have been reported to upregulate expression of angiotensinogen, renin, ACE, and angiotensin AT1 receptors (36, 37), which would produce a RAAS imbalance and may contribute to COVD-19 severity in men, an effect that would be ameliorated by ADT. Estradiol, however, upregulates expression of ACE-2, facilitates angiotensin-(1–7) formation and Mas receptor signaling, and downregulates angiotensin AT1a receptor expression, which may account for more favorable COVID-19 outcome in women (36–39).
An important caveat is that ADT may only be beneficial in the early phase of COVID-19 when T cell activation and other immune responses are critical (40, 41). Low testosterone levels during the late stages of COVID-19 infection have been associated with greater disease severity possibly due to the higher risk for cytokine storms without androgen immunosuppression (42). Genetics may also influence the effects of androgens on COVID-19 outcome. A trinucleotide (CAG) repeat sequence on exon 1 of the androgen receptor gene can modulate the function of the androgen receptor (AR) and serve as a genetic marker of androgen sensitivity. Shorter CAG repeat length increases AR sensitivity to testosterone and DHT, higher AR transcriptional activity, and a more active androgen receptor, while longer CAG repeat length reduces AR sensitivity and activity. Recently, androgen receptor CAG repeat length has been found to predict COVID-19 severity indicating the importance of genetically determined AR sensitivity in the mediation of androgen impact on COVID-19 outcome in men (43, 44). Other androgen genetics may also mediate COVID-19 infection and severity. Genome-wide association studies have identified genes strongly associated with testosterone levels. The VA Million Veteran Program MVP035 COVID-19 Disease Mechanisms study has constructed a testosterone polygenic risk score from GWAS data (45) and is now determining the role of testosterone gene variants in the COVID-19 severity. Androgen mechanisms contributing to COVID-19 will continue to require further investigation to elucidate their complexity.
Data Availability Statement
The data analyzed in this study are subject to the following licenses/restrictions: Data restrictions apply as outlined in the Rules of Behavior for Access and Use of Data from VHA Vital Status File. The data are not publicly available. Further inquiries can be directed to the corresponding author.
Ethics Statement
The studies involving human participants were reviewed and approved by the VA Central Institutional Review Board. Written informed consent for participation was not required for this study in accordance with the national legislation and the institutional requirements.
Author Contributions
MR, KL, JAL, JL, M-CS, DB, SL, and DM contributed to conception and design of the study. KL, AG, and KH prepared the data and performed statistical analysis. MR wrote the first draft of the manuscript. KL, DB, SL, and RH wrote sections of the manuscript. All authors contributed to manuscript revision, read, and approved the submitted version.
Funding
Funding for this study was provided by the US Department of Veterans Affairs (VA), Veterans Health Administration, Cooperative Studies Program, grant number 825-MS-DI-33848, and used resources and facilities at the VA Informatics and Computing Infrastructure (VINCI), VA HSR RES 13-457. MR was supported by the Prostate Cancer Foundation (PCF) David Geffen Precision Oncology Center of Excellence of VAGLAHS and a Department of Veterans Affairs Merit Review Award. DB and DM were supported by the John and Daria Barry Precision Oncology Center of Excellence of the VANYHHS and Edward Blank and Sharon Cosloy–Blank Family Foundation. DB was supported by the VA Career Development Award (CDA 16-206). DM was a Prostate Cancer Foundation Young Investigator Awardee. RH was supported by the Million Veteran Program MVP022 award (I01 CX001727), VISN-22 VA Center of Excellence for Stress and Mental Health, and National Institute of Aging RO1 grants AG050595 (The VETSA Longitudinal Twin Study of Cognition and Aging VETSA 4) and AG05064 (Effects of Androgen Deprivation Therapy on Preclinical Symptoms of Alzheimer’s Disease).
Author Disclaimer
The views expressed are those of the authors and do not necessarily represent the views or policy of the Department of Veterans Affairs or the United States Government.
Conflict of Interest
SL reports equity in Gilead Sciences, Inc. SD reports research grants from the following for-profit organizations outside this submitted work: Alnylam Pharmaceuticals Inc., AbbVie Inc., Astellas Pharma Inc., AstraZeneca Pharmaceuticals LP, Biodesix, Inc., Boehringer Ingelheim International GmbH, Celgene Corporation, Eli Lilly and Company, Genentech Inc., Gilead Sciences Inc., GlaxoSmithKline PLC, Innocrin Pharmaceuticals Inc., Janssen Pharmaceuticals, Inc., Kantar Health, Myriad Genetic Laboratories, Inc., Novartis International AG, and Parexel International Corporation through the University of Utah or Western Institute for Veteran Research.
The remaining authors declare that the research was conducted in the absence of any commercial or financial relationships that could be construed as a potential conflict of interest.
Publisher’s Note
All claims expressed in this article are solely those of the authors and do not necessarily represent those of their affiliated organizations, or those of the publisher, the editors and the reviewers. Any product that may be evaluated in this article, or claim that may be made by its manufacturer, is not guaranteed or endorsed by the publisher.
Supplementary Material
The Supplementary Material for this article can be found online at: https://www.frontiersin.org/articles/10.3389/fmed.2022.774773/full#supplementary-material
References
1. World Health Organization [WHO]. WHO Coronavirus Disease (COVID-19) Dashboard. (2020). Available online at: https://covid19.who.int/ (accessed December 11, 2020).
2. Richardson S, Hirsch JS, Narasimhan M, Crawford JM, McGinn T, Davidson KW, et al. Presenting characteristics, comorbidities, and outcomes among 5700 patients hospitalized with COVID-19 in the New York city area. JAMA. (2020) 323:2052–9. doi: 10.1001/jama.2020.6775
3. Kopel J, Perisetti A, Roghani A, Aziz M, Gajendran M, Goyal H. Racial and gender-based differences in COVID-19. Front Public Heal. (2020) 8:418. doi: 10.3389/fpubh.2020.00418
4. Hussain A, Mahawar K, Xia Z, Yang W, El-Hasani S. Obesity and mortality of COVID-19. Meta-analysis. Obes Res Clin Pract. (2020) 14:295–300. doi: 10.1016/j.orcp.2020.07.002
5. Wenham C, Smith J, Morgan R. COVID-19: the gendered impacts of the outbreak. Lancet. (2020) 395:846–8. doi: 10.1016/S0140-6736(20)30526-2
6. Gebhard C, Regitz-Zagrosek V, Neuhauser HK, Morgan R, Klein SL. Impact of sex and gender on COVID-19 outcomes in Europe. Biol Sex Differ. (2020) 11:29. doi: 10.1186/s13293-020-00304-9
7. Gubbels Bupp MR, Jorgensen TN. Androgen-induced immunosuppression. Front Immunol. (2018) 9:794. doi: 10.3389/fimmu.2018.00794
8. Trigunaite A, Dimo J, Jørgensen TN. Suppressive effects of androgens on the immune system. Cell Immunol. (2015) 294:87–94. doi: 10.1016/j.cellimm.2015.02.004
9. Wiersinga WJ, Rhodes A, Cheng AC, Peacock SJ, Prescott HC. Pathophysiology, transmission, diagnosis, and treatment of coronavirus disease 2019 (COVID-19): a review. JAMA. (2020) 324:782–93. doi: 10.1001/jama.2020.12839
10. Vaduganathan M, Vardeny O, Michel T, McMurray JJV, Pfeffer MA, Solomon SD. Renin-Angiotensin-aldosterone system inhibitors in patients with Covid-19. N Engl J Med. (2020) 382:1653–9. doi: 10.1056/NEJMsr2005760
11. Heurich A, Hofmann-Winkler H, Gierer S, Liepold T, Jahn O, Pöhlmann S. TMPRSS2 and ADAM17 cleave ACE2 differentially and only proteolysis by TMPRSS2 augments entry driven by the severe acute respiratory syndrome coronavirus spike protein. J Virol. (2014) 88:1293–307. doi: 10.1128/JVI.02202-13
12. Hoffmann M, Kleine-Weber H, Schroeder S, Krüger N, Herrler T, Erichsen S, et al. SARS-CoV-2 cell entry depends on ACE2 and TMPRSS2 and is blocked by a clinically proven protease inhibitor. Cell. (2020) 181:271–80.e8. doi: 10.1016/j.cell.2020.02.052
13. Stopsack KH, Mucci LA, Antonarakis ES, Nelson PS, Kantoff PW. TMPRSS2 and COVID-19: serendipity or opportunity for intervention? Cancer Discov. (2020) 10:779–82. doi: 10.1158/2159-8290.CD-20-0451
14. Irham LM, Chou W-H, Calkins MJ, Adikusuma W, Hsieh S-L, Chang W-C. Genetic variants that influence SARS-CoV-2 receptor TMPRSS2 expression among population cohorts from multiple continents. Biochem Biophys Res Commun. (2020) 529:263–9. doi: 10.1016/j.bbrc.2020.05.179
15. Thunders M, Delahunt B. Gene of the month: TMPRSS2 (transmembrane serine protease 2). J Clin Pathol. (2020) 73:773–6. doi: 10.1136/jclinpath-2020-206987
16. Cheng Z, Zhou J, To KK-W, Chu H, Li C, Wang D, et al. Identification of TMPRSS2 as a susceptibility gene for severe 2009 pandemic A(H1N1) influenza and A(H7N9) influenza. J Infect Dis. (2015) 212:1214–21. doi: 10.1093/infdis/jiv246
17. Montopoli M, Zumerle S, Vettor R, Rugge M, Zorzi M, Catapano CV, et al. Androgen-deprivation therapies for prostate cancer and risk of infection by SARS-CoV-2: a population-based study (N = 4532). Ann Oncol. (2020) 31:1040–5. doi: 10.1016/j.annonc.2020.04.479
18. Klein EA, Li J, Milinovich A, Schold JD, Sharifi N, Kattan MW, et al. Androgen deprivation therapy in men with prostate cancer does not affect risk of infection with SARS-CoV-2. J Urol. (2020) 205:441–3. doi: 10.1097/JU.0000000000001338
19. Patel VG, Zhong X, Liaw B, Tremblay D, Tsao CK, Galsky MD, et al. Does androgen deprivation therapy protect against severe complications from COVID-19? Ann Oncol. (2020) 31:1419–20. doi: 10.1016/j.annonc.2020.06.023
20. Koskinen M, Carpen O, Honkanen V, Seppänen MRJ, Miettinen PJ, Tuominen JA, et al. Androgen deprivation and SARS-CoV-2 in men with prostate cancer. Ann Oncol Off J Eur Soc Med Oncol. (2020) 31 1417–8. doi: 10.1016/j.annonc.2020.06.015
21. Kwon DH, Vashisht R, Borno HT, Aggarwal RR, Small EJ, Butte AJ, et al. Androgen-deprivation therapy and SARS-CoV-2 in men with prostate cancer: findings from the University of California Health System registry. Ann Oncol Off J Eur Soc Med Oncol. (2021) 32:678–9. doi: 10.1016/j.annonc.2021.01.067
22. Department of Veterans Affairs [DVA]. VA COVID-19: Shared Data Resource. (2020). https://www.hsrd.research.va.gov/for_researchers/cyber_seminars/archives/3810-notes.pdf (accessed November 3, 2020).
23. Quan H, Sundararajan V, Halfon P, Fong A, Burnand B, Luthi J-C, et al. Coding algorithms for defining comorbidities in ICD-9-CM and ICD-10 administrative data. Med Care. (2005) 43:1130–9. doi: 10.1097/01.mlr.0000182534.19832.83
24. Griffith GJ, Morris TT, Tudball MJ, Herbert A, Mancano G, Pike L, et al. Collider bias undermines our understanding of COVID-19 disease risk and severity. Nat Commun. (2020) 11:5749. doi: 10.1038/s41467-020-19478-2
25. Seaman SR, White IR. Review of inverse probability weighting for dealing with missing data. Stat Methods Med Res. (2013) 22:278–95. doi: 10.1177/0962280210395740
26. Desai RJ, Franklin JM. Alternative approaches for confounding adjustment in observational studies using weighting based on the propensity score: a primer for practitioners. BMJ-BRITISH Med J. (2019) 367:l5657. doi: 10.1136/bmj.l5657
27. Biesheuvel CJ, Vergouwe Y, Oudega R, Hoes AW, Grobbee DE, Moons KG. Advantages of the nested case-control design in diagnostic research. BMC Med Res Methodol. (2008) 8:48. doi: 10.1186/1471-2288-8-48
28. Brown MA, Su MA. An inconvenient variable: sex hormones and their impact on T cell responses. J Immunol. (2019) 202:1927–33. doi: 10.4049/jimmunol.1801403
29. Lucas C, Wong P, Klein J, Castro TBR, Silva J, Sundaram M, et al. Longitudinal analyses reveal immunological misfiring in severe COVID-19. Nature. (2020) 584:463–9. doi: 10.1038/s41586-020-2588-y
30. Majoros A, Platanitis E, Kernbauer-Hölzl E, Rosebrock F, Müller M, Decker T. Canonical and non-canonical aspects of JAK-STAT signaling: lessons from interferons for cytokine responses. Front Immunol. (2017) 8:29. doi: 10.3389/fimmu.2017.00029
31. Kissick HT, Sanda MG, Dunn LK, Pellegrini KL, On ST, Noel JK, et al. Androgens alter T-cell immunity by inhibiting T-helper 1 differentiation. Proc Natl Acad Sci U S A. (2014) 111:9887–92. doi: 10.1073/pnas.1402468111
32. Verma A, Tsao NL, Thomann L, Ho Y-L, Iyengar S, Luoh S-W, et al. A Phenome-Wide Association Study of genes associated with COVID-19 severity reveals shared genetics with complex diseases in the Million Veteran Program. medRxiv[Preprint]. (2021): doi: 10.1101/2021.05.18.21257396
33. Benigni A, Cassis P, Remuzzi G. Angiotensin II revisited: new roles in inflammation, immunology and aging. EMBO Mol Med. (2010) 2:247–57. doi: 10.1002/emmm.201000080
34. Forrester SJ, Booz GW, Sigmund CD, Coffman TM, Kawai T, Rizzo V, et al. Angiotensin II signal transduction: an update on mechanisms of physiology and pathophysiology. Physiol Rev. (2018) 98:1627–738. doi: 10.1152/physrev.00038.2017
35. Sharma RK, Stevens BR, Obukhov AG, Grant MB, Oudit GY, Li Q, et al. ACE2 (Angiotensin-Converting Enzyme 2) in cardiopulmonary diseases: ramifications for the control of SARS-CoV-2. Hypertension. (2020) 76:651–61. doi: 10.1161/HYPERTENSIONAHA.120.15595
36. Sullivan JC. Sex and the renin-angiotensin system: inequality between the sexes in response to RAS stimulation and inhibition. Am J Physiol Integr Comp Physiol. (2008) 294:R1220–6. doi: 10.1152/ajpregu.00864.2007
37. Viveiros A, Rasmuson J, Vu J, Mulvagh SL, Yip CYY, Norris CM, et al. Sex differences in COVID-19: candidate pathways, genetics of ACE2, and sex hormones. Am J Physiol Circ Physiol. (2021) 320:H296–304. doi: 10.1152/ajpheart.00755.2020
38. Gersh FL, O’Keefe JH, Lavie CJ, Henry BM. The renin-angiotensin-aldosterone system in postmenopausal women: the promise of hormone therapy. Mayo Clin Proc. (2021) 96:3130–41. doi: 10.1016/j.mayocp.2021.08.009
39. Wu Z, Maric C, Roesch DM, Zheng W, Verbalis JG, Sandberg K. Estrogen regulates adrenal angiotensin AT1 receptors by modulating AT1 receptor translation. Endocrinology. (2003) 144:3251–61. doi: 10.1210/en.2003-0015
40. Mauvais-Jarvis F. Do Anti-androgens have potential as therapeutics for COVID-19? Endocrinology. (2021) 162:bqab114. doi: 10.1210/endocr/bqab114
41. Salciccia S, Del Giudice F, Eisenberg ML, Mastroianni CM, Berardinis ED, Ricciuti GP, et al. Testosterone target therapy: focus on immune response, controversies and clinical implications in patients with COVID-19 infection. Ther Adv Endocrinol Metab. (2021) 12:20420188211010104. doi: 10.1177/20420188211010105
42. Dhindsa S, Zhang N, McPhaul MJ, Wu Z, Ghoshal AK, Erlich EC, et al. Association of circulating sex hormones with inflammation and disease severity in patients with COVID-19. JAMA Netw Open. (2021) 4:e2111398. doi: 10.1001/jamanetworkopen.2021.11398
43. Baldassarri M, Picchiotti N, Fava F, Fallerini C, Benetti E, Daga S, et al. Shorter androgen receptor polyQ alleles protect against life-threatening COVID-19 disease in European males. EBioMedicine. (2021) 65:103246. doi: 10.1016/j.ebiom.2021.103246
44. McCoy J, Wambier CG, Herrera S, Vaño-Galván S, Gioia F, Comeche B, et al. Androgen receptor genetic variant predicts COVID-19 disease severity: a prospective longitudinal study of hospitalized COVID-19 male patients. J Eur Acad Dermatol Venereol. (2021) 35:e15–7. doi: 10.1111/jdv.16956
45. Pagadala MS, Jasuja GK, Palnati M, Lynch J, Anglin T, Chang N, et al. Discovery of novel trans-ancestry and ancestry-specific gene loci for total testosterone in a multi-ancestral analysis of men in the million veteran program. medRxiv[Preprint]. (2022):2022.02.16.21265846. doi: 10.1101/2022.02.16.21265846
Keywords: androgen deprivation therapy, COVID-19 incidence, COVID-19 severity, prostate cancer, Veterans Health Administration
Citation: Lee KM, Heberer K, Gao A, Becker DJ, Loeb S, Makarov DV, Gulanski B, DuVall SL, Aslan M, Lee J, Shih M-C, Lynch JA, Hauger RL and Rettig M (2022) A Population-Level Analysis of the Protective Effects of Androgen Deprivation Therapy Against COVID-19 Disease Incidence and Severity. Front. Med. 9:774773. doi: 10.3389/fmed.2022.774773
Received: 13 September 2021; Accepted: 07 March 2022;
Published: 04 May 2022.
Edited by:
Alastair George Stewart, The University of Melbourne, AustraliaReviewed by:
Haicheng Tang, Fudan University, ChinaBrandon Henry, Cincinnati Children’s Hospital Medical Center, United States
Copyright © 2022 Lee, Heberer, Gao, Becker, Loeb, Makarov, Gulanski, DuVall, Aslan, Lee, Shih, Lynch, Hauger and Rettig. This is an open-access article distributed under the terms of the Creative Commons Attribution License (CC BY). The use, distribution or reproduction in other forums is permitted, provided the original author(s) and the copyright owner(s) are credited and that the original publication in this journal is cited, in accordance with accepted academic practice. No use, distribution or reproduction is permitted which does not comply with these terms.
*Correspondence: Richard L. Hauger, rhauger@health.ucsd.edu; Matthew Rettig, MRettig@mednet.ucla.edu
†These authors share senior authorship