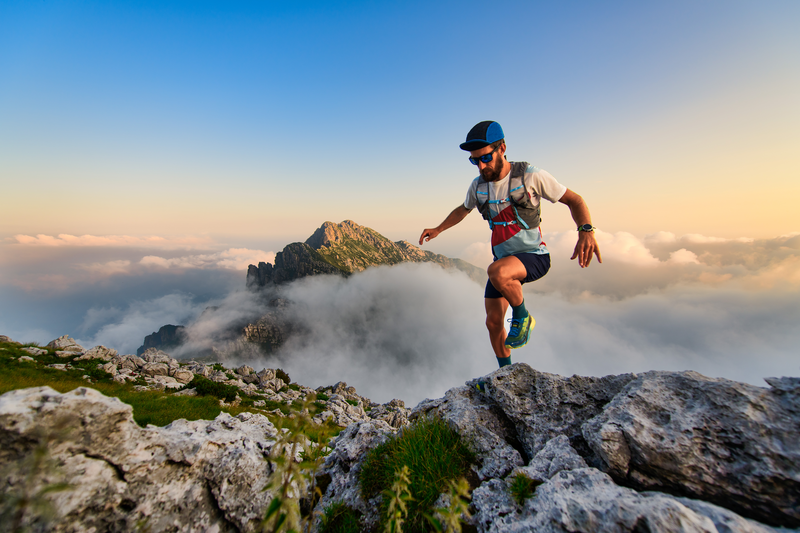
95% of researchers rate our articles as excellent or good
Learn more about the work of our research integrity team to safeguard the quality of each article we publish.
Find out more
ORIGINAL RESEARCH article
Front. Med. , 23 February 2022
Sec. Translational Medicine
Volume 9 - 2022 | https://doi.org/10.3389/fmed.2022.754341
This article is part of the Research Topic Applications of Flow Cytometry in Clinical and Experimental Medicine View all 6 articles
Both interleukin (IL)-7 and human periodontal ligament cells (hPDLCs) have immunomodulatory properties. However, their combined effect on CD4+T cells has never been studied. In this study, we aimed to investigate the effect of conditioned medium of hPDLCs treated with rhIL-7 on the differentiation of CD4+T cells into regulatory T cells/T helper 17 cells (Treg/Th17 cells) and observe the effect of IL-7 on the immunomodulatory properties of PDLCs. After hPDLCs were treated with different concentrations of rhIL-7 for 24 h, the collected supernatants were used to incubate CD4+T cells for 3 days. A gamma-secretase inhibitor (DAPT) was used to suppress the activation of the Notch1 signaling pathway. Cell proliferation, apoptosis, and necrosis were determined using the cell counting kit-8 (CCK-8) and flow cytometry (FCM). The expressions of forkhead box P3 (Foxp3) in CD4+T cells and transforming growth factor (TGF-β) and IL-6 in the supernatants were determined by ELISA. Reverse transcription-quantitative PCR (RT-qPCR), and the Western blot (WB) determined the mRNA levels and protein expression of various target factors. FCM was used to detect the mean fluorescence intensity of PD-L1 in hPDLCs and to analyze the differentiation of Treg/Th17 cells. Our results showed that IL-7 promoted proliferation and inhibited apoptosis in hPDLCs, promoted the expression of TGF-β, PD-L1, Notch1, Jagged1, and Hes1, and inhibited the levels of hypoxia-inducible factor (HIF)-1α and TCF7, whereas the addition of DAPT effectively reversed these effects. Importantly, we found that the conditioned medium of hPDLCs treated with rhIL-7 promoted the polarization of CD4+T cells into Treg cells but had no significant effect on the differentiation of Th17 cells. Our study indicated that treatment of PDLCs with IL-7 can promote the polarization of CD4+T cells into Treg cells by modulating the expression of inflammatory factors and signaling molecules through activating the Notch1 signaling pathway, thus participating in the regulation of immune homeostasis in the periodontal microenvironment.
The periodontal ligament cells (PDLCs) are the most abundant and powerful cells in the periodontal ligament. They are a heterogeneous cell group comprising fibroblasts, mesenchymal stem cells, and precursor cells, which can differentiate into osteoblasts and odontoblasts (1). These cells are characterized by self-renewal, multidirectional differentiation, and immune regulation and play an important role in regeneration and repair, functional reconstruction, and steady-state maintenance of periodontal tissues (2–4). Fibroblast-like cells in the PDLCs are involved in tissue regeneration and repair through cell proliferation and differentiation into tissue-specific cells and regulation of the immune-inflammatory response. PDLCs play their immunomodulatory role by releasing enzymes and soluble factors and by direct contact between cells, affecting various immune cells and participating in the regulation of immune responses in the local periodontal microenvironment (4, 5). The main key factors mediating these immunomodulatory effects are transforming growth factor (TGF-β), interleukin (IL)-6, and PD-L1 (6). The immunoregulatory factors expressed by PDLCs require activation by CD4+T cells to participate in the regulation of periodontal local inflammatory response and osteoclastic activity (7, 8). Therefore, the effect of PDLCs on CD4+T cells is the current focus of research (9).
After receiving external signal stimulation, naive CD4+T cells can differentiate into different subtypes of functional cells, such as T helper cells Th1, Th2, Th17, or regulatory T (Treg) cells. They also participate in the body's immune response and regulate the regeneration and destruction of bone tissue (10). Th17 cells help in promoting inflammatory responses and bone resorption by secreting pro-inflammatory factors, such as IL-17; Treg cells, as a highly immunosuppressive subgroup of CD4+T cells, inhibit the immune response and participate in bone formation by promoting the expression of anti-inflammatory factors, such as TGF-β and IL-10 (11, 12). Treg/Th17 cells antagonize, regulate, and transform through common signaling and metabolic pathways. Therefore, the differentiation and balance of Treg/Th17 cells are important in the occurrence and development of inflammatory responses and to regulate the homeostasis of the local periodontal immune microenvironment and bone metabolism balance (13).
Interleukin-7 is a lymphocyte factor with several immune effects that can regulate the differentiation and survival of CD4+T cells and is crucial for regulating T-cell metabolism (14). The IL-7 expression has been found in both periodontitis and orthodontic tooth movement (15, 16). IL-7 directly acting on CD4+T cells can induce differentiation into Th1 cells by activating the JAK/STAT5 signaling pathway (17). However, IL-7 has also been shown to regulate autoimmune responses and rebuild and maintain immune tolerance by inducing differentiation and proliferation of Treg cells in mouse models of autoimmune diseases and patients with type 1 diabetes (18, 19). In addition, IL-7 can participate in tissue repair by enhancing the fusion characteristics of rat bone marrow mesenchymal stem cells, promoting their proliferation, differentiation, and migration ability, and inducing the secretion of pro-regeneration factors (20, 21).
However, despite being an important immunomodulatory factor produced by PDLCs during periodontal tissue inflammation, the effects of IL-7 on the immunomodulatory function of PDLCs and their combined effect on CD4+T cells are unclear. Therefore, this study aimed to determine the changes in the expression of inflammatory cytokines and signal molecules related to the immune response of hPDLCs after treatment with IL-7 and to investigate their combined effect on the polarization of CD4+T cells into Treg/Th17 cells and the possible underlying mechanism.
All protocols were performed after the approval by the Ethical Committee of Southern Medical University and with the informed consent of all volunteers.
Extracted teeth needed for the primary culture of hPDLCs were provided by the surgical outpatient department of the Stomatological Hospital of Southern Medical University. Periodontally healthy permanent premolars without root caries that required extraction for orthodontic treatment were obtained from donors aged 16–20 years. Primary hPDLC isolation was performed as described previously (22). The periodontium from the middle one-third of the root surface of each premolar was scraped, and 2 mg/ml collagenase I (Sigma, Germany) was used for its digestion at 37°C for 30 min. Next, periodontium pieces were collected by centrifugation and resuspended with RPMI-1640 (Gibco, USA) containing 10% fetal bovine serum (FBS; Gibco, USA) and 1% penicillin/streptomycin (Gibco, USA). The culture medium was changed every 2 days, and the hPDLCs of the 3rd to 5th generations were used for further experiments. Before the experiment, hPDLCs were characterized by immunofluorescence for the characteristic marker fibromodulin, as described previously (8).
Study participants were three volunteer laboratory members. As previously described (23), the density-gradient centrifugation method isolated human peripheral blood mononuclear cells (hPBMCs) from donated blood. We diluted 15 ml anticoagulant blood and 15 ml RPMI-1640 in a 1:1 ratio, and then 30 ml diluted blood was slowly added to the top of 15 ml lymphocyte separation solution (TBD, China). The PBMC layer was carefully extracted after centrifugation. Next, the cells were suspended in RPMI-1640 and centrifuged again. PBMCs were collected and resuspended in 1640 medium containing 1% FBS for cell sorting. Naive CD4+T cells from PBMCs were isolated using the Immunomagnetic Negative Selection Kit (STEMCELL, Canada) and seeded in 24-well plates using 1 × 106 cells per well in 1 ml medium. Naive CD4+T cells were activated with 20 ng/ml IL-2 (PeproTech, USA) and 12 μL/ml CD3/CD28 (STEMCELL, Canada) for 3 days.
About 1 × 105 hPDLCs were seeded in 6-well plates, and a fresh medium was introduced and supplemented with different concentrations of rhIL-7 (PeproTech, USA) after 24 h; cultivating was continued for another 24 h. Cells in the inhibitor (DAPT) group are pre-treated for 2 h at 37°C with 100 μM DAPT (MedChemExpress, USA) and then replaced with fresh medium supplemented with 200 ng/ml rhIL-7. The supernatants were then collected and used to incubate activated CD4+T cells for 3 days for subsequent experiments. CD4+T cells in the negative control group were cultured in an unconditioned medium, whereas cells in the control group were cultured in a conditioned medium of hPDLCs without rhIL-7-treated.
The supernatants of hPDLCs from each group were used to incubate with CD4+T cells for 3 days. The cells were collected, and a 2 μL/ml cell activation cocktail (with Brefeldin A) (BioLegend, USA) was added. Every 100 μl of this cell activation cocktail contains phorbol-12-myristate 13-acetate (40.5 μM), ionomycin (669.3 μM), and Brefeldin A (2.5 mg/mL) in dimethyl sulfoxide (DMSO) (×500). The cells were then cultured for 4–6 h and centrifuged to collect the activated cells. We added 300 μl of phosphate-buffered saline (PBS)-resuspended cells, 2 μl fluorescein isothiocyanate (FITC) anti-human CD4 (BioLegend, USA), 2 μl PE anti-human CD25 (BioLegend, USA), and 2 μl allophycocyanin (APC) anti-human CD127 (BioLegend, USA) for staining. After staining, the cells were incubated for 30 min. The cell precipitates were collected by centrifugation. The cells were resuspended in 100 μl fixation solution (BioLegend, USA), vortex oscillated for 30 s, and incubated for 30 min. Next, a 2-ml permeabilization buffer (BioLegend, USA) was added. The solution was centrifuged to collect the cell precipitates; 100 μl permeabilization buffer was added to resuspend the cells, and 2 μl Brilliant Violet 421 Anti-Human IL-17A (BioLegend, USA) was added for staining. The cells were then incubated for 60 min. The PBS solution was added to maintain a constant volume of 300 μL, and the ratio of CD4+ IL-17A+ Th17 cells and CD4+ CD25+ CD127− Treg cells was determined by flow cytometry (FCM).
After digestion with 0.25% trypsin (Gibco, USA), the hPDLC precipitates in each group were collected by centrifugation. The cells were resuspended in 300 μl binding buffer after rinsing with PBS twice. The hPDLCs were stained with Annexin V-FITC/PI (KeyGEN Biotech, Nanjing, China) and incubated under dark conditions for 15 min. The apoptotic necrosis of hPDLCs was assessed by FCM.
The hPDLC precipitates from each group were collected by centrifugation after digestion with 0.25% trypsin (Gibco, USA); the cells were rinsed and resuspended in PBS. Next, 2 μl PE anti-human CD274 (BioLegend, USA) was added for staining and incubated for 30 min. The mean fluorescence intensity (MFI) of PD-L1 in hPDLCs was determined by FCM. Data were collected using FACSCalibur (BD LSRFortessa X20, USA), and the FlowJo software (San Carlos, CA. USA) was used for further analysis.
Briefly, 1 × 104 hPDLCs were seeded in 96-well plates for 24 h, a fresh medium was introduced and supplemented with rhIL-7 at different concentrations and cultured for 24 h. Next, CCK-8 was added (Dojindo, Japan) to each well and incubated at 37°C for 1 h in dark conditions. The absorbance was recorded at 450 nm.
After hPDLCs were treated with different concentrations of rhIL-7 for 24 h, the supernatants of each well were collected, the concentration of TGF-β and IL-6 was determined using an ELISA Kit (Meimian, Wuhan, China), and optical density (OD) value was measured by an enzyme label instrument at a wavelength of 450 nm. Then, the sample OD values measured by different detection indexes were substituted into the corresponding standard curve regression equation to calculate the actual sample concentration.
After incubated in a conditioned medium of hPDLCs for 3 days, CD4+T cells were collected and lysed with radioimmunoprecipitation assay (RIPA) buffer containing 0.1 mM phenylmethylsulfonyl fluoride (PMSF) reagent and 2% phosphatase inhibitor cocktail (Beyotime, Shanghai, China). Homogenates were centrifuged at 14,000 × g for 10 min at 4°C, and the collected supernatants were used to detect the concentration of Foxp3 using an ELISA Kit (Meimian, Wuhan, China).
The hPDLCs were collected after being treated with different concentrations of rhIL-7 for 24 h. Following the instructions of the manufacturer, total RNA was isolated from hPDLCs using the TRIzol reagent (Thermo Fisher Scientific, USA). Total RNA was reverse-transcribed to complementary cDNA using Servicebio® RT First-Strand cDNA Synthesis Kit (Servicebio, Wuhan, China) according to the instructions of the manufacturer. Target genes were amplified in SYBR Green qPCR Master Mix (Servicebio, Wuhan, China) using ABI 7900 (Applied Biosystems, USA). Considering glyceraldehyde-3-phosphate dehydrogenase (GAPDH) as a reference gene, the relative gene expression of hypoxia-inducible factor (HIF)-1α, TCF7, Notch1, Jagged1, and Hes1 was quantified using the 2−ΔΔCt method. Details of the primer sequences are listed in Table 1.
The hPDLCs were rinsed with pre-cooled PBS and then lysed with the RIPA buffer containing 0.1 mM PMSF reagent and 2% phosphatase inhibitor cocktail (Beyotime, Shanghai, China). The hPDLCs were scraped off with a scraper after being lysed on ice for 15 min, and the supernatants were then collected after the homogenates were centrifuged at 4°C at 14,000 × g for 10 min. Protein concentrations were measured with an enhanced BCA Protein Assay Kit (Beyotime, Shanghai, China). Protein was mixed with 5× sample loading buffer (Beyotime, Shanghai, China) and boiled at 99°C for 5 min. Equal amounts of protein samples were separated by sodium dodecyl sulfate polyacrylamide gel electrophoresis (SDS-PAGE; Epizyme, Shanghai, China) for electrophoresis and then transferred to polyvinylidene fluoride (PVDF) membranes (Millipore, USA). The PageRuler™ Prestained Protein Ladder (10–180 kDa, Thermo Fisher Scientific, USA) was used to help locate and separate the target proteins. After blocking with 5% BSA (Meilunbio, Dalian, China) for 1 h, the membrane was incubated with primary antibodies of GAPDH (1:2,000, Proteintech, Wuhan, China), TGF-β1 (1:1,000, ABclonal, Wuhan, China), IL-6 (1:2,000, Proteintech, Wuhan, China), PD-L1 (1:2,000, Proteintech, Wuhan, China), Notch1 (1:600, Proteintech, Wuhan, China), Jagged1 (1:2,000, Proteintech, Wuhan, China), Hes1 (1:1,000, ABclonal, Wuhan, China), HIF-1α (1:2,000, Proteintech, Wuhan, China), and TCF7 (1:600, Proteintech, Wuhan, China) overnight at 4°C. The antibodies mentioned above were diluted with primary antibody dilution buffer (Servicebio, Wuhan, China). The membrane was incubated with an anti-rabbit or anti-mouse secondary antibody (1:5,000, Proteintech, Wuhan, China) diluted with secondary antibody dilution (Servicebio, Wuhan, China) for 1 h at room temperature after being washed with TBST. Then, protein bands were detected with ECL luminescence reagent (Meilunbio, Dalian, China). The protein level indicated by band intensity was quantitatively analyzed by the ImageJ software (National Institutes of Health, Bethesda, MD, USA).
Statistical analysis was performed using the IBM SPSS 20.0 (IBM Corp., Armonk, NY, USA) software. All data are shown as mean ± SD. Statistical significance was determined using Tamhane's T2 test, one-way ANOVA, or the Kruskal–Wallis test. A p-value < 0.05 was considered statistically significant.
We investigated the effect of a conditioned medium of hPDLCs treated with rhIL-7 on CD4+T-cell differentiation. The results showed that CD4+T cells cultured with supernatants of hPDLCs treated with rhIL-7 significantly upregulated the proportion of Treg cells. However, the proportion of Treg cells in the DAPT group was significantly reduced compared with the 200 ng/ml rhIL-7 group (Figures 1A,B). Although the proportion of Th17 cells decreased slightly, there was no statistical difference compared with the control group (Figures 1D,E). The concentration of Foxp3 in CD4+T cells and the ratio of Treg/Th17 showed the same trend as the proportion of Treg cells (Figures 1C,F).
Figure 1. Effects of conditioned medium of human periodontal ligament cells (hPDLCs) treated with rhIL-7 on the differentiation of Treg/Th17 cells. The supernatants of hPDLCs were collected after being treated with different concentrations of rhIL-7 for 24 h and used to culture CD4+T cells for 3 days. Flow cytometry (FCM) was used to detect the polarization of CD4+T cells into Treg cells (A,B) and Th17 cells (D,E). (C) The concentration of Foxp3 in CD4+T cells was detected by ELISA. (F) The ratio of Treg/Th17. Representative dot plots show the gating strategy of FCM analysis. n = 3, *p < 0.05. **p < 0.01. ##DAPT group compared with 200 ng/mL rhIL-7 group, p < 0.01.
We extracted primary hPDLCs for culture (Figure 2A). Our results showed that the presence of rhIL-7 at different concentrations significantly promoted the proliferation of hPDLCs (Figure 2B) and significantly reduced the proportion of apoptotic cells. The presence of rhIL-7 at higher concentrations also significantly downregulated the proportion of necrotic cells (Figures 2C,D), indicating that IL-7 could promote proliferation and inhibit the apoptotic necrosis of hPDLCs.
Figure 2. Primary human periodontal ligament cells. (A) Under the microscope, it can be seen that many fibroblast cells are crawling out around the dark brown primary tissue block. The cells are adherent to the wall and grow with a clear outline. Most of the cells are flat and spindle-shaped or star-shaped structures arranged in radial or whirlpool shape. Effect of rhIL-7 on the proliferation of hPDLCs. Scale bar = 1,000 μm. (B) After hPDLCs were treated with different concentrations of rhIL-7 for 24 h, CCK-8 was used to detect the cell survival rate of the hPDLCs. A summary of the results is shown in the bar graphs. Effect of rhIL-7 on the apoptosis and necrosis of hPDLCs. (C,D) We used Annexin V-FITC/PI staining to detect the proportion of apoptosis and necrosis in the cells of each group by FCM. Representative dot plots show the gating strategy of FCM analysis. The number in the scatter plot represents the percentage of cells in the corresponding state. n = 3, *p < 0.05. **p < 0.01. CCK-8, cell counting kit-8; FCM, flow cytometry; hPDLCs, human periodontal ligament cells.
We speculated that IL-7 could affect the expression of immunomodulatory cytokines secreted by hPDLCs, thus further influencing the differentiation process of CD4+T cells. Therefore, we further studied the effects of rhIL-7 on hPDLCs and inflammatory factors and signaling molecules related to the immune response. The results of ELISA (Figure 3A) and WB (Figures 3B,C) showed that in the presence of rhIL-7, the concentration and protein level of TGF-β in the supernatants were significantly upregulated and significantly higher than that of IL-6. The addition of DAPT significantly inhibited the expression of TGF-β compared with the 200 ng/ml rhIL-7 group. The concentration and protein level of IL-6 decreased slightly but were already low, and no obvious change was noted. FCM (Figures 4A,B) and WB (Figures 4C,D) detection showed that in the presence of rhIL-7, the expression and protein level of PD-L1 on hPDLCs were significantly upregulated, whereas the DAPT group decreased significantly. RT-qPCR and WB analysis showed that in the presence of rhIL-7, the mRNA (Figure 5A) and protein levels (Figures 5B,C) of HIF-1α and TCF7 in hPDLCs were significantly decreased, whereas the addition of DAPT increased their expression.
Figure 3. Effect of rhIL-7 on inflammatory factors related to the immune response in hPDLCs. (A–C) After hPDLCs were treated with different concentrations of rhIL-7 for 24 h, the concentration and protein level of TGF-β and IL-6 in the supernatants of each group were detected by ELISA and WB. n = 3, *p < 0.05. **p < 0.01. #DAPT group compared with 200 ng/mL rhIL-7 group, p < 0.05. hPDLCs, human periodontal ligament cells; TGF, transforming growth factor; WB, Western blot.
Figure 4. Effects of rhIL-7 on the expression PD-L1 in hPDLCs. (A–D) After hPDLCs were treated with different concentrations of rhIL-7 for 24 h, the MFI and the protein level of PD-L1 in each group were determined by FCM and WB. Representative histograms show the gating strategy of FCM analysis. n = 3, *p < 0.05. **p < 0.01. ##DAPT group compared with 200 ng/mL rhIL-7 group, p < 0.01. FCM, flow cytometry; hPDLCs, human periodontal ligament cells; MFI, mean fluorescence intensity; WB, Western blot.
Figure 5. Effect of rhIL-7 on signal molecules related to the immune response in hPDLCs. (A–C) The mRNA and protein levels of HIF-1α and TCF7 in hPDLCs were detected by RT-qPCR and WB after treatment with different concentrations of IL-7 for 24 h. A summary of the results is shown in the bar graphs. n = 3. **p < 0.01. #DAPT group compared with 200 ng/mL rhIL-7 group, p < 0.05. ##DAPT group compared with 200 ng/mL rhIL-7 group, p < 0.01. HIF, hypoxia-inducible factor; hPDLCs, human periodontal ligament cells; RT-qPCR, reverse transcription quantitative PCR; WB, Western blot.
The results of RT-qPCR (Figure 6A) and WB (Figures 6B,C) demonstrated that in the presence of IL-7, the mRNA and protein levels of Notch1, Jagged1, and Hes1 in hPDLCs were increased, and the addition of DAPT inhibited their expression compared with the 200 ng/ml rhIL-7 group.
Figure 6. Effects of treatment with different concentrations of rhIL-7 in hPDLCs on Notch1 signaling pathway-related molecules. (A–C) After hPDLCs were treated with different concentrations of IL-7 for 24 h, the mRNA level and protein level of Notch1, Jagged1, and Hes1 were detected by RT-qPCR and WB. n = 3, *p < 0.05. **p < 0.01. ##DAPT group compared with 200 ng/mL rhIL-7 group, p < 0.01. hPDLCs, human periodontal ligament cells; RT-qPCR, reverse transcription quantitative PCR; WB, Western blot.
In this study, treatment of hPDLCs with IL-7 promoted cell proliferation and inhibited cell apoptosis, upregulated the expression of TGF-β and PD-L1 and inhibition of the expression of HIF-1α and TCF7 by activating the Notch1 signaling pathway, and promoted the polarization of CD4+T cells into Treg cells, thus regulating immune homeostasis in the periodontal microenvironment.
Interleukin-7 is an important cytokine of the immune system that plays a key role in maintaining T-cell homeostasis. IL-7 can promote the survival of Treg cells and maintain its immunosuppressive effect by upregulating the expression of Foxp3 (24, 25); nevertheless, this is contrary to previous research (17). IL-7 is also involved in periodontitis and orthodontic tooth movement by inhibiting the osteogenic differentiation of periodontal ligament stem cells through the mitogen-activated protein kinase pathway (26). CD4+T cells play a significant role in periodontitis and orthodontic tooth movement. Their function and differentiation can directly affect the local periodontal immune microenvironment and regulate the inflammatory response and osteogenic or osteoclastic activities. Local Treg/Th17 imbalance in periodontitis can promote inflammatory responses and can aggravate the absorption and destruction of alveolar bone (27). The present study showed that a conditioned medium of hPDLCs treated with rhIL-7 could induce Treg-cell differentiation, but had no significant effect on the differentiation of Th17 cells, indicating that IL-7 treatment could promote the immunosuppressive ability of PDLCs and participate in the maintenance of periodontal immune homeostasis by influencing T-cell differentiation.
Interleukin-7 plays an anti-apoptotic and pro-proliferative role by regulating the proteins of the Bcl-2 family to promote cell cycle progression and inhibit cell autophagy (28–30). The results of our study showed that rhIL-7 could promote the proliferation of hPDLCs and inhibit their apoptosis and necrosis. Our results are consistent with the biology of IL-7, which is consistent with the results of previous studies.
Both Th17 cells and Treg cells require the presence of a TGF-β signal in the initial differentiation stage. However, IL-6 can induce the polarization of Th17 cells by phosphorylation and activation of Stat3 and downregulate the expression of Foxp3 to inhibit the differentiation of Treg cells (31). TGF-β is a key regulatory factor of immune response and growth factor, which has a significant immunosuppressive effect, can promote the expression of Foxp3, promote the proliferation and differentiation of Treg cells, and maintain its immunosuppressive function (32). In periodontal tissues, TGF-β induces the expression of osteoblast differentiation proteins, such as ALP, and pro-regeneration factors, such as α-SMA (33, 34), by promoting PDLC proliferation, enhancing the synthesis of extracellular matrix protein of PDLCs and osteoblast differentiation to participate in alveolar bone remodeling and periodontal tissue remodeling, which are crucial processes for maintaining the immune homeostasis of the periodontal microenvironment and also tissue repair and regeneration (35, 36). In this study, IL-7 was found to significantly upregulate the expression of TGF-β in hPDLCs. In contrast, the IL-6 expression was low, and IL-7 had no significant effect on it, consistent with the differentiation results of CD4+T cells. Interestingly, in the absence of IL-7, the expression of TGF-β was significantly higher than that of IL-6, and the polarization ratio of Treg cells was significantly higher than that of Th17 cells, suggesting that PDLCs were involved in maintaining local immune homeostasis, and IL-7 enhanced the immunomodulatory effect of PDLCs.
Both HIF-1 and TCF7 promote inflammation by inhibiting Treg cells and inducing Th17 and Th1 cells. HIF-1α is a major transcription factor involved in hypoxia response, which can promote IL-17 expression and Th17 cell differentiation by inducing RORγt expression (37–39). HIF-1α also promotes PDLC apoptosis and inhibits osteoblastic differentiation in periodontal tissues (40–42). TCF7 plays a key role in the development and differentiation of T lymphocytes and inhibits the differentiation and maturation of Treg cells, whereas Foxp3 can inhibit the expression of TCF7 protein (43). The PD-1/PD-L1 signaling pathway is a negative feedback regulation mechanism of the immune response during the activation of T cells, in which PD-L1 is a negative costimulatory molecule (44). PD-L1 is involved in maintaining immune homeostasis by inhibiting the differentiation function of effector T cells, upregulating the expression of Foxp3, inducing differentiation of Treg cells, and enhancing their immunosuppressive ability (45). In this study, IL-7 significantly downregulated the mRNA expression levels of HIF-1α and TCF7 and significantly upregulated the expression level of PD-L1 in PDLCs. The results of our study are consistent with those of previous studies. These results suggested that IL-7 can participate in the immune regulation of periodontal microenvironment and maintain local immune homeostasis by influencing the expression of inflammatory factors and signal molecules in PDLCs.
The Notch signaling pathway is a highly conserved intercellular system that plays a decisive role in cell proliferation and differentiation (46). DAPT is a Notch-pathway-specific inhibitor. Downregulation of Notch1 and Jagged1 was accompanied by loss of bone, suggesting that the Notch1 signaling pathway plays a key regulatory role in periodontitis and bone metabolism balance (47). Activating the Notch signaling pathway in hPBMCs through Jagged1 can reduce cell senescence and cell cycle arrest, thus promoting tissue regeneration and repair (46). Furthermore, the Notch1 signaling pathway promotes the expression of TGF-β and the formation of Treg cells mediated by the upregulation of PD-L1 expression and phosphorylation of Smad3 (48–51). In addition, the Notch1 signaling pathway can enhance the proliferation, adhesion, and migration ability of PDLCs and can promote osteoblastic differentiation and angiogenesis by enhancing the expression of TGF-β, ALP, and α-SMA, thus participating in the tissue regeneration and repair (52–55). In this study, we found that IL-7 can promote the proliferation and inhibit the apoptosis of hPDLCs, upregulate the expression of PD-L1 and TGF-β, and promote the formation of Treg cells. Meanwhile, we observed a significant increase in the expression level of molecules related to the Notch1 signaling pathway. However, the addition of DAPT effectively reversed these effects. The results suggested that IL-7 may regulate the expression of immunomodulatory factors in PDLCs by activating the Notch1 signaling pathway, thus affecting the differentiation process of CD4+T cells.
In this experiment, we determined the effect of soluble mediators on Treg/Th17 cells polarization through conditioned medium application, which is the simplest method for cell interaction studies. The advantage of conditioned medium is that it can avoid the influence of target cells on conditioned cells, focus on the effect of conditioned cells on target cells, and allow observation of the activity of soluble factors (56, 57). As one of the methods of indirect co-culture, conditioned medium is very effective to observe the interaction between cells in vitro. However, compared to other indirect co-culture methods, such as transwell, conditioned medium has its limitations including nutrient deficiency, the uncontrollability of the optimal concentration and time distribution of these secretory factors, and the inability to study the chemotaxis of soluble factors on target cells (56). Therefore, it will be the focus of our next experiment to study the effect of IL-7-treated PDLCs on CD4+T cells through other co-culture methods.
In summary, we found that IL-7 acting on hPDLCs may regulate the expression of inflammatory factors and signaling molecules through the activation of the Notch1 signaling pathway, the expression changes of these immune regulatory factors further promote the differentiation of CD4+T cells into Treg cells, thus participating in the regulation of immune homeostasis in the periodontal microenvironment. TGF-β, as the endogenous cytokine of hPDLCs, seems to play a key role in regulating periodontal immune homeostasis through Notch signaling.
The self-renewing, multidirectional differentiating, and immunomodulatory characteristics of PDLCs are central to maintaining periodontal homeostasis. These properties and their high availability also reveal the potential usefulness of PDLCs as cell therapy in periodontal disease, regenerative medicine, autoimmune diseases, and inflammatory diseases (58, 59). Autologous periodontal membrane cell transplantation can be used to regenerate periodontal tissue defects (60–62). Our study suggests that PDLCs treated with IL-7 may have more immunosuppressive properties and enhanced ability of periodontal tissue regeneration and repair, which may contribute to the maintenance of periodontal tissue health in future clinical practice and the prevention and treatment of periodontal tissue defects caused by inflammation.
The raw data supporting the conclusions of this article will be made available by the authors, without undue reservation.
The studies involving human participants were reviewed and approved by Ethical Committee of Southern Medical University. The patients/participants provided their written informed consent to participate in this study.
C-FL conceived and supervised the experiments. X-YY, C-FL, and Z-QZ wrote and edited the manuscript. X-YY, J-CH, and J-YL performed the experiments. X-YY and X-PC analyzed the data. All authors contributed to the article and approved the submitted version.
This work was financially supported by the Natural Science Foundation of Guangdong Province, China (No. 2018A0303130261).
The authors declare that the research was conducted in the absence of any commercial or financial relationships that could be construed as a potential conflict of interest.
All claims expressed in this article are solely those of the authors and do not necessarily represent those of their affiliated organizations, or those of the publisher, the editors and the reviewers. Any product that may be evaluated in this article, or claim that may be made by its manufacturer, is not guaranteed or endorsed by the publisher.
We gratefully acknowledge the helpful suggestions and technical support of Prof. Sha Wu, Southern Medical University. We are also grateful to all patients who participated in this study.
The Supplementary Material for this article can be found online at: https://www.frontiersin.org/articles/10.3389/fmed.2022.754341/full#supplementary-material
1. Kaneda-Ikeda E, Iwata T, Mizuno N, Nagahara T, Kajiya M, Takeda K, et al. Periodontal ligament cells regulate osteogenesis via miR-299-5p in mesenchymal stem cells. Differentiation. (2020) 112:47–57. doi: 10.1016/j.diff.2020.01.001
2. Martinez C, Smith PC, Rodriguez JP, Palma V. Sonic hedgehog stimulates proliferation of human periodontal ligament stem cells. J Dent Res. (2011) 90:483–8. doi: 10.1177/0022034510391797
3. Tomokiyo A, Yoshida S, Hamano S, Hasegawa D, Sugii H, Maeda H. Detection, characterization, and clinical application of mesenchymal stem cells in periodontal ligament tissue. Stem Cells Int. (2018) 2018:5450768. doi: 10.1155/2018/5450768
4. Jonsson D, Nebel D, Bratthall G, Nilsson BO. The human periodontal ligament cell: a fibroblast-like cell acting as an immune cell. J Periodontal Res. (2011) 46:153–7. doi: 10.1111/j.1600-0765.2010.01331.x
5. El-Awady AR, Messer RL, Gamal AY, Sharawy MM, Wenger KH, Lapp CA. Periodontal ligament fibroblasts sustain destructive immune modulators of chronic periodontitis. J Periodontol. (2010) 81:1324–35. doi: 10.1902/jop.2010.100054
6. Behm C, Blufstein A, Gahn J, Kubin B, Moritz A, Rausch-Fan X, et al. Pleiotropic effects of vitamin D3 on CD4(+) T lymphocytes mediated by human periodontal ligament cells and inflammatory environment. J Clin Periodontol. (2020) 47:689–701. doi: 10.1111/jcpe.13283
7. D'Amico L, Roato I. The impact of immune system in regulating bone metastasis formation by osteotropic tumors. J Immunol Res. (2015) 2015:143526. doi: 10.1155/2015/143526
8. Kook SH, Jang YS, Lee JC. Human periodontal ligament fibroblasts stimulate osteoclastogenesis in response to compression force through TNF-alpha-mediated activation of CD4+ T cells. J Cell Biochem. (2011) 112:2891–901. doi: 10.1002/jcb.23205
9. Wada N, Gronthos S, Bartold PM. Immunomodulatory effects of stem cells. Periodontol 2000. (2013) 63:198–216. doi: 10.1111/prd.12024
10. Takayanagi H. Osteoimmunology: shared mechanisms and crosstalk between the immune and bone systems. Nat Rev Immunol. (2007) 7:292–304. doi: 10.1038/nri2062
11. Fasching P, Stradner M, Graninger W, Dejaco C, Fessler J. Therapeutic potential of targeting the Th17/Treg axis in autoimmune disorders. Molecules. (2017) 22:134. doi: 10.3390/molecules22010134
12. Ge N, Peng J, Yu L, Huang S, Xu L, Su Y, et al. Orthodontic treatment induces Th17/Treg cells to regulate tooth movement in rats with periodontitis. Iran J Basic Med Sci. (2020) 23:1315–22. doi: 10.22038/ijbms.2020.44437.10419
13. Gao L, Zhao Y, Wang P, Zhang L, Zhang C, Chen Q, et al. Detection of Th17/Treg cells and related factors in gingival tissues and peripheral blood of rats with experimental periodontitis. Iran J Basic Med Sci. (2017) 20:294–300. doi: 10.22038/ijbms.2017.8359
14. Lin J, Zhu Z, Xiao H, Wakefield MR, Ding VA, Bai Q, et al. The role of IL-7 in Immunity and Cancer. Anticancer Res. (2017) 37:963–7. doi: 10.21873/anticanres.11405
15. Ahuja R, Almuzian M, Khan A, Pascovici D, Dalci O, Darendeliler MA, et al. preliminary investigation of short-term cytokine expression in gingival crevicular fluid secondary to high-level orthodontic forces and the associated root resorption: case series analytical study. Prog Orthod. (2017) 18:23. doi: 10.1186/s40510-017-0177-x
16. Colucci S, Mori G, Brunetti G, Coricciati M, Pignataro P, Oranger A, et al. Interleukin-7 production by B lymphocytes affects the T cell-dependent osteoclast formation in an in vitro model derived from human periodontitis patients. Int J Immunopathol Pharmacol. (2005) 18:13–9.
17. Ding ZC, Liu C, Cao Y, Habtetsion T, Kuczma M, Pi W, et al. IL-7 signaling imparts polyfunctionality and stemness potential to CD4(+) T cells. Oncoimmunology. (2016) 5:e1171445. doi: 10.1080/2162402X.2016.1171445
18. Vignali D, Gurth CM, Pellegrini S, Sordi V, Sizzano F, Piemonti L, et al. IL-7 Mediated homeostatic expansion of human CD4+CD25+FOXP3+ regulatory T Cells after depletion with Anti-CD25 monoclonal antibody. Transplantation. (2016) 100:1853–61. doi: 10.1097/TP.0000000000001276
19. Chen X, Fang L, Song S, Guo TB, Liu A, Zhang JZ. Thymic regulation of autoimmune disease by accelerated differentiation of Foxp3+ regulatory T cells through IL-7 signaling pathway. J Immunol. (2009) 183:6135–44. doi: 10.4049/jimmunol.0901576
20. Haneef K, Ali A, Khan I, Naeem N, Jamall S, Salim A. Role of interleukin-7 in fusion of rat bone marrow mesenchymal stem cells with cardiomyocytes in vitro and improvement of cardiac function in vivo. Cardiovasc Ther. (2018) 36:e12479. doi: 10.1111/1755-5922.12479
21. Khalid RS, Khan I, Zaidi MB, Naeem N, Haneef K, Qazi RE, et al. IL-7 overexpression enhances therapeutic potential of rat bone marrow mesenchymal stem cells for diabetic wounds. Wound Repair Regen. (2019) 27:235–48. doi: 10.1111/wrr.12706
22. Kanzaki H, Chiba M, Shimizu Y, Mitani H. Periodontal ligament cells under mechanical stress induce osteoclastogenesis by receptor activator of nuclear factor kappaB ligand up-regulation via prostaglandin E2 synthesis. J Bone Miner Res. (2002) 17:210–20. doi: 10.1359/jbmr.2002.17.2.210
23. Lancioni CL, Thomas JJ, Rojas RE. Activation requirements and responses to TLR ligands in human CD4+ T cells: comparison of two T cell isolation techniques. J Immunol Methods. (2009) 344:15–25. doi: 10.1016/j.jim.2009.02.005
24. Kim GY, Ligons DL, Hong C, Luckey MA, Keller HR, Tai X, et al. An in vivo IL-7 requirement for peripheral Foxp3+ regulatory T cell homeostasis. J Immunol. (2012) 188:5859–66. doi: 10.4049/jimmunol.1102328
25. Silva SL, Albuquerque AS, Serra-Caetano A, Foxall RB, Pires AR, Matoso P, et al. Human naive regulatory T-cells feature high steady-state turnover and are maintained by IL-7. Oncotarget. (2016) 7:12163–75. doi: 10.18632/oncotarget.7512
26. Jian CX, Fan QS, Hu YH, He Y, Li MZ, Zheng WY, et al. IL-7 suppresses osteogenic differentiation of periodontal ligament stem cells through inactivation of mitogen-activated protein kinase pathway. Organogenesis. (2016) 12:183–93. doi: 10.1080/15476278.2016.1229726
27. Cafferata EA, Terraza-Aguirre C, Barrera R, Faundez N, Gonzalez N, Rojas C, et al. Interleukin-35 inhibits alveolar bone resorption by modulating the Th17/Treg imbalance during periodontitis. J Clin Periodontol. (2020) 47:676–88. doi: 10.1111/jcpe.13282
28. Oliveira ML, Akkapeddi P, Ribeiro D, Melao A, Barata JT. IL-7R-mediated signaling in T-cell acute lymphoblastic leukemia: an update. Adv Biol Regul. (2019) 71:88–96. doi: 10.1016/j.jbior.2018.09.012
29. Jiang Q, Li WQ, Aiello FB, Mazzucchelli R, Asefa B, Khaled AR, et al. Cell biology of IL-7, a key lymphotrophin. Cytokine Growth Factor Rev. (2005) 16:513–33. doi: 10.1016/j.cytogfr.2005.05.004
30. Jian M, Yunjia Z, Zhiying D, Yanduo J, Guocheng J. Interleukin 7 receptor activates PI3K/Akt/mTOR signaling pathway via downregulation of Beclin-1 in lung cancer. Mol Carcinog. (2019) 58:358–65. doi: 10.1002/mc.22933
31. Lee GR. The Balance of Th17 versus Treg Cells in Autoimmunity. Int J Mol Sci. (2018) 19:730. doi: 10.3390/ijms19030730
32. Marie JC, Letterio JJ, Gavin M, Rudensky AY. TGF-beta1 maintains suppressor function and Foxp3 expression in CD4+CD25+ regulatory T cells. J Exp Med. (2005) 201:1061–7. doi: 10.1084/jem.20042276
33. Li H, Fan J, Fan L, Li T, Yang Y, Xu H, et al. MiRNA-10b Reciprocally stimulates osteogenesis and inhibits adipogenesis partly through the TGF-beta/SMAD2 signaling pathway. Aging Dis. (2018) 9:1058–73. doi: 10.14336/AD.2018.0214
34. Fujii S, Maeda H, Tomokiyo A, Monnouchi S, Hori K, Wada N, et al. Effects of TGF-beta1 on the proliferation and differentiation of human periodontal ligament cells and a human periodontal ligament stem/progenitor cell line. Cell Tissue Res. (2010) 342:233–42. doi: 10.1007/s00441-010-1037-x
35. Choi H, Ahn YH, Kim TH, Bae CH, Lee JC, You HK, et al. TGF-beta signaling regulates cementum formation through osterix expression. Sci Rep. (2016) 6:26046. doi: 10.1038/srep26046
36. Wei Y, Ye Q, Tang Z, Tian G, Zhu Q, Gao H, et al. Calcitonin induces collagen synthesis and osteoblastic differentiation in human periodontal ligament fibroblasts. Arch Oral Biol. (2017) 74:114–22. doi: 10.1016/j.archoralbio.2016.11.014
37. Hsu TS, Lai MZ. Hypoxia-inducible factor 1alpha plays a predominantly negative role in regulatory T cell functions. J Leukoc Biol. (2018) 104:911–8. doi: 10.1002/JLB.MR1217-481R
38. Dang EV, Barbi J, Yang HY, Jinasena D, Yu H, Zheng Y, et al. Control of T(H)17/T(reg) balance by hypoxia-inducible factor 1. Cell. (2011) 146:772–84. doi: 10.1016/j.cell.2011.07.033
39. Shi LZ, Wang R, Huang G, Vogel P, Neale G, Green DR, et al. HIF1alpha-dependent glycolytic pathway orchestrates a metabolic checkpoint for the differentiation of TH17 and treg cells. J Exp Med. (2011) 208:1367–76. doi: 10.1084/jem.20110278
40. Liu Z, Guo L, Li R, Xu Q, Yang J, Chen J, et al. Transforming growth factor-beta1 and hypoxia inducible factor-1alpha synergistically inhibit the osteogenesis of periodontal ligament stem cells. Int Immunopharmacol. (2019) 75:105834. doi: 10.1016/j.intimp.2019.105834
41. Zhu J, Tang Y, Wu Q, Ji YC, Feng ZF, Kang FW. HIF-1alpha facilitates osteocyte-mediated osteoclastogenesis by activating JAK2/STAT3 pathway in vitro. J Cell Physiol. (2019) 234:21182–92. doi: 10.1002/jcp.28721
42. Song ZC, Zhou W, Shu R, Ni J. Hypoxia induces apoptosis and autophagic cell death in human periodontal ligament cells through HIF-1alpha pathway. Cell Prolif. (2012) 45:239–48. doi: 10.1111/j.1365-2184.2012.00810.x
43. Barra MM, Richards DM, Hansson J, Hofer AC, Delacher M, Hettinger J, et al. Transcription factor 7 limits regulatory T cell generation in the thymus. J Immunol. (2015) 195:3058–70. doi: 10.4049/jimmunol.1500821
44. Bardhan K, Anagnostou T, Boussiotis VA. The PD1:PD-L1/2 pathway from discovery to clinical implementation. Front Immunol. (2016) 7:550. doi: 10.3389/fimmu.2016.00550
45. Francisco LM, Salinas VH, Brown KE, Vanguri VK, Freeman GJ, Kuchroo VK, et al. PD-L1 regulates the development, maintenance, and function of induced regulatory T cells. J Exp Med. (2009) 206:3015–29. doi: 10.1084/jem.20090847
46. Luo Z, Shang X, Zhang H, Wang G, Massey PA, Barton SR, et al. Notch signaling in osteogenesis, osteoclastogenesis, and angiogenesis. Am J Pathol. (2019) 189:1495–500. doi: 10.1016/j.ajpath.2019.05.005
47. Mijailovic I, Nikolic N, Djinic A, Carkic J, Milinkovic I, Peric M, et al. The down-regulation of Notch 1 signaling contributes to the severity of bone loss in aggressive periodontitis. J Periodontol. (2020) 91:554–61. doi: 10.1002/JPER.18-0755
48. Cahill EF, Tobin LM, Carty F, Mahon BP, English K. Jagged-1 is required for the expansion of CD4+ CD25+ FoxP3+ regulatory T cells and tolerogenic dendritic cells by murine mesenchymal stromal cells. Stem Cell Res Ther. (2015) 6:19. doi: 10.1186/s13287-015-0021-5
49. Mathieu M, Cotta-Grand N, Daudelin JF, Thebault P, Labrecque N. Notch signaling regulates PD-1 expression during CD8(+) T-cell activation. Immunol Cell Biol. (2013) 91:82–8. doi: 10.1038/icb.2012.53
50. Lin CL, Huang HM, Hsieh CL, Fan CK, Lee YL. Jagged1-expressing adenovirus-infected dendritic cells induce expansion of Foxp3(+) regulatory T cells and alleviate T helper type 2-mediated allergic asthma in mice. Immunology. (2019) 156:199–212. doi: 10.1111/imm.13021
51. Asano N, Watanabe T, Kitani A, Fuss IJ, Strober W. Notch1 signaling and regulatory T cell function. J Immunol. (2008) 180:2796–804. doi: 10.4049/jimmunol.180.5.2796
52. Wang W, Feng Y, Aimaiti Y, Jin X, Mao X, Li D. TGFβ signaling controls intrahepatic bile duct development may through regulating the Jagged1-Notch-Sox9 signaling axis. J Cell Physiol. (2018) 233:5780–91. doi: 10.1002/jcp.26304
53. Long Y, Qiu S, Li S, She Y, Wang N, Chen X, et al. Effect of transforming growth factor-β1 on biological behavior of human periodontal ligament stem cells. J Chin Pract Diagn Ther. (2018) 32:1044–6. doi: 10.13507/j.issn.1674-3474.2018.11.002
54. Hansamuit K, Osathanon T, Suwanwela J. Effect of Jagged1 on the expression of genes in regulation of osteoblast differentiation and bone mineralization ontology in human dental pulp and periodontal ligament cells. J Oral Biol Craniofac Res. (2020) 10:233–7. doi: 10.1016/j.jobcr.2019.12.003
55. Osathanon T, Nowwarote N, Manokawinchoke J, Pavasant P. bFGF and JAGGED1 regulate alkaline phosphatase expression and mineralization in dental tissue-derived mesenchymal stem cells. J Cell Biochem. (2013) 114:2551–61. doi: 10.1002/jcb.24602
56. Bogdanowicz DR, Lu HH. Studying cell-cell communication in co-culture. Biotechnol J. (2013) 8:395–6. doi: 10.1002/biot.201300054
57. Dogan A, Demirci S, Apdik H, Apdik EA, Sahin F. Mesenchymal stem cell isolation from pulp tissue and co-culture with cancer cells to study their interactions. J Vis Exp. (2019) 143:1–8. doi: 10.3791/58825
58. Wang M, Xie J, Wang C, Zhong D, Xie L, Fang H. Immunomodulatory properties of stem cells in periodontitis: current status and future prospective. Stem Cells Int. (2020) 2020:9836518. doi: 10.1155/2020/9836518
59. Trubiani O, Pizzicannella J, Caputi S, Marchisio M, Mazzon E, Paganelli R, et al. Periodontal ligament stem cells: current knowledge and future perspectives. Stem Cells Dev. (2019) 28:995–1003. doi: 10.1089/scd.2019.0025
60. Amir LR, Soeroso Y, Fatma D, Sunarto H, Sulijaya B, Idrus E, et al. Periodontal ligament cell sheets and RGD-modified chitosan improved regeneration in the horizontal periodontal defect model. Eur J Dent. (2020) 14:306–14. doi: 10.1055/s-0040-1709955
61. Yoo SY, Lee JS, Cha JK, Kim SK, Kim CS. Periodontal healing using a collagen matrix with periodontal ligament progenitor cells in a dehiscence defect model in beagle dogs. J Periodontal Implant Sci. (2019) 49:215–27. doi: 10.5051/jpis.2019.49.4.215
Keywords: IL-7, periodontal ligament cells, TGF-β, Treg cells, Notch1 signaling pathway
Citation: Yu X-Y, Zhang Z-Q, Huang J-C, Lin J-Y, Cai X-P and Liu C-F (2022) IL-7-Treated Periodontal Ligament Cells Regulate Local Immune Homeostasis by Modulating Treg/Th17 Cell Polarization. Front. Med. 9:754341. doi: 10.3389/fmed.2022.754341
Received: 06 August 2021; Accepted: 17 January 2022;
Published: 23 February 2022.
Edited by:
Oleh Andrukhov, University Dental Clinic Vienna, AustriaReviewed by:
Christian Behm, University Dental Clinic Vienna, AustriaCopyright © 2022 Yu, Zhang, Huang, Lin, Cai and Liu. This is an open-access article distributed under the terms of the Creative Commons Attribution License (CC BY). The use, distribution or reproduction in other forums is permitted, provided the original author(s) and the copyright owner(s) are credited and that the original publication in this journal is cited, in accordance with accepted academic practice. No use, distribution or reproduction is permitted which does not comply with these terms.
*Correspondence: Chu-Feng Liu, eHl6bW1va0BzbXUuZWR1LmNu
†These authors have contributed equally to this work
Disclaimer: All claims expressed in this article are solely those of the authors and do not necessarily represent those of their affiliated organizations, or those of the publisher, the editors and the reviewers. Any product that may be evaluated in this article or claim that may be made by its manufacturer is not guaranteed or endorsed by the publisher.
Research integrity at Frontiers
Learn more about the work of our research integrity team to safeguard the quality of each article we publish.