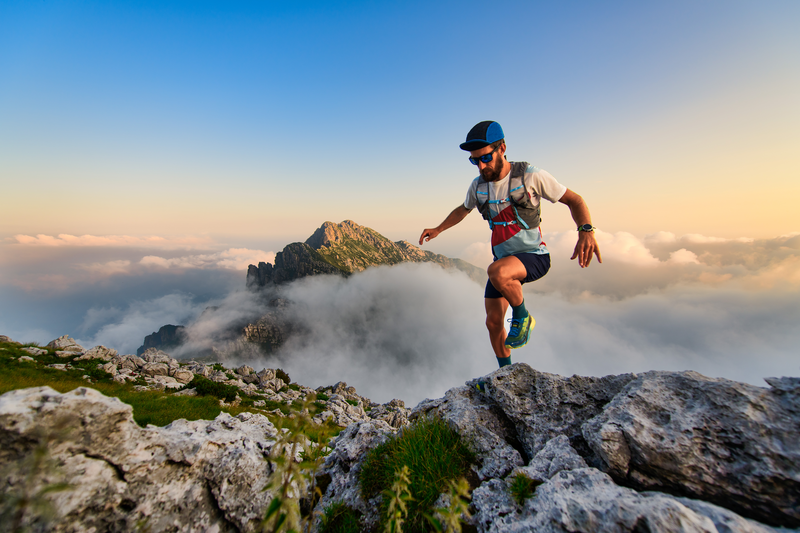
94% of researchers rate our articles as excellent or good
Learn more about the work of our research integrity team to safeguard the quality of each article we publish.
Find out more
ORIGINAL RESEARCH article
Front. Med. , 10 November 2022
Sec. Ophthalmology
Volume 9 - 2022 | https://doi.org/10.3389/fmed.2022.1025853
This article is part of the Research Topic Vascular Involvement in Eye Diseases View all 15 articles
Aims/Hypothesis: There is evidence that diabetes is accompanied by a break-down of functional hyperemia, an intrinsic mechanism of neural tissues to adapt blood flow to changing metabolic demands. However, to what extent functional hyperemia is altered in different stages of diabetic retinopathy (DR) in patients with type II diabetes is largely unknown. The current study set out to investigate flicker-induced retinal blood flow changes in patients with type II diabetes at different stages of DR.
Materials and methods: A total of 76 subjects were included in the present parallel-group study, of which 56 had diabetes with either no DR or different stages of non-proliferative DR (n = 29 no DR, 12 mild DR, 15 moderate to severe DR). In addition, 20 healthy subjects were included as controls. Retinal blood flow was assessed before and during visual stimulation using a combined measurement of retinal vessel calibers and blood velocity by the means of Doppler optical coherence tomography (OCT). To measure systemic autonomic nervous system function, heart rate variability (HRV) was assessed using a short-term orthostatic challenge test.
Results: In healthy controls, retinal blood flow increased by 40.4 ± 27.2% during flicker stimulation. Flicker responses in patients with DR were significantly decreased depending on the stage of the disease (no DR 37.7 ± 26.0%, mild DR 26.2 ± 28.2%, moderate to severe DR 22.3 ± 13.9%; p = 0.035, ANOVA). When assessing systemic autonomous neural function using HRV, normalized low frequency (LF) spectral power showed a significantly different response to the orthostatic maneuver in diabetic patients compared to healthy controls (p < 0.001).
Conclusion/Interpretation: Our study indicates that flicker induced hyperemia is reduced in patients with DR compared to healthy subjects. Further, this impairment is more pronounced with increasing severity of DR. Further studies are needed to elucidate mechanisms behind the reduced hyperemic response in patients with type II diabetes.
Clinical trial registration: [https://clinicaltrials.gov/], identifier [NCT03 552562].
The incidence and prevalence of type II diabetes and its associated co-morbidities is constantly rising (1–3). Traditionally, diabetic complications are classified as macrovascular – leading to cardiovascular and peripheral vascular disease – and microvascular complications, the latter being characterized by the triade of nephropathy, neuropathy, and diabetic retinopathy (DR) (4). Although microvascular damage usually occurs early in the disease process, changes can be clinically silent for a long time before a symptomatic manifestation occurs. Thus, biomarkers of disease progress reflecting microvascular complications are still warranted to identify high-risk patients (5).
The eye has the unique advantage that the microvasculature can be assessed directly and non-invasively without the need for an injection of contrast agents or radiation exposure. As such, microvascular changes such as microaneurysms, hemorrhages, or hard exudates are well known clinical signs of DR that can easily be graded on the slit lamp or using fundus photography. Further, recently developed imaging devices such as optical coherence tomography (OCT) angiography show rarefication of retinal vessels (6–10) indicative for changes in retinal perfusion (11–15).
However, there is evidence that functional alterations may proceed structural changes in patients with diabetes. As such, it has been consistently shown in the brain as well as in the retina that functional hyperemia, a term describing the ability of the tissue to adapt blood flow to different metabolic demands is impaired in patients with diabetes. However, whereas this hyperemic response, also known as neuro-vascular coupling is difficult to investigate in the brain and requires costly investigations such as Positron emission tomography (PET) or functional magnetic resonance imaging (fMRI) (16–18), neuro-vascular coupling can be easily and none-invasively assessed at the level of the retina by visual stimulation with flickering light. As such, most (19–26) but not all (27) human studies indicate a breakdown of neuro-vascular coupling in patients with diabetes. However, previous studies on this topic were limited by the fact that only information regarding vessel diameter not volumetric blood flow was assessed. Thus, the retinal blood flow response to flicker stimulation in patients with type II diabetes is largely unknown (24).
The present study set out to extend our knowledge on neuro-vascular coupling in patients with type two diabetes and different stages of DR. To assess retinal blood flow under visual stimulation, a recently developed, custom-built Doppler OCT system was used. Based on the measurement of retinal vessel diameters and blood flow velocity our approach allows us to expand our knowledge and assess volumetric blood flow during visual stimulation (28, 29). In addition, the current study included a test for measuring autonomic nervous system function. This test assesses heart rate variability (HRV) (30–32) and allows to investigate whether alterations in the autonomic nervous system may correlate with the severity of DR.
The study protocol was approved by the Ethics Committee of the Medical University of Vienna, the national competent authorities and was conducted in compliance with the Declaration of Helsinki and Good Clinical Practice (GCP) guidelines of the European Union. All subjects, selected by the Department of Clinical Pharmacology, provided written informed consent before any study-related procedures were performed. All subjects passed a prestudy screening in the 4 weeks before the first study day including the following examinations: medical history, pregnancy test in women of childbearing potential, blood pressure and heart rate measurement, laboratory testing for HbA1c, blood glucose levels and hemoglobin, assessment of visual acuity, slit lamp biomicroscopy, indirect funduscopy, 7-standard field color fundus photography for grading of DR (33), and measurement of intraocular pressure (IOP) with Goldmann applanation tonometry. Subjects were not included, if any clinically significant medical condition (except diabetes type II in the patient group) was found as part of the screening examination. Exclusion criteria were ametropia of more than six diopters, smoking and history, or family history of epilepsy.
The current study was a prospective parallel group study with observer blinded analysis.
On the screening day and on the study day all subjects arrived in the morning after 7–8 h of sleep and abstained from alcohol and stimulating beverages containing xanthine derivates like tea, coffee, or cola-like drinks 12 h before the study day. At the beginning of the study day, a pregnancy test was performed in females of childbearing potential. Then, one drop of mydriaticum (Mydriaticum “Agepha,” Agepha, Vienna, Austria) was administered into the chosen study eye and a resting period of at least 20 min was scheduled before measurements were started to allow pupil dilatation and ensure constant hemodynamic conditions. Capillary blood glucose level measurements were performed using a glucose meter and a blood draw for laboratory testing for HbA1c was done. Then, retinal vessel diameters were measured at baseline and during flicker stimulation using the dynamic vessel analyzer (DVA) and retinal venous blood flow using DOCT was assessed. Blood pressure, heart rate, and IOP were measured before and after these measurements. Assessment of HRV was done as final study day investigation. Blood flow measurements were performed under light-adapted conditions.
Measurement with the DVA allows the real time determination of retinal vessel diameters in vivo. This commercially available system (IMEDOS, Jena, Germany) combines a fundus camera (FF 450; Carl Zeiss Meditec, Jena, Germany), a video camera, a real time monitor and a personal computer with an analyzing software to measure retinal and venous diameters (34). The device allows the evaluation of the diameters of one temporal retinal artery and vein between 1 and 2 disk diameters from the margin of the optic disk by analyzing digital pictures from a continuous video of the respective vessels (35).
Before flicker stimulation, a baseline recording for 60 s was performed. After the baseline recording, flicker was applied for another period of 60 s. For flicker stimulation, the white light spectrum of a halogen lamp was filtered using a 550 nm low pass cut-off-filter, ensuring that the yellow and red spectral part are filtered and only wavelengths below 550 nm were used for stimulation. Flicker was applied at a frequency of 12.5 Hz.
A previously described system allows quantification of retinal venous blood flow in vivo (13, 28, 29, 36–38). This custom-built dual-beam Doppler Fourier Domain-OCT (DOCT), coupled to a fundus camera, which ensures simultaneous view for selection of the region of interest, used a specific rectangular scanning pattern around the optic nerve head (ONH) to gain measurements from retinal veins with a diameter of at least 40 μm. To obtain averaged blood velocity values, this scanning pattern was applied on each vessel for several pulse periods. For quantification of mean absolute blood velocity (Vabs), phase shifts in the two probe beams and channels, respectively, were calculated. Venous blood flow (Qabs) was calculated using vessel diameters (d), which were extracted from the DVA and Vabs as Qabs = Vabsd2 π/4 for each vessel. DVA and DOCT measurements were performed simultaneously at the same vessel location. The detailed description of the setup has been published previously (28).
Measurement of HRV is a standardized, non-invasive method for quantification of autonomic sympathetic and parasympathetic control and therefore used for evaluation of cardiovascular autonomic neuropathy in diabetes (39–41). This assessment is based on analysis of HRV in frequency-domain, using a short-term modified orthostatic load (42). Using VariaCardio® (Vario Cardio TF5; Advanced Medical Diagnostic Group, Leeds, UK), this short-term spectral analysis of HRV is obtained from recordings consisting of 256 s of artifact-free records in three positions (supine1-standing-supine2). The recorded high-resolution one-channel echocardiogram (ECG) is transferred to a receiver connected to a personal computer and displayed on-line. Then R–R intervals are identified with a sampling rate of 1,000 Hz. Artifacts are identified and labeled and a specific algorithm inserts beat-to-beat intervals throughout an artifact period to preserve the timing relationships of the adjacent, uncorrupted heart rate data. The final results are immediately displayed on the monitor as three-dimensional running spectra. Parameters of frequency-domain HRV are measured within the low-frequency (LF) band (0.05–0.15 Hz) and high-frequency (HF) band (0.15–0.50 Hz). A reduced LF and HF spectral power at rest, a reduced increase of normalized LF spectral power during orthostasis and a reduced normalization after an orthostatic challenge are known to be a sensitive sign of a limited autonomic cardiovascular control (42, 43).
Statistical analysis was performed using IBM SPSS Statistics (Version 26, IBM, Armonk, NY, USA). All values are presented as means ± SD. Normal distribution for all outcome variables was confirmed using the Shapiro–Wilk test. Extreme outliers [according to the 3 (IQR) rule], were removed, these were two data points for the flicker response in arterial diameter in the “no DR” group. Descriptive statistics are reported for all values obtained. Flicker response was calculated as percent change from baseline in vessel diameter and retinal blood flow. A Welch ANOVA was carried out for all parameters to assess overall differences between groups. Planned contrasts between groups were used to assess differences between two groups (in particular between healthy subjects, patients with no DR, patients with mild DR, and patients with moderate to severe DR). To assess differences in HRV during the orthostatic maneuver, a repeated measures ANOVA model was calculated. A p-value < 0.05 was considered as the level of significance.
A total of 76 subjects were included in the present study, of which 56 had diabetes with no DR or different stages of non-proliferative DR. Among these, twenty-nine (29) had no DR, 12 had mild DR, and 15 had moderate to severe DR. In addition, 20 ages matched healthy subjects were included as controls. The demographics and baseline characteristics of the four study groups are shown in Table 1.
A significantly different response to flicker stimulation was observed between the four groups in regards to retinal venous blood flow (Figure 1). While in healthy subjects, retinal venous blood flow increased by 40.4 ± 27.2% and in patients with no DR by 37.7 ± 26.0%, it only increased by 26.2 ± 28.2% in patients with mild DR, and by 22.3 ± 13.9% in patients with moderate to severe DR (p = 0.035).
Figure 1. Flicker response of retinal blood flow in healthy subjects, patients with no diabetic retinopathy (no DR), mild diabetic retinopathy (mild DR), and moderate to severe diabetic retinopathy (moderate to severe DR). Data are presented as mean ± SD. Planned comparisons were used to calculate p-values between groups.
The hyperemic response in retinal arterial diameter was 2.5 ± 2.4% in healthy subjects, 1.7 ± 1.6% in patients with no DR, 1.2 ± 2.3% in patients with mild DR, and 1.0 ± 1.3% in patients with moderate to severe DR, respectively. Although there was a tendency toward a decreased response in arterial diameters with increasing stage of DR, this effect failed to reach significance (p = 0.109, Figure 2A). Planned comparisons revealed that there was a significant difference in arterial diameter response between healthy subjects and patients with moderate to severe DR.
Figure 2. Flicker response of retinal arterial (A) and venous (B) diameter in healthy subjects, patients with no diabetic retinopathy (no DR), mild diabetic retinopathy (mild DR), and moderate to severe diabetic retinopathy (moderate to severe DR). Data are presented as mean ± SD. Planned comparisons were used to calculate p-values between groups.
As for retinal veins, the response of venous diameters to flicker light was significantly different between the four groups (p < 0.001, Welch ANOVA, Figure 2B). Flicker induced vasodilation was 4.1 ± 1.5% in healthy subjects, 3.5 ± 2.2% in patients with no DR, 2.8 ± 2.1% in patients with mild DR, and lowest in patients with moderate to severe DR (1.4 ± 0.9%). When comparing individual groups, the response was significantly different between patients with moderate to severe DR and healthy subjects or patients with no DR.
When looking at HRV, normalized LF spectral power showed a significantly different response to the orthostatic maneuver in diabetic patients compared to healthy controls (p < 0.001). As shown in Figure 3A, the response was more blunted with increasing severity of DR. Data showed a significant difference between healthy subjects and patients with no DR (p = 0.004), healthy subjects and patients with mild DR (p < 0.001) and healthy subjects and moderate to severe DR (p < 0.001).
Figure 3. Normalized low-frequency (LF) spectral power of heart rate variability (HRV) in normalized units (nu) (A) and high-frequency (HF) spectral power (B) in healthy controls (open circles), patients with no diabetic retinopathy (no DR) (black squares), patients with mild diabetic retinopathy (mild DR) (upward triangles), and patients with moderate to severe diabetic retinopathy (moderate to severe DR) (downward triangles) before, during and after the orthostatic challenge. Data are presented as mean ± SD.
The time course of HF spectral power during the orthostatic maneuver was not altered in diabetic patients compared to healthy controls (p = 0.270, Figure 3B). However, HF spectral power was generally lower at all assessments in patients with diabetes compared to healthy subjects. No correlation between the flicker response in retinal blood flow, retinal arterial diameter, retinal venous diameter, and the HRV parameters normalized LF and HF spectral power was found (p > 0.30 each).
The results of the present study indicate that the blood flow response to visual stimulation with flicker light is reduced with increasing severity of DR in patients with type II diabetes. This effect was paralleled by an altered HRV in patients with diabetes, again associated with disease severity. In summary, our data support the concept of a strong neuro-vascular component in the pathogenesis of DR in patients with type II diabetes.
Several lines of evidence indicate that in the brain, as well as in the retina, a breakdown of neuro-vascular coupling may be a pathogenic factor in the development of diabetic complications. Studies in the brain using blood oxygenation level–dependent functional magnetic resonance imaging (BOLD fMRI) to assess functional hyperemia have shown reduced functional hyperemia in patients with diabetes (44, 45). As for the retina, there is compelling evidence that flicker induced vasodilatation is reduced in patients with type I and type II diabetes (20, 23, 46–48). However, the latter studies do not give a full picture of functional hyperemia in human diabetic subjects: First, previous studies report only data regarding flicker induced retinal vasodilatation in large retinal vessels, but not blood flow per se. Given that the microcirculation is the major determinant of vascular resistance and therefore blood flow, caliber changes in large vessel do not necessarily fully predict changes in blood flow. Secondly, studies assessing neuro-vascular coupling in different clinical stages of DR are sparse. Mandecka et al. (46) have reported reduced retinal vasodilatation, an effect that was more pronounced in more severe patients. As the latter study has included patients with both type I and type II diabetes, it remains unclear whether type I and type II diabetics share the same characteristics in neuro-vascular coupling.
Our data expands our knowledge in patients with type II diabetes and indicates that flicker-induced hyperemia is reduced in patients with moderate to severe DR compared to healthy subjects. Indeed, the results show that in patients with moderate to severe non-proliferative DR, blood flow response is approximately only 50% of the response observed in the healthy control group. Further, the reduction in flicker induced hyperemia was also significantly more pronounced in patients with moderate to severe DR compared to patients with no or mild DR indicating that neuro-vascular coupling is reduced depending on the severity of DR. Thus, our data supports the hypothesis of a break-down in neuro-vascular coupling early in the disease processes, which may aggravate with the progression of the disease. They also extend our previous findings in patients with type I diabetes, showing that the blood flow response to flicker stimulation is significantly reduced (22). However, since in this previous study only patients with no DR were included, no conclusion can be made on a potential difference between different grades of severity.
The reason for the diminished hyperemic response in patients with type II diabetes is still not fully elucidated. It has been suggested that the uncoupling between neural function and blood flow in diabetes may be related to endothelial dysfunction (23, 46). Following this hypothesis, the impaired hyperemic response is related to an impaired dilatory response of the microvasculature. Indeed, an impaired endothelial-dependent vasodilatation has been demonstrated in other vascular beds such as the brachial artery (49). Further, it has been reported that conditions associated with endothelial dysfunction such as smoking or systemic hypertension are also characterized by diminished flicker induced vasodilatation (47, 50). On the other hand, it has been shown that the vasodilatory response of retinal vessels to exogenous nitric oxide is preserved in patients with diabetes, indicating that the impaired flicker response is not caused by a generally reduced retinal vascular reactivity of retinal vessels (51). Thus, the question whether reduced flicker response can mainly be attributed to endothelial dysfunction is not entirely clear.
Alternatively, it has been hypothesized that impaired neural activity may be responsible for the observed uncoupling of visual stimulation and blood flow (52). Along this line of thought it has consistently been demonstrated that both the retinal nerve fiber layer as well as the ganglion cell layer are considerably thinner in patients with diabetes and no DR as compared to healthy controls (53, 54). This observed retinal thinning is associated with HbA1c, duration of diabetes and the severity of DR, indicating that neural degeneration plays an important role in the pathogenesis of the disease (55, 56). Whether these neurodegenerative changes observed in patients with diabetes occur prior to vascular manifestations or develop independently from them is yet to be investigated (52). Finally, longitudinal trials are warranted to clarify whether neural dysfunction plays a causative role in the observed breakdown of neuro-vascular coupling.
Impairment of neural function is also reflected in the data of the current study. In particular, differences between the four groups were also found for HRV, a marker for the autonomic nervous system (31, 32). While HF spectral power reflects vagal activity, the LF component is interpreted as a marker for sympathetic modulation (31). LF spectral power showed a statistically significant different response to the orthostatic maneuver in all patients with diabetes compared to healthy subjects and the difference became more pronounced with increasing disease severity. This all points toward dysfunction of the autonomic system in patients with type II diabetes. Along this line of thought, several studies report disturbed function of the autonomic nervous system as a risk factor for cardiovascular disease (57, 58), which also has a higher prevalence in diabetic patients (59, 60). Interestingly, there also seems to be a link between the autonomic nervous system and the vascular endothelium in that sense that both act in opposition in order to regulate vascular tone (61). However, as the retinal blood supply is not under direct autonomic nerve control, a potential effect of the observed dysfunction of the autonomic system in patients with type II diabetes on ocular blood flow needs to be further investigated.
Some strengths and limitations of the present study need to be addressed. The strength of the current study is that by using the Doppler OCT technique, retinal vessel diameter and blood flow could be measured concomitantly, which allows for the exact determination of volumetric blood flow. We have recently shown that this system provides excellent repeatability and reproducibility in detecting even subtle changes in retinal blood flow (29). However, as a disadvantage of the used approach, the measurements during visual stimulation are challenging for the subjects and require excellent target fixation. Thus, blood flow measurement data have only been obtained from one vessel and no information on total retinal blood flow is available from the current study. In this context, it needs to be noted that there is still no general agreement in the literature on blood flow changes in patients with diabetes. Whereas some studies found decreased retinal perfusion in patients with diabetes (11, 12, 62, 63), others have reported an increase, especially in early stages (13–15, 64). Thus, further studies are needed to clarify this issue. Further, it also needs to be mentioned that some of the patients were under systemic antihypertensive medication and we cannot fully exclude that this may have influenced ocular perfusion parameters. However, as the outcome of the study was flicker induced hyperemia not absolute blood flow and systemic hemodynamic procedures were comparable between the study days, we deem that this does not interfere with our conclusions.
An additional limitation of the current study is the cross-sectional design, which allows only for conclusions regarding one certain time point, but does not finally clarify whether impaired neuro-vascular coupling is causative for the development of retinal vascular complications of diabetes type II. Longitudinal studies are required to further investigate this issue.
In conclusion, the results of the present study show that retinal neurovascular coupling and HRV are both impaired in patients with type II diabetes. These impairments seem to be more pronounced in later stages of DR. Further studies are required to assess whether the break-down of flicker induced hyperemia may be used as a predictive marker for the individual risk assessment of DR.
The raw data supporting the conclusions of this article will be made available by the authors, without undue reservation.
The studies involving human participants were reviewed and approved by the Ethics Committee of the Medical University of Vienna. The patients/participants provided their written informed consent to participate in this study.
NH, KH, DS, LS, and GG: conceptualization. NH, RW, LS, and GG: methodology. RW: software. NH, DS, and GG: validation, formal analysis, and visualization. NH, MK, AS, PJ, and GG: investigation. KH and GG: resources. DS and GG: data curation. NH and GG: writing – original draft preparation and project administration. MK, AS, PJ, RW, KH, DS, and LS: writing – review and editing. RW and GG: supervision. GG: funding acquisition. All authors contributed to the article and approved the submitted version.
Financial support from the Austrian Science Foundation FWF Projects Grant numbers: KLI529 and KLI721 are gratefully acknowledged.
The authors declare that the research was conducted in the absence of any commercial or financial relationships that could be construed as a potential conflict of interest.
All claims expressed in this article are solely those of the authors and do not necessarily represent those of their affiliated organizations, or those of the publisher, the editors and the reviewers. Any product that may be evaluated in this article, or claim that may be made by its manufacturer, is not guaranteed or endorsed by the publisher.
1. Andreadi A, Bellia A, Di Daniele N, Meloni M, Lauro R, Della-Morte D, et al. The molecular link between oxidative stress, insulin resistance, and type 2 diabetes: a target for new therapies against cardiovascular diseases. Curr Opin Pharmacol. (2021) 62:85–96. doi: 10.1016/j.coph.2021.11.010
2. Liggi S, Griffin JL. Metabolomics applied to diabetes-lessons from human population studies. Int J Biochem Cell Biol. (2017) 93:136–47. doi: 10.1016/j.biocel.2017.10.011
3. Ali MK, Pearson-Stuttard J, Selvin E, Gregg EW. Interpreting global trends in type 2 diabetes complications and mortality. Diabetologia. (2022) 65:3–13. doi: 10.1007/s00125-021-05585-2
4. Cole JB, Florez JC. Genetics of diabetes mellitus and diabetes complications. Nat Rev Nephrol. (2020) 16:377–90. doi: 10.1038/s41581-020-0278-5
5. Marcovecchio ML. Importance of identifying novel biomarkers of microvascular damage in type 1 diabetes. Mol Diagn Ther. (2020) 24:507–15. doi: 10.1007/s40291-020-00483-6
6. Veiby N, Simeunovic A, Heier M, Brunborg C, Saddique N, Moe MC, et al. Associations between macular oct angiography and nonproliferative diabetic retinopathy in young patients with type 1 diabetes mellitus. J Diabetes Res. (2020) 2020:8849116. doi: 10.1155/2020/8849116
7. Koçer AM, Şekeroğlu MA. Evaluation of the neuronal and microvascular components of the macula in patients with diabetic retinopathy. Doc Ophthalmol. (2021) 143:193–205. doi: 10.1007/s10633-021-09834-y
8. Frizziero L, Parrozzani R, Londei D, Pilotto E, Midena E. Quantification of vascular and neuronal changes in the peripapillary retinal area secondary to diabetic retinopathy. Br J Ophthalmol. (2021) 105:1577–83. doi: 10.1136/bjophthalmol-2020-316468
9. Tan B, Chua J, Lin E, Cheng J, Gan A, Yao X, et al. Quantitative microvascular analysis with wide-field optical coherence tomography angiography in eyes with diabetic retinopathy. JAMA Netw Open. (2020) 3:e1919469. doi: 10.1001/jamanetworkopen.2019.19469
10. Tey KY, Teo K, Tan ACS, Devarajan K, Tan B, Tan J, et al. Optical coherence tomography angiography in diabetic retinopathy: a review of current applications. Eye Vis. (2019) 6:37. doi: 10.1186/s40662-019-0160-3
11. Pechauer AD, Hwang TS, Hagag AM, Liu L, Tan O, Zhang X, et al. Assessing total retinal blood flow in diabetic retinopathy using multiplane en face doppler optical coherence tomography. Br J Ophthalmol. (2018) 102:126–30. doi: 10.1136/bjophthalmol-2016-310042
12. Srinivas S, Tan O, Nittala MG, Wu JL, Fawzi AA, Huang D, et al. Assessment of retinal blood flow in diabetic retinopathy using doppler fourier-domain optical coherence tomography. Retina. (2017) 37:2001–7. doi: 10.1097/iae.0000000000001479
13. Fondi K, Wozniak PA, Howorka K, Bata AM, Aschinger GC, Popa-Cherecheanu A, et al. Retinal oxygen extraction in individuals with type 1 diabetes with no or mild diabetic retinopathy. Diabetologia. (2017) 60:1534–40. doi: 10.1007/s00125-017-4309-0
14. Patel V, Rassam S, Newsom R, Wiek J, Kohner E. Retinal blood flow in diabetic retinopathy. BMJ. (1992) 305:678–83. doi: 10.1136/bmj.305.6855.678
15. Grunwald JE, DuPont J, Riva CE. Retinal haemodynamics in patients with early diabetes mellitus. Br J Ophthalmol. (1996) 80:327–31. doi: 10.1136/bjo.80.4.327
16. Sander CY, Hooker JM, Catana C, Normandin MD, Alpert NM, Knudsen GM, et al. Neurovascular coupling to D2/D3 dopamine receptor occupancy using simultaneous pet/functional mri. Proc Natl Acad Sci U.S.A. (2013) 110:11169–74. doi: 10.1073/pnas.1220512110
17. Tournier N, Comtat C, Lebon V, Gennisson JL. Challenges and perspectives of the hybridization of pet with functional mri or ultrasound for neuroimaging. Neuroscience. (2021) 474:80–93. doi: 10.1016/j.neuroscience.2020.10.015
18. Abraham T, Feng J. Evolution of brain imaging instrumentation. Semin Nucl Med. (2011) 41:202–19. doi: 10.1053/j.semnuclmed.2010.12.001
19. Lott ME, Slocomb JE, Shivkumar V, Smith B, Gabbay RA, Quillen D, et al. Comparison of retinal vasodilator and constrictor responses in type 2 diabetes. Acta Ophthalmol. (2012) 90:e434–41. doi: 10.1111/j.1755-3768.2012.02445.x
20. Garhöfer G, Zawinka C, Resch H, Kothy P, Schmetterer L, Dorner GT. Reduced response of retinal vessel diameters to flicker stimulation in patients with diabetes. Br J Ophthalmol. (2004) 88:887–91. doi: 10.1136/bjo.2003.033548
21. Lott ME, Slocomb JE, Shivkumar V, Smith B, Quillen D, Gabbay RA, et al. Impaired retinal vasodilator responses in prediabetes and type 2 diabetes. Acta Ophthalmol. (2013) 91:e462–9. doi: 10.1111/aos.12129
22. Lasta M, Pemp B, Schmidl D, Boltz A, Kaya S, Palkovits S, et al. Neurovascular dysfunction precedes neural dysfunction in the retina of patients with type 1 diabetes. Invest Ophthalmol Vis Sci. (2013) 54:842–7. doi: 10.1167/iovs.12-10873
23. Nguyen TT, Kawasaki R, Wang JJ, Kreis AJ, Shaw J, Vilser W, et al. Flicker light-induced retinal vasodilation in diabetes and diabetic retinopathy. Diabetes Care. (2009) 32:2075–80. doi: 10.2337/dc09-0075
24. Garhöfer G, Chua J, Tan B, Wong D, Schmidl D, Schmetterer L. Retinal neurovascular coupling in diabetes. J Clin Med. (2020) 9:2829. doi: 10.3390/jcm9092829
25. Streese L, Kotliar K, Deiseroth A, Infanger D, Vilser W, Hanssen H. Retinal endothelial function, physical fitness and cardiovascular risk: a diagnostic challenge. Front Physiol. (2019) 10:831. doi: 10.3389/fphys.2019.00831
26. Sörensen BM, Houben AJ, Berendschot TT, Schouten JS, Kroon AA, van der Kallen CJ, et al. Prediabetes and type 2 diabetes are associated with generalized microvascular dysfunction: the maastricht study. Circulation. (2016) 134:1339–52. doi: 10.1161/circulationaha.116.023446
27. Baier JM, Funck KL, Petersen L, Vernstrøm L, Knudsen ST, Bek T, et al. Retinal vessel reactivity is not attenuated in patients with type 2 diabetes compared with matched controls and is associated with peripheral endothelial function in controls. J Diabetes Complications. (2019) 33:641–7. doi: 10.1016/j.jdiacomp.2019.05.024
28. Doblhoff-Dier V, Schmetterer L, Vilser W, Garhöfer G, Gröschl M, Leitgeb RA, et al. Measurement of the total retinal blood flow using dual beam fourier-domain doppler optical coherence tomography with orthogonal detection planes. Biomed Opt Express. (2014) 5:630–42. doi: 10.1364/boe.5.000630
29. Szegedi S, Hommer N, Kallab M, Puchner S, Schmidl D, Werkmeister RM, et al. Repeatability and reproducibility of total retinal blood flow measurements using bi-directional doppler Oct. Transl Vis Sci Technol. (2020) 9:34. doi: 10.1167/tvst.9.7.34
30. McCraty R, Shaffer F.. Heart rate variability: new perspectives on physiological mechanisms, assessment of self-regulatory capacity, and health risk. Glob Adv Health Med. (2015) 4:46–61. doi: 10.7453/gahmj.2014.073
31. Horsten M, Ericson M, Perski A, Wamala SP, Schenck-Gustafsson K, Orth-Gomér K. Psychosocial factors and heart rate variability in healthy women. Psychosom Med. (1999) 61:49–57. doi: 10.1097/00006842-199901000-00009
32. Hjortskov N, Rissén D, Blangsted AK, Fallentin N, Lundberg U, Søgaard K. The effect of mental stress on heart rate variability and blood pressure during computer work. Eur J Appl Physiol. (2004) 92:84–9. doi: 10.1007/s00421-004-1055-z
33. Wilkinson CP, Ferris FL III, Klein RE, Lee PP, Agardh CD, Davis M, et al. Proposed international clinical diabetic retinopathy and diabetic macular edema disease severity scales. Ophthalmology. (2003) 110:1677–82. doi: 10.1016/s0161-6420(03)00475-5
34. Blum M, Bachmann K, Wintzer D, Riemer T, Vilser W, Strobel J. Noninvasive measurement of the bayliss effect in retinal autoregulation. Graefes Arch Clin Exp Ophthalmol. (1999) 237:296–300. doi: 10.1007/s004170050236
35. Garhofer G, Bek T, Boehm AG, Gherghel D, Grunwald J, Jeppesen P, et al. Use of the retinal vessel analyzer in ocular blood flow research. Acta Ophthalmol. (2010) 88:717–22. doi: 10.1111/j.1755-3768.2009.01587.x
36. Werkmeister RM, Dragostinoff N, Palkovits S, Told R, Boltz A, Leitgeb RA, et al. Measurement of absolute blood flow velocity and blood flow in the human retina by dual-beam bidirectional doppler fourier-domain optical coherence tomography. Invest Ophthalmol Vis Sci. (2012) 53:6062–71. doi: 10.1167/iovs.12-9514
37. Werkmeister RM, Palkovits S, Told R, Groschl M, Leitgeb RA, Garhofer G, et al. Response of retinal blood flow to systemic hyperoxia as measured with dual-beam bidirectional doppler fourier-domain optical coherence tomography. PLoS One (2012) 7:e45876. doi: 10.1371/journal.pone.0045876
38. Werkmeister RM, Schmidl D, Aschinger G, Doblhoff-Dier V, Palkovits S, Wirth M, et al. Retinal oxygen extraction in humans. Sci Rep. (2015) 5:15763. doi: 10.1038/srep15763
39. Ewing DJ, Martyn CN, Young RJ, Clarke BF. The value of cardiovascular autonomic function tests: 10 years experience in diabetes. Diabetes Care. (1985) 8:491–8. doi: 10.2337/diacare.8.5.491
40. Howorka K, Pumprla J, Schabmann A. Optimal parameters of short-term heart rate spectrogram for routine evaluation of diabetic cardiovascular autonomic neuropathy. J Auton Nerv Syst. (1998) 69:164–72. doi: 10.1016/s0165-1838(98)00015-0
41. Pumprla J, Howorka K, Groves D, Chester M, Nolan J. Functional assessment of heart rate variability: physiological basis and practical applications. Int J Cardiol. (2002) 84:1–14.
42. Howorka K, Pumprla J, Jirkovska A, Lacigova S, Nolan J. Modified orthostatic load for spectral analysis of short-term heart rate variability improves the sensitivity of autonomic dysfunction assessment. J Diabetes Complications. (2010) 24:48–54. doi: 10.1016/j.jdiacomp.2008.10.003
43. Bellavere F, Balzani I, De Masi G, Carraro M, Carenza P, Cobelli C, et al. Power spectral analysis of heart-rate variations improves assessment of diabetic cardiac autonomic neuropathy. Diabetes. (1992) 41:633–40. doi: 10.2337/diab.41.5.633
44. Hu B, Yan LF, Sun Q, Yu Y, Zhang J, Dai YJ, et al. Disturbed neurovascular coupling in type 2 diabetes mellitus patients: evidence from a comprehensive fmri analysis. Neuroimage Clin. (2019) 22:101802. doi: 10.1016/j.nicl.2019.101802
45. Duarte JV, Pereira JM, Quendera B, Raimundo M, Moreno C, Gomes L, et al. Early disrupted neurovascular coupling and changed event level hemodynamic response function in type 2 diabetes: an fmri study. J Cereb Blood Flow Metab (2015) 35:1671–80. doi: 10.1038/jcbfm.2015.106
46. Mandecka A, Dawczynski J, Blum M, Müller N, Kloos C, Wolf G, et al. Influence of flickering light on the retinal vessels in diabetic patients. Diabetes Care (2007) 30:3048–52. doi: 10.2337/dc07-0927
47. Pemp B, Weigert G, Karl K, Petzl U, Wolzt M, Schmetterer L, et al. Correlation of flicker-induced and flow-mediated vasodilatation in patients with endothelial dysfunction and healthy volunteers. Diabetes Care. (2009) 32:1536–41. doi: 10.2337/dc08-2130
48. Bek T, Hajari J, Jeppesen P. Interaction between flicker-induced vasodilatation and pressure autoregulation in early retinopathy of type 2 diabetes. Graefes Arch Clin Exp Ophthalmol. (2008) 246:763–9. doi: 10.1007/s00417-008-0766-y
49. McVeigh GE, Brennan GM, Johnston GD, McDermott BJ, McGrath LT, Henry WR, et al. Impaired endothelium-dependent and independent vasodilation in patients with type 2 (non-insulin-dependent) diabetes mellitus. Diabetologia. (1992) 35:771–6. doi: 10.1007/bf00429099
50. Garhöfer G, Resch H, Sacu S, Weigert G, Schmidl D, Lasta M, et al. Effect of regular smoking on flicker induced retinal vasodilatation in healthy subjects. Microvasc Res. (2011) 82:351–5. doi: 10.1016/j.mvr.2011.07.001
51. Pemp B, Garhofer G, Weigert G, Karl K, Resch H, Wolzt M, et al. Reduced retinal vessel response to flicker stimulation but not to exogenous nitric oxide in type 1 diabetes. Invest Ophthalmol Vis Sci. (2009) 50:4029–32. doi: 10.1167/iovs.08-3260
52. Sachdeva MM. Retinal neurodegeneration in diabetes: an emerging concept in diabetic retinopathy. Curr Diab Rep. (2021) 21:65. doi: 10.1007/s11892-021-01428-x
53. Carpineto P, Toto L, Aloia R, Ciciarelli V, Borrelli E, Vitacolonna E, et al. Neuroretinal alterations in the early stages of diabetic retinopathy in patients with type 2 diabetes mellitus. Eye. (2016) 30:673–9. doi: 10.1038/eye.2016.13
54. van Dijk HW, Verbraak FD, Kok PH, Garvin MK, Sonka M, Lee K, et al. Decreased retinal ganglion cell layer thickness in patients with type 1 diabetes. Invest Ophthalmol Vis Sci. (2010) 51:3660–5. doi: 10.1167/iovs.09-5041
55. Garcia-Martin E, Cipres M, Melchor I, Gil-Arribas L, Vilades E, Polo V, et al. Neurodegeneration in patients with type 2 diabetes mellitus without diabetic retinopathy. J Ophthalmol. (2019) 2019:1825819. doi: 10.1155/2019/1825819
56. Chatziralli I, Karamaounas A, Dimitriou E, Kazantzis D, Theodossiadis G, Kozobolis V, et al. Peripapillary retinal nerve fiber layer changes in patients with diabetes mellitus: a case-control study. Semin Ophthalmol. (2020) 35:257–60. doi: 10.1080/08820538.2020.1810289
57. Souza HCD, Philbois SV, Veiga AC, Aguilar BA. Heart rate variability and cardiovascular fitness: what we know so far. Vasc Health Risk Manag. (2021) 17:701–11. doi: 10.2147/vhrm.S279322
58. Scott-Solomon E, Boehm E, Kuruvilla R. The sympathetic nervous system in development and disease. Nat Rev Neurosci. (2021) 22:685–702. doi: 10.1038/s41583-021-00523-y
59. Nørgaard CH, Starkopf L, Gerds TA, Malmborg M, Bonde AN, Zareini B, et al. Temporal changes in risk of cardiovascular events in people with newly diagnosed type 2 diabetes with and without cardiovascular disease. J Diabetes Complications (2022) 36:108126. doi: 10.1016/j.jdiacomp.2022.108126
60. Sattar N, Rawshani A, Franzén S, Rawshani A, Svensson AM, Rosengren A, et al. Age at diagnosis of type 2 diabetes mellitus and associations with cardiovascular and mortality risks. Circulation. (2019) 139:2228–37. doi: 10.1161/circulationaha.118.037885
61. Harris KF, Matthews KA. Interactions between autonomic nervous system activity and endothelial function: a model for the development of cardiovascular disease. Psychosom Med. (2004) 66:153–64. doi: 10.1097/01.psy.0000116719.95524.e2
62. Nagaoka T, Sato E, Takahashi A, Yokota H, Sogawa K, Yoshida A. Impaired retinal circulation in patients with type 2 diabetes mellitus: retinal laser doppler velocimetry study. Invest Ophthalmol Vis Sci. (2010) 51:6729–34. doi: 10.1167/iovs.10-5364
63. Dimitrova G, Chihara E, Takahashi H, Amano H, Okazaki K. Quantitative retinal optical coherence tomography angiography in patients with diabetes without diabetic retinopathy. Invest Ophthalmol Vis Sci. (2017) 58:190–6. doi: 10.1167/iovs.16-20531
Keywords: diabetes type II, diabetic retinopathy, functional hyperemia, neuro-vascular coupling, retinal blood flow, heart rate variability
Citation: Hommer N, Kallab M, Schlatter A, Janku P, Werkmeister RM, Howorka K, Schmidl D, Schmetterer L and Garhöfer G (2022) Neuro-vascular coupling and heart rate variability in patients with type II diabetes at different stages of diabetic retinopathy. Front. Med. 9:1025853. doi: 10.3389/fmed.2022.1025853
Received: 23 August 2022; Accepted: 26 October 2022;
Published: 10 November 2022.
Edited by:
Ovidiu Constantin Baltatu, Anhembi Morumbi University, BrazilReviewed by:
Rebekka Heitmar, University of Huddersfield, United KingdomCopyright © 2022 Hommer, Kallab, Schlatter, Janku, Werkmeister, Howorka, Schmidl, Schmetterer and Garhöfer. This is an open-access article distributed under the terms of the Creative Commons Attribution License (CC BY). The use, distribution or reproduction in other forums is permitted, provided the original author(s) and the copyright owner(s) are credited and that the original publication in this journal is cited, in accordance with accepted academic practice. No use, distribution or reproduction is permitted which does not comply with these terms.
*Correspondence: Gerhard Garhöfer, Z2VyaGFyZC5nYXJob2VmZXJAbWVkdW5pd2llbi5hYy5hdA==
Disclaimer: All claims expressed in this article are solely those of the authors and do not necessarily represent those of their affiliated organizations, or those of the publisher, the editors and the reviewers. Any product that may be evaluated in this article or claim that may be made by its manufacturer is not guaranteed or endorsed by the publisher.
Research integrity at Frontiers
Learn more about the work of our research integrity team to safeguard the quality of each article we publish.