- 1Dermatology Unit, University of Rome Tor Vergata, Rome, Italy
- 2Italy State Police Health Service Department, Ministry of Interior, Rome, Italy
- 3Anatomic Pathology Unit, University of Rome Tor Vergata, Rome, Italy
- 4Anatomic Pathology, Santa Maria di Ca' Foncello Hospital, Treviso, Italy
- 5Department of Biology, University of Rome Tor Vergata, Rome, Italy
- 6Istituto di Ricovero e Cura a Carattere Scientifico (IRCCS) Fondazione Santa Lucia, Rome, Italy
- 7Virology Unit, University of Rome Tor Vergata, Rome, Italy
- 8Unit of Dermatology, Department of Surgery and Translational Medicine, University of Florence, Florence, Italy
- 9Department of Surgical Sciences, University Nostra Signora del Buon Consiglio, Tirana, Albania
Psoriasis vulgaris is a chronic inflammatory skin disease characterized by well-demarcated scaly plaques. Oxidative stress plays a crucial role in the psoriasis pathogenesis and is associated with the disease severity. Dimethyl fumarate modulates the activity of the pro-inflammatory transcription factors. This is responsible for the downregulation of inflammatory cytokines and an overall shift from a pro-inflammatory to an anti-inflammatory/regulatory response. Both steps are necessary for the amelioration of psoriatic inflammation, although additional mechanisms have been proposed. Several studies reported a long-term effectiveness and safety of dimethyl fumarate monotherapy in patients with moderate-to-severe psoriasis. Furthermore, psoriasis is a chronic disease often associated to metabolic comorbidities, as obesity, diabetes, and cardiovascular diseases, in which glutathione-S transferase deregulation is present. Glutathione-S transferase is involved in the antioxidant system. An increase of its activity in psoriatic epidermis in comparison with the uninvolved and normal epidermal biopsies has been reported. Dimethyl fumarate depletes glutathione-S transferase by formation of covalently linked conjugates. This review investigates the anti-inflammatory role of dimethyl fumarate in oxidative stress and its effect by reducing oxidative stress. The glutathione-S transferase regulation is helpful in treating psoriasis, with an anti-inflammatory effect on the keratinocytes hyperproliferation, and in modulation of metabolic comorbidities.
Introduction
Psoriasis vulgaris is a chronic inflammatory skin disease characterized by well-demarcated erythema and scaly plaques. It is reported that an enhanced oxidative stress is associated with the severity of psoriasis (1). Karabowicz et al. investigated the intensity of oxidative stress and the expression and activity of the proteasomal system, as well as the autophagy, responsible for the degradation of oxidatively modified proteins in the blood cells of patients with psoriasis (2). Oxidative-antioxidant system plays a crucial role in the psoriasis pathogenesis (3). Numerous studies reveal significantly increased levels of oxidative stress markers, as malondialdehyde, nitric oxide end products, and 8-hydroxy-2′ -deoxyguanosine in the plasma of psoriatic patients. Meanwhile, a decreased total antioxidant capacity, reduced vitamin A and E levels, and a diminished activity of the main antioxidant enzymes were also detected in these patients (4). The antioxidant system involved in oxidative stress reduction is constituted by the glutathione-S transferase (GST). An increased reactive oxygen species (ROS) and insufficient antioxidant activity have been detected in psoriatic lesions (5). Pro-inflammatory cytokines are involved in redox skin balance perturbation in patients with psoriasis (3). Dimethyl fumarate (DMF) and its metabolite monomethyl fumarate (MMF) modulate some signaling proteins activity and intracellular concentration, such as the nuclear factor (erythroid-derived 2)-like 2 (Nrf2), nuclear factor-kappa B (Nf-κB), and cyclic adenosine monophosphate. Some studies showed that DMF can also affect the hypoxia-inducible factor-1 alpha. These actions seem to be responsible for i) the downregulation of inflammatory cytokines and ii) an overall shift from a pro-inflammatory (Th1/Th17) response to an anti-inflammatory/regulatory (Th2) response. Both steps are necessary for the amelioration of psoriatic inflammation, although additional mechanisms have been proposed. There is a growing body of evidence to support the notion that DMF/MMF may also exert effects on granulocytes and non-immune cell lineages, including keratinocytes and endothelial cells. A better understanding of the multiple molecular mechanisms involved in the cellular action of fumaric acid esters (FAEs) will help to adapt and to further improve the use of such small molecules for the treatment of psoriasis and other chronic inflammatory diseases (6). Superoxide dismutase (SOD) and glutathione peroxidase (GP) activity in erythrocytes are involved in the psoriasis onset (7). Imbalance in the oxidant-antioxidant system in psoriasis is involved. The DMF is considered as a prodrug, after oral administration, rapidly hydrolyzed by esterases in the small intestine and converted to MMF representing an intermediate of tricarboxylic acid cycle (TCA) (7). This molecule has been successfully used in psoriasis treatment for more than 40 years. Several clinical trials have demonstrated the FAEs efficacy in this role (6, 8). In 1994, a mixture of MMF and DMF (Fumaderm®) was approved for the oral treatment of psoriasis in Germany, Switzerland, and Austria (9). In 2019, DMF was approved for the treatment of mild-to-moderate plaque psoriasis. Several studies reported a long-term effectiveness and safety of DMF monotherapy in patients with moderate-to-severe psoriasis (9). In humans, people with polymorphisms in GST genes were described to be susceptible to various disorders, including psoriasis (10, 11), coronary artery diseases (12), chronic obstructive pulmonary diseases (13), rheumatoid arthritis (14), or neoplastic diseases, as breast, esophageal, and gastric cancers (15, 16). Furthermore, psoriasis is a chronic disease often associated with metabolic comorbidities, as obesity, diabetes, and cardiovascular diseases, wherein GST deregulation is present (17). Environmental and genetic risk factors have been implicated in obesity etiopathology (18). Also, the oxidative stress could lead to obesity, and the related comorbidities, by promoting a white adipose tissue deposition (19). Several in vitro studies documented that an increased oxidative stress and an ROS could augment adipocyte proliferation, differentiation, and growth (20–22), and control hunger and satiety behaviors (23). Interestingly, there is a mutual relation between oxidative stress and obesity, as abnormal fat accumulation can stimulate a pro-inflammatory and a pro-oxidant state through various biochemical and cellular mechanisms (24–26). The GST, which removes the electrophilic compounds, including the lipid peroxidation products, showed a white adipose tissue-specific downregulation (26). Additionally, the antioxidant enzyme activities of GP and superoxide dismutase were reported to be dysregulated in red blood cells and serum of obese individuals compared to controls (27, 28). Enzyme-converting glutathione is constitutionally expressed by keratinocytes (29). An increase of GST activity in psoriatic epidermis in comparison with uninvolved and normal epidermal biopsies has been reported. The DMF depletes glutathione by formation of covalently linked conjugates. Consequently, oxidized glutathione is converted to a reduced glutathione and is also depleted by DMF (30). The GST includes glutathione enzyme catalyzing conjugation with various hydrophobic compounds (29). Many data evaluated the role of conjugating activity of hydrophobic molecules, such as bilirubin and hematin linkage and selenium-independent GP activity, toward organic hydroperoxides in the oxidative stress cycle (31).
This review investigates the anti-inflammatory role of DMF in oxidative stress and its effect by reducing ROS through glutathione modulation. The GST regulation is helpful in treating psoriasis, with anti-inflammatory effect on the keratinocytes hyperproliferation and in modulation of metabolic comorbidity.
DMF Antioxidant Activity
Dimethyl fumarate (DMF) is considered a prodrug as, after oral administration, it is rapidly hydrolyzed by esterases in the small intestine and converted to MMF (32). The MMF is highly bioavailable and is rapidly hydrolyzed inside cells to fumaric acid, which in mitochondria, represent an intermediate of TCA (33, 34). It is mostly believed that DMF exerts its therapeutic effects through antioxidant and anti-inflammatory pathways (Figure 1). Both MMF and fumarate are believed to be responsible for the primary therapeutic effects of DMF through activation and inhibition of the transcription factors, Nrf2 (35, 36) and Nf-κB (37), respectively. It has been well-described that DMF activates the Nrf2 signaling pathway through the electrophilic modification of Kelch-like ECH-associated protein 1 (35). The DMF exerts its immuno-modulatory activity also via the agonism of the hydroxycarboxylic acid receptor 2 (38). Such important mechanisms, nonetheless, fail to fully account for the in vitro and in vivo immunologic actions of DMF (39). Recent evidence has suggested that modulation of innate and adaptive immune processes is Nrf2 independent (40). Some of the neuro-protective effects seen with this drug are secondary to its anti-inflammatory and antioxidant actions and appear to rely on the modulation of cellular metabolism. Accordingly, a short-term DMF treatment of an oligodendrocyte cell line did not prevent a hydrogen peroxide-mediated death, and a DMF treatment in a model of toxic demyelination was not able to prevent demyelination (41). Importantly, methylated esters of TCA intermediates, such as DMF, are cell permeable and can modify the activity of this pathway by increasing the level of metabolic intermediates' proximate to fumarate. In the TCA, succinate is oxidized to fumarate and then hydrated to malate through the activity of two enzymes, succinate dehydrogenase, and fumarase. Administration of DMF in vitro causes a rise in the concentration of succinate (42, 43). Prolonged treatment with DMF in a human oligodendrocyte cell line elicited increases in both succinate and fumarate (44). This event is associated with augmented lipid synthesis, thus, preserving mature oligodendrocytes viability, and protecting myelin through the modulation of cellular lipid metabolism. These data were confirmed in vivo by using global metabolomics profiling of blood plasma of patients with relapsing-remittent multiple sclerosis treated for 6 weeks with DMF. Significant changes in TCA intermediates fumarate and succinate, and in the secondary TCA metabolites succinyl-carnitine and methyl succinyl-carnitine were observed, arguing that the potential anti-inflammatory properties of these metabolites are mediated by metabolic rewiring. Interestingly these changes were not observed in the control population (45). A metabolic switch toward aerobic glycolysis is mandatory for immune cells activation. Impinging a metabolic rewiring toward mitochondrial oxidative metabolism is considered a valid strategy to counteract the inflammatory process in immune diseases (46). The DMF was shown to covalently modify protein cysteine residues in a process termed succinylation. In activated myeloid and lymphoid cells, DMF was able to downregulate aerobic glycolysis via the succinylation and inactivation of the glycolytic enzyme glyceraldehyde 3-phosphate dehydrogenase, thereby inhibiting the autoimmune response (47). Immune cell activation also depends on calcium signaling. Among the proposed mechanisms for the immunoregulatory role of DMF, the rise of intracellular calcium is also included. In particular, DMF promotes an immediate extracellular calcium influx, long-term increase of cytosolic calcium, and reduced intracellular calcium storage. Upon DMF treatment, the glutathionylation of a cysteine of sarco/endoplasmic reticulum Ca2+-ATPase SERCA2b is critical to the modulation of intracellular calcium concentration. The SERCA2b is downregulated but more active due to glutathionylation of the redox-sensitive cysteine. A net increase of cytosolic calcium due to a diminished calcium storage is, therefore, obtained (48). Fumarate also functions as an immuno-modulator by controlling chromatin modifications. Fumarate can also rewire the epigenetic landscape of the cells through inhibiting either histone or DNA demethylases. Fumarate accumulation has been demonstrated in activated immune cells, and this event inhibits KDM5 histone demethylase activity, thus, promoting the transcription of promoters of TNF-α and IL-6 cytokines (49). Upon DMF treatment, different proteins in T cells are susceptible to covalent modifications of cysteines. Protein kinase C θ modification avoids its association with the co-stimulatory receptor CD28, preventing a T-cell activation (50). Besides such immuno-modulatory actions, DMF has an important antioxidant activity; the way by which it reduces oxidative stress is very peculiar. Actually, it scavenges the major intracellular non-enzymatic thiol antioxidant glutathione (51–54), likely, via the immediate formation of glutathione-DMF adducts (55), and this results in the stabilization and in the raise of Nrf2. Nrf2 then translocates into the nucleus and binds to antioxidant response elements in the promoter region of several antioxidant genes, such as heme-oxygenase-1 and NADPH-quinone-oxidoreductase-1. This, in turn, increases the intracellular concentration of glutathione (35, 56), making the cell more resistant to oxidative stress. However, DMF is able to raise glutathione levels also when the rate-limiting enzyme of glutathione synthesis, i.e., glutamate-cysteine ligase, is inhibited, thanks to the Nrf2-mediated induction of glutathione reductase that enhances the molecule recycling (57).
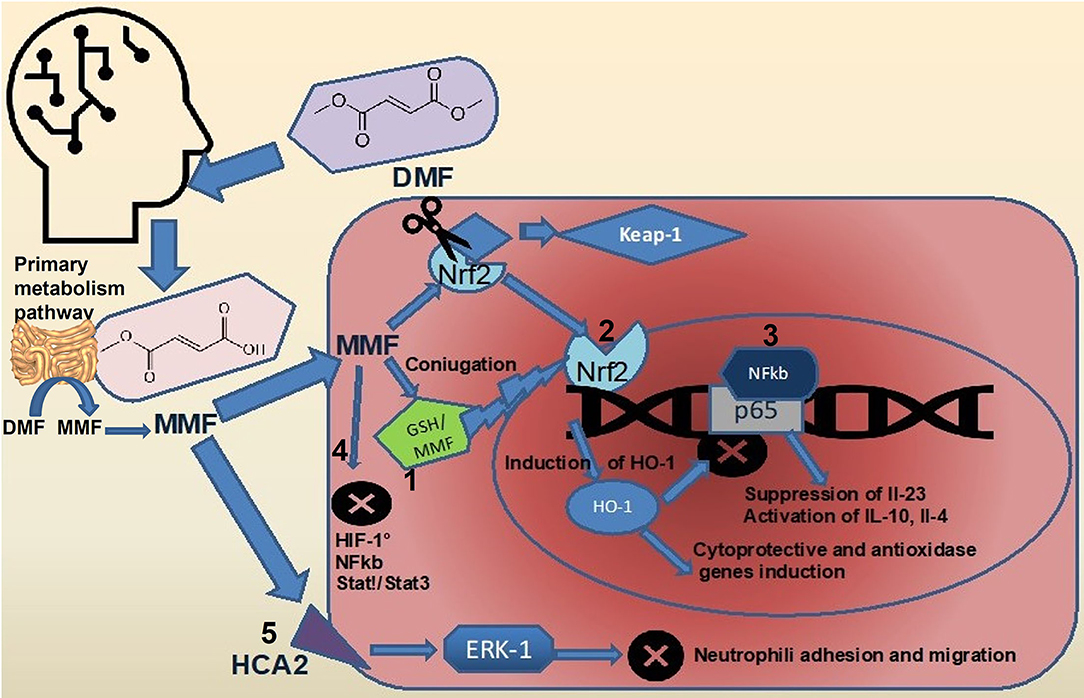
Figure 1. Effects of DMF on intracellular signaling pathways. Upon ingestion, most of the DMF (about 90%) is rapidly converted to MMF by hydrolization in the small intestine (5). The full pharmacokinetic profile of DMF and MMF remains to be elucidated. DMF, dimethyl fumarate; MMF, monomethil fumarate; FAEs, fumaric acid esters; GP, glutathione peroxidase; GST, glutathione-S transferase; NF-kappaB, nuclear factor-kappa B; Nrf2, nuclear factor (erythroid-derived 2)-like 2; ROS, reactive oxygen species; and TCA, tricarboxylic acid cycle.
The Role of Glutathione and DMF in Psoriasis
Several studies have demonstrated that glutathione binding to DNA is able to regulate Nf-κB proinflammatory activity. In particular, the Nf-κB complex and the upstream proteins, as TRAF6, are negatively regulated by glutathione (58). Genetic polymorphisms affecting GST produce a decrease in intracellular concentration of glutathione, with consequent raising of skin inflammation, as seen in atopic and allergic dermatitis, psoriasis, lichen planus, urticaria, and vitiligo (59–62).
Glutathione plasmic levels and GP activity in patients with psoriasis were significantly lower than in general population (63). Consequently, GST activity reduction leads to the accumulation of ROS in inflamed lesions, as it was reported in psoriatic plaques, where ROS levels are 3-fold higher than in non-lesioned skin (64). The DMF action in this context is not yet completely clear. It irreversibly binds the glutathione in a 1:1 ratio, decreasing its production and favoring its excretion through urine as glutathione-DMF adducts (65). In this way, fumarate compounds influence cellular redox state, affecting intracellular signaling pathways (66).
Glutathione intracellular depletion in human antigen-presenting cells causes IL-10 production, with immuno-modulatory action, instead of the pro-inflammatory cytokines IL-12 and IL-23, responsible for Th1/Th17 immune system response switch in psoriasis. In this context, DMF promotes Th2 cell differentiation, with immunoregulatory functions (67).
In summary, the rationale of employing DMF in psoriasis consists in reducing cellular inflammation both by decreasing glutathione intracellular levels and by inducing a switch in immune response toward an anti-inflammatory/immunoregulatory setting (68, 69). European guidelines recommend FAEs in induction and long-term therapy of moderate-to-severe plaque psoriasis (70). With more than 220,000 patients per year treated with FAEs, Germany has been one of the first nations in Europe to adopt this systemic therapy for psoriasis (71), but also other countries, like Italy, are aligned with European guidelines (7). The recommendation in the treatment with DMF is to begin with a low dose followed by gradual increases. This flexible approach is tailored on the need of each patient, and the most used regimen is between 240 mg and 480 mg of DMF per day. Several randomized clinical trials have demonstrated efficacy and safety of FAEs in psoriasis. At week 16 of the phase III, randomized, BRIDGE study, PASI 75 was reached by more than one third of patients enrolled (8), while in the large retrospective FUTURE study it was demonstrated a mean reduction of 79% in PASI from baseline (72). Combination of topical treatments, biological agents, or phototherapy to FAEs in the induction phase showed to reach a faster response (73–75). The FAEs are also characterized by a mild spectrum of side effects, including gastrointestinal disorders and flushing during the treatment, which are not responsible for therapy discontinuation. Among the others, the most important is lymphopenia, which is, generally, of a mild entity and experienced during induction or when it is necessary to increase the dose regimen. It is necessary in such cases to adjust the dosage at the higher tolerance. Treatment discontinuation is required only in rare cases to minimize opportunistic infections' risk (76).
The Role of Small Molecules in the Metabolic Syndrome
Patients with psoriasis are characterized by a higher prevalence of cardiovascular disease and metabolic syndrome (77). In particular, visceral fat has a critical role in the development of cardiovascular disease in patients with psoriasis, including coronary arteries disease, heart infarction, stroke, and related mortality. Moreover, the inflammatory background of the patients with psoriasis both increases and accelerates the atherosclerosis (77). Small molecules, as the phosphodiesterase-4 inhibitor apremilast, approved for the treatment of adults with moderate-to-severe plaque psoriasis and/or psoriatic arthritis, have demonstrated a broad anti-inflammatory activity, which may influence metabolism (17, 78). It has been demonstrated that liver steatosis is reduced by limiting the fat deposition and increasing lipolysis (17). The patients with diabetes reached better results in terms of psoriasis response when treated with apremilast. Moreover, it was observed as a better control of serum glucose levels, a significant reduction of insulin resistance and cholesterol levels, and the restoration of endothelial function, which are all factors strongly associated with propensity to cardiovascular diseases. Finally, apremilast also decreases the systemic inflammatory status of patients with psoriasis, decreasing TNF-α, IFN-γ, IL-12, and IL-23 production (17). As an apremilast, DMF also exhibits strong anti-inflammatory and immunomodulatory effects and was tested in a laboratory to evaluate its role in ameliorating basal inflammation and metabolic disturbances (79). Compared to control rats, those treated with FAEs showed lower levels of C-reactive protein, IL-6, and TNF-α. Moreover, it was demonstrating less fat accumulation, with lower visceral fat weight in liver and muscles. These results suggest the potential crucial role of DMF, as an apremilast, in the treatment of patients with psoriasis with concurrent metabolic comorbidities, which are probably the largest part.
Author Contributions
Conceptualization was contributed by EC, SM, MD, AD, TC, DL, GC, CL, VM, RG, FP, FCo, KA, and LB. Methodology was contributed by EC, MD, AD, TC, DL, GC, CL, VM, RG, FP, FCo, FCi, KA, and LB. Validation was contributed by EC, SM, MD, TC, DL, GC, CL, VM, RG, FP, FCo, FCi, KA, and LB. Formal analysis was contributed by EC, SM, MD, AD, DL, GC, CL, VM, RG, FP, FCo, KA, and LB. Investigation was contributed by EC, SM, MD, AD, TC, GC, CL, VM, RG, FP, FCo, KA, and LB. Resources was contributed by EC, SM, MD, AD, TC, DL, CL, VM, RG, FP, FCo, KA, and LB. Data curation was contributed by EC, SM, MD, AD, TC, DL, GC, VM, RG, FP, FCo, KA, and LB. Writing—original draft preparation was contributed by EC, SM, MD, AD, TC, DL, GC, CL, RG, FP, FCo, KA, and LB. Writing—review and editing were contributed by EC, SM, MD, AD, TC, DL, GC, CL, VM, FP, FCo, FCi, KA, and LB. Visualization was contributed by EC, SM, MD, AD, TC, DL, GC, CL, VM, RG, FCo, KA, and LB. Supervision was contributed by EC, SM, MD, AD, TC, DL, GC, CL, VM, RG, FP, FCi, KA, and LB. Project administration was contributed by EC, SM, MD, AD, TC, DL, GC, CL, VM, RG, FP, FCo, and LB. All authors approved the submitted version and agreed to be personally accountable for the author's own contributions and for ensuring that questions related to the accuracy or integrity of any part of the work, even ones in which the author was not personally involved, are appropriately investigated, resolved, and documented in the literature.
Funding
This study received funding from Almirall S.p.A. The funder was not involved in the study design, collection, analysis, interpretation of data, the writing of this article or the decision to submit it for publication.
Conflict of Interest
The authors declare that the research was conducted in the absence of any commercial or financial relationships that could be construed as a potential conflict of interest.
Publisher's Note
All claims expressed in this article are solely those of the authors and do not necessarily represent those of their affiliated organizations, or those of the publisher, the editors and the reviewers. Any product that may be evaluated in this article, or claim that may be made by its manufacturer, is not guaranteed or endorsed by the publisher.
Acknowledgments
Medical writing and editorial assistance were provided by Irene Scagnol, a biologist with Ph.D. in Clinical Pharmacology - University of Milan.
References
1. Mazzone P, Congestrì M, Scudiero I, Polvere I, Voccola S, Zerillo L, et al. UBAC1/KPC2 Regulates TLR3 signaling in human keratinocytes through functional interaction with the CARD14/CARMA2sh-TANK Complex. Int J Mol Sci. (2020) 21:9365. doi: 10.3390/ijms21249365
2. Karabowicz P, Wroński A, Ostrowska H, Waeg G, Zarkovic N, Skrzydlewska E. Reduced proteasome activity and enhanced autophagy in blood cells of psoriatic patients. Int J Mol Sci. (2020) 21:7608. doi: 10.3390/ijms21207608
3. Barygina VV, Becatti M, Soldi G, Prignano F, Lotti T, Nassi P, et al. Altered redox status in the blood of psoriatic patients: involvement of NADPH oxidase and role of anti-TNF-α therapy. Redox Rep. (2013) 8:100–6. doi: 10.1179/1351000213Y.0000000045
4. Akbulak O, Karadag AS, Akdeniz N, Ozkanli S, Ozlu E, Zemheri E, et al. Evaluation of oxidative stress via protein expression of glutathione S-transferase and cytochrome p450 (CYP450) isoenzymes in psoriasis vulgaris patients treated with methotrexate. Cutan Ocul Toxicol. (2018) 37:180–5. doi: 10.1080/15569527.2017.1369431
5. Brück J, Dringen R, Amasuno A, Pau-Charles I, Ghoreschi K. A review of the mechanisms of action of dimethylfumarate in the treatment of psoriasis. Exp Dermatol. (2018) 27:611–24. doi: 10.1111/exd.13548
6. Yildirim M, Inaloz HS, Baysal V, Delibas N. The role of oxidants and antioxidants in psoriasis. J Eur Acad Dermatol Venereol. (2003) 17:34–6. doi: 10.1046/j.1468-3083.2003.00641.x
7. Carboni I, De Felice C, De Simoni I, Soda R, Chimenti S. Fumaric acid esters in the treatment of psoriasis: an Italian experience. J Dermatolog Treat. (2004) 5:23–6. doi: 10.1080/09546630310019346
8. Mrowietz U, Szepietowski JC, Loewe R, van de Kerkhof P, Lamarca R, Ocker WG, et al. Efficacy and safety of LAS41008 (dimethyl fumarate) in adults with moderate-to-severe chronic plaque psoriasis: a randomized, double-blind, Fumaderm® - and placebo-controlled trial (BRIDGE). Br J Dermatol. (2017) 176:615–23. Erratum in: Br J Dermatol (2018) 178:308. doi: 10.1111/bjd.14947
9. Lijnen R, Otters E, Balak D, Thio B. Long-term safety and effectiveness of high-dose dimethylfumarate in the treatment of moderate to severe psoriasis: a prospective single-blinded follow-up study. J Dermatol Treat. (2016) 27:31–6. doi: 10.3109/09546634.2015.1050980
10. Di Pietro G, Magno LA, Rios-Santos F. Glutathione S-transferases: an overview in cancer research. Expert Opin Drug Metab Toxicol. (2010) 6:153–70. doi: 10.1517/17425250903427980
11. Bolt HM, Thier R. Relevance of the deletion polymorphisms of the glutathione S-transferases GSTT1 and GSTM1 in pharmacology and toxicology. Curr Drug Metab. (2006) 7:613–28. doi: 10.2174/138920006778017786
12. Song Y, Shan Z, Luo C, Kang C, Yang Y, He P, et al. Glutathione S-Transferase T1 (GSTT1) null polymorphism, smoking, and their interaction in coronary heart disease: a comprehensive meta-analysis. Heart Lung Circ. (2017) 26:362–70. doi: 10.1016/j.hlc.2016.07.005
13. Fryer AA, Bianco A, Hepple M, Jones PW, Strange RC, Spiteri MA. Polymorphism at the glutathione S-transferase GSTP1 locus. A new marker for bronchial hyperresponsiveness and asthma. Am J Respir Crit Care Med. (2000) 161:1437–42. doi: 10.1164/ajrccm.161.5.9903006
14. Mattey DL, Hassell AB, Plant M, Dawes PT, Ollier WR, Jones PW, et al. Association of polymorphism in glutathione S-transferase loci with susceptibility and outcome in rheumatoid arthritis: comparison with the shared epitope. Ann Rheum Dis. (1999) 58:164–8. doi: 10.1136/ard.58.3.164
15. Tang J, Zhou Q, Zhao F, Wei F, Bai J, Xie Y, et al. Association of glutathione S-transferase T1, M1 and P1 polymorphisms in the breast cancer risk: a meta-analysis in Asian population. Int J Clin Exp Med. (2015) 8:12430–47. doi: 10.2147/TCRM.S104339
16. Zendehdel K, Bahmanyar S, McCarthy S, Nyren O, Andersson B, Ye W. Genetic polymorphisms of glutathione S-transferase genes GSTP1, GSTM1, and GSTT1 and risk of esophageal and gastric cardia cancers. Cancer Causes Control. (2009) 20:2031–8. doi: 10.1007/s10552-009-9399-7
17. Mazzilli S, Lanna C, Chiaramonte C, Cesaroni GM, Zangrilli A, Palumbo V, et al. Real life experience of apremilast in psoriasis and arthritis psoriatic patients: preliminary results on metabolic biomarkers. J Dermatol. (2020) 47:578–82. doi: 10.1111/1346-8138.15293
18. Fawzy MS, Alhadramy O, Hussein MH, Ismail HM, Ismail NM, Biomy NM, et al. Functional and structural impact of ATP-binding cassette transporter A1 R219K and I883M gene polymorphisms in obese children and adolescents. Mol Diagn Ther. (2015) 9:221–34. doi: 10.1007/s40291-015-0150-7
19. Manna P, Jain SK. Obesity, oxidative stress, adipose tissue dysfunction, and the associated health risks: causes and therapeutic strategies. Metab Syndr Relat Disord. (2015) 13:423–44. doi: 10.1089/met.2015.0095
20. Furukawa S, Fujita T, Shimabukuro M, Iwaki M, Yamada Y, Nakajima Y, et al. Increased oxidative stress in obesity and its impact on metabolic syndrome. J Clin Investig. (2004) 114:1752–61. doi: 10.1172/JCI21625
21. Higuchi M, Dusting GJ, Peshavariya H, Jiang F, Hsiao ST, et al. Differentiation of human adipose-derived stem cells into fat involves reactive oxygen species and forkhead box o1 mediated upregulation of antioxidant enzymes. Stem Cells Dev. (2013) 22:878–8. doi: 10.1089/scd.2012.0306
22. Lee H, Lee Y, Choi H, Ko EH, Kim JW. Reactive oxygen species facilitate adipocyte differentiation by accelerating mitotic clonal expansion. J Biol Chem. (2009) 284:10601–9. doi: 10.1074/jbc.M80874220
23. Horvath TL, Andrews ZB, Diano S. Fuel utilization by hypothalamic neurons: roles for ROS. Trends Endocrinol. Metab. (2009) 20:78–87. doi: 10.1016/j.tem.2008.10.003
24. Savini I, Catani MV, Evangelista D, Gasperi V, Avigliano L. Obesity-associated oxidative stress: strategies finalized to improve redox state. Int J Mol Sci. (2013) 14:10497–538. doi: 10.3390/ijms140510497
25. Colak E, Pap D. The role of oxidative stress in the development of obesity and obesity-related metabolic disorders. J Med Biochem. (2021) 40:1–9. doi: 10.5937/jomb0-24652
26. Picklo MJ, Long EK, Vomhof-DeKrey EE. Glutathionyl systems and metabolic dysfunction in obesity. Nutr Rev. (2015) 73:858–68. doi: 10.1093/nutrit/nuv042
27. Yang X, Deignan JL, Qi H, Zhu J, Qian S, Zhong J, et al. Validation of candidate causal genes for obesity that affect shared metabolic pathways and networks. Nat Genet. (2009) 41:415–23. doi: 10.1038/ng.325
28. Baez-Duarte BG, Zamora-Ginez I, Mendoza-Carrera F, Ruiz-Vivanco G, Torres-Rasgado E, Gonzalez-Mejia ME, et al. Serum levels of glutathione peroxidase 3 in overweight and obese subjects from central Mexico. Arch Med Res. (2012) 43:541–7. doi: 10.1016/j.arcmed.2012.09.001
29. Aceto A, Martini F, Dragani B, Bucciarelli T, Sacchetta P, Di Ilio C. Purification and characterization of glutathione transferase from psoriatic skin. Biochem Med Metab Biol. (1992) 48:212–8. doi: 10.1016/0885-4505(92)90067-9
30. Gambichler T, Kreuter A, Susok L, Skrygan M, Rotterdam S, Höxtermann S, et al. Glutathione-S-transferase T1 genotyping and phenotyping in psoriasis patients receiving treatment with oral fumaric acid esters. J Eur Acad Dermatol Venereol. (2014) 28:574–80. doi: 10.1111/jdv.12137
31. Mannervik B. The isoenzymes of glutathione transferases. Adv Enzymol Relat Areas Mol Biol. (1985) 57:357–417. doi: 10.1002/9780470123034.ch5
32. Nibbering PH, Thio B, Zomerdijk TP, Bezemer AC, Beijersbergen RL, van Furth R. Effects of monomethylfumarate on human granulocytes. J Invest Dermatol. (1993) 101:37–42. doi: 10.1111/1523-1747.ep12358715
33. Litjens NH, Burggraaf J, van Strijen E, van Gulpen C, Mattie H, Schoemaker RC et al. Pharmacokinetics of oral fumarates in healthy subjects. Br J Clin Pharmacol. (2004) 58:429–32. doi: 10.1111/j.1365-2125.2004.02145.x
34. Venci JV, Gandhi MA. Dimethyl fumarate (Tecfidera): a new oral agent for multiple sclerosis. Ann Pharmacother. (2013) 47:1697–702. doi: 10.1177/1060028013509232
35. Linker RA, Lee DH, Ryan S, van Dam AM, Conrad R, Bistaet P, et al. Fumaric acid esters exert neuroprotective effects in neuroinflammation via activation of the Nrf2 antioxidant pathway. Brain. (2011) 134(Pt. 3):678–92. doi: 10.1093/brain/awq386
36. Scannevin RH, Chollate S, Jung MY, Shackett M, Patel H, Bista P, et al. Fumarates promote cytoprotection of central nervous system cells against oxidative stress via the nuclear factor (erythroidderived 2)-like 2 pathway. J Pharmacol Exp Ther. (2012) 341:274–84. doi: 10.1124/jpet.111.190132
37. Gerdes S, Shakery K, Mrowietz U. Dimethylfumarate inhibits nuclear binding of nuclear factor kappaB but not of nuclear factor of activated T cells and CCAAT/enhancer binding protein beta in activated human T cells. Br J Dermatol. (2007) 156:838–42. doi: 10.1111/j.1365-2133.2007.07779.x
38. Chen H, Assmann JC, Krenz A, Rahman M, Grimm M, Karsten CM, et al. Hydroxycarboxylic acid receptor 2 mediates dimethyl fumarate's protective effect in EAE. J Clin Invest. (2014) 124:2188–192. doi: 10.1172/JCI72151
39. Michell-Robinson MA, Moore CS, Healy LM, Osso LA, Zorko N, Grouza V, et al. Effects of fumarates on circulating and CNS myeloid cells in multiple sclerosis. Ann Clin Transl Neurol. (2015) 3:27–41. doi: 10.1002/acn3.270
40. Schulze-Topphoff U, Varrin-Doyer M, Pekarek K, Spencer CM, Shetty A, Sagan SA, et al. Dimethyl fumarate treatment induces adaptive and innate immune modulation independent of Nrf2. Proc Natl Acad Sci USA. (2016) 113:4777–82. doi: 10.1073/pnas.1603907113
41. Moharregh-Khiabani D. Effects of fumaric acids on cuprizone induced central nervous system de- and remyelination in the mouse. PLoS ONE. (2010) 5:e11769. doi: 10.1371/journal.pone.0011769
42. Koivunen P, Hirsilä M, Remes AM, Hassinen IE, Kivirikko KI, Myllyharju J. Inhibition of HIF-1α hydroxylases by citric acid cycle intermediates: possible links between cell metabolism and stabilization of HIF-1α. J Biol Chem. (2007) 282:4524–32. doi: 10.1074/jbc.M610415200
43. Dodd MS, Sousa Fialho MDL, Montes Aparicio CN, Kerr M, Timm KN, Griffin JL, et al. Fatty acids prevent hypoxia-inducible factor-1alpha signaling through decreased succinate in diabetes. JACC Basic Transl Sci. (2018) 3:485–98. doi: 10.1016/j.jacbts.2018.04.005
44. Huang H, Taraboletti A, Shriver LP. Dimethyl fumarate modulates antioxidant and lipid metabolism in oligodendrocytes. Redox Biol. (2015) 5:169–75. doi: 10.1016/j.redox.2015.04.011
45. Gafson AR, Savva C, Thorne T, David M, Gomez-Romero M, Lewis MR, et al. Breaking the cycle: reversal of flux in the tricarboxylic acid cycle by dimethyl fumarate. Neurol Neuroimmunol Neuroinflamm. (2019) 6:e562. doi: 10.1212/NXI.0000000000000562
46. Kornberg MD. The immunologic Warburg effect: evidence and therapeutic opportunities in autoimmunity. Wiley Interdiscip Rev Syst Biol Med. (2020) 12:e1486. doi: 10.1002/wsbm.1486
47. Kornberg MD, Bhargava P, Kim PM, Putluri V, Snowman AM, Putluri N, et al. Dimethyl fumarate targets GAPDH and aerobic glycolysis to modulate immunity. Science. (2018) 360:449–53. doi: 10.1126/science.aan4665
48. Herrmann A, Wüllner V, Moos S, Graf J, Chen J, Kieseier B, et al. Dimethyl fumarate alters intracellular Ca 2+ handling in immune cells by redox-mediated pleiotropic effects. Free Radic Biol Med. (2019) 141:338–47. doi: 10.1016/j.freeradbiomed.2019.07.005
49. Arts RJ, Novakovic B, Horst RT, Carvalho A, Bekkering S, Lachmandas E, et al. Glutaminolysis and fumarate accumulation integrate immunometabolic and epigenetic programs in trained immunity. Cell Metab. (2016) 24:807–19. doi: 10.1016/j.cmet.2016.10.008
50. Blewett MM, Xie J, Zaro BW, Backus KM, Altman A, Teijaro JR, et al. Chemical proteomic map of dimethyl fumarate-sensitive cysteines in primary human T cells. Sci Signal. (2016) 9:rs10. doi: 10.1126/scisignal.aaf7694
51. Schmidt TJ, Ak M, Mrowietz U. Reactivity of dimethyl fumarate and methylhydrogen fumarate towards glutathione and N-acetyl-L-cysteine–preparation of S-substituted thiosuccinic acid esters. Bioorg Med Chem. (2007) 15:333–42. doi: 10.1016/j.bmc.2006.09.053
52. Schmidt MM, Dringen R. Fumaric acid diesters deprive cultured primary astrocytes rapidly of glutathione. Neurochem Int. (2010) 57:460–7. doi: 10.1016/j.neuint.2010.01.006
53. Held KD, Epp ER, Awad S, Biaglow JE. Postirradiation sensitization of mammalian cells by the thiol-depleting agent dimethyl fumarate. Radiat Res. (1991) 127:75–80. doi: 10.2307/3578091
54. Dethlefsen LA, Lehman CM, Biaglow JE, Peck VM. Toxic effects of acute glutathione depletion by buthionine sulfoximine and dimethylfumarate on murine mammary carcinoma cells. Radiat Res. (1988) 114:215–24. doi: 10.2307/3577219
55. Dibbert S, Clement B, Skak-Nielsen T, Mrowietz U, Rostami-Yazdi M. Detection of fumarate-glutathione adducts in the portal vein blood of rats: evidence for rapid dimethylfumarate metabolism. Arch Dermatol Res. (2013) 305:447–51. doi: 10.1007/s00403-013-1332-y
56. Lin SX, Lisi L, Dello Russo C, Polak PE, Sharp A, Weinberg G, et al. The anti-inflammatory effects of dimethyl fumarate in astrocytes involve glutathione and haem oxygenase-1. ASN Neuro. (2011) 3:e00055. doi: 10.1042/AN20100033
57. Hoffmann C, Dietrich M, Herrmann AK, Schacht T, Albrecht P, Methner A. Dimethyl fumarate induces glutathione recycling by upregulation of glutathione reductase. Oxid Med Cell Longev. (2017) 2017:6093903. doi: 10.1155/2017/6093903
58. Checconi P, Limongi D, Baldelli S, Ciriolo MR, Nencioni L, Palamara AT. Role of glutathionylation in infection and inflammation. Nutrients. (2019) 11:1952. doi: 10.3390/nu11081952
59. Lutz W, Tarkowski M, Nowakowska E. Polimorfizm genetyczny s-transferaz glutationowych jako czynnik predysponujacy do wystapienia alergii skórnej [Genetic polymorphism of glutathione s-transferase as a factor predisposing to allergic dermatitis]. Med Pr. (2001) 52:45–51. doi: 10.1034/j.1600-0536.2001.045001052.x
60. Hsu CH, Chua KY, Huang SK, Chiang IP, Hsieh KH. Glutathione-S-transferase induces murine dermatitis that resembles human atopic dermatitis. Clin Exp Allergy. (1996) 26:1329–37. doi: 10.1046/j.1365-2222.1996.d01-279.x
61. Speeckaert R, Dugardin J, Lambert J, Lapeere H, Verhaeghe E, Speeckaert MM, et al. Critical appraisal of the oxidative stress pathway in vitiligo: a systematic review and meta-analysis. J Eur Acad Dermatol Venereol. (2018) 32:1089–98. doi: 10.1111/jdv.14792
62. Campione E, Mazzilli S, Lanna C, Cosio T, Palumbo V, Cesaroni G, et al. The effectiveness of a new topical formulation containing GSH-C4 and hyaluronic acid in seborrheic dermatitis: preliminary results of an exploratory pilot study. Clin Cosmet Investig Dermatol. (2019) 12:881–5. doi: 10.2147/CCID.S231313
63. Kökçam I, Naziroglu M. Antioxidants and lipid peroxidation status in the blood of patients with psoriasis. Clin Chim Acta. (1999) 289:23–31. doi: 10.1016/S0009-8981(99)00150-3
64. Fairris GM, Lloyd B, Hinks L, Perkins PJ, Clayton BE. The effect of supplementation with selenium and vitamin E in psoriasis. Ann Clin Biochem. (1989) 26(Pt. 1):83–8. doi: 10.1177/000456328902600113
65. Rostami-Yazdi M, Clement B, Schmidt TJ, Schinor D, Mrowietz U. Detection of metabolites of fumaric acid esters in human urine: implications for their mode of action. J Invest Dermatol. (2009) 129:231–4. doi: 10.1038/jid.2008.197
66. Peterson JD, Herzenberg LA, Vasquez K, Waltenbaugh C. Glutathione levels in antigen-presenting cells modulate Th1 versus Th2 response patterns. Proc Natl Acad Sci USA. (1998) 95:3071–6. doi: 10.1073/pnas.95.6.3071
67. Ghoreschi K, Brück J, Kellerer C, Deng C, Peng H, Rothfuss O, et al. Fumarates improve psoriasis and multiple sclerosis by inducing type II dendritic cells. J Exp Med. (2011) 208:2291–303. doi: 10.1084/jem.20100977
68. Ricceri F, Bardazzi F, Buggiani G, Burlando M, Campione E, Corazza M, et al. Efficacy and safety of dimethylfumarate in elderly psoriasis patients: a multicentric Italian study. J Dermatolog Treat. (2021) 1–4. doi: 10.1080/09546634.2021.1962000
69. Mazzilli S, Cosio T, Botti E, Petruzzellis A, Lanna C, Diluvio L, et al. Dimethylfumarate efficacy and safety in palmoplantar psoriasis patient affected by hepatitis b and depression: a case report. Dermatol Ther. (2020) 33:e13659. doi: 10.1111/dth.13659
70. Nast A, Gisondi P, Ormerod AD, Saiag P, Smith C, Spuls PI, et al. European S3-Guidelines on the systemic treatment of psoriasis vulgaris-Update 2015-Short version-EDF in cooperation with EADV and IPC. J Eur Acad Dermatol Venereol. (2015) 29:2277–94. doi: 10.1111/jdv.13354
71. Reich K, Mrowietz U, Radtke MA, Thaci D, Rustenbach SJ, Spehr C, et al. Drug safety of systemic treatments for psoriasis: results from The German Psoriasis Registry PsoBest. Arch Dermatol Res. (2015) 307:875–83. doi: 10.1007/s00403-015-1593-8
72. Reich K, Thaci D, Mrowietz U, Kamps A, Neureither M, Luger T. Efficacy and safety of fumaric acid esters in the long-term treatment of psoriasis–a retrospective study (FUTURE). J Dtsch Dermatol Ges. (2009) 7:603–11. doi: 10.1111/j.1610-0387.2009.07120.x
73. Weisenseel P, Reich K, Griemberg W, Merten K, Gröschel C, Gomez NN, et al. Efficacy and safety of fumaric acid esters in combination with phototherapy in patients with moderate-to-severe plaque psoriasis (FAST). J Dtsch Dermatol Ges. (2017) 15:180–6. doi: 10.1111/ddg.12837
74. Tzaneva S, Geroldinger A, Trattner H, Tanew A. Fumaric acid esters in combination with a 6-week course of narrowband ultraviolet B provides an accelerated response compared with fumaric acid esters monotherapy in patients with moderate-to-severe plaque psoriasis: a randomized prospective clinical study. Br J Dermatol. (2018) 178:682–8. doi: 10.1111/bjd.16106
75. Wilsmann-Theis D, Frambach Y, Philipp S, Weyergraf AJ, Jacobi A, Mössner R, et al. Systemic antipsoriatic combination therapy with fumaric acid esters for plaque-type psoriasis: report on 17 cases. Dermatology. (2015) 230:119–27. doi: 10.1159/000367890
76. Hoefnagel JJ, Thio HB, Willemze R, Bouwes Bavinck JN. Long-term safety aspects of systemic therapy with fumaric acid esters in severe psoriasis. Br J Dermatol. (2003) 149:363–9. doi: 10.1046/j.1365-2133.2003.05433.x
77. Teklu M, Zhou W, Kapoor P, Patel N, Dey AK, Sorokin AV, et al. Metabolic syndrome and its factors are associated with noncalcified coronary burden in psoriasis: an observational cohort study. J Am Acad Dermatol. (2021) 84:1329–38. doi: 10.1016/j.jaad.2020.12.044
78. Campione E, Cosio T, Di Prete M, Lanna C, Dattola A, Bianchi L. Experimental pharmacological management of psoriasis. J Exp Pharmacol. (2021) 13:725–37. doi: 10.2147/JEP.S265632
Keywords: comorbidities, dimethyl fumarate, glutathione-S-transferase, psoriasis, pathway
Citation: Campione E, Mazzilli S, Di Prete M, Dattola A, Cosio T, Lettieri Barbato D, Costanza G, Lanna C, Manfreda V, Gaeta Schumak R, Prignano F, Coniglione F, Ciprani F, Aquilano K and Bianchi L (2022) The Role of Glutathione-S Transferase in Psoriasis and Associated Comorbidities and the Effect of Dimethyl Fumarate in This Pathway. Front. Med. 9:760852. doi: 10.3389/fmed.2022.760852
Received: 18 August 2021; Accepted: 05 January 2022;
Published: 08 February 2022.
Edited by:
Anupam Mitra, University of California, Davis, United StatesReviewed by:
Irina Khamaganova, Pirogov Russian National Research Medical University, RussiaNehal Mehta, National Institutes of Health Clinical Center (NIH), United States
Copyright © 2022 Campione, Mazzilli, Di Prete, Dattola, Cosio, Lettieri Barbato, Costanza, Lanna, Manfreda, Gaeta Schumak, Prignano, Coniglione, Ciprani, Aquilano and Bianchi. This is an open-access article distributed under the terms of the Creative Commons Attribution License (CC BY). The use, distribution or reproduction in other forums is permitted, provided the original author(s) and the copyright owner(s) are credited and that the original publication in this journal is cited, in accordance with accepted academic practice. No use, distribution or reproduction is permitted which does not comply with these terms.
*Correspondence: Elena Campione, elena.campione@uniroma2.it