- 1Renal Division, Hospital das Clinicas, University of São Paulo Medical School, São Paulo, Brazil
- 2Laboratory of Cellular, Genetic and Molecular Nephrology, University of São Paulo Medical School, São Paulo, Brazil
- 3Geriatric Division, Hospital das Clinicas, University of São Paulo Medical School, São Paulo, Brazil
In the last decades, improvements in the average life expectancy in the world population have been associated with a significant increase in the proportion of elderly people, in parallel with a higher prevalence of non-communicable diseases, such as hypertension and diabetes. As the kidney is a common target organ of a variety of diseases, an adequate evaluation of renal function in the approach of this population is of special relevance. It is also known that the kidneys undergo aging-related changes expressed by a decline in the glomerular filtration rate (GFR), reflecting the loss of kidney function, either by a natural senescence process associated with healthy aging or by the length of exposure to diseases with potential kidney damage. Accurate assessment of renal function in the older population is of particular importance to evaluate the degree of kidney function loss, enabling tailored therapeutic interventions. The present review addresses a relevant topic, which is the effects of aging on renal function. In order to do that, we analyze and discuss age-related structural and functional changes. The text also examines the different options for evaluating GFR, from the use of direct methods to the implementation of several estimating equations. Finally, this manuscript supports clinicians in the interpretation of GFR changes associated with age and the management of the older patients with decreased kidney function.
Introduction
The proportion of older people in the population is increasing worldwide, reflecting profound demographics changes overall. In 2020, there were 727 million elderly individuals aged 65 years or over, representing 9.3% of the world's population—a number expected to double by 2050 (1). As a result of the increase in life expectancy, non-communicable diseases such as diabetes and hypertension have become more prevalent, with a consequent increase in the prevalence of chronic kidney disease (CKD).
Over the years, the kidneys undergo aging-related changes expressed by a decline in the glomerular filtration rate (GFR), reflecting the loss of kidney function. Anatomical and functional changes become more evident in advanced ages, accounting for the GFR decrease. The loss of kidney function with advancing age indicates a physiological decline in GFR associated with healthy aging as a natural senescence process (2), a condition difficult to distinguish from the pathological decline in GFR related to kidney diseases. Half of adults over 70 years have a GFR, either measured or estimated, of <60 mL/min/1.73 m2 (3). However, it is still not clear whether a reduction in GFR is a consequence of the aging process or if it denotes a pathological process. The frequent comorbidities particularly present in the geriatric population that can affect kidney function, such as diabetes and cardiovascular diseases, amongst others, together with the increased prevalence of albuminuria in older subjects, and the recognition of glomerular sclerosis, tubular atrophy and vascular sclerosis in biopsy samples, represent further challenges to distinguish normal aging kidney function loss from pathological CKD. This is also important considering that CKD substantially increases the risk for cardiovascular and all-cause mortality, with low GFR correlating with worse outcome.
Since 2002, when the Kidney Disease Outcomes Quality Initiative (KDOQI) published a new chronic kidney disease (CKD) classification based on five categories of estimated glomerular filtration rate (eGFR), CKD has been defined as GFR <60 mL/min/1.73 m2 in the absence of kidney damage, as defined by structural of functional abnormalities (4). This threshold reflects a mean loss of 50% of kidney function in the healthy adult population. The KDIGO recommendations also define a GFR below 60 mL/min/1.73 m2 as a CKD, regardless of age (5). Although there is no precise definition of the normal range of GFR for older individuals, the use of this classification, with a fixed threshold for defining CKD based on eGFR values <60 mL/min/1.73 m2, does not consider the physiological GFR decline with aging and may result in an overdiagnosis of CKD in the elderly (6–8). In fact, an alternative age-adapted CKD definition, stratifying the GFR threshold according to age (75, 60, and 45 mL/min/1.73 m2 for ages younger than 40, 40–64, and 65 years or older, respectively) was proposed and analyzed in a large Canadian cohort, consisted of 127,132 individuals. Using a single, fixed eGFR threshold irrespective of age resulted in a 60% higher incidence of CKD. In this study, it is of note that 75% of individuals classified as CKD according to current fixed eGFR criteria <60 mL/min/1.73 m2 were 65 years or older and had an eGFR of 45–59 mL/min/1.73 m2 without significant albuminuria, raising the concern whether these individuals should be classified as CKD patients. The classification of older patients with age-related eGFR decline as having CKD is of crucial relevance, as it leads to an increase the risk of unnecessary interventions in much of this elderly population with additional implications for clinical practice and health policies (6–8).
Accurate assessment of renal function in the older population is of particular clinical significance for the diagnosis and classification of CKD, enabling tailored therapeutic interventions, and avoiding treatments of unnecessary and/or potential risk. GFR measurement can be carried out using conventional approaches through renal or plasma clearances of specific filtration markers, by measuring the marker concentration in plasma and urine or only in plasma, respectively (7). Alternatively, the use of eGFR is a more accessible approach for evaluating kidney function in a daily clinical practice. Several equations available have different performances characteristics concerning precision and accuracy and to avoid bias.
The present review is aimed at understanding the physiology of glomerular filtration in the elderly, highlighting the structural and functional changes associated with normal healthy aging leading to age related loss of kidney function. Also of interest is reviewing the different options to evaluate GFR in the elderly population to provide support to clinicians in the option and interpretation of the most appropriate method to either measure or estimate GFR.
Glomerular filtration changes in the aging kidney
The kidneys receive 20–25% of the cardiac output. Appropriate glomerular filtration occurs to excrete waste products of metabolism and to maintain internal homeostasis of water and electrolytes. The most important physiological function of renal hemodynamics is to keep the blood flow and pressure within the glomerular capillary at levels that provide adequate filtration rate with subsequent optimum tubular reabsorption and secretion functions (9). An adequate glomerular filtration rate (GFR) depends on the maintenance of the structural glomerular filtration barrier such as fenestrated endothelium, glomerular basement membrane, and podocyte and slit diaphragm besides endothelial glycocalyx and specialized glycocalyx between the foot processes and basement membrane (10).
GFR is the best parameter for measuring kidney function. GFR represents the volume of plasma filtered by functioning nephrons over a specified period. Among normal adult individuals, GFR ranges from 100 to 125 mL/min/1.73 m2 adjusted to demographic parameters such as gender, race and age (11–17). The GFR adjustment for race is a subject of recent discussion, as reported elsewhere (18–20).
With the natural aging process, all organs, including the kidneys, are progressively affected by the decline in biological functions and progressive structural changes, regardless of the presence of disease. Indeed, in healthy individuals, age-related GFR decline has long been recognized. The first report on the decline of GFR with age, published in 1950, describes a decrease in GFR, measured by inulin clearance, from 123 ml/min/1.73 m2 to 65 ml/min/1.73 m2 at the age of 90, decreasing about 8 mL/min/1.73 m2 per decade (21, 22). The Baltimore Longitudinal Study of Aging (BLSA) reported a decrease in creatinine clearance of 0.75 mL/min/year in normal subjects aged 30–90 years followed up for a period of 14 years (23). The overall decline in GFR with age, analyzed by inulin clearance, starts at 30–40 years of age and becomes more prominent in adults older than 70 years (15, 24, 25).
Although the decrease in GFR with aging has been well-recognized, the exact estimation of the magnitude of the renal function decline with healthy aging is not yet well-established. Different methodological strategies, the use of endogenous or exogenous filtration markers to measure GFR, the equation applied to estimate GFR, the demographic representation of the healthy participants' population and the follow-up period represent issues that might account for discrepancies. Besides large geographical cohort and meta-analysis studies (11), evaluation of normal GFR has also been carried out with living kidney donor healthy individuals (12, 26–28).
Studies with living kidney donors confirmed a decrease in GFR with age, although with different decline rates. In analyzing GFR measured with 51Cr-EDTA in 241 subjects 40–73 years of age, Grewal et al. found that GFR decreased by 0.91 mL/min/1.73 m2 per year (29). Poggio et al., measuring GFR with 125I-iothalamate clearances in 1,057 donors, found a decline of 1.49 ± 0.61 ml/min/1.73 m2 per decade (12), whereas Rule et al., in a cross-sectional study including 1,203 adult living kidney donors, showed that GFR declined at a rate of 6.3 mL/min/1.73 m2 per decade (2). Pottel et al. measured the GFR of 633 potential living kidney donors (by inulin, iohexol, or 51Cr-EDTA) and confirmed a progressive decline with the age of an average of −0.89 ml/min/1.73 m2 per year, corresponding to a Full Age Spectrum (FAS) equation prediction of an average decline rate of −0.92 ml/min/1.73 m2 per year (30, 31).
More recently, the Renal Iohexol Clearance Survey in Tromsø 6 (RENIS-T6), a robust study including 1,594 healthy individuals from the general population (age 50–62 years) followed for more than 5 years, showed a measured GFR decline rate of 0.95 ± 2.23 ml/min/1.73 m2 per year (32). A meta-analysis of iohexol clearance measurements in three European population-based cohorts of individuals aged 50–97 showed that the mean GFR was lower by −0.72 mL/min/1.73 m2 per year for healthy men and −1.03 ml/min/1.73 m2 per year for unhealthy men, and −0.92 mL/ min/1.73 m2 per year for healthy women and −1.22 ml/min/1.73 m2 per year for unhealthy women (33). Table 1 summarizes the demographic characteristics, age ranges of study population, the corresponding changes of GFR, and methods used to measure GFR in the different mentioned studies.
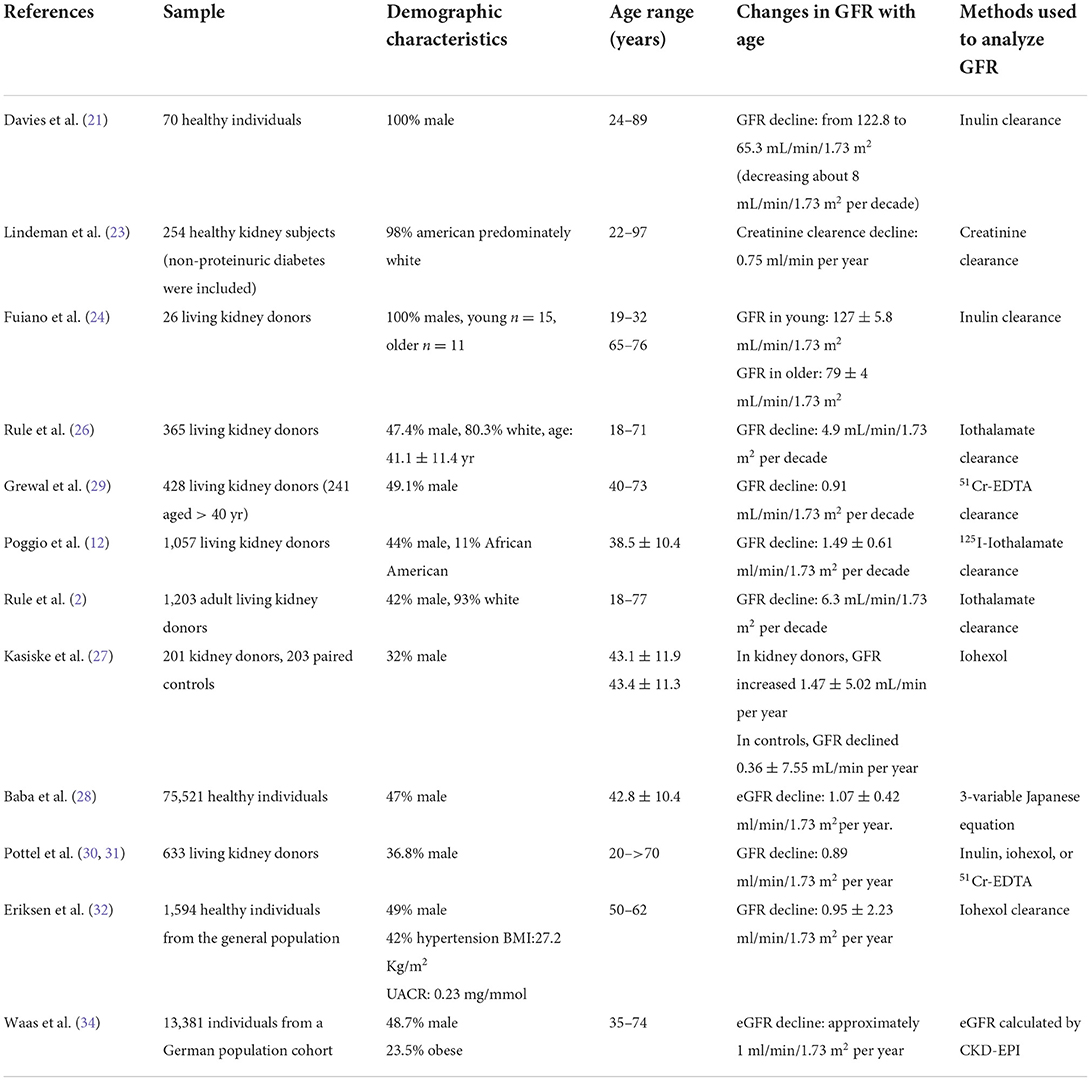
Table 1. Demographic characteristics, age ranges of study population, the corresponding changes of GFR, methods used to measure GFR.
In summary, a slow decline in GFR with age is expected in healthy individuals as shown in different geographical regions (11), with a median loss of eGFR per year of ~1 ml/min/1.73 m2 (34).
Structural and functional changes in the aging kidney
The age-related decline in GFR is considered a physiological process after 30–40 years of age, with a more significant decline after the 70 s. With normal aging, nephron loss occurs and is detectable to some extent by the age-related decrease in GFR. Senescence causes functional and structural changes in the kidneys. Not only does the GFR decline with aging but so does the renal plasma flow, the glomerular capillary plasma flow rate, and the glomerular capillary ultrafiltration coefficient (Kf) (35–37).
Structural changes
Structural changes are associated with the senescence kidney process. With aging, the number of nephrons decreases among healthy adults (38–41). Under the age of 40, sclerotic glomeruli comprise <5% of the total, increasing thereafter, reaching as much as 30% of the glomerular population by the eighth decade, which can result in a progressive loss of 20–25% renal mass, mainly in the cortical region, in more advanced ages (42).
The loss of nephrons likely reflects a progressive degree of glomerulosclerosis, which increases with normal aging, even in healthy aging (2, 43–47). The accurate detection of the nephron number, which can only be assessed through histological analysis of kidney biopsies, is limited due to the invasive nature of this procedure. In this setting, the available information has been provided by studies carried out in the healthy kidney transplant donor population by means of biopsies obtained during the transplant surgery, enabling the analysis of kidney histomorphometry in different aged individuals (41, 48). Kidney biopsies obtained from the latter showed that nephron loss was characterized by a reduction in the total number of glomeruli at the expense of sclerotic glomeruli and non-sclerotic glomeruli. Evaluation of kidney cortical volume in this healthy population by imaging with computer tomography scans also identified a decrease in cortical volume (41). It is of note that the loss of nephrons with aging seems to be closely correlated not with the number of sclerotic glomeruli but, rather, with the decreased number of non-sclerotic glomeruli (41). A possible explanation for this apparent inconsistent discordance between nephron loss with aging and a relatively low detection of glomerulosclerosis relies on the theoretical concept that globally sclerotic glomeruli can be fully reabsorbed or may undergo changes such as atrophy and obsolescence (38). Hence, they can no longer be easily detected on tissue sections examined by light microscopy (41). This is of relevance due to the fact that the percentage of glomerulosclerosis routinely seen in kidney biopsy reports from elderly patients may substantially undervalue the true age-related loss of glomeruli. The process has not been fully elucidated yet, and further studies are needed to explain the pathophysiology of age-related renal dysfunction.
Along with the structural and functional impairment changes, an age-related decline in GFR occurs with healthy aging (48–51). Progressive loss of filtering glomeruli due to glomerulosclerosis or vanished glomeruli affects the remaining preserved nephrons to undergo compensatory hypertrophy responses (52, 53), with a compensatory increase in the single-nephron GFR and glomerular capillary hydraulic pressure aiming to preserve global GFR (48). However, the enlarged glomeruli derived from compensatory hypertrophy induce an increased tension in the glomerular capillary walls, leading to hypertension, hyperfiltration, and damage to the remaining nephrons (54). Experimental studies have also shown a reduction in Kf in older rats as a result of glomerular capillary permeability and the surface area available for filtration, and confirmed an increase in glomerular capillary hydraulic pressure due to the decrease in the afferent arteriolar resistance (36).
Increased glomerular volume associated with compensatory hypertrophy can induce podocyte injury and loss. Podocytes are crucial cells for maintaining the normal glomerular architecture and capillary permeability but, due to their terminal differentiation nature, they have a limited capacity to undergo cell division, regeneration, and repair (55). The degenerative aging phenotype along with the structural and functional changes that occur in the aging process, promote a greater podocyte detachment rate, with a decrease in the density of podocytes per glomerulus and ultimately capillary wrinkling, tuff collapse and periglomerular fibrosis (56).
The pathophysiology of renal mass decrease may be related to several mechanisms, such as a decrease in klotho expression, an increase in telomere shortening, DNA instability, increased oxygen radicals, and overexpression of proteins that induce cell cycle arrest, with induction of p16 and p21 cell cycle inhibitors (57–59). Klotho, expressed predominantly in the kidney distal convoluted tubules, has been recognized as a key modulator of aging, with antiaging effects on several pathways. The klotho deficiency that occurs with the aging process accelerates aging-related diseases, including stroke, atherosclerosis, and osteoporosis. In contrast, overexpression of the gene encoding klotho extends lifespan in mice (60). In addition, the aging process increases the oxidant activity, decreases the autophagy, and enhances the capillary rarefaction contributing to the development of renal fibrosis (59) (Figure 1).
Besides glomerulosclerosis, other structural changes become more evident in the kidneys of elderly patients, including tubular atrophy, interstitial fibrosis, arteriolar hyalinosis, and atherosclerosis that increase with aging (2). One hypothesis is that fibrointimal hyperplasia of renal arterioles occurring with advanced age triggers glomerulosclerosis and consequent interstitial fibrosis and focal atrophy (61). Indeed, said age-related histological features are challenging to distinguish from disease-related renal pathological changes. The presence of comorbidities, abnormal urinary albumin excretion, and elevated blood pressure may weigh in favor of disease-related renal pathological changes. Finally, the number of renal cysts also increases with normal aging becoming very common in the kidney parenchyma (56).
Arterial hypertension, a high-sodium diet, the Western lifestyle, and its correlated comorbidities such as obesity and diabetes have already been associated with age-related loss of kidney function, although there is evidence that this process occurs despite the presence of these factors. Studies performed in patients without arterial hypertension or in diabetic patients showed similar declines in renal function rates (62, 63), suggesting not necessarily a pathological process.
Functional changes
Several studies indicate that renal hemodynamics is impaired with aging due primarily to a vascular process. The altered renovascular tone can be due to structural lesions in the renal vasculature, more evident in older patients with cardiovascular comorbidities, whilst pronounced renal vasoconstriction is also expressed in healthy normotensive elderly subjects, possibly due to functional abnormalities (24, 64, 65). With aging, there is compromised endothelium-dependent vasodilatation in the kidney characterized by a reduced vasodilatory response to stimuli such as acetylcholine, dopamine and nitric oxide, and greater sensitivity to vasoconstrictor stimuli, such as angiotensin, norepinephrine, and endothelin (24, 64, 66, 67). The tendency toward increased renal vasoconstriction due to a defect in nitric oxide-dependent response is also responsible for sodium retention (68) and the deregulation of the pressure-natriuresis response (69). In the elderly, the renin angiotensin system is suppressed. Although renin production and release are decreased with aging, leading to lower renin and aldosterone levels, the response to these hormones is exaggerated (36).
With the aging process, other changes in kidney function can be observed (Table 2). The ability to urinary concentration or dilution is impaired with advancing age and GFR deterioration. After fluid overload, older individuals have a decreased diluting capacity due possibly to a defect to generate free water. Older individuals also have a decreased ability for urinary concentration. Compared to younger individuals, older adults have about a 50% reduced capacity to conserve water and solutes under water deprivation. Reduced thirst response to osmotic changes, and changes in plasma osmolality, make elderly subjects more susceptible to developing disturbances in water homeostasis and volume depletion (42, 70). Decreased water-conserving capacity can have important clinical implications when older individuals do not have adequate access to water (70).
Renal regulation of sodium/potassium balance is also impaired in the elderly. Individuals older than 60 years submitted to sodium restriction have a lower capacity to reduce sodium excretion. On the other hand, older individuals submitted to sodium load do not properly excrete sodium, leading to sodium retention and fluid volume expansion (42). Potassium homeostasis is also altered in aging. Animal studies demonstrated that potassium excretion was less efficient in aging rats fed with a high-potassium diet (71). In clinical settings, medications that interfere with potassium excretion should be carefully evaluated due to the propensity of older patients to develop hyperkalemia.
Aging people are also prone to developing acid-base dysregulation. The age-related decline in the GFR reduces the capacity of the kidney to buffer metabolic changes and excrete the excess H+ load and ammonium (72).
In addition, calcium homeostasis could be affected by aging. Older individuals present impaired vitamin D production in the skin, impaired production of 1,25-(OH) 2D3, and an age-related decrease in the capacity of 1,25-dihydroxyvitamin D3 to promote intestinal absorption of calcium, resulting in increased PTH secretion and higher FGF23 levels (73).
How to evaluate and interpret GFR in the elderly
Several studies have addressed the discussion of the accuracy of GFR measurements in the elderly (15, 74–78). Conventional approaches for determining measured GFR use the principle of renal clearance of various substances, including endogenous filtration markers (creatinine or cystatin C) or exogenous markers (such as inulin, iohexol, iothalamate, or other radiolabeled compounds). These substances, excreted by the kidney, should ideally be exclusively excreted by glomerular filtration with no or minimal secretion by the tubules.
GFR measurement using endogenous filtration markers
The determination of inulin clearance performed under continuous intravenous infusion and urine collection is considered the most accurate, and still the gold standard method for GFR measurement, but it is cumbersome, expensive and not feasible in daily clinical practice (79). Alternatively, renal clearance can be performed using creatinine, an endogenous product freely filtered by the glomerulus, though also secreted by tubular cells. Thus, urinary creatinine clearance, a simple and accessible method, remains a common conventional method used for renal function evaluation. However, in the elderly population, besides the potential errors associated with inadequate urine sample collection, the use of creatinine as a marker for determining GFR in this age group, characterized by progressive loss of muscle mass, represents an additional drawback. Creatinine is a nitrogenous substance derived from muscle metabolism. Reduction in muscle mass is a common aging-related condition associated with decreased appetite, decreased protein intake, reduced physical activity, and sarcopenia, causing not only reduction of muscle strength but also the loss of muscle mass, reflected as lower serum creatinine levels, which impact the creatinine-based GFR estimation. Therefore, the use of serum creatinine as a marker for estimating GFR in the elderly results in an overestimation of the renal function (80).
A disadvantage of urinary clearances for measuring GFR, particularly in the frail elderly population, is the troublesome related to urine sample collection, which can lead to errors due mainly to urinary losses and incomplete urinary bladder emptying, causing sampling errors that might jeopardize the results. In this setting, the evaluation of plasma clearances for measuring GFR provides more precise results than urinary clearances (77).
Cystatin C is a more reliable endogenous marker for the evaluation of renal function compared to creatinine. Cystatin is a substance constitutively produced by all nucleated cells. As a low molecular weight protein, cystatin is freely filtered by kidneys and catabolized in the tubules, where all metabolites are reabsorbed. Cystatin C is not dependent on muscle conditions (81) but is influenced by factors such as smoking, obesity, and inflammation. These features make this substance a more accurate alternative for measuring glomerular filtration.
GFR measurement using exogenous filtration markers
Plasma clearances can also be performed using exogenous markers. The most widely used are the non-ionic contrast agent iohexol, particularly in Europe, and the iothalamate, more broadly used in the USA. Radiolabeled compounds such as 51Cr-EDTA, 99mTc-DTPA, and 125I-iothalamate have also been employed for GFR measurements with different availability in different centers. Determination of plasma clearances comprises intravenous injection of the compound and subsequent blood-sample drawings at different time-points to construct a plasma disappearance curve of the exogenous marker. Although methods based on exogenous markers likely provide higher accuracy in determining GFR, they are usually more expensive and not widely accessible in routine practice. While they have also been considered as gold standard for GFR determination in many studies, it is important to be aware that substances such as iothalamate are secreted by renal tubules and therefore, can overestimate GFR as compared to inulin clearances (79). Notwithstanding these considerations, plasma clearances are considered accurate methods of measuring GFR.
GFR estimation using equations
In clinical settings, an alternative to provide reliable and easy evaluation of kidney function in the elderly is the use of estimated GFR (eGFR) equations rather than direct measurement of GFR. eGFR based on equations is a simple and less expensive assessment, recommended by clinical guidelines from the National Kidney Foundation (NKF KDOQI) and Kidney Disease Improving Global Outcomes (KDIGO) (5, 82). Different formulas derived from large cohorts have been developed to estimate GFR in adults, based on serum creatinine or cystatin C (18, 83, 84).
The Cockcroft-Gault equation was the first equation developed for estimating creatinine clearance. In 1999, Levey and coworkers developed the first equation to estimate GFR, the Modification of Diet in Renal Disease (MDRD), normalized to 1.73 m2 body surface area (BSA) derived from measured iothalamate-measured GFR (83). In both equations, however, the geriatric population was underrepresented. In addition, these equations are based on serum creatinine, with the concerns for older patients already addressed. Both equations have been widely worldwide used, but they usually overestimate measured GFR in older patients (77, 78, 85), although Cockcroft-Gault adjusted for BSA yields a smaller bias (77). Of note, BSA-GFR is not a good normalization index in frail patients. In such a case, a BSA-GFR is usually overestimated, impairing the fair evaluation and clinical management.
The Chronic Kidney Disease-Epidemiology Collaboration equation (CKD-EPI) (18), also developed by Levey et al., is the most common currently used equation in routine clinical practice. Although CKD-EPI was developed to improve the estimation of GFR in older patients, participants older than 65 years of age were less represented in the CKD-EPI study population (only 13% of the whole population). Compared to iohexol plasma clearance, creatinine based CKD-EPI (CKD-EPICr) in older patients also overestimates GFR (77).
Equations based on serum cystatin C levels have proved their superiority over creatinine-based formulas in the elderly (86–88). A meta-analysis of 46 cross-sectional studies confirmed that the accuracy of cystatin C is superior to creatinine-based equations (eGFRCr) (34, 78, 89–91). Alternatively, equations based on the combination of cystatin C with creatinine (CKD-EPICr+Cys) have been developed, with even better precision and accuracy compared to equations based on creatinine alone or cystatin C alone (77, 92, 93). In fact, current guidelines, besides recommending CKD-EPI equations for estimating glomerular filtration, recommend the combination of creatinine and cystatin C (eGFRCr+Cys) as a more accurate approach (5).
To provide a more precise and accurate GFR evaluation in the elderly, the Berlin Initiative Study (BIS) analyzed a cohort of subjects aged over 70 years (77), resulting in the development of two equations designed for older individuals: the BIS1, a creatinine-based equation, and the BIS2, a creatinine and cystatin C–based equation. Both equations provided very good agreements with measured GFR, including in Chinese older subjects (94).
Other eGFR equations for the elderly population have been described such as the Caucasian, Asian, Pediatric and Adult (CAPA) (95), the Japanese equations (96, 97), in a country where the population of persons older than 65 years reached more than 25% of the total population, and the Lund-Malmö revised creatinine equation (LMRCr), developed in a Swedish cohort population population (98). LMRCr showed better performances than CKD-EPIcr. Additionally, combining LMRCr with the CAPA cystatin C equation (CAPACys), by performing the arithmetic mean of the LMRCr and CAPA equations (MEANLMR+CAPA), improved the accuracy of the GFR estimation (99).
More recently, in 2016, Pottel et al. developed, in European healthy individuals, a new eGFR equation for the elderly population, the FAS (100), based on serum creatinine. The validation was carried out comparing with measured GFR (inulin, iohexol, and iothalamate clearance). The FAS equation improved the precision and accuracy of eGFR, especially in older adults, also in chinese older individuals (101). Subsequently, besides the Full Age Spectrum creatinine equation (FASCr), its combination with cystatin C (FASCr+Cys) was proposed (31).
Table 3 summarizes the main studies evaluating eGFR against a gold-standard method to measure GFR.
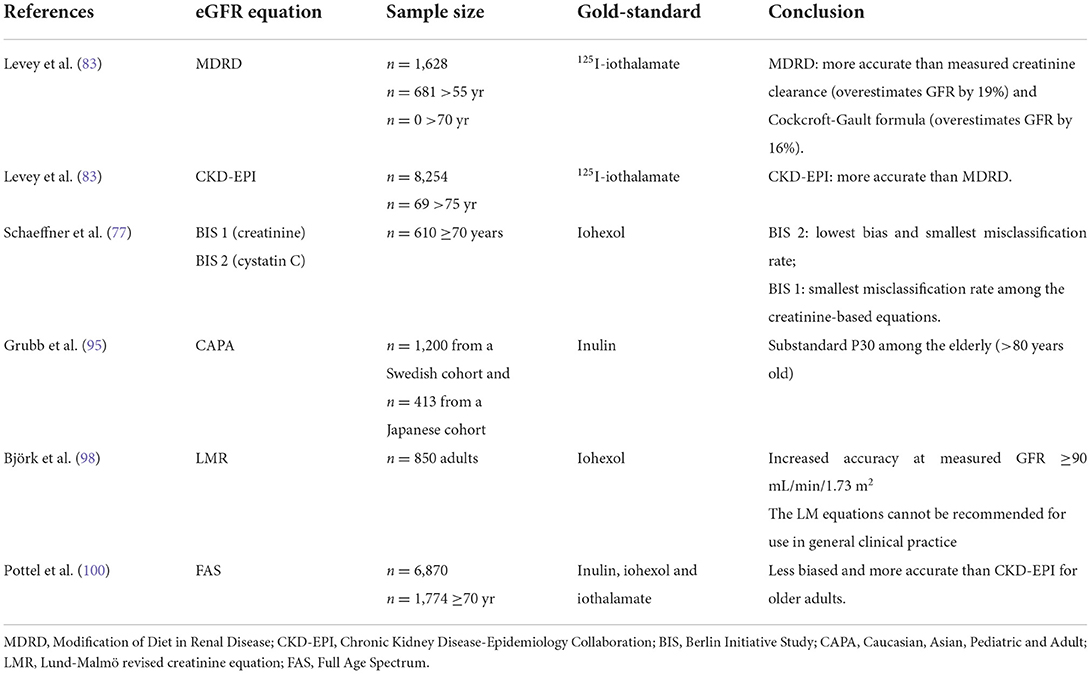
Table 3. Summary of studies addressing equations to estimate glomerular filtration rate and participation of older individuals.
For the geriatric population, which equation is more accurate to estimate GFR?
Compared to gold standard clearance methods, the currently available equations for determining eGFR have different performances related to bias, precision, and accuracy, which highlights the difficulty in defining an accurate estimation of GFR in older patients. It is important to emphasize that the Cockcroft-Gault equation is not similar to CKD-EPI or other eGFR equations.
Table 4 summarizes the results of studies comparing discrepancies of GFR estimating equations in older adults examining performances between the eGFR analyzed by metrics such as bias, precision, and accuracy. Bias represents the median difference between measured GFR and estimated GFR. It is important to identify the eGFR equation with the smallest bias. Bias with negative values indicates that the equation overestimates the GFR. Precision also represents the differences between measured GFR and estimated GFR but it is expressed as the interquartile range (IQR). Accuracy can be evaluated as P30, which represents the percentage of estimates within 30% of the measured value. The highest P30 values reflect better accuracy of eGFR compared to measured GFR.
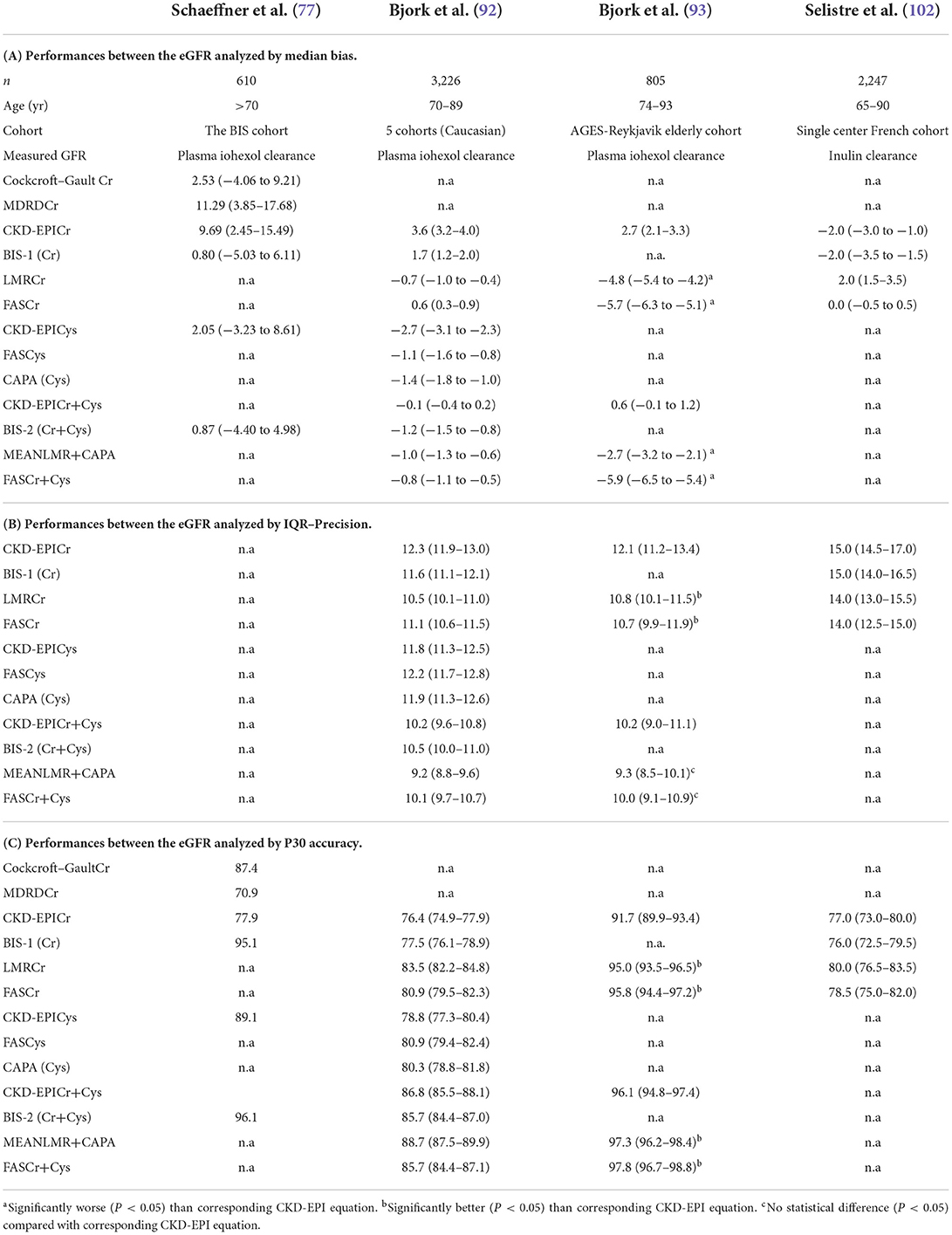
Table 4. Studies comparing discrepancies of GFR estimating equations and estimated creatinine clearance (Cockcroft–Gault) in older adults examining performances between the eGFR analyzed by metrics such as bias, precision, and accuracy.
Schaeffner and coworkers compared performances of the eGFR equations, consisting of the BS1 and BS2 equations, Cockcroft-Gault, MDRD, CKD-EPICr, and CKD-EPICys, with the gold standard iohexol plasma clearance measurement in an elderly population-based cohort [the Berlin Initiative Study (BIS)] (77). BIS equations presented the smallest median bias compared with Cockcroft–Gault, MDRD, and CKD-EPICr equations, which have a higher median bias that overestimates GFR. Accordingly, the P30 values were highest for the BIS equations, followed by the CKD-EPICys and Cockcroft–Gault equations.
The 2017 study of Bjork, a large multicenter cohort of Europeans that enrolled 3,226 individuals 74–93 years of age, validated four creatinine equations (the CKD-EPICr, BIS1Cr, LMRCr, and FASCr) compared to iohexol plasma clearance as a gold-standard method (92). LMRCr had the most stable performance compared to measured GFR. FASCr can also be a good alternative to CKD-EPICr in creatinine based estimation of GFR. The addition of cystatin C to the equations (BIS2Cr+Cys, CKD-EPICr+Cys, FASCr+Cys, and MEANLMR+CAPA) improved the performances, particularly P30 accuracy.
In 2018, Björk et al. compared the performance of GFR estimating equations using creatinine and cystatin C in a cohort of elderly patients from Iceland who participated in the AGES-Reykjavik Study (93). A subgroup of 805 Caucasian individuals, 74–93 years of age, was enrolled in the period between 2007 and 2011 to participate in a substudy that measured GFR using plasma iohexol clearance (the AGES-Kidney Study). Different GFR equations such as LMRCr MEANLMR+CAPA, FASCr, and FASCr+Cys were compared to CKD-EPICr and CKD-EPICr+Cys, All equations showed high accuracy (P30 >90%). Although there were differences in performance, none of the creatinine-based equations was clearly superior in this cohort of older individuals. However, the addition of cystatin C in creatinine-based equations improved the performance of the equations. Fan et al. (103) also analyzed the 805 participants of this AGES-Reykjavik Study, comparing the performance of the Japanese, BIS, and CAPA equations with that of the CKD-EPI equations. They concluded that none of the Japanese, BIS, and CAPA equations were superior to the CKD-EPI equations. The addition of cystatin C in the CKD-EPIcr equation improved the performance of CKD-EPICr and CKD-EPICys.
Selistre et al., in a large single-center French population (n = 2,247) aged 65–90 years with various degrees of kidney impairment, tested four different equations (CKD-EPI, BIS-1, LMR, and FAS) compared to measured GFR using inulin clearance (102). The authors tested the bias, precision, and accuracy of these equations and found that the performance of the CKD-EPI equation was not different from that of BIS-1, LMR and FAS. In patients 75 years or older with measured GFR <45 ml/min/1,73 m2, LMR and BIS were more accurate than CKD-EPI and FAS but none of them had a superior diagnostic performance.
Recently, Yamaguchi et al. estimated GFR based both on serum creatinine and cystatin C in 19,764 individuals aged 18–103 years (mean 77.0 years) separated into two groups, <75 and >75 years old (the older group, n = 12,518, mean age 82.8 years old). The analysis of discrepancies between the CKD-EPI, Japanese, and BIS equations showed that eGFRcr was overestimated with CKD-EPI or the Japanese equation. They concluded that estimation of GFR using serum cystatin C provided more accuracy in elderly people (78).
It is worth mentioning that measured GFR is considered the gold-standard approach to access GFR. This is important because any equation used to estimate GFR may have an average error as wide as 30% or even larger, even while choosing that with higher accuracy, it has a bias that may reach 30 ml/min of difference from the measured filtration rate (104). Therefore, this should be kept in mind when interpreting studies.
Clinical management of age-related GFR changes in the elderly
Accurate determination of GFR in the older population is of crucial importance for the correct evaluation of age-related kidney dysfunction, which implies appropriate management related to the stage of kidney disease.
In clinical practice, one of the most important aspects to be considered is the adequate adjustment of the dosage of prescribed drugs to avoid overdosing. Elderly patients have often been submitted to polypharmacy and many of these medications have renal excretion, which requires dose reductions adjusted to the degree of GFR compromised, more easily calculated by the GFR equations. Whether it is a pathological or age-related reduction in GFR, the dosage of renal-eliminating drugs should be adjusted according to the GFR, to avoid potential harm.
A great number of older individuals suffer chronic and acute pain, with a particularly high prevalence of joint pains and neuralgia. Non-steroidal anti-inflammatory drugs (NSAIDs) are often prescribed for the management of pain relief in the elderly population. NSAIDs are considered nephrotoxic drugs, with the exposure associated with a high risk of GFR decline of ≥30% in individuals with eGFR <60 ml/min/1.73 m2 (105). Hence, the prescription of NSAIDs should be done carefully in this population.
The use of iodinated contrast media is another potential cause of kidney injury possibly leading to kidney adverse outcomes such as renal dysfunction characterized by abrupt GFR decline and, eventually, the need for dialysis. The use of iodine-based contrast media for diagnostic and interventional radiology for the geriatric population has exponentially increased in the last decades, in oncological settings, cardiovascular imaging examinations, and other clinical situations. Risk factors for developing contrast nephrotoxicity include pre-existing CKD, dehydration, diabetes, and the use of ACE inhibitors, diuretics, and NSAIDs, all common situations in older patients providing high susceptibility to developing worsening kidney function (106, 107). The advent of iso-osmolar and hypo-osmolar agents decreased the incidence of contrast-induced AKI. Thus, the decision on its use must be considered, evaluating the risk-benefit of the imaging examination. Prehydration and n-acetylcysteine, traditionally used as protective measures, have been questioned since the latest clinical trials and have not shown benefits in their use (108, 109).
Adjustment of drug dosing in the elderly according to GFR is widely employed in clinical settings important for avoiding harmful complications. In the aging population, type 2 diabetes is a prevalent condition, and for instance, the risk of hypoglycemia can be avoided, at least in part, by the adjustment of oral antidiabetic agents. Older patients are often affected by infections for which antimicrobials are prescribed. Therefore, the knowledge of GFR is crucial in determining the ideal dose adjustment. In situations of a moderate/severe reduction in GFR, attention must be paid to avoid toxicity, and the risk of worsening renal function is needed.
In summary, the clinical management of older individuals should be based on “do not harm.” The use of contrast should be leveraged according to each indication. Besides antimicrobials, all prescriptions for older individuals should consider possible the risk of further reduction in GFR. Adjustment according to measured or estimated GFR is advised. All patients should be alert against the use of potential renal-damage drugs.
Conclusion
The population is aging. With the natural aging process, all organs, including the kidneys, undergo aging-related changes characterized by a progressively decline in biological functions and anatomical changes. The loss of kidney function due to advancing age is expressed by GFR decline. Accurate assessment of renal function in the elderly is of particular clinical importance for the appropriate evaluation and management of age-related kidney dysfunction.
The gold standard methods to measure GFR include inulin clearance and plasma clearances based on clearance of radiolabelled or non-radiolabelled exogenous markers, which are laborious, expensive and not feasible in daily clinical practice. In clinical settings, an alternative to gold standard methods still capable of easily provide reliable evaluation of kidney function in the elderly is the use of estimated GFR (eGFR) equations. Hitherto, no equation precisely mirrors the measured GFR in older patients, and therefore, the results should be meticulously interpreted. The currently available equations for determining eGFR have different performances that lead to important discrepancies even in equations specifically developed for older patients. New techniques to measure GFR should be the target of future studies. The ideal method should be cost-effective and not rely only upon serum creatinine, particularly in the elderly and frail patients with sarcopenia and loss of muscle mass. Until then, equations should be used based on either creatinine or preferentially cystatin C, when available. So far, the CKD-EPI seems to be the best choice.
Author contributions
IN planned the review article and drafted the manuscript. GS-C, LA, and RE helped to draft the manuscript. RE, LA, VAC, WJ-F, and IN gave important intellectual contributions. All authors read and approved the final version.
Acknowledgments
The authors are very grateful to Luciana Beatriz Andrade for her excellent design support in Figure 1.
Conflict of interest
The authors declare that the research was conducted in the absence of any commercial or financial relationships that could be construed as a potential conflict of interest.
Publisher's note
All claims expressed in this article are solely those of the authors and do not necessarily represent those of their affiliated organizations, or those of the publisher, the editors and the reviewers. Any product that may be evaluated in this article, or claim that may be made by its manufacturer, is not guaranteed or endorsed by the publisher.
References
1. United Nations. Department of Economic and Social Affairs. Population Division. World population ageing 2020 Highlights : Living Arrangements of Older Persons. New York, US: United Nations (2020).
2. Rule AD, Amer H, Cornell LD, Taler SJ, Cosio FG, Kremers WK, et al. The association between age and nephrosclerosis on renal biopsy among healthy adults. Ann Intern Med. (2010) 159:561–7. doi: 10.7326/0003-4819-152-9-201005040-00006
3. Ebert N, Jakob O, Gaedeke J, Van Der Giet M, Kuhlmann MK, Martus P, et al. Prevalence of reduced kidney function and albuminuria in older adults: The Berlin initiative study. Nephrol Dial Transplant. (2017) 32:997–1005. doi: 10.1093/ndt/gfw079
4. Kidney disease outcomes quality initiative (KDOQI). Part 4. Definition and classification of stagesof chronic kidney disease. 39:S46–75. doi: 10.1053/ajkd.2002.30943
5. Andrassy KM. Comments on ‘KDIGO 2012 clinical practice guideline for the evaluation and management of chronic kidney disease. Kidney Int. (2013) 84:622–3. doi: 10.1038/ki.2013.243
6. O'Hare AM, Rodriguez RA, Rule AD. Overdiagnosis of chronic kidney disease in older adults—an inconvenient truth. JAMA Intern Med. (2021) 181:1366. doi: 10.1001/jamainternmed.2021.4823
7. Delanaye P, Schaeffner E, Ebert N, Cavalier E, Mariat C, Krzesinski JM, et al. Normal reference values for glomerular filtration rate: what do we really know? Nephrol Dial Transplant. (2012) 27:2664–72. doi: 10.1093/ndt/gfs265
8. Liu P, Quinn RR, Lam NN, Elliott MJ, Xu Y, James MT, et al. Accounting for age in the definition of chronic kidney disease. JAMA Intern Med. (2021) 181:1359–66. doi: 10.1001/jamainternmed.2021.4813
9. Neal CR, Arkill KP, Bell JS, Betteridge KB, Bates DO, Winlove CP, et al. Novel hemodynamic structures in the human glomerulus. Am J Physiol Ren Physiol. (2018) 315:1370–84. doi: 10.1152/ajpre
10. Schlöndorff D, Wyatt CM, Campbell KN. Revisiting the determinants of the glomerular filtration barrier: what goes round must come round. Kidney Int. (2017) 92:533–6. doi: 10.1016/j.kint.2017.06.003
11. Delanaye P, Jager KJ, Bökenkamp A, Christensson A, Dubourg L, Eriksen BO, et al. CKD: A call for an age-adapted definition. J Am Soc Nephrol. (2019) 30:1785–805. doi: 10.1681/ASN.2019030238
12. Poggio ED, Rule AD, Tanchanco R, Arrigain S, Butler RS, Srinivas T, et al. Demographic and clinical characteristics associated with glomerular filtration rates in living kidney donors. Kidney Int. (2009) 75:1079–87. doi: 10.1038/ki.2009.11
13. Ma Y-C, Zuo L, Chen L, Su Z-M, Meng S, Li J-J, et al. Distribution of measured GFR in apparently healthy Chinese adults. Am J Kidney Dis. (2010) 56:420–1. doi: 10.1053/j.ajkd.2010.05.005
14. Fliser D, Ritz E. Serum cystatin C concentration as a marker of renal dysfunction in the elderly. Am J Kidney Dis. (2001) 37:79–83. doi: 10.1053/ajkd
15. Stevens LA, Coresh J, Greene T, Levey AS. Assessing kidney function-measured and estimated glomerular filtration rate. N Engl J Med. (2006) 354:2473–83. doi: 10.1056/NEJMra054415
16. Diao JA, Inker LA, Levey AS, Tighiouart H, Powe NR, Manrai AK. In search of a better equation — performance and equity in estimates of kidney function. N Engl J Med. (2021) 384:396–9. doi: 10.1056/NEJMp2028243
17. Inker LA, Eneanya ND, Coresh J, Tighiouart H, Wang D, Sang Y, et al. New creatinine- and cystatin C–based equations to estimate GFR without race. N Engl J Med. (2021) 385:1737–49. doi: 10.1056/nejmoa2102953
18. Levey AS, Stevens LA, Schmid CH, Zhang Y, Castro III AF, Feldman HI, et al. A new equation to estimate glomerular filtration rate. Ann Intern Med. (2009) 150:604–12. doi: 10.7326/0003-4819-150-9-200905050-00006
19. Quaggin SE, Palevsky PM. Removing race from kidney disease diagnosis. J Am Soc Nephrol. (2021) 32:2987–9. doi: 10.1681/ASN.2021091284
20. Delanaye P, Pottel H, Glassock RJ. Americentrism in estimation of glomerular filtration rate equations. Kidney Int. (2022) 101:856–8. doi: 10.1016/j.kint.2022.02.022
21. Davies DF, Shock NW. Age changes in glomerular filtration rate, effective renal plasma flow, and tubular excretory capacity in adult males. J Clin Invest. (1950) 29:496–507. doi: 10.1172/JCI102286
22. Glassock RJ, Rule AD. The implications of anatomical and functional changes of the aging kidney: with an emphasis on the glomeruli. Kidney Int. (2012) 82:270–7. doi: 10.1038/ki.2012.65
23. Lindeman RD, Tobin J, Shock NW. Longitudinal studies on the rate of decline in renal function with age. J Am Geriatr Soc. (1985) 33:278–85. doi: 10.1111/j.1532-5415.1985.tb07117.x
24. Fuiano G, Sund S, Mazza G, Rosa M, Caglioti A, Gallo G, et al. Renal hemodynamic response to maximal vasodilating stimulus in healthy older subjects. Kidney Int. (2001) 59:1052–8. doi: 10.1046/j.1523-1755.2001.0590031052.x
26. Rule AD, Gussak HM, Pond GR, Bergstralh EJ, Stegall MD, Cosio FG, et al. Transplantation measured and estimated GFR in healthy potential kidney donors. Am J Kidney Dis. (2004) 43:112–9. doi: 10.1053/j.ajkd.2003.09.026
27. Kasiske BL, Anderson-Haag T, Israni AK, Kalil RS, Kimmel PL, Kraus ES, et al. A prospective controlled study of living kidney donors: three-year follow-up. Am J Kidney Dis. (2015) 66:114–24. doi: 10.1053/j.ajkd.2015.01.019
28. Baba M, Shimbo T, Horio M, Ando M, Yasuda Y, Komatsu Y, et al. Longitudinal study of the decline in renal function in healthy subjects. PLoS ONE. (2015) 10:e0129036. doi: 10.1371/journal.pone.0129036
29. Grewal GS, Blake GM, Guy's K', Thomas S. Reference Data for 51 Cr-EDTA Measurements of the Glomerular Filtration Rate Derived From Live Kidney Donors. London, UK: Lippincott Williams & Wilkins (2005). p. 61–65.
30. Pottel H, Delanaye P, Weekers L, Selistre L, Goffin K, Gheysens O, et al. Age-dependent reference intervals for estimated and measured glomerular filtration rate. Clin Kidney J. (2017) 10:545–51. doi: 10.1093/ckj/sfx026
31. Pottel H, Hoste L, Yayo E, Delanaye P. Glomerular filtration rate in healthy living potential kidney donors: a meta-analysis supporting the construction of the full age spectrum equation. Nephron. (2017) 135:105–19. doi: 10.1159/000450893
32. Eriksen BO, Stefansson VTN, Jenssen TG, Mathisen UD, Schei J, Solbu MD, et al. Elevated blood pressure is not associated with accelerated glomerular filtration rate decline in the general non-diabetic middle-aged population. Kidney Int. (2016) 90:404–10. doi: 10.1016/j.kint.2016.03.021
33. Eriksen BO, Palsson R, Ebert N, Melsom T, van der Giet M, Gudnason V, et al. GFR in healthy aging: an individual participant data meta-analysis of iohexol clearance in european population-based cohorts. J Am Soc Nephrol. (2020) 31:1602–15. doi: 10.1681/ASN.2020020151
34. Waas T, Schulz A, Lotz J, Rossmann H, Pfeiffer N, Beutel ME, et al. Distribution of estimated glomerular filtration rate and determinants of its age dependent loss in a German population-based study. Sci Rep. (2021) 11:10165. doi: 10.1038/s41598-021-89442-7
35. Hoang K, Tan JC, Derby G, Blouch KL, Masek M, Ma I, et al. Determinants of glomerular hypofiltration in aging humans. Kidney Int. (2003) 64:1417-24. doi: 10.1046/j.1523-1755.2003.00207.x
36. Weinstein JR, Anderson S. The aging kidney: physiological changes. Adv Chronic Kidney Dis. (2010) 17:302–7. doi: 10.1053/j.ackd.2010.05.002
37. Kielstein JT, Bode-Böger SM, Frölich JC, Ritz E, Haller H, Fliser D. Asymmetric dimethylarginine, blood pressure, and renal perfusion in elderly subjects. Circulation. (2003) 107:1891–5. doi: 10.1161/01.CIR.0000060496.23144.A7
38. Nyengaard JR, Bendtsen TF. Glomerular number and size in relation to age, kidney weight, and body surface in normal man. Anat Rec. (1992) 232:194–201.
39. McNamara BJ, Diouf B, Douglas-Denton RN, Hughson MD, Hoy WE, Bertram JF, et al. comparison of nephron number, glomerular volume and kidney weight in Senegalese Africans and African Americans. Nephrol Dial Transplant. (2010) 25:1514–20. doi: 10.1093/ndt/gfq030
40. Hoy WE, Ingelfinger JR, Hallan S, Hughson MD, Mott SA, Bertram JF. The early development of the kidney and implications for future health. J Dev Orig Health Dis. (2010) 1:216–33. doi: 10.1017/S204017441000022X
41. Denic A, Lieske JC, Chakkera HA, Poggio ED, Alexander MP, Singh P, et al. The substantial loss of nephrons in healthy human kidneys with aging. J Am Soc Nephrol. (2017) 28:313–20. doi: 10.1681/ASN.2016020154
42. Karam Z, Tuazon J. Anatomic and physiologic changes of the aging kidney. Clin Geriatr Med. (2013) 29:555–64. doi: 10.1016/j.cger.2013.05.006
43. Kaplan C, Pasternack B, Shah H, Gallo G. Age-related incidence of sclerotic glomeruli in human kidneys. Am J Pathol. (1975) 80:227–34.
44. Anderson S, Brenner BM. Effects of aging on the renal glomerulus. Am J Med. (1986) 80:435–42. doi: 10.1016/0002-9343(86)90718-7
45. Kremers WK, Denic A, Lieske JC, Alexander MP, Kaushik V, Elsherbiny HE, et al. Distinguishing age-related from disease-related glomerulosclerosis on kidney biopsy: the aging kidney anatomy study. Nephrol Dial Transplant. (2015) 30:2034–9. doi: 10.1093/ndt/gfv072
46. Neugarten J, Gallo G, Silbiger S, Kasiske B. Glomerulosclerosis in aging humans is not influenced by gender. Am J Kidney Dis. (1999) 34:884–8. doi: 10.1016/S0272-6386(99)70046-6
47. Hoy WE, Douglas-Denton RN, Hughson MD, Cass A, Johnson K, Bertram JF. A stereological study of glomerular number and volume: preliminary findings in a multiracial study of kidneys at autopsy. Kidney Int Suppl. (2003) 63:S31–7. doi: 10.1046/j.1523-1755.63.s83.8.x
48. Denic A, Mathew J, Lerman LO, Lieske JC, Larson JJ, Alexander MP, et al. Single-nephron glomerular filtration rate in healthy adults. N Engl J Med. (2017) 376:2349–57. doi: 10.1056/nejmoa1614329
49. Roseman DA, Hwang S-J, Oyama-Manabe N, Chuang ML, O'Donnell CJ, Manning WJ, et al. Clinical associations of total kidney volume: the framingham heart study. Nephrol Dial Transplant. (2016) 32:1344–50. doi: 10.1093/ndt/gfw237
50. Wang X, Vrtiska TJ, Avula RT, Walters LR, Chakkera HA, Kremers WK, et al. Age, kidney function, and risk factors associate differently with cortical and medullary volumes of the kidney. Kidney Int. (2014) 85:677–85. doi: 10.1038/ki.2013.359
51. Denic A, Glassock RJ, Rule AD. Structural and Functional Changes With the Aging Kidney. Adv Chronic Kidney Dis. (2016) 23:19–28. doi: 10.1053/j.ackd.2015.08.004
52. Abdi R, Slakey D, Kittur D, Racusen LC. Heterogeneity of glomerular size in normal donor kidneys: impact of race. Am J Kidney Dis. (1998) 32:43–6. doi: 10.1053/ajkd.1998.v32.pm9669422
53. Fulladosa X, Moreso F, Narváez JA, Grinyó JM, Serón D. Estimation of total glomerular number in stable renal transplants. J Am Soc Nephrol. (2003) 14:2662–8. doi: 10.1097/01.ASN.0000088025.33462.B0
54. Daniels BS, Hostetter TH. Adverse effects of growth in the glomerular microcirculation. Am J Physiol Ren Fluid Electrolyte Physiol. (1990) 258:1409–16. doi: 10.1152/ajprenal.1990.258.5.f1409
55. Wiggins JE, Goyal M, Sanden SK, Wharram BL, Shedden KA, Misek DE, et al. Podocyte hypertrophy, “adaptation” and “decompensation” associated with glomerular enlargement and glomerulosclerosis in the aging rat: Prevention by calorie restriction. J Am Soc Nephrol. (2005) 16:2953–66. doi: 10.1681/ASN.2005050488
56. Hommos MS, Glassock RJ, Rule AD. Structural and functional changes in human kidneys with healthy aging. J Am Soc Nephrol. (2017) 28:2838–44. doi: 10.1681/ASN.2017040421
57. Andrade L, Rodrigues CE, Gomes SA, Noronha IL. Acute kidney injury as a condition of renal senescence. Cell Transplant. (2018) 27:739–53. doi: 10.1177/0963689717743512
58. Schmitt R, Cantley LG. The impact of aging on kidney repair. Am J Physiol Ren Physiol. (2008) 294:1265–72. doi: 10.1152/ajprenal.00543.2007.-The
59. Yang H, Fogo AB. Cell senescence in the aging kidney: figure 1. J Am Soc Nephrol. (2010) 21:1436–9. doi: 10.1681/ASN.2010020205
60. Kuro-o M. Klotho and aging. Biochim Biophys Acta - Gen Sub. (2009) 1790:1049–58. doi: 10.1016/j.bbagen.2009.02.005
62. Hollenberg NK, Rivera A, Meinking T, Martinez G, McCullough M, Passan D, et al. Age, renal perfusion and function in island-dwelling indigenous Kuna Amerinds of Panama. Nephron. (1999) 82:131–8. doi: 10.1159/000045389
63. Lindeman RD, Tobin JD, Shock NW. Association between blood pressure and the rate of decline in renal function with age. Kidney Int. (1984) 26:861–8. doi: 10.1038/ki.1984.229
64. Fliser D, Franek E, Joest M, Block S, Mutschler E, Ritz E. Renal function in the elderly: Impact of hypertension and cardiac function. Kidney Int. (1997) 51:1196–204.
65. Fliser D, Ritz E. Relationship between hypertension and renal function and its therapeutic implications in the elderly. Gerontology. (1998) 44:123–31. doi: 10.1159/000021995
66. Higashi Y, Oshima T, Ozono R, Matsuura H, Kajiyama G. Aging and severity of hypertension attenuate endothelium-dependent renal vascular relaxation in humans. Hypertension. (1997) 30:252–8. doi: 10.1161/01.HYP.30.2.252
67. Sabbatini M, Sansone G, Uccello F, De Nicola L, Giliberti A, Sepe V, et al. Functional versus structural changes in the pathophysiology of acute isehemic renal failure in aging rats. Kidney Int. (1994) 45:1355–61. doi: 10.1038/ki.1994.177
68. Baylis C. Sexual dimorphism in the aging kidney: Differences in the nitric oxide system. Nat Rev Nephrol. (2009) 5:384–96. doi: 10.1038/nrneph.2009.90
69. Granger A. Abnormal pressure-natriuresis in hypertension: role of nitric oxide. Acta Physiol Scand. (2000) 168:161–8. doi: 10.1046/j.1365-201x.2000.00655.x
70. Sands JM. Urine concentrating and diluting ability during aging. J Gerontol Ser A Biol Sci Med Sci. (2012) 67:1352–7. doi: 10.1093/gerona/gls128
71. Bengele HH, Mathias R, Perkins JH, McNamara ER, Alexander EA. Impaired renal and extrarenal potassium adaptation in old rats. Kidney Int. (1983) 23:684–90. doi: 10.1038/ki.1983.79
72. Berkemeyer S, Vormann J, Günther ALB, Rylander R, Frassetto LA, Remer T. Renal net acid excretion capacity is comparable in prepubescence, adolescence, and young adulthood but falls with aging. J Am Geriatr Soc. (2008) 56:1442–8. doi: 10.1111/j.1532-5415.2008.01799.x
73. Gallagher JC. Vitamin D and Aging. Endocrinol Metab Clin North Am. (2013) 42:319–32. doi: 10.1016/j.ecl.2013.02.004
74. Schaeffner ES, Van Der Giet M, Gaedeke J, Tölle M, Ebert N, Kuhlmann MK, et al. The Berlin initiative study: the methodology of exploring kidney function in the elderly by combining a longitudinal and cross-sectional approach. Eur J Epidemiol. (2010) 25:203–10. doi: 10.1007/s10654-010-9424-x
75. Garasto S, Fusco S, Corica F, Rosignuolo M, Marino A, Montesanto A, et al. Estimating glomerular filtration rate in older people. Biomed Res Int. (2014) 2014:916542. doi: 10.1155/2014/916542
76. Liu X, Wang Y, Wang C, Shi C, Cheng C, Chen J, et al. A new equation to estimate glomerular filtration rate in Chinese elderly population. PLoS ONE. (2013) 8:0079675. doi: 10.1371/journal.pone.0079675
77. Schaeffner ES, Ebert N, Delanaye P, Frei U, Gaedeke J, Jakob O, et al. Two novel equations to estimate kidney function in persons aged 70 years or older. Ann Intern Med. (2012) 157:471–81. doi: 10.7326/0003-4819-157-7-201210020-00003
78. Yamaguchi Y, Itabashi M, Yumura W, Takei T. Geriatric assessment of estimated glomerular filtration rate: a cross-sectional study. Clin Exp Nephrol. (2020) 24:216–24. doi: 10.1007/s10157-019-01797-4
79. Sterner G, Frennby B, Mansson S, Nyman U, Van Westen D, Almén T. Determining “true” glomerular filtration rate in healthy adults using infusion of inulin and comparing it with values obtained using other clearance techniques or prediction equations. Scand J Urol Nephrol. (2008) 42:278–85. doi: 10.1080/00365590701701806
80. Iacomelli I, Giordano A, Rivasi G, Rafanelli M, Tortù V, Cartei A, et al. Low creatinine potentially overestimates glomerular filtration rate in older fracture patients: a plea for an extensive use of cystatin C? Eur J Intern Med. (2021) 84:74–9. doi: 10.1016/j.ejim.2020.06.016
81. Baxmann AC, Ahmed MS, Marques NC, Menon VB, Pereira AB, Kirsztajn GM, et al. Influence of muscle mass and physical activity on serum and urinary creatinine and serum cystatin C. Clin J Am Soc Nephrol. (2008) 3:348–54. doi: 10.2215/CJN.02870707
82. Levey AS, Coresh J, Balk E, Kausz AT, Levin A, Steffes MW, et al. National kidney foundation practice guidelines for chronic kidney disease: evaluation, classification, and stratification. Ann Intern Med. (2003) 139:137. doi: 10.7326/0003-4819-139-2-200307150-00013
83. Levey AS, Bosch JP, Lewis JB, Greene T, Rogers N, Roth D. Annals of internal medicine a more accurate method to estimate glomerular filtration rate from serum creatinine: a new prediction equation. Ann Intern Med. (1999) 130:461–70.
84. Inker LA, Schmid CH, Tighiouart H, Eckfeldt JH, Feldman HI, Greene T, et al. Estimating glomerular filtration rate from serum creatinine and cystatin C. N Engl J Med. (2012) 367:20–9. doi: 10.1056/nejmoa1114248
85. Stevens LA, Coresh J, Feldman HI, Greene T, Lash JP, Nelson RG, et al. Evaluation of the modification of diet in renal disease study equation in a large diverse population. J Am Soc Nephrol. (2007) 18:2749–57. doi: 10.1681/ASN.2007020199
86. Peralta CA, Katz R, Sarnak MJ, Ix J, Fried LF, De Boer I, et al. Cystatin C identifies chronic kidney disease patients at higher risk for complications. J Am Soc Nephrol. (2011) 22:147–55. doi: 10.1681/ASN.2010050483
87. Kazama JJ, Kutsuwada K, Ataka K, Maruyama H, Gejyo F. Serum cystatin C reliably detects renal dysfunction in patients with various renal diseases. Nephron. (2002) 91:13–20. doi: 10.1159/000057599
88. Rule AD, Bailey KR, Lieske JC, Peyser PA, Turner ST. Estimating the glomerular filtration rate from serum creatinine is better than from cystatin C for evaluating risk factors associated with chronic kidney disease. Kidney Int. (2013) 83:1169–76. doi: 10.1038/ki.2013.7
89. Dharnidharka VR, Kwon C, Stevens G. Serum cystatin C is superior to serum creatinine as a marker of kidney function: A meta-analysis. Am J Kidney Dis. (2002) 40:221–6. doi: 10.1053/ajkd.2002.34487
90. Willey JZ, Moon YP, Husain SA, Elkind MS V, Sacco RL, Wolf M, et al. Creatinine versus cystatin C for renal function-based mortality prediction in an elderly cohort: the northern manhattan study. PLoS ONE. (2020) 15:e0226509. doi: 10.1371/journal.pone.0226509
91. Shlipak MG, Matsushita K, Ärnlöv J, Inker LA, Katz R, Polkinghorne KR, et al. Cystatin C versus creatinine in determining risk based on kidney function. N Engl J Med. (2013) 369:932–43. doi: 10.1056/NEJMoa1214234
92. Björk J, Bäck SE, Ebert N, Evans M, Grubb A, Hansson M, et al. GFR estimation based on standardized creatinine and cystatin C: a European multicenter analysis in older adults. Clin Chem Lab Med. (2018) 56:422–35. doi: 10.1515/cclm-2017-0563
93. Björk J, Grubb A, Gudnason V, Indridason OS, Levey AS, Palsson R, et al. Comparison of glomerular filtration rate estimating equations derived from creatinine and cystatin C: validation in the age, gene/environment susceptibility-reykjavik elderly cohort. Nephrol Dial Transplant. (2018) 33:1380–8. doi: 10.1093/ndt/gfx272
94. Changjie G, Xusheng Z, Feng H, Shuguang Q, Jianwen L, Junzhou F. Evaluation of glomerular filtration rate by different equations in Chinese elderly with chronic kidney disease. Int Urol Nephrol. (2017) 49:133–41. doi: 10.1007/s11255-016-1359-z
95. Grubb A, Horio M, Hansson LO, Björk J, Nyman U, Flodin M, et al. Generation of a new cystatin C-based estimating equation for glomerular filtration rate by use of 7 assays standardized to the international calibrator. Clin Chem. (2014) 60:974–86. doi: 10.1373/clinchem.2013.220707
96. Matsuo S, Imai E, Horio M, Yasuda Y, Tomita K, Nitta K, et al. Revised equations for estimated GFR from serum creatinine in Japan. Am J Kidney Dis. (2009) 53:982–92. doi: 10.1053/j.ajkd.2008.12.034
97. Horio M, Imai E, Yasuda Y, Watanabe T, Matsuo S. Performance of GFR equations in Japanese subjects. Clin Exp Nephrol. (2013) 17:352–8. doi: 10.1007/s10157-012-0704-5
98. Björk J, Grubb A, Sterner G, Nyman U. Revised equations for estimating glomerular filtration rate based on the lund-malmö study cohort. Scand J Clin Lab Invest. (2011) 71:232–9. doi: 10.3109/00365513.2011.557086
99. Björk J, Grubb A, Larsson A, Hansson L-O, Flodin M, Sterner G, et al. Accuracy of GFR estimating equations combining standardized cystatin C and creatinine assays: a cross-sectional study in Sweden. Clin Chem Lab Med. (2015) 53:403–14. doi: 10.1515/cclm-2014-0578
100. Pottel H, Hoste L, Dubourg L, Ebert N, Schaeffner E, Eriksen BO, et al. An estimated glomerular filtration rate equation for the full age spectrum. Nephrol Dial Transplant. (2016) 31:798–806. doi: 10.1093/ndt/gfv454
101. Chai L, Wang M, Cai K, Luo Q, Yi H, Wu J. Full age spectrum equation may be an alternative method to estimate the glomerular filtration rate in Chinese patients with chronic kidney disease. Clin Nephrol. (2018) 89:413–21. doi: 10.5414/CN109256
102. Da Silva Selistre L, Rech DL, De Souza V, Iwaz J, Lemoine S, Dubourg L. Diagnostic performance of creatinine-based equations for estimating glomerular filtration rate in adults 65 years and older. JAMA Intern Med. (2019) 179:796–804. doi: 10.1001/jamainternmed.2019.0223
103. Fan L, Levey AS, Gudnason V, Eiriksdottir G, Andresdottir MB, Gudmundsdottir H, et al. Comparing GFR estimating equations using cystatin C and creatinine in elderly individuals. J Am Soc Nephrol. (2015) 26:1982–9. doi: 10.1681/ASN.2014060607
104. Porrini E, Ruggenenti P, Luis-Lima S, Carrara F, Jiménez A, de Vries APJ, et al. Estimated GFR: time for a critical appraisal. Nat Rev Nephrol. (2019) 15:177–90. doi: 10.1038/s41581-018-0080-9
105. Wan EYF, Yu EYT, Chan L, Mok AHY, Wang Y, et al. Comparative risks of nonsteroidal anti-inflammatory drugs on CKD. Clin J Am Soc Nephrol. (2021) 16:898–907. doi: 10.2215/CJN.18501120
106. Alsafi A, Alsafi Z, Lakhani A, Strickland NH. Changes in renal function in elderly patients following intravenous iodinated contrast administration: a retrospective study. Radiol Res Pract. (2014) 2014:1–4. doi: 10.1155/2014/459583
107. Marenzi G, Lauri G, Assanelli E, Campodonico J, De Metrio M, Marana I, et al. Contrast-induced nephropathy in patients undergoing primary angioplasty for acute myocardial infarction. J Am Coll Cardiol. (2004) 44:1780–5. doi: 10.1016/j.jacc.2004.07.043
108. Weisbord SD, Gallagher M, Jneid H, Garcia S, Cass A, Thwin S-S, et al. Outcomes after angiography with sodium bicarbonate and acetylcysteine. N Engl J Med. (2018) 378:603–14. doi: 10.1056/nejmoa1710933
109. Timal RJ, Kooiman J, Sijpkens YWJ, De Vries JPPM, Verberk-Jonkers IJAM, Brulez HFH, et al. Effect of no prehydration vs sodium bicarbonate prehydration prior to contrast-enhanced computed tomography in the prevention of postcontrast acute kidney injury in adults with chronic kidney disease: the kompas randomized clinical trial. JAMA Intern Med. (2020) 180:533–41. doi: 10.1001/jamainternmed.2019.7428
Keywords: glomerular filtration rate (GFR), elderly, renal function, senescence, aging, estimated GFR
Citation: Noronha IL, Santa-Catharina GP, Andrade L, Coelho VA, Jacob-Filho W and Elias RM (2022) Glomerular filtration in the aging population. Front. Med. 9:769329. doi: 10.3389/fmed.2022.769329
Received: 01 September 2021; Accepted: 24 August 2022;
Published: 15 September 2022.
Edited by:
Ana Cusumano, Centro de Educación Médica e Investigaciones Clínicas Norberto Quirno (CEMIC), ArgentinaReviewed by:
Matias Trillini, Mario Negri Pharmacological Research Institute (IRCCS), ItalyGaetano Alfano, University of Modena and Reggio Emilia, Italy
Copyright © 2022 Noronha, Santa-Catharina, Andrade, Coelho, Jacob-Filho and Elias. This is an open-access article distributed under the terms of the Creative Commons Attribution License (CC BY). The use, distribution or reproduction in other forums is permitted, provided the original author(s) and the copyright owner(s) are credited and that the original publication in this journal is cited, in accordance with accepted academic practice. No use, distribution or reproduction is permitted which does not comply with these terms.
*Correspondence: Irene L. Noronha, aXJlbmVub3JAdXNwLmJy