- 1Quantitative and Systems Biology Graduate Program, University of California, Merced, Merced, CA, United States
- 2Department of Molecular and Cell Biology, School of Natural Sciences, University of California, Merced, Merced, CA, United States
- 3Health Sciences Research Institute, University of California, Merced, Merced, CA, United States
CXCR5+CD8 T cells have attracted significant interest within multiple areas of immunology, cancer, and infection. This is in part due to their apparent dual functionality. These cells perform as cytotoxic cells in a variety of infection states including LCMV, HBV, HIV and SIV. However, CXCR5+CD8 T cells also associate with B cells in peripheral organs and function to stimulate B cell proliferation, antibody/B cell receptor class-switch, and antibody production. CXCR5+CD8 T cells are similar to CXCR5+CD4 T follicular helpers in their genetic make-up, B cell interactions, and functionality despite possessing elevated programmed cell death 1 and cytotoxic proteins. Within cancer CXCR5+CD8 T cells have risen as potential prognostic markers for overall survival and are functionally cytotoxic within tumor microenvironments. In inflammatory disease and autoimmunity, CXCR5+CD8 T cells are implicated in disease progression. During viral infection and cancer, CXCR5 expression on CD8 T cells generally is indicative of progenitor memory stem-like exhausted cells, which are more responsive to immune checkpoint blockade therapy. The use of immune checkpoint inhibitors to overcome immune exhaustion in cancer, and subsequent consequence of immune adverse events, highlights the dual nature of the cellular immune response. This review will detail the functionality of CXCR5+CD8 T cells in cancer and autoimmunity with potential repercussions during immune checkpoint blockade therapy discussed.
Introduction
In the past decade research has focused on elucidating the regulation and functionality of CXCR5+ T follicular cells (Tfh) during infection, cancer, and autoimmune disease. CXCR5+ T cells interact with germinal center B cells and initiate differentiation into antibody-producing (plasma) cells or memory formation through proliferation, somatic hypermutation, and class-switch recombination (1–6). CXCR5+CD4 T follicular helpers (CD4 Tfh) have received most of the limelight for their contribution to germinal center migration, functionality, and B cell help within infection, cancer, and autoimmunity (6–9). CXCR5+CD8 T cells have slowly come into focus with their unique ability to provide B cell help within germinal centers similar to CD4 Tfh and also maintain a cytolytic capacity in infection, autoimmunity and tumor microenvironments resembling CD8 T effector cells (Figure 1) (1–5, 10, 11). This mini-review focuses on CXCR5+CD8 T cells in cancer, and implications for immune-mediated adverse event (IMAEs) development in patient immunotherapy treatments.
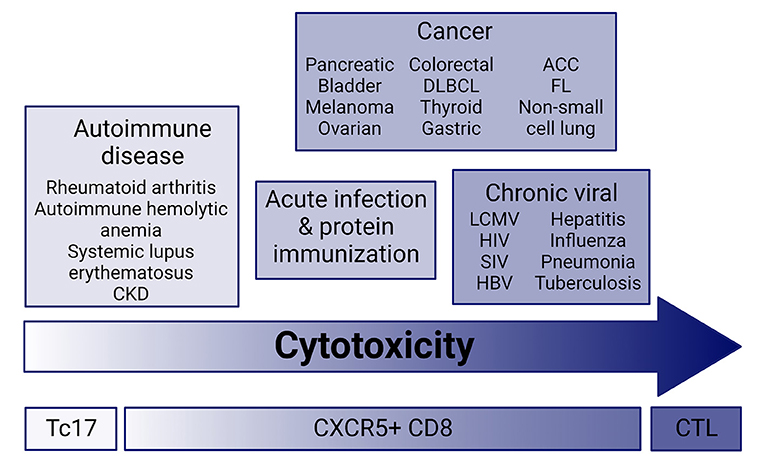
Figure 1. Cytotoxic potential across disease. CXCR5+CD8 T cells have a range of cytolytic potential in infection, cancer, and autoimmune disease. Current research suggests that cytolytic and effector molecule expression ranges from very low in autoimmune disease similar to Tc17 effector cells, and high in chronic infection and cancer. CXCR5+CD8 T cells from acute infection and immunization with protein maintain an intermediate cytolytic potential. CKD, chronic kidney disease; ACC, Adenoid cystic carcinoma; FL, follicular lymphoma; DLBCL, diffuse large B cell lymphoma; CTL, cytotoxic T lymphocyte; Tc17, IL-17-secreting CD8 T cells.
CXCR5+CD8 T cell cytotoxicity and cancer
Tumor microenvironments contain an abundance of innate and adaptive immune cells ranging from tissue-resident cells, such as macrophages, to migratory cells, such as T and B lymphocytes, each with a specific purpose (12). CD8 T cells traditionally activate and differentiate into cytolytic effectors responsible for killing virally infected and cancerous cells. Cytotoxic CD8 T cells are defined by the ability to induce antigen-specific apoptosis of target cells (13). Cytotoxicity is mediated by granzymes, perforin, CD107a, IFNγ, TNFα, TNFβ, and FasL and regulated and defined by key transcription factors, including Blimp-1, eomesodermin (eomes), T-bet, Id1, Id2, and Id3 (14). CXCR5+CD8 T cells localize within blood circulation and tumor microenvironments of cancer patients (15–18). T follicular cells produce varying levels of IL-21 and effector molecules within blood circulation compared to lymph nodes and organs, but their ability to assist B cells remains unaltered indicating that T follicular cell functionality may not be site specific (9, 10).
CXCR5+CD8 T cells have cytotoxic and proliferative capacity within cancer ranging from liquid to solid tumors. CXCL13 upregulation by tumors induces migration, signaling and functional changes by CXCR5-expressing immune cells, but the specific functional capacity of CXCR5+CD8 T cells versus other CXCR5-expressing cells, including B cells and tumors, is poorly characterized in cancer. CXCR5+CD8 T cells from human peripheral blood mononuclear cells, tumor tissues and tumor associated lymph nodes upregulate effector molecules such as IFNγ, TNFα, granzyme B, and perforin compared to CXCR5−CD8 T cells (10, 17–28). CXCR5+CD8 T cells upregulate CD107a, proliferate, and induce specific cell lysis of in vitro co-cultured tumor cells (17, 19, 21, 22, 24, 25). CXCR5+CD8 T cell infiltration of hepatocellular carcinomas generate a robust anti-tumor response in association with B cell antibody production, through IL-21 production, that correlates with a reduction in early tumor recurrence, and is not associated with peritumoral liver or blood tissues (29). This demonstrates the importance of CXCR5+CD8 T cells within the tumor microenvironment and surrounding tissues to patient outcomes and identifies possible tumor eradication mechanisms utilized by CD8 T cells. Furthermore, CXCR5+CD8 T cells appear resistant to immune checkpoint blockade therapy (ICB) induced apoptosis compared to susceptible CXCR5−CD8 T cells and, instead, demonstrate an effector-like phenotype in infection and chronic lymphocytic leukemia (11, 27). CXCR5+CD8 T cells maintain resistance to immune modulation in spite of high program cell death-1 (PD-1) expression, an inhibitory receptor, that is a marker of Tfh. PD-1 on CD4 Tfh retains these cells within germinal centers, with their localization regulated by PD-L1 ligand expression on B cells (6, 30). Since CXCR5+CD8 T cells are similar in gene regulation, action, and surface protein expression to CD4 Tfh this mechanism may also regulate CXCR5+CD8 T cell homing to germinal centers of lymphoid organs and tertiary lymphoid structures in tumors.
CXCR5+CD8 T cells and markers of exhaustion in infection, cancer, and autoimmunity
T cell exhaustion is defined by successive upregulation of inhibitory receptors on CD8 and CD4 T cells in the presence of chronic antigen stimulation in cancer, infection, and autoimmune disease. T cell exhaustion status is sequential and reversible with a phenotypic range from dysfunctional T effector cells to memory progenitors with cytolytic ability to terminal exhaustion with unknown capacity for functional reactivation (31, 32). Under high antigen and inflammation, a step-wise process of inhibitory receptor activation, effector cytokine reduction, and cytolytic effector downregulation on CD8+ T effectors leads to two exhaustion stages, pre-exhausted (Tpex) and terminally exhausted (Tex) (33). Tpex cells are defined by PD-1intTCF1hi and Tex cells by PD-1hiTIM3hi expression (34). Subsequently, nine Tex subtypes were identified through high-dimensional mass cytometry suggesting that transition between Tpex and Tex involves multiple stages or that multiple inhibitory receptors have overlapping function in driving terminal exhaustion (35). Initiation of exhaustion to Tex begins with loss of IL-2 signaling leading to decreases in TNFα and perforin (32, 36). Cytolytic activity of CD107a degranulation is initially maintained along with regulatory cytokines, such as IL-10, which may upregulate and prompt the exhaustive state. Finally, it is thought that some Tex cells, such as CD8 tumor-infiltrating lymphocytes, undergo activation-induced cell death (AICD) following the loss of proliferative capacity (33). This cell death is FAS ligand activated by FAS upregulation on tumor infiltrating lymphocytes and FAS ligand on adjacent tumor cells (37). AICD associates with Tfh and regulates peripheral tolerance, and AICD loss leads to autoimmune progression possibly due to release of self-reactive lymphocytes from cell death mechanisms (6, 38). In tumor microenvironments, ICB T cell reactivation reduces AICD influence potentially enabling self-reactive Tfh activation and driving IMAEs (39, 40).
PD-1 is the canonical marker of T cell exhaustion and a marker of germinal center immune cells. In infection, PD-1 upregulation induces functional CD8+ T cell exhaustion resembling a Tpex cell type (6, 11). CD8+PD-1+ T cells are heterogeneous across infection, cancer, and autoimmunity based on identification of multiple T cell subsets within each disease (10, 41–43). CXCR5+CD8 T cells appear functional with effector/cytolytic capacity in cancer despite high PD-1 expression. CXCR5+CD8 T cells are also described as memory precursor effector cells (MPECs) in various settings (Figure 2; Supplementary Table S1) (11, 27, 44–46). MPECs express CD127, TCF1 (TCF7), and T-bet, lack KLRG1, and produce low effector cytokines (33, 47). These cells persist when transferred generating memory and terminally exhausted cells (33, 47). CXCR5+CD8 T cell subset identified as TCF1+PD-1+TIM3low maintain low cytotoxic capability (27, 45), resembling a Tpex subset with limited proliferation but cytokine production (27, 34, 45). Due to their self-renewal capacity and responsiveness to anti-PD-1 therapy these cells have potential as therapeutic targets (11, 46). A tissue resident exhausted progenitor population expressing TCF1 and cytolytic effector molecules is a self-renewing precursor to circulatory and terminally exhausted populations in LCMV and cancer (44). Upregulation of multiple inhibitory receptors regulate the pathway to Tex, including PD-1, TIM3, CTLA4, Lag3, 2B4 in various combinations. Within LCMV infection, CXCR5+TCF1+TIM3− Tex expand after PD-1 blockade into CXCR5−Tcf1−Tim3+ Tex cells, and in other murine and human settings less exhaustive states exist such as PD-1loKLRG1hiTIM3lo and CXCR5+PD-1+Tim3− that maintain cytolytic capacity (11, 48, 49). CXCR5+TCF1+TIM3− Tex is possibly a memory population that upon functional reactivation becomes terminally exhausted by upregulating the inhibitory receptor, TIM3, as a secondary means of inhibition in high antigen environments, whereas less exhaustive states with downregulated TIM3 expression demonstrate highly functional CXCR5+CD8 T cells.
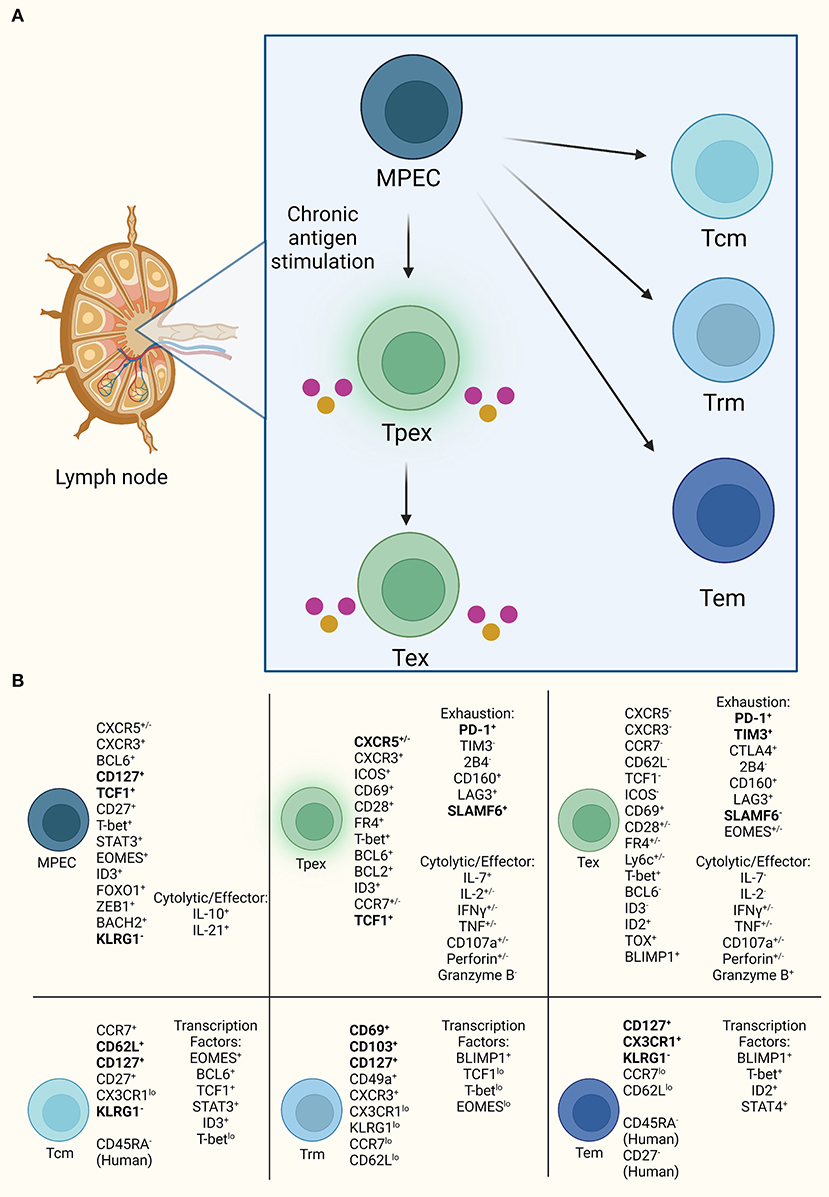
Figure 2. CXCR5+CD8 T cell progenitor lineages. (A) CXCR5+CD8 T cells resemble progenitor cells with self-renewal, such as MPECs, that are capable of diverging into two lineages of either exhausted T cells under high antigen exposure or memory cells with effector functionality. (B) Established markers defining CD8 T cells for each subset diverging from MPEC. Bold indicates consistently used markers for population identification. Also, listed are the currently demonstrated cytolytic and effector molecules in exhausted subsets.
CXCR5+CD8 T cell heterogeneity also exists within cancer and complicates our use of therapies directed at CD8 T effectors (44). CXCR5+ T cell subset variation is found across solid and liquid tumors, from non-small cell lung cancer to follicular B cell non-Hodgkin's lymphoma, where PD-1 and TIM3 expression creates diverging populations (10, 50, 51). In hepatocellular carcinoma, follicular lymphoma, thyroid and high-grade serous ovarian cancer, PD-1 is upregulated on CXCR5+CD8 T cells while TIM3 and CTLA4 are downregulated compared to CXCR5−CD8 T cells possibly indicating a Tpex versus Tex phenotype (15, 21, 24, 25). CXCR5+CD8 T cells in pancreatic cancer express both PD-1 and TIM3 suggesting a Tex phenotype that varies from other cancers (17). Inhibitory marker expression also fluctuates by tissue region, for example in hepatocellular carcinoma upregulated PD-1 is expressed in human liver and tumor tissue compared to blood circulation (29). PD-1, TIM3, 2B4, and Lag3 are downregulated in CXCR5+CD8 T cells in colon cancer, but within gastric cancer PD-1, Lag3, CTLA4, and Tigit are upregulated (23, 26). Furthermore, differences are associated with human versus murine models of disease, such as in metastatic melanoma where CXCR5+PD-1+CD8 T cells were identified by single-cell sequencing in humans, but not identified in a B16 melanoma mouse model (10, 52). Additional research in CXCR5+CD8 T cell effector status and ability to maintain functionality or diverge into exhaustive states within high antigen environments is needed. Characterization of CXCR5+CD8 T cell exhaustion in chronic or high antigen environments is limited for infection, cancer and autoimmunity.
Investigating exhaustion dynamics of CXCR5+ T cells within autoimmunity is challenging due to the association of PD-1 as an activating and inhibitory receptor. There is potential for functional differences between CXCR5+CD8 and CD4 Tfh cells within germinal centers that may resemble antibody-suppressor CXCR5+IFNg+CD8 T cells (53, 54). Antibody-suppressor cells, namely CXCR5+PD-1−CD8 T cells, may activate effector functions on self-reactive B cells and CD4 Tfh cells leading to tolerance and inhibition of plasma cell formation thus halting autoantibody production associated with many autoimmune diseases. Lag3 loss on intra-islet non-obese diabetic CD8 T cells accelerates autoimmune diabetes and highlights Lag3 T cell inhibition differences between autoimmunity, cancer and infection (55, 56). Rao et al. identified a CXCR5+PD-1hiCD4 population within peripheral blood from rheumatoid arthritis patients, but not within synovial fluid, that expressed Tigit and SLAMF6 suggesting Tfh interaction with B cells, and regulation of inhibitory receptor functionality (57). Within human chronic kidney disease, CCR6−CXCR3−CXCR5+PD-1+CD4 and exhausted CD8 T cells are upregulated compared to end-stage kidney disease showcasing exhausted populations in CD4 and CD8 T cells during autoimmunity (58). These studies suggest that non-exhausted and exhausted CXCR5+ T cell subsets exist and influence autoimmunity, and their functions may be different than in infection and cancer. ICB induced reactivation of exhausted CXCR5+ T cells during autoimmunity may enable new autoimmune disease therapies. Alternatively, since exhaustion downregulates T cell effector function, exhaustion itself may provide an additional inhibitory tool in regulating self-reactive T cells.
Could CXCR5+CD8 T cells initiate the development of immune mediated adverse events?
ICB created a paradigm shift in how we understand cancer, the immune system and treatment modalities for cancer patients. A deeper immune-driven exploration of tumor microenvironments is needed to understand how these treatments enable or disrupt immune cell functions promoting patient survival or tumor progression. ICB reactivates exhausted T cells through downregulating inhibitory receptors and ligands on tumors and immune populations by blocking specific protein receptors such as PD-1, PD-L1, and CTLA4 with monoclonal antibodies (59). By blocking T cell exhaustion, effector T cells regain cytolytic function, including CXCR5+CD8 T cells. CXCR5+CD8 T cells initiate a proliferative burst of effector T cells following PD-1 immunotherapy administration in LCMV (11). This reinvigoration increases tumor destruction plus prolongs overall survival, approximately 21 months, in many cancer types: melanoma, non-small cell lung, renal cell, head and neck squamous cell, bladder, Merkel cell, hepatocellular, Hodgkin's lymphoma and more (60, 61). Of the patients approved for treatment with ICBs only 15-20% experience cancer remission and majority develop IMAEs or immune-related adverse events following treatment (62).
IMAEs are adverse events resembling organ specific to systemic autoimmunity and cause patients to end treatment early (63). IMAE biomarker development has focused on autoantibodies associated with known autoimmune conditions, such as Anti-Smith, Anti-dsDNA, ACPAs, Rh factor and more, however, these autoimmune biomarkers are poorly defined in cancer and vary in their baseline associations of IMAE outcomes (64). To account for the large autoantibody repertoire for identifying IMAE progression, blood serum from ICB treated patients could be collected pre- and post-treatment to establish a baseline and standardized by high-throughput proteome techniques (65–67). Current studies have utilized microarray, SEREX cDNA library, immunofluorescence, and immunoassay technologies for screening of IMAE associated autoantibodies, such as thyroiditis, hypophysitis, rash, colitis, arthritis, myocarditis, myalgia, and endocrine disorders in response to singular/combination treatments of CTLA4, PD-1 and PD-L1 in melanoma, advanced/metastatic solid tumors, renal cell carcinoma, non-small cell lung, prostate, and bladder cancers (68–73).
CXCR5+CD8 T cells correlate with disease-free or overall survival in pancreatic, colon, follicular lymphoma, gastric, high-grade serous ovarian, hepatocellular, and bladder cancers and thus is considered a potential biomarker (15, 17, 20, 23, 25, 26). On the contrary, decreases in disease-free survival in non-small cell lung and salivary adenoid cystic carcinomas are associated with infiltrating CXCR5+CD8 T cells (74, 75). Functional studies beyond correlative data and mRNA expression remains lacking as to how these cells benefit or negate a tumor microenvironment. Chemokine, CXCR5, knockdown has proven to have negative prognostic value in certain cancers and cell lines (76). Tumors express CXCR5 and produce its ligand, CXCL13, to recruit B cells and T cells into tumor microenvironments where tertiary lymphoid structures, resembling germinal centers, are developed and antibody production occurs (77–79). CXCR5 promotes proliferation of clear cell renal carcinoma through activating PI3K/AKT/mTOR pathway in the presence of its ligand, CXCL13, and this signaling pathway has also been reported in colon cancer proliferation (76, 80). CXCL13-CXCR5 axis in tumors leads to increased migration, invasion of tumor cells and unfavorable tumor prognostic values in patients, but not in the case of ICB treatment (77, 79, 81). In patients treated with ICB, high CXCL13 expression leads to more favorable tumor outcomes perhaps due to CXCR5+CD8 T cells expanding the effector T cell pool or as a consequence of these cells increasing proliferation while resisting apoptosis (11, 82). CXCR5+CD8 T cell persistence following ICB may generate a greater antibody repertoire based on their known B cell interactions that could be beneficial for tumor eradication or generate organ specific detriments and IMAEs (1, 79, 83).
CXCR5+CD8 T cell ability to initiate IMAEs during ICB remains unknown. These cells are capable of upregulating effector molecules in cancer and lysing tumor cells in co-culture. In autoimmune disease, CXCR5+CD8 T cells enable B cell differentiation and exacerbate disease (1, 2). Correlations exist based on immune cell infiltration and IMAE development in patients without a complete understanding of tolerance mechanisms. For example, granzyme B producing CD4 T cells infiltrate thyroids in response to anti-PD1 therapy at a greater frequency than CD8 T cells and are associated with development of mouse and human thyroiditis (84). Production of IL-21, CXCL13, and infiltration by cytolytic CD4 and CD8 T cell infiltrates associate with IMAE development in tumor models and human plasma (85). Whether or not these cells express CXCR5 remains unknown but their ability to produce granzyme B, IL-21, and migrate to CXCL13 warrants further CXCR5+ T cell research in IMAE development. A direct link between IMAEs and autoimmune mechanisms is currently unknown. PD-1 elimination and ICB accelerates autoimmunity in murine models of systemic lupus erythematosus and autoimmune diabetes (86, 87). Reversal of inhibitory tolerance mechanisms is capable of reactivating T cell receptors to recognize self-antigen (86). Autoreactive CD8 T cell activation after PD-1 ICB induces autoimmune diabetes in Rip-mOVA mice indicating that loss of CD8 T cell self-tolerance following immune modulation therapies increases autoimmunity (88). Additional studies are needed to determine if self-reactive cells lose tolerance and transform into active effector T cells capable of cytolytic activity on tumor and self-tissues. Also, research is needed to determine if initiation of IMAEs and autoimmunity occur via similar or distinct pathways.
Discussion
CXCR5+CD8 T cell research is expanding in multiple areas as they are identified in advanced technologies, such as single-cell sequencing. As with most areas of cancer research heterogeneity between cancer types, biomarkers, and tumor microenvironments creates cumbersome and often contrasting phenotypic conclusions regarding CXCR5+CD8 T cell functions. CXCR5+CD8 T cells are a newly developing area of study that allow for a unique perspective and growth in the fields of cancer research and immune checkpoint blockade. ICB research is now focused on combining treatment modulations to identify ways to overcome immune-mediated adverse events. Awareness and focus on IMAEs is in its initial stages. How CXCR5+CD8 T cells affect the tumor microenvironment, B cell infiltration, autoantibody response, T cell tissue residency, and peripheral organ involvement after ICB remains unknown. Defining functionality in these different areas, particularly identifying how these cells respond in cancer initiation and progression, would provide a major step forward. We speculate that CXCR5+CD8 T cells may slowly migrate into circulation after ICB treatment but become primed and activated by dendritic cells in peripheral organs generating an effector phenotype that then infiltrates tissues and establishes IMAEs (45, 86, 89). Because of cancer heterogeneity, identifying CXCR5+CD8 T cells requires a robust screen across multiple cancer types to enable a sound and clear prognostic value for using CXCR5+CD8 T cells as a biomarker or immune cell therapy target.
Questions about CXCR5+CD8 T cell effects on IMAEs remain. CXCR5+CD8 T cell ability to interact with B cells and promote antibodies suggests that some CXCR5+CD8 T cells, after ICB, could reverse tolerance and initiate autoantibody production. Data from multiple studies now suggests that PD-1 blockade releases self-tolerance constraints increasing autoimmunity. As ICB induces IMAEs, it raises the question of whether IMAEs are caused by autoimmune mechanisms or some other immune dysfunction. With sequencing techniques advancing, teasing out the implications of protein antigens from tumor proteins or self-proteins would delineate if CXCR5+CD8 T cells pose a threat to the development of IMAEs (10). Studying CXCR5+CD8 T cells using animal models that perpetuate autoimmunity would explain how these cells respond to ICB and if they initiate disease development. An autoimmune disease model, B6/lpr, treated with combination ICB in the presence of colon adenocarcinoma develops T cell immune-infiltration of multiple organs with increased tumor growth following steroid treatment (90). Corticosteroids are a drug of choice when patients develop IMAEs but based on this study could result in decreased ICB effectiveness. Promoting tolerance mechanisms in T cells while patients receive ICB may enhance therapy effectiveness. VISTA regulates tolerance mechanisms and its loss induces autoimmune disease in mice, by incorporating a VISTA agonist along with ICB may enhance cancer therapy while eliminating IMAEs (91, 92). In humanized mice, CXCR5+CD8 T cells develop after human cord blood hematopoietic stem cell engraftment and HIV infection while maintaining PD-1, cytotoxicity, cytokine production, and homing to peripheral organs (93). These studies provide novel models for elucidating CXCR5+CD8 T cells in the context of mouse and human responses, in addition to, existing human tumor resections and peripheral blood mononuclear cells for translational research.
Author contributions
CT and GM conceptualization, literature evaluation, original draft writing, and generated and visualized figures. KH conceptualization, writing and review, visualization, funding acquisition, and supervision. All authors contributed to the article and approved the submitted version.
Funding
This work was supported by the National Institutes of Health Grant R15HL146779 to KH.
Acknowledgments
The authors thank Maria Pimentel for her assistance with reference management. All figures were created with BioRender.com.
Conflict of interest
The authors declare that the research was conducted in the absence of any commercial or financial relationships that could be construed as a potential conflict of interest.
Publisher's note
All claims expressed in this article are solely those of the authors and do not necessarily represent those of their affiliated organizations, or those of the publisher, the editors and the reviewers. Any product that may be evaluated in this article, or claim that may be made by its manufacturer, is not guaranteed or endorsed by the publisher.
Supplementary material
The Supplementary Material for this article can be found online at: https://www.frontiersin.org/articles/10.3389/fmed.2022.1034764/full#supplementary-material
References
1. Valentine KM, Davini D, Lawrence TJ, Mullins GN, Manansala M, Al-Kuhlani M, et al. CD8 follicular T cells promote B cell antibody class switch in autoimmune disease. J Immunol. (2018) 201:31–40. doi: 10.4049/jimmunol.1701079
2. Valentine KM, Mullins GN, Davalos OA, Seow LW, Hoyer KK. CD8 follicular T cells localize throughout the follicle during germinal center reactions and maintain cytolytic and helper properties. J Autoimmun. (2021) 123:102690. doi: 10.1016/j.jaut.2021.102690
3. Li Y, Tang L, Guo L, Chen C, Gu S, Zhou Y, et al. CXCL13-mediated recruitment of intrahepatic CXCR5+CD8+ T cells favors viral control in chronic HBV infection. J Hepatol. (2020) 72:420–30. doi: 10.1016/j.jhep.2019.09.031
4. Tyllis TS, Fenix KA, Norton TS, Kara EE, McKenzie DR, David SC, et al. CXCR5+CD8+ T cells shape antibody responses in vivo following protein immunisation and peripheral viral infection. Front Immunol. (2021) 12:1–14. doi: 10.3389/fimmu.2021.626199
5. Chen Y, Yu M, Zheng Y, Fu G, Xin G, Zhu W, et al. CXCR5+PD-1+ follicular helper CD8 T cells control B cell tolerance. Nat Commun. (2019) 10:4415. doi: 10.1038/s41467-019-12446-5
6. Shi J, Hou S, Fang Q, Liu X, Liu X, Qi H. PD-1 Controls follicular T helper cell positioning and function. Immunity. (2018) 49:264–74. doi: 10.1016/j.immuni.2018.06.012
7. Cao G, Chi S, Wang X, Sun J, Zhang Y. CD4+CXCR5+PD-1+ T follicular helper cells play a pivotal role in the development of rheumatoid arthritis. Med Sci Monit Int Med J Exp Clin Res. (2019) 25:3032–40. doi: 10.12659/MSM.914868
8. Crotty S. Follicular helper CD4 T cells (TFH). Annu Rev Immunol. (2011) 29:621–63. doi: 10.1146/annurev-immunol-031210-101400
9. Spaan M, Kreefft K, Graav GN de, Brouwer WP, Knegt RJ de, Kate FJW, et al. CD4+CXCR5+ T cells in chronic HCV infection produce less IL-21, yet are efficient at supporting B cell responses. J Hepatol. (2015) 62:303–10. doi: 10.1016/j.jhep.2014.09.024
10. Brummelman J, Mazza EMC, Alvisi G, Colombo FS, Grilli A, Mikulak J, et al. High-dimensional single cell analysis identifies stem-like cytotoxic CD8+ T cells infiltrating human tumors. J Exp Med. (2018) 215:2520–35. doi: 10.1084/jem.20180684
11. Im SJ, Hashimoto M, Gerner MY, Lee J, Kissick HT, Burger MC, et al. Defining CD8+ T cells that provide the proliferative burst after PD-1 therapy. Nature. (2016) 537:417–21. doi: 10.1038/nature19330
12. Lei X, Lei Y, Li J-K, Du W-X, Li R-G, Yang J, et al. Immune cells within the tumor microenvironment: biological functions and roles in cancer immunotherapy. Cancer Lett. (2020) 470:126–33. doi: 10.1016/j.canlet.2019.11.009
13. Gravano DM, Hoyer KK. Promotion and prevention of autoimmune disease by CD8+ T cells. J Autoimmun. (2013) 45:68–79. doi: 10.1016/j.jaut.2013.06.004
14. Zhang N, Bevan MJ. CD8+ T Cells: foot soldiers of the immune system. Immunity. (2011) 35:161–8. doi: 10.1016/j.immuni.2011.07.010
15. Yang M, Lu J, Zhang G, Wang Y, He M, Xu Q, et al. CXCL13 shapes immunoactive tumor microenvironment and enhances the efficacy of PD-1 checkpoint blockade in high-grade serous ovarian cancer. J Immunother Cancer. (2021) 9:e001136. doi: 10.1136/jitc-2020-001136
16. Simon S, Voillet V, Vignard V, Wu Z, Dabrowski C, Jouand N, et al. PD-1 and TIGIT coexpression identifies a circulating CD8 T cell subset predictive of response to anti-PD-1 therapy. J Immunother Cancer. (2020) 8:e001631. doi: 10.1136/jitc-2020-001631
17. Bai M, Zheng Y, Liu H, Su B, Zhan Y, He H. CXCR5+ CD8+ T cells potently infiltrate pancreatic tumors and present high functionality. Exp Cell Res. (2017) 361:39–45. doi: 10.1016/j.yexcr.2017.09.039
18. Xing J, Li X. E J, Wang C, Wang H. Inverse relationship between CD40L expression and cytolytic molecule expression by CD8+CXCR5+ T follicular cytotoxic cells in colorectal cancer. Exp Cell Res. (2020) 389:111892. doi: 10.1016/j.yexcr.2020.111892
19. Tang J, Zha J, Guo X, Shi P, Xu B. CXCR5+CD8+ T cells present elevated capacity in mediating cytotoxicity toward autologous tumor cells through interleukin 10 in diffuse large B-cell lymphoma. Int Immunopharmacol. (2017) 50:146–51. doi: 10.1016/j.intimp.2017.06.020
20. Huang Q, Zhou Q, Zhang H, Liu Z, Zeng H, Chen Y, et al. Identification and validation of an excellent prognosis subtype of muscle-invasive bladder cancer patients with intratumoral CXCR5+ CD8+ T cell abundance. Oncoimmunology. (n.d.) 9:1810489. doi: 10.1080/2162402X.2020.1810489
21. Jin Y, Lang C, Tang J, Geng J, Song HK, Sun Z, et al. CXCR5+CD8+ T cells could induce the death of tumor cells in HBV-related hepatocellular carcinoma. Int Immunopharmacol. (2017) 53:42–8. doi: 10.1016/j.intimp.2017.10.009
22. Xing J, Zhang C, Yang X, Wang S, Wang Z, Li X, et al. CXCR5+CD8+ T cells infiltrate the colorectal tumors and nearby lymph nodes, and are associated with enhanced IgG response in B cells. Exp Cell Res. (2017) 356:57–63. doi: 10.1016/j.yexcr.2017.04.014
23. E J, Yan F, Kang Z, Zhu L, Xing J, Yu E. CD8+CXCR5+ T cells in tumor-draining lymph nodes are highly activated and predict better prognosis in colorectal cancer. Hum Immunol. (2018) 79:446–452. doi: 10.1016/j.humimm.2018.03.003
24. Zhou Y, Guo L, Sun H, Xu J, Ba T. CXCR5+ CD8 T cells displayed higher activation potential despite high PD-1 expression, in tumor-involved lymph nodes from patients with thyroid cancer. Int Immunopharmacol. (2018) 62:114–9. doi: 10.1016/j.intimp.2018.07.002
25. Chu F, Li HS, Liu X, Cao J, Ma W, Ma Y, et al. CXCR5+CD8+ T cells are a distinct functional subset with antitumor activity. Leukemia. (2019) 33:2640–53. doi: 10.1038/s41375-019-0464-2
26. Wang J, Li R, Cao Y, Gu Y, Fang H, Fei Y, et al. Intratumoral CXCR5+CD8+T associates with favorable clinical outcomes and immunogenic contexture in gastric cancer. Nat Commun. (2021) 12:3080. doi: 10.1038/s41467-021-23356-w
27. Hofland T, Martens AWJ, van Bruggen JAC, de Boer R, Schetters S, Remmerswaal EBM, et al. Human CXCR5+PD-1+ CD8 T cells in healthy individuals and patients with hematologic malignancies. Eur J Immunol. (2021) 51:703–13. doi: 10.1002/eji.202048761
28. Valentine KM, Hoyer KK. CXCR5+ CD8 T cells: protective or pathogenic? Front Immunol. (2019) 10:1–10. doi: 10.3389/fimmu.2019.01322
29. Ye L, Li Y, Tang H, Liu W, Chen Y, Dai T, et al. CD8+CXCR5+T cells infiltrating hepatocellular carcinomas are activated and predictive of a better prognosis. Aging. (2019) 11:8879–91. doi: 10.18632/aging.102308
30. Good-Jacobson KL, Szumilas CG, Chen L, Sharpe AH, Tomayko MM, Shlomchik MJ. PD-1 regulates germinal center B cell survival and the formation and affinity of long-lived plasma cells. Nat Immunol. (2010) 11:535–42. doi: 10.1038/ni.1877
31. Kao C, Oestreich KJ, Paley MA, Crawford A, Angelosanto JM, Ali MA, et al. T-bet represses expression of PD-1 and sustains virus-specific CD8 T cell responses during chronic infection. Nat Immunol. (2011) 12:663–71. doi: 10.1038/ni.2046
32. Wherry EJ, Kurachi M. Molecular and cellular insights into T cell exhaustion. Nat Rev Immunol. (2015) 15:486–99. doi: 10.1038/nri3862
33. Verdon DJ, Mulazzani M, Jenkins MR. Cellular and molecular mechanisms of CD8+ T cell differentiation, dysfunction, and exhaustion. Int J Mol Sci. (2020) 21:7357. doi: 10.3390/ijms21197357
34. Kallies A, Zehn D, Utzschneider DT. Precursor exhausted T cells: key to successful immunotherapy? Nat Rev Immunol. (2020) 20:128–36. doi: 10.1038/s41577-019-0223-7
35. Bengsch B, Ohtani T, Herati RS, Bovenschen N, Chang KM, Wherry EJ. Deep immune profiling by mass cytometry links human T and NK cell differentiation and cytotoxic molecule expression patterns. J Immunol Methods. (2018) 453:3–10. doi: 10.1016/j.jim.2017.03.009
36. Wherry EJ, Blattman JN, Murali-Krishna K, van der Most R, Ahmed R. Viral persistence alters CD8 T-cell immunodominance and tissue distribution and results in distinct stages of functional impairment. J Virol. (2003) 77:4911–27. doi: 10.1128/JVI.77.8.4911-4927.2003
37. Radoja S, Saio M, Frey AB. CD8+ Tumor-infiltrating lymphocytes are primed for fas-mediated activation-induced cell death but are not apoptotic in situ. J Immunol. (2001) 166:6074–83. doi: 10.4049/jimmunol.166.10.6074
38. Zhang J, Bárdos T, Mikecz K, Finnegan A, Glant TT. Impaired Fas signaling pathway is involved in defective T cell apoptosis in autoimmune murine arthritis. J Immunol. (2001) 166:4981–6. doi: 10.4049/jimmunol.166.8.4981
39. Gargett T, Yu W, Dotti G, Yvon ES, Christo SN, Hayball JD, et al. GD2-specific CAR T cells undergo potent activation and deletion following antigen encounter but can be protected from activation-induced cell death by PD-1 blockade. Mol Ther. (2016) 24:1135–49. doi: 10.1038/mt.2016.63
40. Perez-Ruiz E, Minute L, Otano I, Alvarez M, Ochoa MC, Belsue V, et al. Prophylactic TNF blockade uncouples efficacy and toxicity in dual CTLA-4 and PD-1 immunotherapy. Nature. (2019) 569:428–32. doi: 10.1038/s41586-019-1162-y
41. Yao C, Sun H-W, Lacey NE Ji Y, Moseman EA, Shih H-Y, et al. Single-Cell RNA-Seq reveals TOX as a key regulator of CD8+ T cell persistence in chronic infection. Nat Immunol. (2019) 20:890–901. doi: 10.1038/s41590-019-0403-4
42. Mitchell AM, Michels AW. T cell receptor sequencing in autoimmunity. J Life Sci Westlake Village Calif. (2020) 2:38–58. doi: 10.36069/JoLS/20201203
43. Shen Y, Qu Q, Jin M, Chen C. Investigating the role of circulating CXCR5-expressing CD8+ T-cells as a biomarker for bacterial infection in subjects with pneumonia. Respir Res. (2019) 20:54. doi: 10.1186/s12931-019-1011-4
44. Beltra J-C, Manne S, Abdel-Hakeem MS, Kurachi M, Giles JR, Chen Z, et al. Developmental relationships of four exhausted CD8+ T cell subsets reveals underlying transcriptional and epigenetic landscape control mechanisms. Immunity. (2020) 52:825–41. doi: 10.1016/j.immuni.2020.04.014
45. Herve M-G de G de, Abdoh M, Jaafoura S, Durali D, Taoufik Y. Follicular CD4 t cells tutor CD8 early memory precursors: an initiatory journey to the frontier of B cell territory. iScience. (2019) 20:100–9. doi: 10.1016/j.isci.2019.09.012
46. Im SJ, Konieczny BT, Hudson WH, Masopust D, Ahmed R. PD-1+ stemlike CD8 T cells are resident in lymphoid tissues during persistent LCMV infection. Proc Natl Acad Sci. (2020) 117:4292–9. doi: 10.1073/pnas.1917298117
47. Herndler-Brandstetter D, Ishigame H, Shinnakasu R, Plajer V, Stecher C, Zhao J, et al. KLRG1+ Effector CD8+ T cells lose KLRG1, differentiate into all memory T cell lineages, and convey enhanced protective immunity. Immunity. (2018) 48:716–29. doi: 10.1016/j.immuni.2018.03.015
48. Hudson WH, Gensheimer J, Hashimoto M, Wieland A, Valanparambil RM, Li P, et al. Proliferating transitory T cells with an effector-like transcriptional signature emerge from PD-1+ stem-like CD8+ T cells during chronic infection. Immunity. (2019) 51:1043–58. doi: 10.1016/j.immuni.2019.11.002
49. He R, Hou S, Liu C, Zhang A, Bai Q, Han M, et al. Follicular CXCR5-expressing CD8+ T cells curtail chronic viral infection. Nature. (2016) 537:412–6. doi: 10.1038/nature19317
50. Shi W, Yang B, Sun Q, Meng J, Zhao X, Du S, et al. PD-1 regulates CXCR5+ CD4 T cell-mediated proinflammatory functions in non-small cell lung cancer patients. Int Immunopharmacol. (2020) 82:106295. doi: 10.1016/j.intimp.2020.106295
51. Yang Z-Z, Grote DM, Ziesmer SC, Xiu B, Novak AJ, Ansell SM. PD-1 expression defines two distinct T-cell sub-populations in follicular lymphoma that differentially impact patient survival. Blood Cancer J. (2015) 5:e281. doi: 10.1038/bcj.2015.1
52. Martinez-Usatorre A, Carmona SJ, Godfroid C, Yacoub Maroun C, Labiano S, Romero P. Enhanced phenotype definition for precision isolation of precursor exhausted tumor-infiltrating CD8 T cells. Front Immunol. (2020) 11:340. doi: 10.3389/fimmu.2020.00340
53. Zimmerer JM, Ringwald BA, Elzein SM, Avila CL, Warren RT, Abdel-Rasoul M, et al. Antibody-suppressor CD8+ T cells require CXCR5. Transplantation. (2019) 103:1809–20. doi: 10.1097/TP.0000000000002683
54. Zimmerer JM, Basinger MW., Ringwald BA, Abdel-Rasoul M, Pelletier RP, Rajab A, et al. Inverse association between the quantity of human peripheral blood CXCR5+IFN-γ+CD8+T cells with De Novo DSA production in the first year after kidney transplant. Transplantation. (2020) 104:2424–34. doi: 10.1097/TP.0000000000003151
55. Grebinoski S, Zhang Q, Cillo AR, Manne S, Xiao H, Brunazzi EA, et al. Autoreactive CD8+ T cells are restrained by an exhaustion-like program that is maintained by LAG3. Nat Immunol. (2022) 23:868–77. doi: 10.1038/s41590-022-01210-5
56. Crowl JT, Heeg M, Ferry A, Milner JJ, Omilusik KD, Toma C, et al. Tissue-resident memory CD8+ T cells possess unique transcriptional, epigenetic and functional adaptations to different tissue environments. Nat Immunol. (2022) 23:1121–31. doi: 10.1038/s41590-022-01229-8
57. Rao DA, Gurish MF, Marshall JL, Slowikowski K, Fonseka CY, Liu Y, et al. Pathologically expanded peripheral T helper cell subset drives B cells in rheumatoid arthritis. Nature. (2017) 542:110–4. doi: 10.1038/nature20810
58. Hartzell S, Bin S, Cantarelli C, Haverly M, Manrique J, Angeletti A, et al. Kidney failure associates with T cell exhaustion and imbalanced follicular helper T cells. Front Immunol. (2020) 11.
59. Waldman AD, Fritz JM, Lenardo MJ. A guide to cancer immunotherapy: from T cell basic science to clinical practice. Nat Rev Immunol. (2020) 20:651–68. doi: 10.1038/s41577-020-0306-5
60. Esfahani K, Roudaia L, Buhlaiga N, Del Rincon SV, Papneja N, Miller WH, et al. Review of cancer immunotherapy: from the past, to the present, to the future. Curr Oncol. (2020) 27:S87–97. doi: 10.3747/co.27.5223
61. Sharma P, Hu-Lieskovan S, Wargo JA, Ribas A. Primary, adaptive, and acquired resistance to cancer immunotherapy. Cell. (2017) 168:707–23. doi: 10.1016/j.cell.2017.01.017
62. Haslam A, Prasad V. Estimation of the percentage of US patients with cancer who are eligible for and respond to checkpoint inhibitor immunotherapy drugs. JAMA Netw Open. (2019) 2:e192535. doi: 10.1001/jamanetworkopen.2019.2535
63. Postow MA, Sidlow R, Hellmann MD. Immune-related adverse events associated with immune checkpoint blockade. N Engl J Med. (2018) 378:158–68. doi: 10.1056/NEJMra1703481
64. Ghosh N, Chan KK, Jivanelli B, Bass AR. Autoantibodies in patients with immune-related adverse events from checkpoint inhibitors: a systematic literature review. JCR J Clin Rheumatol. (2022) 28:e498. doi: 10.1097/RHU.0000000000001777
65. Yu R, Yang S, Liu Y, Zhu Z. Identification and validation of serum autoantibodies in children with B-cell acute lymphoblastic leukemia by serological proteome analysis. Proteome Sci. (2022) 20:3. doi: 10.1186/s12953-021-00184-w
66. Wang Z, Liu X, Muther J, James JA, Smith K, Wi S. Top-down mass spectrometry analysis of human serum autoantibody antigen-binding fragments. Sci Rep. (2019) 9:2345. doi: 10.1038/s41598-018-38380-y
67. Ignjatovic V, Geyer PE, Palaniappan KK, Chaaban JE, Omenn GS, Baker MS, et al. Mass spectrometry-based plasma proteomics: considerations from sample collection to achieving translational data. J Proteome Res. (2019) 18:4085–97. doi: 10.1021/acs.jproteome.9b00503
68. Tahir SA, Gao J, Miura Y, Blando J, Tidwell RSS, Zhao H, et al. Autoimmune antibodies correlate with immune checkpoint therapy-induced toxicities. Proc Natl Acad Sci. (2019) 116:22246–51. doi: 10.1073/pnas.1908079116
69. Purde M-T, Niederer R, Wagner NB, Diem S, Berner F, Hasan Ali O, et al. Presence of autoantibodies in serum does not impact the occurrence of immune checkpoint inhibitor-induced hepatitis in a prospective cohort of cancer patients. J Cancer Res Clin Oncol. (2022) 148:647–56. doi: 10.1007/s00432-021-03870-6
70. Labadzhyan A, Wentzel K, Hamid O, Chow K, Kim S, Piro L, et al. Endocrine Autoantibodies determine immune checkpoint inhibitor-induced endocrinopathy: a prospective study. J Clin Endocrinol Metab. (2022) 107:1976–82. doi: 10.1210/clinem/dgac161
71. Gowen MF, Giles KM, Simpson D, Tchack J, Zhou H, Moran U, et al. Baseline antibody profiles predict toxicity in melanoma patients treated with immune checkpoint inhibitors. J Transl Med. (2018) 16:82. doi: 10.1186/s12967-018-1452-4
72. Ghosh N, Postow M, Zhu C, Jannat-Khah D, Li Q-Z, Vitone G, et al. Lower baseline autoantibody levels are associated with immune-related adverse events from immune checkpoint inhibition. J Immunother Cancer. (2022) 10:e004008. doi: 10.1136/jitc-2021-004008
73. de Moel EC, Rozeman EA, Kapiteijn EH, Verdegaal EME, Grummels A, Bakker JA, et al. Autoantibody development under treatment with immune-checkpoint inhibitors. Cancer Immunol Res. (2019) 7:6–11. doi: 10.1158/2326-6066.CIR-18-0245
74. Yue Z, Ningning D, Lin Y, Jianming Y, Hongtu Z, Ligong Y, et al. Correlation between CXCR4, CXCR5 and CCR7 expression and survival outcomes in patients with clinical T1N0M0 non-small cell lung cancer. Thorac Cancer. (2020) 11:2955–65. doi: 10.1111/1759-7714.13645
75. Zhang M, Wu J-S, Xian H-C, Chen B-J, Wang H-F, Yu X-H, et al. CXCR5 induces perineural invasion of salivary adenoid cystic carcinoma by inhibiting microRNA-187. Aging. (2021) 13:15384–99. doi: 10.18632/aging.203097
76. Zheng Z, Cai Y, Chen H, Chen Z, Zhu D, Zhong Q, et al. CXCL13/CXCR5 Axis predicts poor prognosis and promotes progression through PI3K/AKT/MTOR pathway in clear cell renal cell carcinoma. Front Oncol. (2019) 8:1–10. doi: 10.3389/fonc.2018.00682
77. Tan P, Shi M, Lai L, Tang Z, Xie N, Xu H, et al. Regulative role of the CXCL13-CXCR5 axis in the tumor microenvironment. Precis Clin Med. (2018) 1:49–56.
78. Hsieh C-H, Jian C-Z, Lin L-I, Low G-S, Ou P-Y, Hsu C, et al. Potential role of CXCL13/CXCR5 signaling in immune checkpoint inhibitor treatment in cancer. Cancers. (2022) 14:294. doi: 10.3390/cancers14020294
79. Meylan M, Petitprez F, Becht E, Bougoüin A, Pupier G, Calvez A, et al. Tertiary lymphoid structures generate and propagate anti-tumor antibody-producing plasma cells in renal cell cancer. Immunity. (2022) 55:527–41. doi: 10.1016/j.immuni.2022.02.001
80. Zhu Z, Zhang X, Guo H, Fu L, Pan G, Sun Y. CXCL13-CXCR5 axis promotes the growth and invasion of colon cancer cells via PI3K/AKT pathway. Mol Cell Biochem. (2015) 400:287–95. doi: 10.1007/s11010-014-2285-y
81. Chen L, Huang Z, Yao G, Lyu X, Li J, Hu X, et al. The expression of CXCL13 and its relation to unfavorable clinical characteristics in young breast cancer. J Transl Med. (2015) 13:168. doi: 10.1186/s12967-015-0521-1
82. Siddiqui I, Schaeuble K, Chennupati V, Fuertes Marraco SA, Calderon-Copete S, Pais Ferreira D, et al. Intratumoral Tcf1+PD-1+CD8+ T cells with stem-like properties promote tumor control in response to vaccination and checkpoint blockade immunotherapy. Immunity. (2019) 50:195–211.e10. doi: 10.1016/j.immuni.2018.12.021
83. Liudahl SM, Coussens LM. B cells as biomarkers: predicting immune checkpoint therapy adverse events. J Clin Invest. (2018) 128:577–9. doi: 10.1172/JCI99036
84. Yasuda Y, Iwama S, Sugiyama D, Okuji T, Kobayashi T, Ito M, et al. CD4+ T cells are essential for the development of destructive thyroiditis induced by anti–PD-1 antibody in thyroglobulin-immunized mice. Sci Transl Med. (2021) 13:eabb7495. doi: 10.1126/scitranslmed.abb7495
85. Tsukamoto H, Komohara Y, Tomita Y, Miura Y, Motoshima T, Imamura K, et al. Aging-associated and CD4 T-cell–dependent ectopic CXCL13 activation predisposes to anti–PD-1 therapy-induced adverse events. Proc Natl Acad Sci. (2022) 119:e2205378119. doi: 10.1073/pnas.2205378119
86. Fife BT, Pauken KE, Eagar TN, Obu T, Wu J, Tan Q, et al. Interactions between PD-1 and PD-L1 promote tolerance by blocking the TCR–induced stop signal. Nat Immunol. (2009) 10:1185–92. doi: 10.1038/ni.1790
87. Nishimura H, Nose M, Hiai H, Minato N, Honjo T. Development of lupus-like autoimmune diseases by disruption of the PD-1 gene encoding an ITIM motif-carrying immunoreceptor. Immunity. (1999) 11:141–51. doi: 10.1016/S1074-7613(00)80089-8
88. Martin-Orozco N, Wang Y-H, Yagita H, Dong C. Cutting edge: programed death (PD) ligand-1/PD-1 interaction is required for CD8+ T cell tolerance to tissue antigens. J Immunol. (2006) 177:8291–5. doi: 10.4049/jimmunol.177.12.8291
89. Tilstra JS, Avery L, Menk AV, Gordon RA, Smita S, Kane LP, et al. Kidney-infiltrating T cells in murine lupus nephritis are metabolically and functionally exhausted. J Clin Invest. (2018) 128:4884–97. doi: 10.1172/JCI120859
90. Adam K, Iuga A, Tocheva AS, Mor A. A novel mouse model for checkpoint inhibitor-induced adverse events. PLoS ONE. (2021) 16:e0246168. doi: 10.1371/journal.pone.0246168
91. Han X, Vesely MD, Yang W, Sanmamed MF, Badri T, Alawa J, et al. PD-1H (VISTA)–mediated suppression of autoimmunity in systemic and cutaneous lupus erythematosus. Sci Transl Med. (2019) 11:eaax1159. doi: 10.1126/scitranslmed.aax1159
92. Pourakbari R, Hajizadeh F, Parhizkar F, Aghebati-Maleki A, Mansouri S, Aghebati-Maleki L. Co-stimulatory agonists: an insight into the immunotherapy of cancer. Excli J. (2021) 20:1055–85.
Keywords: immunotherapy (ICB), immune-related adverse events (IRAE), immune-mediated adverse events (IMAE), CD4 T follicular helper (CD4 Tfh), T follicular helper (Tfh), CD8 Tfc, CXCR5+ CD8 T cell, autoimmune disease
Citation: Turner CN, Mullins GN and Hoyer KK (2022) CXCR5+CD8 T cells: Potential immunotherapy targets or drivers of immune-mediated adverse events? Front. Med. 9:1034764. doi: 10.3389/fmed.2022.1034764
Received: 02 September 2022; Accepted: 23 September 2022;
Published: 13 October 2022.
Edited by:
Bernhard F. Gibbs, University of Oldenburg, GermanyReviewed by:
Vadim V. Sumbayev, University of Kent, United KingdomCopyright © 2022 Turner, Mullins and Hoyer. This is an open-access article distributed under the terms of the Creative Commons Attribution License (CC BY). The use, distribution or reproduction in other forums is permitted, provided the original author(s) and the copyright owner(s) are credited and that the original publication in this journal is cited, in accordance with accepted academic practice. No use, distribution or reproduction is permitted which does not comply with these terms.
*Correspondence: Katrina K. Hoyer, khoyer2@ucmerced.edu
†These authors have contributed equally to this work and share first authorship
‡ORCID: Christi N. Turner orcid.org/0000-0003-2314-6689
Genevieve N. Mullins orcid.org/0000-0003-1646-5710
Katrina K. Hoyer orcid.org/0000-0001-5443-0733