- 1Department of Intensive Methods of Therapy, V.A. Nasonova Research Institute of Rheumatology, Moscow, Russia
- 2Laboratory of Molecular Hematology, National Medical Research Center for Hematology, Moscow, Russia
- 3Hematopoiesis Immunology Laboratory, Russian Cancer Research Center N.N. Blokhin, Moscow, Russia
A persistently increased T-cell large granular lymphocyte (T-LGL) count in the blood of more than 2 × 109/L for at least 6 months is necessary for a reliable diagnosis of T-LGL leukemia. In cases with LGL counts of approximately 0.5–2 × 109/L, a diagnosis of T-LGL leukemia can be made if clonal rearrangement of T-cell receptor (TCR) genes is present and if the patient shows typical manifestations of T-LGL leukemia, such as cytopenia, splenomegaly, or concomitant autoimmune disease. However, in cases with LGL counts of less than 0.5 × 109/L, the diagnosis of T-LGL leukemia is questionable (termed as “gray-zone” cases). Although mutations in signal transducer and activator of transcription 3 (STAT3) gene are the molecular hallmark of T-LGL leukemia, their diagnostic value in the “gray-zone” cases of T-LGL leukemia has not been evaluated – our study has been aimed to examine the prevalence of STAT3 mutations in these cases. Herein, we describe 25 patients with autoimmune rheumatic diseases, neutropenia, clonal rearrangement of TCR genes, and circulating LGL count of less than 0.5 × 109/L. Splenomegaly was observed in 19 (76%) patients. Mutations in the STAT3 were detected in 56% of patients using next-generation sequencing. Importantly, in 3 patients, no involvement of the blood and bone marrow by malignant LGLs was noted, but examination of splenic tissue revealed infiltration by clonal cytotoxic T-lymphocytes within the red pulp, with greater prominence in the cords. We suggest using the term “splenic variant of T-LGL leukemia” for such cases.
Introduction
Large granular lymphocytic (LGL) leukemia is characterized by the expansion of lymphocytes with abundant cytoplasm containing variably sized azurophilic granules and a reniform or round nucleus with mature chromatin (1). Approximately 85% of LGL leukemia patients exhibit T-cells with a mature post-thymic phenotype (1). T-cell LGL (T-LGL) leukemia has an indolent clinical course and involves the peripheral blood, bone marrow, and spleen (2). A typical manifestation of T-LGL leukemia includes neutropenia, splenomegaly, and LGL lymphocytosis, which are detected in up to 84%, 50%, and 52% of patients, respectively (3). Moreover, up to 40% of patients with T-LGL leukemia have concomitant autoimmune disorders, of which rheumatoid arthritis (RA) is the most common (1, 4, 5).
Historically, a definitive diagnosis of LGL leukemia is made if the LGL count in the peripheral blood is more than 2 × 109/L (normal range, < 0.3 × 109/L) over a period of 6 months, with no clearly identified cause (3, 6). Although this definition of T-LGL leukemia remained unchanged in the 2016 revision of the World Health Organization (WHO) classification of tumors of hematopoietic and lymphoid tissues, cases wherein the LGL count is less than 2 × 109/L may be suggestive of this diagnosis (2). While a lower threshold of the absolute LGL count in the peripheral blood for T-LGL leukemia has not been defined by the WHO, the threshold of 0.5 × 109/L is now generally accepted (5, 7). In cases with LGL counts of approximately 0.5–2 × 109/L, a diagnosis of T-LGL leukemia can be made if the LGLs are clonal and if the patient shows typical manifestations of T-LGL leukemia, such as cytopenia, splenomegaly, or concomitant autoimmune disorder (5, 7–10). Infiltration of bone marrow by clonal T-LGLs and/or characteristic histopathological findings of LGL leukemia in the bone marrow with typical manifestations of T-LGL leukemia also confirm the diagnosis of T-LGL leukemia (8, 11, 12). However, cases with circulating LGL counts of less than 0.4–0.5 × 109/L (termed as “gray-zone” cases) present a specific diagnostic challenge because of the difficulty in making a differential diagnosis with reactive T-LGL proliferation.
Although T-cell clonality is necessary to distinguish T-LGL leukemia from reactive T-LGL proliferation, clonality does not equate to malignancy and is not enough to classify T-LGL proliferation as leukemia (13, 14).
Constitutive activation of the signal transducer and activator of transcription 3 (STAT3) signaling pathway is a molecular hallmark of T-LGL leukemia (5). Activating point mutations in the STAT3 gene are found in up to 72% of T-LGL leukemia patients (15–18). Thus, detection of STAT3 mutations may serve as a valid reason for classifying the condition in diagnostically challenging cases as T-cell LGL leukemia (15). However, their prevalence in “gray-zone” cases has not been evaluated.
In the present study, STAT3 gene mutations were evaluated using next-generation sequencing (NGS) in a cohort of 25 patients with autoimmune rheumatic diseases (ARDs), clinical presentation typical for T-LGL leukemia, clonal rearrangement of the T-cell receptor (TCR) gene, and absolute circulating LGL count of less than 0.5 × 109/L.
Patients and methods
The inclusion criteria were as follows: patients aged > 18 years with diagnosed ARD and absolute neutrophil counts of < 1.5 × 109/L. From 2008 to 2021, 106 patients admitted to V.A. Nasonova Research Institute of Rheumatology met the inclusion criteria and were included in the primary analysis. A flowchart of the patient selection process is shown in Figure 1.
Peripheral blood smears were available for 83 patients. Manual differential tests were performed using Wright-Giemsa-stained peripheral blood smears to quantify the LGL percentage and calculate the absolute LGL counts. The circulating LGL count was ≤ 0.5 × 109/L in 39 patients. In 25 of the 39 patients, T-cell clonality testing revealed clonal populations. These 25 patients were included in the final analysis.
The clinical data of patients were collected, including age of onset of neutropenia or splenomegaly, sex, rheumatologic diagnosis, and bone marrow differential counts. Splenic tissue specimens (from 5 patients) and bone marrow trephine biopsies (from 15 patients) had previously been fixed in 10% formalin, routinely processed, embedded in paraffin, and stained with hematoxylin and eosin.
Screening for STAT3 mutations was performed on samples in which a monoclonal TCR gene rearrangement pattern was detectable. For samples with a polyclonal TCR gene rearrangement pattern, STAT3 mutation screening was conducted if a monoclonal TCR gene rearrangement pattern was detected in other tissue samples from the same patient. For example, if a monoclonal TCR gene rearrangement was detected in the bone marrow but was absent in the blood sample, screening for STAT3 mutations was performed in both the bone marrow and blood samples.
Ethics statement
This study was approved by the Ethics Committee of the V.A. Nasonova Research Institute of Rheumatology (protocol #10 on the 20-01-2022). All patients gave written consent for collection, analysis of specimens and for publication of their data as results of the study.
Evaluation of T-cell clonality
T-cell clonality was examined using genomic DNA extracted from blood (25 patients), bone marrow (16 patients), and spleen tissue (5 patients) samples. T-cell clonality was evaluated based on the rearrangements of TCR-γ (Vγ–Jγ) and TCR-β (Vβ–Jβ, Dβ–Jβ) in all patients. T-cell clonality assays were performed according to the BIOMED-2 standardized protocol (19). Polymerase chain reaction (PCR) amplification was conducted using an automated DNA Engine Thermal Cycler (Bio-Rad, Hercules, United States), and fragments were detected using an ABI PRISM 3130 Genetic Analyzer (Applied Biosystems, Foster City, CA, United States). The data were analyzed using GeneMapper software version 4.0 (Applied Biosystems).
Detection of a weak clonal signal in the context of polyclonal signals was interpreted as ambiguous clonality. False positive results (pseudo-monoclonality) were excluded by identical dominant PCR products in repeated PCR analyses of the same sample and/or identical dominant PCR products in different test samples (blood, bone marrow, or spleen samples) from the same patient.
Immunophenotypic analysis
Immunohistochemical studies of the spleen (5 patients) and bone marrow (15 patients) were conducted using the formalin-fixed paraffin-embedded tissue. The following antibodies were used at dilutions suggested by the manufacturers: CD3 (polyclonal, Dako, Carpinteria, CA, United States); CD4 (clone 4B12, Dako); CD8 (clone C8/144B, Dako); CD16 (clone 2H7, Novocastra Laboratories, Newcastle upon Tyne, United Kingdom); CD20 (clone L26, Dako); granzyme B (clone GrB-7, Dako); T-cell restricted intracellular antigen 1 (TIA-1) (clone 2G9, Immunotech, France); TCR-β F1 (clone 8A3, Thermo Scientific, Waltham, MA, United States); and TCR-γ (clone γ3.20, Thermo Scientific). After dewaxing and heat-induced antigen retrieval, immunostaining was performed using an Autostainer Link 48 (Dako, Denmark) according to the manufacturer’s instructions. All immunostained samples were counter-stained with hematoxylin.
Evaluation of STAT3 mutations
STAT3 mutations were examined using genomic DNA extracted from specimens of peripheral blood (24 patients), bone marrow (15 patients), and the spleen (2 patients).
Mutations in exons 19–21 of the STAT3 gene were identified by NGS. Appropriate DNA regions were amplified using primers for exons 19–20 (product length 502 bp) and exon 21 (product length 522 bp), as described previously (15).
Amplified DNA fragments were converted to sequencing libraries using Nextera XT DNA Library Prep and Nextera XT Index Kit v2 (Illumina, United States) according to the manufacturer’s instructions. Nucleotide sequences were analyzed on a MiSeq sequencer (Illumina) using MiSeq Reagents Kit v2 for 300 cycles (Illumina). Raw data filtering, removal of accessory sequences, mapping of reads, and searching for variants were performed using the SAMtools (20), BWA (21), Trimmomatic (22), and VarDict (23). Usually, 2,000–5,000 reads were obtained for each target, and the cutoff for reporting variants was set to 0.5%. Discovered variants were annotated with ANNOVAR (24) utility using snp138 (25), refGene (26), ClinVar (27), and COSMIC (28) open databases. In two samples, STAT3 mutations were assessed by real-time allele-specific PCR assay for the most common substitutions: Y640F, D661Y, N647I, D661V, D661H, and D661N.
Statistical analysis
Descriptive statistics are presented as numbers and percentages for categorical data and as medians and ranges for continuous data. A two-sample test for equality of proportions with continuity correction was used for statistical analysis. A p-value of < 0.05 was considered statistically significant.
Results
The clinical, laboratory, and pathologic characteristics of the 25 patients included in the final analysis are shown in Table 1. According to the inclusion criteria, all patients had ARD, neutropenia counts of ≤ 1.5 × 109/L (median 0.240 × 109/L, range, 0.016–1.428 × 109/L), LGL counts of ≤ 0.5 × 109/L in the peripheral blood (median 0.381 × 109/L, range, 0.028–0.499 × 109/L), and a clonal T-cell population.
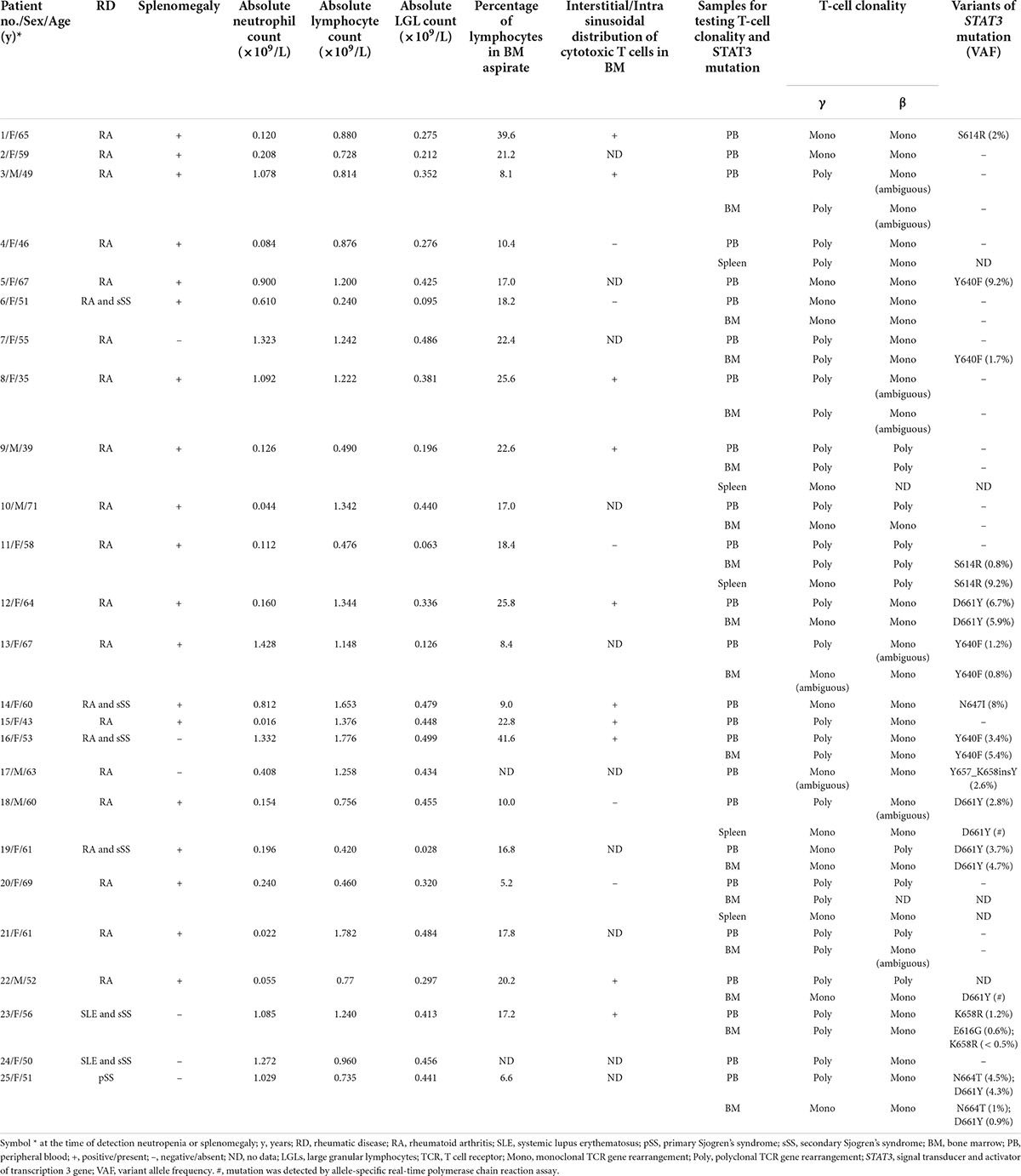
Table 1. Characteristics of 25 patients with rheumatic diseases, clonal T-LGL proliferations, and LGL counts of ≤ 0.5 × 109/L in blood.
Overall, 22 patients had RA, 2 had systemic lupus erythematosus (SLE), and 1 had primary Sjogren’s syndrome (SS). Six patients had SS in combination with other rheumatic diseases (secondary SS): 4 with RA and 2 with SLE.
The median age of the patients at the time of neutropenia detection and/or splenomegaly was 58 years (range, 35–71 years). The female-to-male ratio was 3.2:1. Splenomegaly was observed in 19 (76%) patients, among whom 5 underwent splenectomy for diagnostic or therapeutic purposes. The absolute lymphocyte counts in the blood ranged from 0.24 to 1.782 × 109/L, with a median of 0.96 × 109/L.
Bone marrow aspirate differential counts were available for 23 patients. In all cases, there were no signs of myelodysplasia. The proportion of lymphocytes in the bone marrow was between 5.2% and 41.6% (median 17.8%) of all nucleated cells, but only 4 (17%) patients had bone marrow lymphocytosis, defined as exceeding the upper limit of lymphocytes of > 23.8% of nucleated bone marrow cells. Interstitial/intrasinusoidal distribution of cytotoxic T-cells in the bone marrow was detected in 10 (67%) of the 15 patients examined.
Examination of splenic tissue revealed lymphoid infiltration of both splenic cords and sinusoids within the red pulp, with greater prominence in the cords. The white pulp was preserved and contained prominent germinal centers in 3 patients and was atrophied in 2 patients. Immunohistochemical staining revealed that the lymphocytes infiltrating the red pulp were CD3+/TIA1+ in all patients and CD16+ in 4 patients. Beta-F1 and TCR-γ positivity was observed in 3 and 1 patient, respectively. In another patient, approximately one-third of the T-cells expressed Beta-F1, and another third of the T cells expressed TCR-γ. In addition, approximately one-third of the T-cell population did not express TCR. Lymphocytes were CD8+/CD4– in three TCRαβ+ cases and CD8–/CD4– in the remaining cases.
Clonal (or ambiguous clonal) rearrangement of TCR genes in the peripheral blood was detected in 19 of the 25 patients: TCR-γ gene in 7 (28%) of the 25 patients and TCR-β gene in 18 (72%) of the 25 patients. Out of 16 bone marrow samples examined, clonal (or ambiguous clonal) rearrangement of TCR genes was detected in 13 cases (in 3 of these patients, it was not detected in the blood): TCR-γ gene in 7 (44%) of 16 patients and TCR-β gene in 13 (87%) of 15 patients (in one case this study was not performed). Clonal TCR-β gene rearrangements were detected more often than TCR-γ gene rearrangements both in the peripheral blood (72 vs. 28%, p = 0.004678) and bone marrow samples (87 vs. 44%, p = 0.03399). Clonal rearrangements of TCR genes in the spleen samples were detected in all 5 patients examined: TCR-γ gene in 4 of 5 patients and TCR-β gene in 3 of 4 patients (in one case this study was not performed). Overall, a clonal (or ambiguous clonal) rearrangement of TCR genes was detected in the blood and/or bone marrow samples in 22 (88%) of the 25 patients. In the 3 remaining patients, it was found only in the spleen samples.
Point mutations in the STAT3 gene were identified in 14 (56%) of the 25 patients examined, including D661Y (5 patients), Y640F (4 patients), S614R (2 patients) and N647I, Y657_K658insY, K658R, N664T, and E616G in one patient each. Double mutation in the STAT3 gene was observed in 2 patients. STAT3 mutations were detected in 11 (46%) of 24 blood samples, 9 (60%) of 15 bone marrow samples, and in both the spleen samples (100%). In one bone marrow sample, STAT3 mutation was detected in the absence of a clonal rearrangement of the TCR genes.
Discussion
Clonal proliferation of T-LGLs has been detected in various clinical conditions, including autoimmune disorders, myeloid or lymphoid clonal hematologic malignancies, pure red cell aplasia, aplastic anemia, and paroxysmal nocturnal hemoglobinuria (29–33). In addition, small clonal proliferations of T-LGLs have been observed after allogeneic stem cell transplantation (34, 35) and solid organ transplantation (36–38) as well as in patients with HIV (39) or cytomegalovirus infection (40). It is likely that some of these cases may represent an intensive immune response to antigen exposure more than would be expected for physiological T-cell clones, rather than true T-LGL leukemia. Although the etiology and pathogenesis of T-LGL leukemia remains unclear, the triggering event underlying T-LGL leukemia is believed to be related to chronic antigenic stimulation initially leading to a poly- or oligoclonal expansion of LGLs (41, 42). The proliferation of LGLs is supported by several cytokines, including interleukin (IL)-6, IL-12, and IL-15 (43–47). The activating point mutations of STAT3 in these antigen-driven LGLs can cause aberrant STAT3 signaling and, in combination with other factors, ultimately lead to the monoclonal expansion of LGLs (42, 48).
Monoclonal expansions of CD8+ T-lymphocytes have also been detected in healthy individuals (49–52). Bigouret et al. suggested that T-LGL clones exist in a significant number of healthy individuals and clinical symptoms, such as neutropenia and autoimmune diseases, can occur when T-LGL clone encounters an antigen and begins producing large amounts of effector molecules (49).
The diagnosis of T-LGL leukemia in cases with small clonal populations in blood has been debated. The term “T-cell clonopathy of undetermined significance” was coined to emphasize the indolent clinical course of T-LGL leukemia in many patients and to eliminate the stigma associated with the term “leukemia.” The term “T-cell clonopathy of undetermined significance” has been used regardless of the peripheral blood LGL count, clinical symptoms, and hematological abnormalities observed in patients (53, 54). Subsequently, the term “T-cell clone of undetermined significance” (TCUS) was proposed for patients with a small clonal population of T-LGL and no diagnostic features of T-LGL leukemia (51, 55). However, the diagnostic threshold of circulating LGLs to distinguish T-LGL leukemia from TCUS has not been defined, and the researchers proposed a threshold range from 500 cells/μL (51) to 2,000 cells/μL (55). In addition, the immunophenotypic signature of TCUS closely resembles that of T-LGL leukemia (51), and the immunohistochemical pattern of bone marrow in TCUS has not been studied yet.
Activating point mutations in STAT3 are not pathognomonic for T-LGL leukemia and can be detected in various hematological and non-hematological malignancies (56). In addition, Kim et al. recently found somatic STAT3 mutations in CD8+ T-cells of healthy blood donors carrying the human T-cell leukemia virus type 2 (57). However, in an appropriate clinical context, STAT3 mutations are a molecular marker that is highly specific for T-LGL leukemia (15–18, 56). Nevertheless, the significance of STAT3 mutations in distinguishing T-LGL leukemia from TCUS is not clear.
Similar to the MYD88L265P mutation, which alone is not an absolute diagnostic marker for Waldenstrom’s macroglobulinemia and is found in 50–80% of patients with IgM monoclonal gammopathy of uncertain significance (58), STAT3 mutations alone are unlikely to allow a reliable diagnosis of T-LGL leukemia when clonal T-LGL proliferation is found.
Monoclonal B-cell lymphocytosis (MBL) is a hematological condition characterized by the presence of a monoclonal population of B-lymphocytes with a count of less than 5 × 109/L in the peripheral blood and without other features of a B-cell lymphoproliferative disorder, such as cytopenia, organomegaly, lymphadenopathy, or extramedullary involvement (59). The genomic aberrations characteristic of chronic lymphocytic leukemia (CLL) can be found in cases of MBL with an immunophenotype similar to that observed in CLL (60). Drawing an analogy between TCUS/T-LGL leukemia and MBL/CLL, it seems logical that the distinction between T-LGL leukemia and TCUS should be based on the blood LGL count and/or the presence of signs of T-LGL leukemia, such as cytopenia and/or splenomegaly.
Although all patients in our cohort had absolute LGL counts in the peripheral blood below the currently accepted threshold of 0.5 × 109/L, the typical manifestations of T-LGL leukemia, including neutropenia and splenomegaly, allowed us to classify these cases as T-LGL leukemia rather than TCUS.
Patients with STAT3 mutations develop RA and neutropenia more often than patients without these mutations (15, 16, 61). This likely explains the higher frequency of STAT3 mutations in our cohort than in T-LGL leukemia patients in two recently reported large studies: 56% vs. 36%–40% (61, 62).
The majority (88%) of patients in our cohort had RA. Manifestations of T-LGL leukemia in the setting of RA are sometimes clinically indistinguishable from Felty’s syndrome (FS), a rare subset of seropositive RA complicated by neutropenia and often splenomegaly. The detection of a clonal rearrangement of TCR genes and/or an expanded T-LGL population (≥ 2 × 109/L) supports the diagnosis of T-LGL leukemia but not of FS (63–66). As reported previously, PCR-based TCR gene rearrangement analysis according to the BIOMED-2 protocol followed by GeneScan analysis made the detection of clonal T-cell populations possible with a sensitivity of 5% (19, 67). Due to the low clonal expansion of T-LGLs in “gray-zone” cases, detection of a clonal peak may be impossible or questionable, making diagnosis much more difficult. Immunohistochemical examination of bone marrow biopsies is recommended in “gray-zone” T-LGL leukemia cases (7), but due to the expansion of reactive cytotoxic T-lymphocytes in patients with RA, this study cannot reliably distinguish T-LGL leukemia in the setting of RA from FS (64, 66).
Because TCR-γ gene rearrangement occurs in both αβTCR+ and γδTCR+ T-cells, it is the best target for analysis of T-cell clonality (19, 67). On the other hand, the TCR-β gene has more expansive combinatorial diversity in contrast to the TCR-γ gene, making the TCR-β gene the preferred target for identifying a true clonal process in αβTCR+ proliferation. In our patient cohort, a clonal rearrangement of the TCR-β gene was detected significantly more often than monoclonal rearrangements of the TCR-γ gene both in the peripheral blood and bone marrow. According to the BIOMED-2 group recommendations (68, 69), TCR-γ and TCR-β gene rearrangements were analyzed simultaneously in our work. Blood and bone marrow examination provided complementary information and allowed the detection of T-cell clonality in 22 (88%) of the 25 patients.
Three patients in our cohort presented a particular diagnostic challenge. These patients had seropositive RA, severe neutropenia, and massive splenomegaly, which, in the absence of evidence for clonal proliferation of LGLs in the blood and bone marrow, allowed an initial misdiagnosis of FS. Morphological examination of spleen samples showed infiltration of cords and sinusoids of the red pulp by cytotoxic T-lymphocytes with more marked involvement within the splenic cords, which is characteristic of spleen involvement by T-LGL leukemia (70). A monoclonal TCR gene rearrangement was detected in the spleen in all the 3 patients, and a STAT3 mutation was present in 1 of them. These patients presented an unusual variant of T-LGL leukemia, in which tumor cells are localized in the spleen, while very few or no tumor cells in the blood and bone marrow prevented the diagnosis of T-LGL leukemia. Because the term “leukemia” is used when there is extensive peripheral blood and bone marrow involvement, we suggest the term “splenic variant T-LGL leukemia” for such cases.
In conclusion, we have described a cohort of patients with various ARDs and expansion of T-LGLs of less than 0.5 × 109/L in the peripheral blood. Such cases present a diagnostic challenge because of the low clone size and require both TCR-γ and TCR-β gene rearrangement analysis in the blood and bone marrow. Although cases with a very small clonal population of T-LGLs remain the subject of terminological debate (T-LGL leukemia versus TCUS), clinical manifestations such as neutropenia and splenomegaly have allowed us to classify these cases as T-LGL leukemia.
STAT3 mutations were detected by NGS in peripheral blood, bone marrow, and spleen in a total of 56% of patients in our cohort, providing an argument for considering these cases as atypical aleukemic presentation of T-LGL leukemia. In diagnostically unclear cases of neutropenia and/or splenomegaly in patients with ARDs, the detection of STAT3 mutations by NGS can indicate the diagnosis of T-LGL leukemia.
Data availability statement
The data presented in this study are deposited in the SRA repository, accession number: PRJNA867539.
Ethics statement
The studies involving human participants were reviewed and approved by the V.A. Nasonova Research Institute of Rheumatology (protocol #10 on the 20-01-2022). The patients/participants provided their written informed consent to participate in this study.
Author contributions
VG: data collection, analysis and interpretation, literature search, study design, and writing – review and editing the manuscript. YS, BB, NK, NR, and AS: data collection, analysis and interpretation, literature search, editing, and reviewing of the manuscript. All authors contributed to the manuscript and approved the submitted version.
Acknowledgments
We thank Editage (www.editage.com) for English language editing.
Conflict of interest
The authors declare that the research was conducted in the absence of any commercial or financial relationships that could be construed as a potential conflict of interest.
Publisher’s note
All claims expressed in this article are solely those of the authors and do not necessarily represent those of their affiliated organizations, or those of the publisher, the editors and the reviewers. Any product that may be evaluated in this article, or claim that may be made by its manufacturer, is not guaranteed or endorsed by the publisher.
References
1. Lamy T, Loughran TP Jr. Clinical features of large granular lymphocyte leukemia. Semin Hematol. (2003) 40:185–95. doi: 10.1016/s0037-1963(03)00133-1
2. Chan WC, Foucar K, Morice WG, Matutes E. T-cell large granular lymphocytic leukemia. 4th ed. In: Swerdlow SH, Campo E, Harris NL, Jaffe ES, Pileri SA, Stein H, et al. editors. WHO Classification of Tumours of Haematopoietic and Lymphoid Tissues. Revised. Lyon: International Agency for Research on Cancer (2017). p. 348–50.
4. Semenzato G, Zambello R, Starkebaum G, Oshimi K, Loughran TP Jr. The lymphoproliferative disease of granular lymphocytes: updated criteria for diagnosis. Blood. (1997) 89:256–60.
5. Moignet A, Lamy T. Latest advances in the diagnosis and treatment of large granular lymphocytic leukemia. Am Soc Clin Oncol Educ Book. (2018) 38:616–25. doi: 10.1200/EDBK_200689
6. Semenzato G, Pandolfi F, Chisesi T, De Rossi G, Pizzolo G, Zambello R, et al. The lymphoproliferative disease of granular lymphocytes. A heterogeneous disorder ranging from indolent to aggressive conditions. Cancer. (1987) 60:2971–8. doi: 10.1002/1097-0142(19871215)60:123.0.co;2-o
7. Lamy T, Moignet A, Loughran TP Jr. LGL leukemia: from pathogenesis to treatment. Blood. (2017) 129:1082–94. doi: 10.1182/blood-2016-08-692590
8. Bareau B, Rey J, Hamidou M, Donadieu J, Morcet J, Reman O, et al. Analysis of a French cohort of patients with large granular lymphocyte leukemia: a report on 229 cases. Haematologica. (2010) 95:1534–41. doi: 10.3324/haematol.2009.018481
9. Cheon H, Dziewulska KH, Moosic KB, Olson KC, Gru AA, Feith DJ, et al. Advances in the diagnosis and treatment of large granular lymphocytic leukemia. Curr Hematol Malig Rep. (2020) 15:103–12. doi: 10.1007/s11899-020-00565-6
10. Lamy T, Loughran TP Jr. Clinical manifestations, pathologic features, and diagnosis of T cell large granular lymphocyte leukemia. In: Larson RA editor. UpToDate. Waltham, MA: UpToDate (2021).
11. Evans HL, Burks E, Viswanatha D, Larson RS. Utility of immunohistochemistry in bone marrow evaluation of T lineage large granular lymphocyte leukemia. Hum Pathol. (2000) 31:1266–73. doi: 10.1053/hupa.2000.19298
12. Morice WG, Kurtin PJ, Tefferi A, Hanson CA. Distinct bone marrow findings in T-cell granular lymphocytic leukemia revealed by paraffin section immunoperoxidase stains for CD8. TIA-1, and granzyme B. Blood. (2002) 99:268–74. doi: 10.1182/blood.v99.1.268
13. Posnett DN, Sinha R, Kabak S, Russo C. Clonal populations of T cells in normal elderly humans: the T cell equivalent to “benign monoclonal gammapathy”. J Exp Med. (1994) 179:609–18. doi: 10.1084/jem.179.2.609
14. Grunewald J, Jeddi-Tehrani M, Dersimonian H, Andersson R, Wigzell H. A persistent T cell expansion in the peripheral blood of a normal adult male: a new clinical entity? Clin Exp Immunol. (1992) 89:279–84. doi: 10.1111/j.1365-2249.1992.tb06945.x
15. Koskela HL, Eldfors S, Ellonen P, van Adrichem AJ, Kuusanmäki H, Andersson EI, et al. Somatic STAT3 mutations in large granular lymphocytic leukemia. N Engl J Med. (2012) 366:1905–13. doi: 10.1056/NEJMoa1114885
16. Jerez A, Clemente MJ, Makishima H, Koskela H, Leblanc F, Peng NGK, et al. STAT3 mutations unify the pathogenesis of chronic lymphoproliferative disorders of NK cells and T-cell large granular lymphocyte leukemia. Blood. (2012) 120:3048–57. doi: 10.1182/blood-2012-06-435297
17. Fasan A, Kern W, Grossmann V, Haferlach C, Haferlach T, Schnittger S. STAT3 mutations are highly specific for large granular lymphocytic leukemia. Leukemia. (2013) 27:1598–600. doi: 10.1038/leu.2012.350
18. Shi M, He R, Feldman AL, Viswanatha DS, Jevremovic D, Chen D, et al. STAT3 mutation and its clinical and histopathologic correlation in T-cell large granular lymphocytic leukemia. Hum Pathol. (2018) 73:74–81. doi: 10.1016/j.humpath.2017.12.014
19. van Dongen JJ, Langerak AW, Brüggemann M, Evans PA, Hummel M, Lavender FL, et al. Design and standardization of PCR primers and protocols for detection of clonal immunoglobulin and T-cell receptor gene recombinations in suspect lymphoproliferations: report of the BIOMED-2 Concerted Action BMH4-CT98-3936. Leukemia. (2003) 17:2257–317. doi: 10.1038/sj.leu.2403202
20. Li H, Handsaker B, Wysoker A, Fennell T, Ruan J, Homer N, et al. 1000 Genome project data processing subgroup. The sequence alignment/map format and SAMtools. Bioinformatics. (2009) 25:2078–9. doi: 10.1093/bioinformatics/btp352
21. Li H, Durbin R. Fast and accurate long-read alignment with Burrows-Wheeler transform. Bioinformatics. (2010) 26:589–95. doi: 10.1093/bioinformatics/btp698
22. Bolger AM, Lohse M, Usadel B. Trimmomatic: a flexible trimmer for Illumina sequence data. Bioinformatics. (2014) 30:2114–20. doi: 10.1093/bioinformatics/btu170
23. Lai Z, Markovets A, Ahdesmaki M, Chapman B, Hofmann O, McEwen R, et al. VarDict: a novel and versatile variant caller for next-generation sequencing in cancer research. Nucleic Acids Res. (2016) 44:e108. doi: 10.1093/nar/gkw227
24. Wang K, Li M, Hakonarson H. ANNOVAR: functional annotation of genetic variants from high-throughput sequencing data. Nucleic Acids Res. (2010) 38:e164. doi: 10.1093/nar/gkq603
25. Sherry ST, Ward MH, Kholodov M, Baker J, Phan L, Smigielski EM, et al. DBSNP: the NCBI database of genetic variation. Nucleic Acids Res. (2001) 29:308–11. doi: 10.1093/nar/29.1.308
26. O’Leary NA, Wright MW, Brister JR, Ciufo S, Haddad D, McVeigh R, et al. Reference sequence (RefSeq) database at NCBI: current status, taxonomic expansion, and functional annotation. Nucleic Acids Res. (2016) 44:D733–45. doi: 10.1093/nar/gkv1189
27. Sayers EW, Beck J, Bolton EE, Bourexis D, Brister JR, Canese K, et al. Database resources of the national center for biotechnology information. Nucleic Acids Res. (2021) 49:D10–7. doi: 10.1093/nar/gkaa892
28. Tate JG, Bamford S, Jubb HC, Sondka Z, Beare DM, Bindal N, et al. COSMIC: the catalogue of somatic mutations in cancer. Nucleic Acids Res. (2019) 47:D941–7. doi: 10.1093/nar/gky1015
29. Schwaneck EC, Renner R, Junker L, Einsele H, Gadeholt O, Geissinger E, et al. Prevalence and characteristics of persistent clonal T cell large granular lymphocyte expansions in rheumatoid arthritis: a comprehensive analysis of 529 patients. Arthritis Rheumatol. (2018) 70:1914–22. doi: 10.1002/art.40654
30. Zhang R, Shah MV, Loughran TP Jr. The root of many evils: indolent large granular lymphocyte leukaemia and associated disorders. Hematol Oncol. (2010) 28:105–17. doi: 10.1002/hon.917
31. Rose MG, Berliner N. T-cell large granular lymphocyte leukemia and related disorders. Oncologist. (2004) 9:247–58. doi: 10.1634/theoncologist.9-3-247
32. O’Malley DP. T-cell large granular leukemia and related proliferations. Am J Clin Pathol. (2007) 127:850–9. doi: 10.1309/A8FHDA0VVRJ05GJP
33. Bravo-Pérez C, Carrillo-Tornel S, García-Torralba E, Jerez A. Persistent large granular lymphocyte clonal expansions: “the root of many evils”-and of some goodness. Cancers. (2022) 14:1340. doi: 10.3390/cancers14051340
34. Mohty M, Faucher C, Vey N, Chabannon C, Sainty D, Arnoulet C, et al. Features of large granular lymphocytes (LGL) expansion following allogeneic stem cell transplantation: a long-term analysis. Leukemia. (2002) 16:2129–33. doi: 10.1038/sj.leu.2402645
35. Kim D, Al-Dawsari G, Chang H, Panzarella T, Gupta V, Kuruvilla J, et al. Large granular lymphocytosis and its impact on long-term clinical outcomes following allo-SCT. Bone Marrow Transplant. (2013) 48:1104–11. doi: 10.1038/bmt.2013.5
36. Kataria A, Cohen E, Saad E, Atallah E, Bresnahan B. Large granular lymphocytic leukemia presenting late after solid organ transplantation: a case series of four patients and review of the literature. Transplant Proc. (2014) 46:3278–81. doi: 10.1016/j.transproceed.2014.05.083
37. Qiu ZY, Tian GY, Zhang Z, Zhang YQ, Xu W, Li JY. Large granular lymphocytosis after transplantation. Oncotarget. (2017) 8:81697–708. doi: 10.18632/oncotarget.21009
38. Alfano G, Fontana F, Colaci E, Mori G, Cerami C, Messerotti A, et al. T-cell large granular lymphocyte leukemia in solid organ transplant recipients: case series and review of the literature. Int J Hematol. (2019) 110:313–21. doi: 10.1007/s12185-019-02682-2
39. Smith PR, Cavenagh JD, Milne T, Howe D, Wilkes SJ, Sinnott P, et al. Benign monoclonal expansion of CD8+ lymphocytes in HIV infection. J Clin Pathol. (2000) 53:177–81. doi: 10.1136/jcp.53.3.177
40. Delobel P, Godel A, Thebault S, Alric L, Duffaut M. Transient clonal expansion of T-large granular lymphocytes during primary cytomegalovirus infection. J Infect. (2006) 53:e65–7. doi: 10.1016/j.jinf.2005.10.013
42. Barilà G, Calabretto G, Teramo A, Vicenzetto C, Gasparini VR, Semenzato G, et al. T cell large granular lymphocyte leukemia and chronic NK lymphocytosis. Best Pract Res Clin Haematol. (2019) 32:207–16. doi: 10.1016/j.beha.2019.06.006
43. Teramo A, Gattazzo C, Passeri F, Lico A, Tasca G, Cabrelle A, et al. Intrinsic and extrinsic mechanisms contribute to maintain the JAK/STAT pathway aberrantly activated in T-type large granular lymphocyte leukemia. Blood. (2013) 121:3843–54. doi: 10.1182/blood-2012-07-441378
44. Gentile TC, Loughran TP Jr. Interleukin-12 is a costimulatory cytokine for leukemic CD3+ large granular lymphocytes. Cell Immunol. (1995) 166:158–61. doi: 10.1006/cimm.1995.0018
45. Zambello R, Trentin L, Cassatella MA, Raimondi R, Cerutti A, Enthammer C, et al. IL-12 is involved in the activation of CD3+ granular lymphocytes in patients with lymphoproliferative disease of granular lymphocytes. Br J Haematol. (1996) 92:308–14. doi: 10.1046/j.1365-2141.1996.d01-1495.x
46. Zambello R, Facco M, Trentin L, Sancetta R, Tassinari C, Perin A, et al. Interleukin-15 triggers the proliferation and cytotoxicity of granular lymphocytes in patients with lymphoproliferative disease of granular lymphocytes. Blood. (1997) 89:201–11.
47. Chen J, Petrus M, Bamford R, Shih JH, Morris JC, Janik JE, et al. Increased serum soluble IL-15Rα levels in T-cell large granular lymphocyte leukemia. Blood. (2012) 119:137–43. doi: 10.1182/blood-2011-04-346759
48. Epling-Burnette PK, Liu JH, Catlett-Falcone R, Turkson J, Oshiro M, Kothapalli R, et al. Inhibition of STAT3 signaling leads to apoptosis of leukemic large granular lymphocytes and decreased Mcl-1 expression. J Clin Invest. (2001) 107:351–62. doi: 10.1172/JCI9940
49. Bigouret V, Hoffmann T, Arlettaz L, Villard J, Colonna M, Ticheli A, et al. Monoclonal T-cell expansions in asymptomatic individuals and in patients with large granular leukemia consist of cytotoxic effector T cells expressing the activating CD94:NKG2C/E and NKD2D killer cell receptors. Blood. (2003) 101:3198–204. doi: 10.1182/blood-2002-08-2408
50. Bernardin F, Doukhan L, Longone-Miller A, Champagne P, Sekaly R, Delwart E. Estimate of the total number of CD8+clonal expansions in healthy adults using a new DNA heteroduplex tracking assay for CDR3 repertoire analysis. J Immunol Methods. (2003) 274:159–75. doi: 10.1016/s0022-1759(02)00514-8
51. Shi M, Olteanu H, Jevremovic D, He R, Viswanatha D, Corley H, et al. T-cell clones of uncertain significance are highly prevalent and show close resemblance to T-cell large granular lymphocytic leukemia. Implications for laboratory diagnostics. Mod Pathol. (2020) 33:2046–57. doi: 10.1038/s41379-020-0568-2
52. Sidorova YV, Sychevskaya KA, Chernova NG, Julhakyan HL, Smirnova SJ, Ryzhikova NV, et al. High incidence of clonal CD8+ T-cell proliferation in non-malignant conditions may reduce the significance of T-cell clonality assay for differential diagnosis in oncohematology. Clin Lymphoma Myeloma Leuk. (2020) 20:203–8. doi: 10.1016/j.clml.2019.12.021
53. Dhodapkar MV, Li CY, Lust JA, Tefferi A, Phyliky RL. Clinical spectrum of clonal proliferations of T-large granular lymphocytes: a T-cell clonopathy of undetermined significance? Blood. (1994) 84:1620–7.
54. Sabnani I, Tsang P. Are clonal T-cell large granular lymphocytes to blame for unexplained haematological abnormalities? Br J Haematol. (2007) 136:30–7. doi: 10.1111/j.1365-2141.2006.06374.x
55. Singleton TP, Yin B, Teferra A, Mao JZ. Spectrum of clonal large granular lymphocytes (LGLs) of alphabeta T cells: T-cell clones of undetermined significance. T-cell LGL leukemias, and T-cell immunoclones. Am J Clin Pathol. (2015) 144:137–44. doi: 10.1309/AJCPJ57YTEGLIUOI
56. Shahmarvand N, Nagy A, Shahryari J, Ohgami RS. Mutations in the signal transducer and activator of transcription family of genes in cancer. Cancer Sci. (2018) 109:926–33. doi: 10.1111/cas.13525
57. Kim D, Myllymäki M, Kankainen M, Jarvinen T, Park G, Bruhn R, et al. Somatic STAT3 mutations in CD8+ T cells of healthy blood donors carrying human T-cell leukemia virus Type 2. Haematologica. (2022) 107:550–4. doi: 10.3324/haematol.2021.279140
58. Kastritis E, Leblond V, Dimopoulos MA, Kimby E, Staber P, Kersten MJ, et al. ESMO Guidelines Committee. Waldenström’s macroglobulinaemia: ESMO clinical practice guidelines for diagnosis, treatment and follow-up. Ann Oncol. (2018) 29(Suppl. 4):iv41–50. doi: 10.1093/annonc/mdy146
59. Marti GE, Rawstron AC, Ghia P, Hillmen P, Houlston RS, Kay N, et al. International familial CLL consortium. Diagnostic criteria for monoclonal B-cell lymphocytosis. Br J Haematol. (2005) 130:325–32. doi: 10.1111/j.1365-2141.2005.05550.x
60. Galigalidou C, Zaragoza-Infante L, Iatrou A, Chatzidimitriou A, Stamatopoulos K, Agathangelidis A. Understanding monoclonal B cell lymphocytosis: an interplay of genetic and microenvironmental factors. Front Oncol. (2021) 11:769612. doi: 10.3389/fonc.2021.769612
61. Sanikommu SR, Clemente MJ, Chomczynski P, Afable MG II, Jerez A, Thota S, et al. Clinical features and treatment outcomes in large granular lymphocytic leukemia (LGLL). Leuk Lymphoma. (2018) 59:416–22. doi: 10.1080/10428194.2017.1339880
62. Dong N, Castillo Tokumori F, Isenalumhe L, Zhang Y, Tandon A, Knepper TC, et al. Large granular lymphocytic leukemia - a retrospective study of 319 cases. Am J Hematol. (2021) 96:772–80. doi: 10.1002/ajh.26183
63. Balint GP, Balint PV. Felty’s syndrome. Best Pract Res Clin Rheumatol. (2004) 18:631–45. doi: 10.1016/j.berh.2004.05.002
64. Burks EJ, Loughran TP Jr. Pathogenesis of neutropenia in large granular lymphocyte leukemia and Felty syndrome. Blood Rev. (2006) 20:245–66. doi: 10.1016/j.blre.2006.01.003
65. Shah A, Diehl LF, St Clair EW. T cell large granular lymphocyte leukemia associated with rheumatoid arthritis and neutropenia. Clin Immunol. (2009) 132:145–52. doi: 10.1016/j.clim.2009.03.515
66. Gorodetskiy VR, Sidorova YV, Kupryshina NA, Vasilyev VI, Probatova NA, Ryzhikova NV, et al. Analysis of a single-institution cohort of patients with Felty’s syndrome and T-cell large granular lymphocytic leukemia in the setting of rheumatoid arthritis. Rheumatol Int. (2021) 41:147–56. doi: 10.1007/s00296-020-04757-4
67. Mendoza H, Tormey CA, Rinder HM, Howe JG, Siddon AJ. The utility and limitations of B- and T-cell gene rearrangement studies in evaluating lymphoproliferative disorders. Pathology. (2021) 53:157–65. doi: 10.1016/j.pathol.2020.09.024
68. van Krieken JH, Langerak AW, Macintyre EA, Kneba M, Hodges E, Sanz RG, et al. Improved reliability of lymphoma diagnostics via PCR-based clonality testing: report of the BIOMED-2 Concerted Action BHM4-CT98-3936. Leukemia. (2007) 21:201–6. doi: 10.1038/sj.leu.2404467
69. Groenen PJ, Langerak AW, van Dongen JJ, van Krieken JH. Pitfalls in TCR gene clonality testing: teaching cases. J Hematop. (2008) 1:97–109. doi: 10.1007/s12308-008-0013-9
Keywords: T-cell large granular lymphocytic leukemia, low tumor burden, STAT3 mutation, next-generation sequencing, autoimmune rheumatic diseases
Citation: Gorodetskiy V, Sidorova Y, Biderman B, Kupryshina N, Ryzhikova N and Sudarikov A (2022) STAT3 mutations in “gray-zone” cases of T-cell large granular lymphocytic leukemia associated with autoimmune rheumatic diseases. Front. Med. 9:1000265. doi: 10.3389/fmed.2022.1000265
Received: 21 July 2022; Accepted: 08 August 2022;
Published: 31 August 2022.
Edited by:
Ching-Mao Chang, Taipei Veterans General Hospital, TaiwanReviewed by:
Sinem Civriz Bozdağ, Ankara University, TurkeyHongqiang Shen, Zhejiang University School of Medicine, China
Copyright © 2022 Gorodetskiy, Sidorova, Biderman, Kupryshina, Ryzhikova and Sudarikov. This is an open-access article distributed under the terms of the Creative Commons Attribution License (CC BY). The use, distribution or reproduction in other forums is permitted, provided the original author(s) and the copyright owner(s) are credited and that the original publication in this journal is cited, in accordance with accepted academic practice. No use, distribution or reproduction is permitted which does not comply with these terms.
*Correspondence: Vadim Gorodetskiy, gorodetskiyblood@mail.ru