- 1Department of Infectious Disease, The People’s Hospital of Longhua, Shenzhen, China
- 2Shenzhen Key Laboratory of Pathogen and Immunity, State Key Discipline of Infectious Disease, Shenzhen Third People’s Hospital, Second Hospital Affiliated to Southern University of Science and Technology, Shenzhen, China
- 3Shenzhen Polytechnic, Shenzhen, China
Echovirus 18 has been recognized as an important causative pathogen of aseptic meningitis in young children worldwide, and echovirus 18-induced meningitis is rarely found in adults with immunocompetence. In this case study, we report the clinical and virological characteristics of aseptic meningitis caused by recombinant echovirus 18 in an adult with immunocompetence. A 31-year-old woman with immunocompetence was admitted to our hospital with fever, dizziness, severe headache, nausea, and vomiting for the past 1 day and was diagnosed with viral meningitis based on the clinical manifestations and laboratory results from cerebrospinal fluid (CSF). The patient received antiviral treatment with ribavirin and interferon as soon as the enterovirus infection was identified using qRT-PCR and was cured after 4 days. From the oropharyngeal swab and CSF samples, two echovirus 18 strains were isolated with a single nucleotide difference located at the 5′ UTR. Phylogenetic analyses based on the VP1 gene showed that the two strains belonged to the subgenotype C2 and were clustered with sequences obtained from China after 2015, while the results from the 3D polymerase region showed that the two strains were closely related to the E30 strains. Bootscanning results using the 5′ UTR to 2A region and the 2B to 3′ UTR region showed that potential intertypic recombination had occurred in the 2B gene. Recombination analyses further confirmed that the two strains (echovirus 18) presented genome recombination with echovirus 30 in the nucleotide regions of the 2B gene. To the best of our knowledge, this is the first report of echovirus 18-induced meningitis in an adult with immunocompetence from mainland China, highlighting the need for close surveillance of echovirus 18 both in children and adults in the future.
Introduction
Meningitis is inflammation of the meninges characterized by an abnormal number of white blood cells (WBC) in cerebrospinal fluid (CSF) with few or no focal neurological findings or brain abnormalities on imaging and accompanied by the acute onset of fever, headache, and neck stiffness (1, 2). Infectious agents, including viruses, bacteria, and fungi, are the most common causes of meningitis (3). Viral meningitis is associated with the acute onset of meningeal symptoms and fever, pleocytosis of the cerebrospinal fluid, and no growth on routine bacterial cultures, usually affecting young children (1, 4). Many viruses have been shown to cause viral meningitis, such as enteroviruses (EVs), parechovirus, herpesviruses, influenza viruses, arboviruses, and coronaviruses, and viral etiology varies according to age and country (3).
Human enteroviruses belong to the Enterovirus genus of the Picornaviridae family and are characterized as non-enveloped, positive-sense, single-stranded RNA viruses with ∼7,500 nucleotides (5). The enteroviruses contain four enterovirus species (A to D) and three rhinovirus species (A to C) and infect millions of people worldwide every year with mostly mild and self-limited symptoms, such as hand-foot-and-mouth disease, herpangina, pleurodynia, rashes, and rhinitis (5, 6). Moreover, an increasing number of enteroviruses have been found to infect the central nervous system and result in various neurological diseases, of which non-polio human enteroviruses (NPEV) have become the leading recognizable cause of viral meningitis (5, 6). Notably, Enterovirus B (EV-B), a species of the genus Enterovirus, particularly echovirus 6, 9, 13, 14, 16, and 30, accounted for the majority of meningitis cases with worldwide distribution (5). As a member of EV-B, echovirus 18 was first discovered in 1955 (7), and since then, it has been reported as a pathogen causing aseptic meningitis in many countries of the world (8–14). Most of the patients in these reports were young children, and an outbreak of encephalitis/meningitis caused by echovirus 18 in children has been reported in 2015 in mainland China (9). There was no report of echovirus 18-induced meningitis in adults with immunocompetence in mainland China and scant report in other countries of world (10–12). In the present study, we report the clinical and virological characteristics of aseptic meningitis caused by the recombinant echovirus 18 in an adult with immunocompetence from Shenzhen, China.
Materials and methods
Sample collection and virus isolation
Oropharyngeal swabs and CSF samples were collected from the patient on the first and second days following admission, respectively. All specimens positive for enterovirus infection by qRT-PCR were cultured in Rhabdomyosarcoma (RD) cells for virus isolation. The study was performed in accordance with guidelines approved by the ethics committees of Shenzhen People’s Hospital Longhua Branch and the Shenzhen Third People’s Hospital. Written informed consent was obtained from the patient for the publication of any potentially identifiable images or data included in this article.
Quantitative reverse transcriptase PCR (qRT-PCR)
Nucleic acids were extracted from 140 μl of the oropharyngeal swab, CSF, and cell culture supernatant samples using the QIAamp RNA Viral Kit (Qiagen, Germany) according to the instructions of the manufacturer. Nucleic acids were eluted in 50 μl of AVE buffer and stored at −80°C for subsequent use. Nucleic acids samples were tested by quantitative reverse transcription polymerase chain reaction (qRT-PCR) in an ABI QuantStudio Dx Real-Time cycler (Applied Biosystems, USA), using commercial kits targeting the common pathogens that induce meningitis (Mabsky Biotech Co., Ltd.), including herpes simplex virus (HSV)-1, HSV-2, varicella-zoster virus (VZV), human cytomegalovirus (HCMV), Epstein-Barr virus (EBV), human herpes virus 6 (HHV-6), HHV7, JC virus (JCV), human parechovirus (HPeV), enterovirus (EV), mumps virus, measles virus, Haemophilus influenzae, Streptococcus pneumoniae, Staphylococcus aureus, Listeria monocytogenes, Neisseria meningitidis, Streptococcus agalactiae, Cryptococcus neoformans, and Escherichia coli.
Full-length genome amplification
The amplification and sequencing of our two echovirus strains were performed, as previously reported with some modifications (15). Three long-distance PCR amplifications were performed using a PrimeScriptTM One-Step RT-PCR Kit Version 2 (Takara, China). The primers used for RT-PCR and sequencing of the full-length genome were designed using a “primer-walking” strategy and are listed in Supplementary Table 1. The PCR products were purified using the QIAquick PCR purification kit (Qiagen, Germany) and sequenced (Sangon, China).
Phylogenetic analysis
The phylogenetic analyses were done as previously reported with slight modifications (16). Sequence alignment was performed using MEGA software version 7.0. Phylogenetic trees of the 5′ UTR to 2A region, the 2B to 3′ UTR regions, and the 3D gene were constructed by the neighbor-joining method, with bootstrap values obtained from 1,000 replicates and bootstrap values of >80% were shown. The phylogenetic tree of the VP1 gene and the evolution rates were constructed and determined with Bayesian Evolutionary Analysis Sampling Trees (BEAST) software version 1.8 using a lognormal relaxed clock, a constant-size tree prior, and the GTR + G substitution model. Each Bayesian MCMC analysis was run for 100 million generations. Bootstrap testing with 1,000 replicates was used to estimate the strength of the phylogenetic trees.
Recombination analysis
The recombination analyses were done as described in the previous report (16). A potential recombination within the complete genome sequences of echovirus 18 was assessed using the similarity plot and the bootscanning method with the neighbor-joining method and the Kimura 2-parameter substitution model with a window size of 500 nucleotides (nt) and a step size of 20 nt. The value of the permuted trees of > 80% indicated potential recombination events.
Results
Case presentation
A 31-year-old immunocompetent woman was admitted to our hospital in May 2019 and presented with fever (37.8°C), dizziness, severe headache, nausea, and vomiting for 1 day (Figure 1). Upon admission, the patient had a normal heart rate (71 bpm) and blood pressure (116/76 mmHg). There were no abnormalities in the skin, in muscle tension, and during defecation, and there was no manifestation of Babinski’s sign but of nuchal rigidity. She was primarily diagnosed as having an intracranial infection based on the clinical manifestations above and hospitalized (4). Serum laboratory tests revealed leukopenia (WBC count: 3.28 × 109/L) and lymphopenia (LYM count: 0.97 × 109/L). Furthermore, pleocytosis (WBC in CSF: 40 × 106/L) (17, 18), moderately increased levels of protein (564 mg/dl), and normal glucose (2.93 mmol/L) were found in CSF (4) (Table 1). Based on the manifestations and laboratory results, viral meningitis was diagnosed. To reduce the intracranial pressure, an empirical therapy with intravenous acyclovir for antiviral treatments and mannitol and glycerol fructose injections was used (Figure 1). A panel of common pathogens that induce meningitis was detected using commercial qRT-PCR kits, and the results showed that EV was positive in both the oropharyngeal swab and the CSF samples. Then, ribavirin and interferon were used as soon as possible instead of acyclovir. The fever lasted for another 3 days with a higher body temperature of 38.4°C, and the headache also resolved completely 3 days after the therapy. The treatment with interferon, mannitol, and glycerol fructose injections was stopped after 12 h of the fever breaks. Meanwhile, the result of the Gram staining smear test was negative 1 day after submission, and the bacterial culture with CSF was also negative during the cultivation time, which further confirmed aseptic meningitis.
Isolation and identification of echovirus 18
The product of qRT-PCR was sequenced using Sanger sequencing, and the results of BLAST showed the highest similarity with echovirus 18. Then, the oropharyngeal swab and CSF samples were subjected to virus isolation using RD cells. After two rounds of passage, both the supernatant from the oropharyngeal swab and the CSF samples showed typical cytopathic effects (CPEs) and positive qRT-PCR results of EV, indicating the successful isolation of the virus (data not shown). Overlapping fragments covering the entire genome of the virus were amplified and then sequenced by Sanger sequencing. The assembled genome sequences were submitted to the GenBank database with strain names LJ/0530/2019 (GenBank accession no. MN215884) and LJ/0601/2019 (GenBank accession no. MN337405) from oropharyngeal swab and CSF samples, respectively. Only one nucleotide at the 5′ UTR differed between the two strains with C in the CSF and T in the oropharyngeal swab, and this difference was confirmed using the original specimens. The complete genomes of the two strains showed an identity of 89.07% with the prototype strain of echovirus 18 strain Metcalf (GenBank accession no. AF317694). Meanwhile, the two strains showed the highest similarity of approximately 99% with the two sequences collected in Beijing, China, in 2018 (GenBank accession no. MN815810.1 and MN815811.1).
Phylogenetic characteristics of the isolated echovirus 18
To analyze the evolutionary relationship of the two strains to the other 18 echovirus strains in our study, phylogenetic analyses based on the VP1 coding region and 3D polymerase genes were performed. A total of 95 representative complete VP1 gene sequences of echovirus 18, including the 14 strains dated in 2019 and 2020, were downloaded from GenBank and analyzed using MEGA7.0 and BEAST software. As previously reported (9), all the strains were divided into three genotypes (genotypes A, B, and C), and genotype C was further divided into the C1 and C2 subgroups (Figure 2). The mean genetic distance between genotypes A and B was 0.17 (ranging from 0.15 to 0.21), between genotypes A and C was 0.22 (ranging from 0.19 to 0.25), and between genotypes B and C was 0.19 (ranging from 0.15 to 0.24). The two strains in our study belonged to the subgenotype C2 and were clustered with sequences obtained in China after 2015. Phylogenetic analysis of 3D the polymerase region was conducted using sequences obtained from the 3D gene of the LJ-0601 and its first 100 hits obtained from a BLAST search in GenBank (Supplementary Figure 1). Our two strains were also found to be closely related to the E30 strain (GenBank accession no. MW080372, MW080377, and MK238483), which differs from the results with the VP1 gene, indicating that possible recombination has occurred. Notably, strains E18-221, E18-398, and E18-314 were also found to be recombinants based on our analysis, which is consistent with a previous report (16).
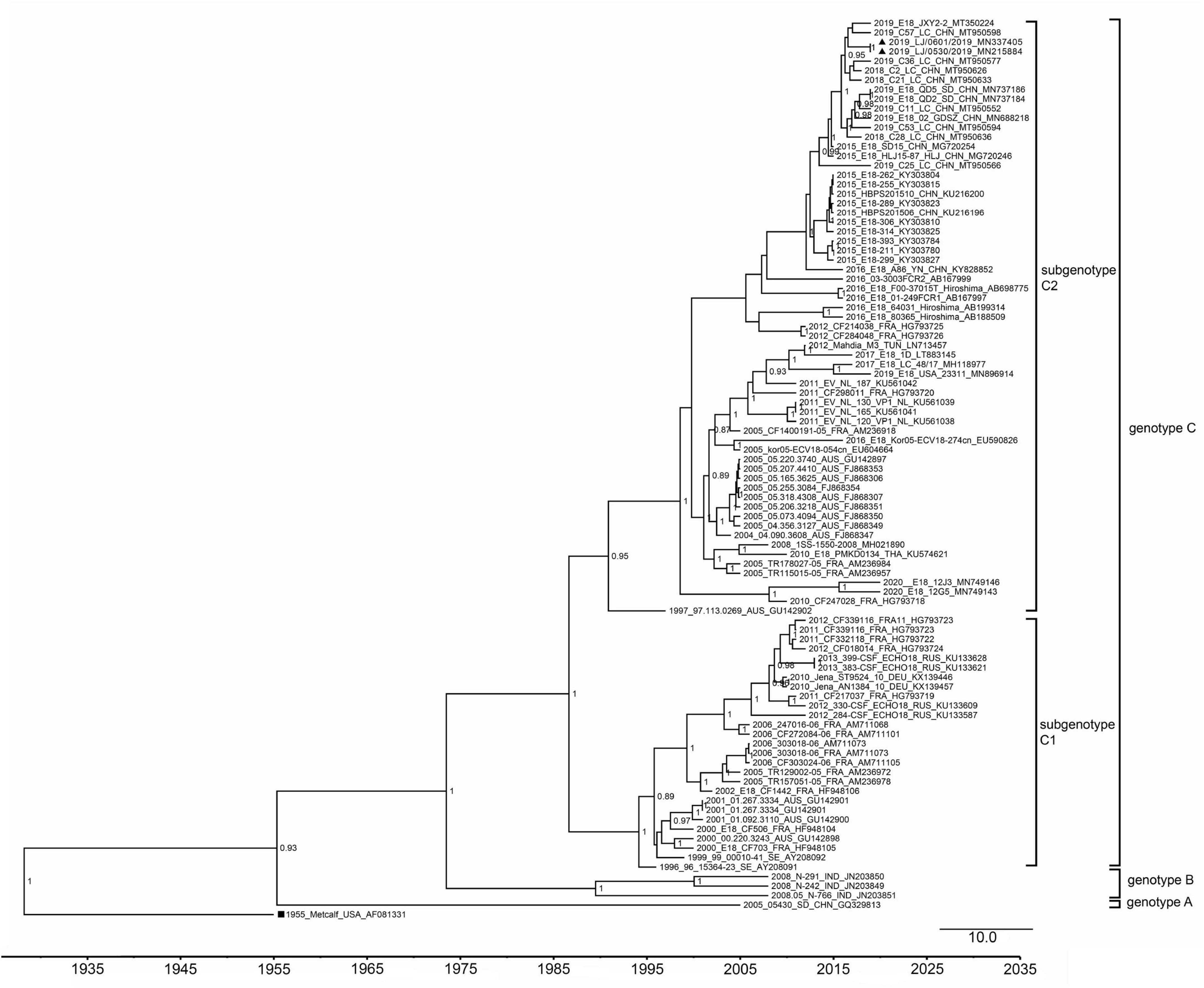
Figure 2. Phylogenetic tree based on the VP1 gene of echovirus 18. The phylogenetic tree was constructed using BEAST software. The posterior probabilities from the MrBayes analyses supporting the trees are indicated at the nodes. The triangle indicates the two strains in our study. The scale bar indicates years. The strain name, year of sampling, and GenBank accession numbers are shown.
Moreover, phylogenetic relationship analyses based on the bootscanning results were performed using the 5′ UTR to 2A region (Figure 3A) and the 2B to 3′ UTR region (Figure 3B) of our two strains and the other 79 EV-B strains available in GenBank. The results showed that the 5′ UTR to 2A region of our two strains was closely clustered with other echovirus 18 strains, especially the strains found in Hebei, China, in 2015 (Figure 3), which is consistent with the preliminary molecular typing results. However, for the 2B to 3′ UTR region, our two strains did not cluster with other echovirus 18 strains while showing high similarity with the two echovirus E30 strains (GenBank accession no. MW080372 and MW080377), as shown in Supplementary Figure 1, suggesting that potential intertypic recombination had occurred in the 2B gene.
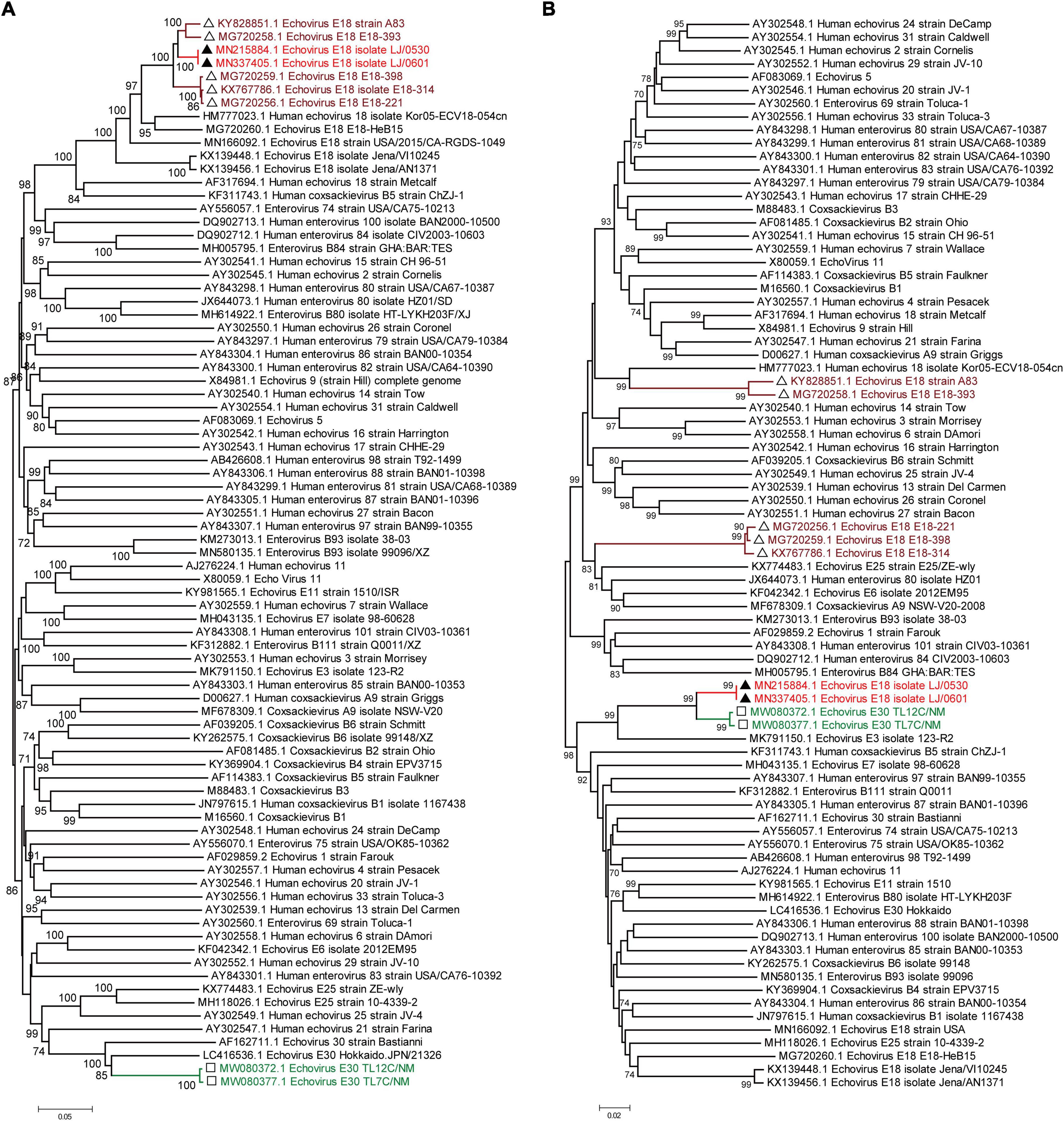
Figure 3. Phylogenetic relationships based on the 5′ UTR to 2A region and the 2B region to 3′ UTR of EV-B strains. Panels (A,B) show the phylogenetic trees of the representative 5′ UTR to 2A region (A) and the 2B to 3′ UTR region of the EV-B strains. Numbers at the nodes indicate bootstrap support for that node (percentage of 1,000 bootstrap replicates). The scale bars represent the genetic distance. Red indicates the two strains in our study. Brown indicates the echovirus 18 strains that are highly homologous to our strains in the 5′ UTR to 2A region. Green indicates the echovirus 30 strains that are highly homologous to our strains in the 2B region to 3′ UTR.
Recombination analysis of the isolated echovirus 18
First, the RDP V4.1 software was used to analyze the potential recombinant sequences (19). The results showed that the most possible major and minor parent strains were echovirus 18 isolate E18-393/HeB/CHN/2015 (GenBank accession no. MG720258) and echovirus E30 isolate TL7C/NM/CHN/2016 (GenBank accession no. MW080377), respectively, and other possible parent strains include echovirus 18 isolate Metcalf (GenBank accession no. AF317694) and echovirus E30 isolate TL7C/NM/CHN/2016 (GenBank accession no. MW080377) (data not shown). By combining the results of the phylogenetic and RDP analyses, four potential parental strains were subjected to similarity plots and bootscanning analyses using Simplot v3.1 software (Figure 4). Consistent with the results in Figure 3, our two strains showed the highest similarity with the echovirus 18 isolate E18-393/HeB/CHN/2015 in the 5′ UTR to 2A region and with the echovirus E30 isolate TL7C/NM/CHN/2016 in the 2B to 3′ UTR region. Furthermore, the bootscanning results further confirmed that the intratypic recombination site was in the 2B gene (Figure 4).
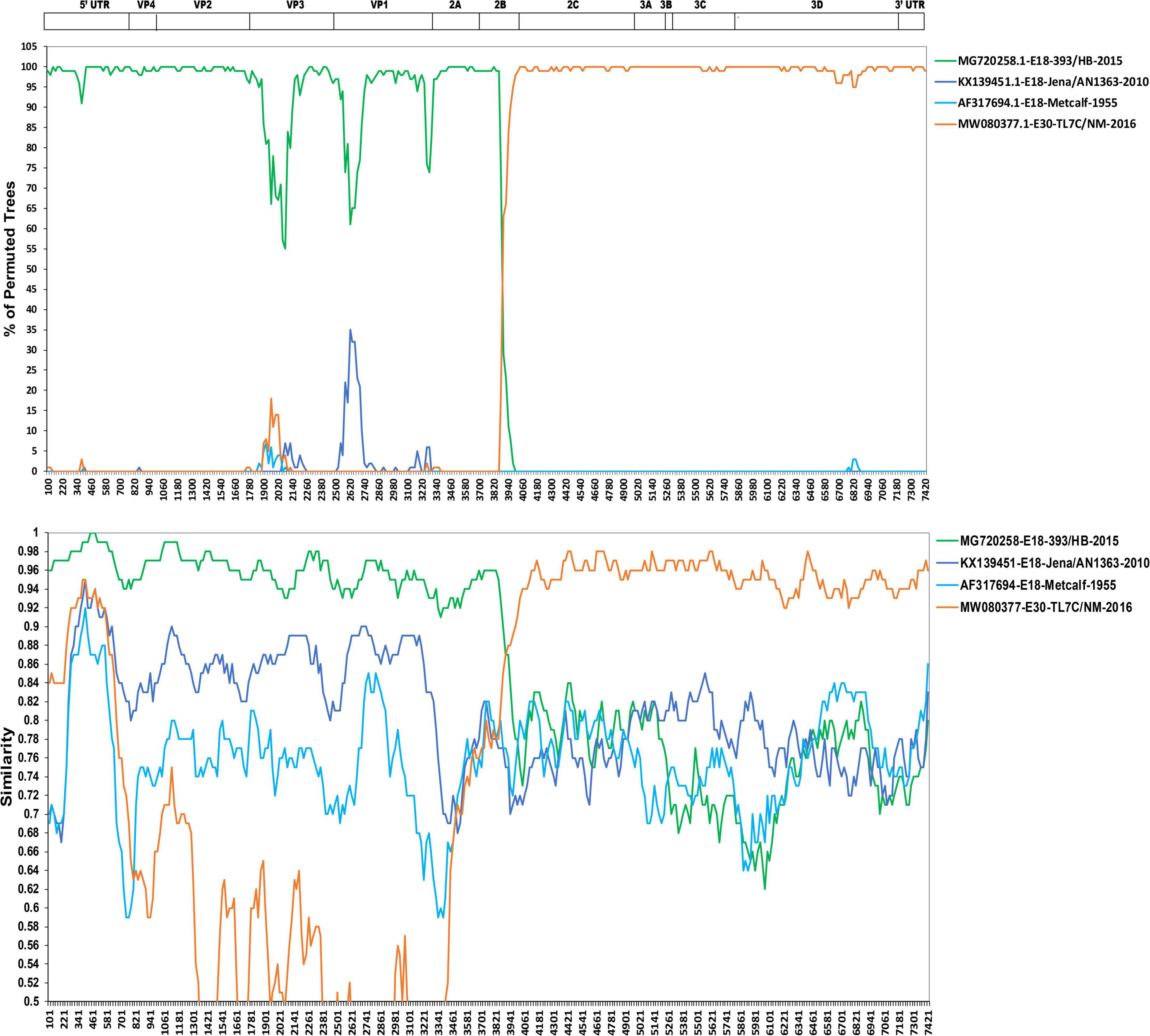
Figure 4. Similarity plot and bootscanning analyses of the two echovirus 18 strains based on their full-length genomes. Four potential parental strains of echovirus 18 and echovirus 30 were subjected to similarity plots and bootscanning analyses using Simplot v3.1 software.
Discussion
For the clinical diagnosis of meningitis, clinical symptoms are always not typical enough, as fever, chills, abdominal pain, nausea, and headaches are common in patients with meningitis, and short and fast breathing, loss of appetite, neck stiffness and pain, and sensitivity to bright light were also found in some patients (2, 20). Meanwhile, the presence of Brudzinski’s sign, Kering’s sign, or nuchal rigidity indicates that these bedside tests can be used by physicians to assess whether a patient should have a lumbar puncture (20). WBC, the proportion of neutrophils, glucose, and concentration of lactic acid in the CSF are key for differentiating the different types of meningitis (20–23). Although bacterial meningitis is usually characterized as a high WBC count (≥ 500 cells/μl) with a large proportion of neutrophils (>80%), studies also found that patients with bacterial meningitis may present with normal CSF leukocyte counts (24, 25), which is of concern for the diagnosis based on a lumbar puncture. Moreover, with few exceptions, the clinical manifestations and symptoms associated with viral meningitis are similar despite different causative viruses, making it difficult to choose antiviral agents (1, 4). Currently, molecular diagnosis methods, including nested-PCR, multiplex PCR, and qRT-PCR assays targeting specific viruses, serve as the new golden standard tool for the diagnosis of viral meningitis with high sensitivity and specificity, which enables healthcare providers to rapidly diagnose certain infections and therefore allow clinical management decisions (4, 26). In our study, the patient received empirical antiviral therapy with intravenous acyclovir upon admission, which is effective for Herpesviridae family members but not for enteroviruses (27). The antiviral agents were changed into ribavirin and interferon as soon as the EV infection was identified using qRT-PCR, which could significantly improve the antiviral treatment efficiency with the rapid cure of this patient.
Aseptic meningitis encompasses broad differential diagnoses related to inflammation of the meninges not caused by pyogenic bacteria with both infective and noninfective causes, while viral pathogens are the most common causative agents (20). Notably, the viral etiology varies by age, the immune status of the host, geographic location, season, and exposure history (2). For example, arbovirus-induced meningitis was highly correlated with geographical location and season; Herpesviridae family members, including CMV, VZV, HHV-6, and EBV-induced meningitis, were mainly found in patients who were immunocompromised but rarely in individuals with immunocompetence (28); mumps, measles, and VZV-induced CNS infections significantly declined after effective vaccines became available (2). Enteroviruses are the most common viruses that could cause meningitis in children and adults (5, 29). Studies showed that approximately 58.6% of the infected children and 51.6% of the infected adults diagnosed with meningitis/encephalitis were due to enterovirus (30, 31). One recent nationwide active surveillance study in China showed that EVs accounted for approximately 64.14% of acute meningitis/encephalitis in children and 25.19% in adults (32).
Echovirus 18-induced aseptic meningitis was mainly found in children, from sporadic infection to outbreak in various countries (12, 14, 15, 33–36), and the most recent outbreak in children has been reported in 2015 in mainland China (9). Adult patients with echovirus 18-induced aseptic meningitis have been previously reported in a limited number of cases (10, 12, 14), while severe manifestations and possibly long-term neurofunctional disability were also found to be associated (37, 38). Studies showed that the echovirus 18 strains were phylogenetically divided into three genotypes (16), and our strain belonged to the C1 subgroup, clustered with strains from China found after 2015. Moreover, the results based on the bootscanning analyses using the 5′ UTR to 2A region and the 2B to 3′ UTR region suggested a potential intratypic recombination had occurred in the 2B gene, and this was further confirmed by the recombination analysis. Recombination and mutations in enteroviruses have been recognized as the main mechanisms for the observed high rate of evolution and subsequently lead to changes in the tropism and virulence of enterovirus (39, 40). Similar to other enteroviruses, recombination was also found to be frequent for echovirus 18 (15, 16). Previous studies suggested that the current echovirus 18 strains were potential multiple-recombinant viruses containing many other EV-B donor sequences, and recombination was frequently detected in the 5′ UTR, P2, and P3 regions (15, 16). Consistent with previous studies, the recombination site of our strain was also in the 2B protein of the P2 region. Moreover, as studies showed that mutations and deletions in the UTR of some viruses are associated with replication and virulence (41–45), the single nucleotide difference between the two strains from the CSF and oropharyngeal swab samples in the 5′ UTR of echovirus 18 merits further investigation.
Conclusion
Although echovirus 18 has been circulating in mainland China for years, induced aseptic meningitis by echovirus 18 was only found in children previously. To the best of our knowledge, this is the first report of echovirus 18 in an adult with immunocompetence from mainland China, highlighting the need for close surveillance of echovirus 18 both in children and adults in the future.
Data availability statement
The datasets presented in this study can be found in online repositories. The names of the repository/repositories and accession number (s) can be found in the Genbank database: MN215884 and MN337405.
Ethics statement
This study was performed in accordance with guidelines approved by the Ethics Committees from the People’s Hospital of Longhua and Shenzhen Third People’s Hospital. Written informed consent was obtained from the patient for the publication of any potentially identifiable images or data included in this article.
Author contributions
YY, YL, and HG contributed to the conception and design of the study. CJ, ZX, JL, JZ, and XX enrolled the patient, performed the experiments, and analyzed the clinical data and sequences. JJ, GJ, XW, YP, TC, ZL, and LX collected the clinical data and performed some of the experiments. JL and YY drafted the manuscript. CJ, HG, and YL contributed to the critical revision of the manuscript. All authors reviewed and revised the manuscript and approved the final version.
Funding
This study was supported by the National Science and Technology Major Project (grant no. 2021YFC2301803), the Shenzhen Science and Technology Research and Development Project (grant no. JSGG20201103153802007), and the Shenzhen High-Level Hospital Construction Fund. The funding bodies had no role in the design of the study; the collection, analysis, and interpretation of data; or the writing of the manuscript.
Conflict of interest
The authors declare that the research was conducted in the absence of any commercial or financial relationships that could be construed as a potential conflict of interest.
Publisher’s note
All claims expressed in this article are solely those of the authors and do not necessarily represent those of their affiliated organizations, or those of the publisher, the editors and the reviewers. Any product that may be evaluated in this article, or claim that may be made by its manufacturer, is not guaranteed or endorsed by the publisher.
Supplementary material
The Supplementary Material for this article can be found online at: https://www.frontiersin.org/articles/10.3389/fmed.2022.1094347/full#supplementary-material
References
2. Polage CR, Cohen SH. State-of-the-Art microbiologic testing for community-acquired meningitis and encephalitis. J Clin Microbiol. (2016) 54:1197–202. doi: 10.1128/jcm.00289-16
3. Al-Qahtani SM, Shati AA, Alqahtani YA, Ali AS. Etiology, clinical phenotypes, epidemiological correlates, laboratory biomarkers and diagnostic challenges of pediatric viral meningitis: descriptive review. Front Pediatr. (2022) 10:923125. doi: 10.3389/fped.2022.923125
4. Wright WF, Pinto CN, Palisoc K, Baghli S. Viral (aseptic) meningitis: a review. J Neurol Sci. (2019) 398:176–83. doi: 10.1016/j.jns.2019.01.050
5. Chen BS, Lee HC, Lee KM, Gong YN, Shih SR. Enterovirus and encephalitis. Front Microbiol. (2020) 11:261. doi: 10.3389/fmicb.2020.00261
6. Huang HI, Shih SR. Neurotropic enterovirus infections in the central nervous system. Viruses. (2015) 7:6051–66. doi: 10.3390/v7112920
7. Eichenwald HF, Ababio A, Arky AM, Hartman AP. Epidemic diarrhea in premature and older infants caused by ECHO virus type 18. J Am Med Assoc. (1958) 166:1563–6. doi: 10.1001/jama.1958.02990130023005
8. Baek K, Park K, Jung E, Chung E, Park J, Choi H, et al. Molecular and epidemiological characterization of enteroviruses isolated in Chungnam, Korea from 2005 to 2006. J Microbiol Biotechnol. (2009) 19:1055–64. doi: 10.4014/jmb.0810.584
9. Chen X, Li J, Guo J, Xu W, Sun S, Xie Z. An outbreak of echovirus 18 encephalitis/meningitis in children in Hebei Province, China, 2015. Emerg Microbes Infect. (2017) 6:e54. doi: 10.1038/emi.2017.39
10. Krumbholz A, Egerer R, Braun H, Schmidtke M, Rimek D, Kroh C, et al. Analysis of an echovirus 18 outbreak in Thuringia, Germany: insights into the molecular epidemiology and evolution of several enterovirus species B members. Med Microbiol Immunol. (2016) 205:471–83. doi: 10.1007/s00430-016-0464-z
11. Miyamura K, Yamashita K, Yamadera S, Kato N, Akatsuka M, Yamazaki S. An epidemic of echovirus 18 in 1988 in Japan–high association with clinical manifestation of exanthem. a report of the national epidemiological surveillance of infectious agents in Japan. Jpn J Med Sci Biol. (1990) 43:51–8. doi: 10.7883/yoken1952.43.51
12. Turabelidze G, Lin M, Butler C, Fick F, Russo T. Outbreak of echovirus 18 meningitis in a rural Missouri community. Mo Med. (2009) 106:420–4.
13. Wang SM, Ho TS, Shen CF, Wang JR, Liu CC. Echovirus 18 meningitis in southern Taiwan. Pediatr Infect Dis J. (2011) 30:259–60. doi: 10.1097/INF.0b013e3181f7cb69
14. Wilfert CM, Lauer BA, Cohen M, Costenbader ML, Myers E. An epidemic of echovirus 18 meningitis. J Infect Dis. (1975) 131:75–8. doi: 10.1093/infdis/131.1.75
15. Zhang H, Zhao Y, Liu H, Sun H, Huang X, Yang Z, et al. Molecular characterization of two novel echovirus 18 recombinants associated with hand-foot-mouth disease. Sci Rep. (2017) 7:8448. doi: 10.1038/s41598-017-09038-y
16. Chen X, Ji T, Guo J, Wang W, Xu W, Xie Z. Molecular epidemiology of echovirus 18 circulating in Mainland China from 2015 to 2016. Virol Sin. (2019) 34:50–8. doi: 10.1007/s12250-018-0080-8
17. Centers for Disease Control and Prevention (CDC). Outbreaks of aseptic meningitis associated with echoviruses 9 and 30 and preliminary surveillance reports on enterovirus activity–United States, 2003. MMWR Morb Mortal Wkly Rep. (2003) 52:761–4.
18. Khetsuriani N, Lamonte-Fowlkes A, Oberst S, Pallansch MA. Enterovirus surveillance–United States, 1970-2005. MMWR Surveill Summ. (2006) 55:1–20.
19. Martin D, Rybicki E. RDP detection of recombination amongst aligned sequences. Bioinformatics. (2000) 16:562–3. doi: 10.1093/bioinformatics/16.6.562
20. Kohil A, Jemmieh S, Smatti MK, Yassine HM. Viral meningitis: an overview. Arch Virol. (2021) 166:335–45. doi: 10.1007/s00705-020-04891-1
21. Davis LE. Acute bacterial meningitis. Continuum (Minneap Minn). (2018) 24:1264–83. doi: 10.1212/con.0000000000000660
22. Dumaidi K, Al-Jawabreh A. Molecular detection and genotyping of enteroviruses from CSF samples of patients with suspected sepsis-like illness and/or aseptic meningitis from 2012 to 2015 in West Bank, Palestine. PLoS One. (2017) 12:e0172357. doi: 10.1371/journal.pone.0172357
24. Lambert HP. Diagnosing viral meningitis: other important diagnoses must be excluded. BMJ. (2008) 336:110. doi: 10.1136/bmj.39458.446551.3A
25. van Soest TM, Chekrouni N, van Sorge NM, Brouwer MC, van de Beek D. Bacterial meningitis presenting with a normal cerebrospinal fluid leukocyte count. J Infect. (2022) 84:615–20. doi: 10.1016/j.jinf.2022.02.029
26. Ramanan P, Bryson AL, Binnicker MJ, Pritt BS, Patel R. Syndromic panel-based testing in clinical microbiology. Clin Microbiol Rev. (2018) 31:e00024-17. doi: 10.1128/cmr.00024-17
27. Meyfroidt G, Kurtz P, Sonneville R. Critical care management of infectious meningitis and encephalitis. Intensive Care Med. (2020) 46:192–201. doi: 10.1007/s00134-019-05901-w
28. Poplin V, Boulware DR, Bahr NC. Methods for rapid diagnosis of meningitis etiology in adults. Biomark Med. (2020) 14:459–79. doi: 10.2217/bmm-2019-0333
29. Le Govic Y, Demey B, Cassereau J, Bahn YS, Papon N. Pathogens infecting the central nervous system. PLoS Pathog. (2022) 18:e1010234. doi: 10.1371/journal.ppat.1010234
30. Balada-Llasat JM, Rosenthal N, Hasbun R, Zimmer L, Bozzette S, Duff S, et al. Cost of managing meningitis and encephalitis among infants and children in the United States. Diagn Microbiol Infect Dis. (2019) 93:349–54. doi: 10.1016/j.diagmicrobio.2018.10.012
31. Hasbun R, Rosenthal N, Balada-Llasat JM, Chung J, Duff S, Bozzette S, et al. Epidemiology of meningitis and encephalitis in the United States, 2011-2014. Clin Infect Dis. (2017) 65:359–63. doi: 10.1093/cid/cix319
32. Wang LP, Yuan Y, Liu YL, Lu QB, Shi LS, Ren X, et al. Etiological and epidemiological features of acute meningitis or encephalitis in China: a nationwide active surveillance study. Lancet Reg Health West Pac. (2022) 20:100361. doi: 10.1016/j.lanwpc.2021.100361
33. Chen XP, Sun SZ, Guo JY, Li JJ, Xie ZD. Complete genome sequence analysis of echovirus 18 associated with aseptic meningitis in hebei province, China, in 2015. Genome Announc. (2016) 4:e01165-16. doi: 10.1128/genomeA.01165-16
34. McLaughlin JB, Gessner BD, Lynn TV, Funk EA, Middaugh JP. Association of regulatory issues with an echovirus 18 meningitis outbreak at a children’s summer camp in Alaska. Pediatr Infect Dis J. (2004) 23:875–7. doi: 10.1097/01.inf.0000136867.18026.22
35. Tsai HP, Huang SW, Wu FL, Kuo PH, Wang SM, Liu CC, et al. An echovirus 18-associated outbreak of aseptic meningitis in Taiwan: epidemiology and diagnostic and genetic aspects. J Med Microbiol. (2011) 60(Pt. 9):1360–5. doi: 10.1099/jmm.0.027698-0
36. Wang J, Meng M, Xu H, Wang T, Liu Y, Yan H, et al. Analysis of enterovirus genotypes in the cerebrospinal fluid of children associated with aseptic meningitis in Liaocheng, China, from 2018 to 2019. BMC Infect Dis. (2021) 21:405. doi: 10.1186/s12879-021-06112-9
37. Bodilsen J, Mens H, Midgley S, Brandt CT, Petersen PT, Larsen L, et al. Enterovirus meningitis in adults: a prospective nationwide population-based cohort study. Neurology. (2021) 97:e454–63. doi: 10.1212/wnl.0000000000012294
38. McGill F, Griffiths MJ, Bonnett LJ, Geretti AM, Michael BD, Beeching NJ, et al. Incidence, aetiology, and sequelae of viral meningitis in UK adults: a multicentre prospective observational cohort study. Lancet Infect Dis. (2018) 18:992–1003. doi: 10.1016/s1473-3099(18)30245-7
39. Lukashev AN, Shumilina EY, Belalov IS, Ivanova OE, Eremeeva TP, Reznik VI, et al. Recombination strategies and evolutionary dynamics of the human enterovirus a global gene pool. J Gen Virol. (2014) 95(Pt. 4):868–73. doi: 10.1099/vir.0.060004-0
40. Lukashev AN, Lashkevich VA, Ivanova OE, Koroleva GA, Hinkkanen AE, Ilonen J. Recombination in circulating human enterovirus B: independent evolution of structural and non-structural genome regions. J Gen Virol. (2005) 86(Pt. 12):3281–90. doi: 10.1099/vir.0.81264-0
41. Zhang QY, Liu SQ, Li XD, Li JQ, Zhang YN, Deng CL, et al. Sequence duplication in 3′ UTR modulates virus replication and virulence of Japanese encephalitis virus. Emerg Microbes Infect. (2022) 11:123–35. doi: 10.1080/22221751.2021.2016354
42. Xiong J, Cui X, Zhao K, Wang Q, Huang X, Li D, et al. Novel motif in the 3′-UTR of PRRSV-2 is critical for viral multiplication and contributes to enhanced replication ability of highly pathogenic or L1 PRRSV. Viruses. (2022) 14:166. doi: 10.3390/v14020166
43. Wang D, Yang C, Deng Y, Cao X, Xu W, Han Z, et al. Conserved RNA secondary structure in Cherry virus A 5′-UTR associated with translation regulation. Virol J. (2022) 19:91. doi: 10.1186/s12985-022-01824-z
44. Ben Youssef A, Gharbi J, George B, Das S, Ben M’hadheb MA. Single mutation in the cryptic AUG (cAUG) affects in vitro translation and replication efficiencies and in vivo virulence of coxsackievirus B3 (CVB3). Curr Microbiol. (2022) 79:288. doi: 10.1007/s00284-022-02986-3
Keywords: aseptic meningitis, echovirus 18, immunocompetent adult, recombination < evolution, case report
Citation: Jiang C, Xu Z, Li J, Zhang J, Xue X, Jiang J, Jiang G, Wang X, Peng Y, Chen T, Liu Z, Xie L, Gao H, Liu Y and Yang Y (2023) Case report: Clinical and virological characteristics of aseptic meningitis caused by a recombinant echovirus 18 in an immunocompetent adult. Front. Med. 9:1094347. doi: 10.3389/fmed.2022.1094347
Received: 10 November 2022; Accepted: 14 December 2022;
Published: 12 January 2023.
Edited by:
Francesco Paolo Bianchi, University of Bari Aldo Moro, ItalyReviewed by:
Chenguang Shen, Southern Medical University, ChinaYanqun Wang, Guangzhou Medical University, China
Xiangpeng Chen, Beijing Children’s Hospital, Capital Medical University, China
Copyright © 2023 Jiang, Xu, Li, Zhang, Xue, Jiang, Jiang, Wang, Peng, Chen, Liu, Xie, Gao, Liu and Yang. This is an open-access article distributed under the terms of the Creative Commons Attribution License (CC BY). The use, distribution or reproduction in other forums is permitted, provided the original author(s) and the copyright owner(s) are credited and that the original publication in this journal is cited, in accordance with accepted academic practice. No use, distribution or reproduction is permitted which does not comply with these terms.
*Correspondence: Yang Yang, young@mail.sustech.edu.cn; Yingxia Liu,
yingxialiu@hotmail.com; Haibin Gao,
315219232@qq.com
†These authors have contributed equally to this work