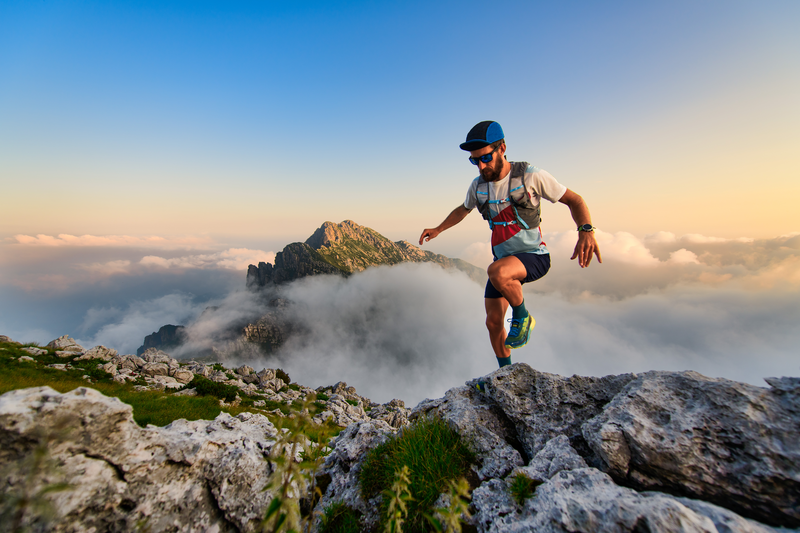
94% of researchers rate our articles as excellent or good
Learn more about the work of our research integrity team to safeguard the quality of each article we publish.
Find out more
REVIEW article
Front. Med. , 17 January 2022
Sec. Ophthalmology
Volume 8 - 2021 | https://doi.org/10.3389/fmed.2021.815075
This article is part of the Research Topic Reviews in: Ophthalmology 2022 View all 22 articles
Dry eye is currently one of the most common ocular surface disease. It can lead to ocular discomfort and even cause visual impairment, which greatly affects the work and quality of life of patients. With the increasing incidence of dry eye disease (DED) in recent years, the disease is receiving more and more attention, and has become one of the hot research fields in ophthalmology research. Recently, with the in-depth research on the etiology, pathogenesis and treatment of DED, it has been shown that defects in immune regulation is one of the main pathological mechanisms of DED. Since the non-specific and specific immune response of the ocular surface are jointly regulated, a variety of immune cells and inflammatory factors are involved in the development of DED. The conventional treatment of DED is the application of artificial tears for lubricating the ocular surface. However, for moderate-to-severe DED, treatment with anti-inflammatory drugs is necessary. In this review, the immunomodulatory mechanisms of DED and the latest research progress of its related treatments including Chinese medicine will be discussed.
The global prevalence of DED is 5 to 50%, and it increases linearly with age, with a higher prevalence among Asians (1–4). Common symptoms of DED include dryness of eye, foreign body sensation, burning sensation, itching, photophobia, eye redness, blurred vision, fluctuating vision and visual fatigue. In severe cases, corneal epithelial peeling, filiform adhesion and keratoconjunctival lesions can also occur. Traditionally, DED is defined as a disorder of the eye caused by tear film instability and ocular surface damage due to abnormal tear quality or quantity. In 2017, the TFOS DEWS II Working Group of the Tear Film and Ocular Surface Society (TFOS DEWS II) defined DED as a disorder of the ocular surface with a loss of tear film homeostasis and ocular symptoms caused by tear film instability, increased osmolarity, ocular surface inflammation, damage to the ocular surface and neurosensory abnormalities (5).
The ocular surface (cornea, conjunctiva and meibomian glands), the lacrimal gland, and the neural connections between them together form the Lacrimal Functional Unit (LFU), which regulates tear secretion, tear film formation and maintains the health of the ocular surface (6). Structural or functional damage to any component of the LFU can disrupt the integrity and function of the tear film, leading to dry eye disease (DED). Although the pathogenic mechanisms of DED has not been fully elucidated, ocular surface inflammation in DED becomes an important focus. Therefore, there have been relatively more studies investigating the roles of immune factors such as ocular surface inflammatory cells and inflammatory mediators in DED in recent years.
Both innate and adaptive immunity are tightly regulated in the ocular surface environment to protect and maintain ocular surface homeostasis (7). When this immune homoeostasis is being disrupted, it can lead to DED (8–12). Ocular surface immune responses, involving the participation of helper T (Th), memory T and regulatory T (Treg) lymphocytes, usually occur on the surface of the cornea, including the ocular tissues and regional lymph nodes (13). Innate immunity, also known as intrinsic immunity or nonspecific immunity, is gradually developed by organisms during long-term evolution. It is the body's front-line defense against pathogen invasion and can initiate and interact with adaptive immunity. Innate immune cells include monocytes, macrophages, neutrophils, dendritic cells, natural killer cells (NK cells), γδ T lymphocytes, etc. (14–16). Adaptive immunity, also known as acquired or specific immunity, is highly specific to a particular pathogenic microorganism (17). Innate immunity and adaptive immunity are complementary and inseparable (Table 1) (34). Unlike other mucosal surfaces, the ocular surface microenvironment is continuously exposed to the environmental factors and helps to monitor the invasion of microorganisms, contaminants, allergens and other harmful substances. The immune system respond accordingly to the acute or chronic nature of the invasion process of the ocular surface in DED (Figure 1).
Figure 1. The immunoinflammatory response of the ocular surface in dry eye disease. Desiccating, oxidative and hyperosmolarity stress activate cell signaling pathways at the ocular surface, which leads to the production of pro-inflammatory cytokines (TNF-α, IL-1β and IL-6) and matrix metalloproteinase (mainly MMP9). These factors promote the maturation of antigen-presenting cells and allow mature antigen-presenting cells to migrate to the lymph nodes through the afferent lymphatic vessels. In the lymph nodes, mAPCs induce effector T cells (Th 1 and Th17) and recruit them to migrate to the ocular surface. Meanwhile, mAPCs activate the NLRP3 inflammasome, promotes the secretion of IL-1β and IL-18, and further aggravates the ocular surface inflammation. MAPK, mitogen-activated protein kinase; JNK, Jun N-terminal kinase; ERK, extracellular regulated protein kinase; NF-κB, nuclear transcription factor-κB; MMPs, matrix metalloproteinases; TNF-α, tumor necrosis factor-α; IL, interleukin; IFN-γ, interferon-γ; NLRP3, NLR family pyrin domain containing 3; TGF-β, transforming growth factor β; Th, T helper; Treg, regulatory T cell; LFA-1, lymphocyte function associated antigen 1; ICAM-1, intercellular adhesion molecule 1; APC, antigen presenting cell; mAPC, mature antigen presenting cell; ASC, apoptosis speck-like protein.
A key function of the innate immune system is to provide a physical barrier between the eye and the external environment, and thus to prevent the microbial attachment and toxins from crossing the surface epithelium. Components responsible for this function include mucins in the tear fluid, glycocalyx, corneal and conjunctival epithelium, and a series of anti-microbial defense proteins (lactoferrin, lysozyme, lipid transport proteins, tripeptides, defensins) (35).
Existing studies have shown that a large number of lymphocytes infiltration can be seen in the lacrimal gland and conjunctival tissue of dry eye patients (36). The infiltrated inflammatory cells produce pro-inflammatory cytokines. The secretion of natural anti-inflammatory factors (lactoferrin) in the tear fluid is reduced, and the scope of inflammation is gradually expanded (37, 38). At present, the pathogenic mechanism of dry eye has not been fully elucidated, but inflammation is closely related to the occurrence and development of the dry eye. Inflammation can affect the stability of the tear film on the ocular surface, thereby increasing the tear osmotic pressure (39). These changes further induce damage to the ocular surface and initiate an inflammatory cascade of innate and adaptive immune responses.
In 2007, the international dry eye workshop (DEWS) introduced the increase in tear osmotic pressure into the concept of dry eye, indicating that the increase in tear osmotic pressure is an important feature of dry eye pathological damage. Tomlinson et al. (40) used an osmotic pressure exceeding 316 mOsm/L as the diagnostic criteria for dry eye, and found that the sensitivity and specificity were 59 and 94% respectively. Suzuki et al. (41) evaluated the symptoms and signs of 19 dry eye patients and found that the increase in tear osmotic pressure was highly correlated with the severity of dry eye. Increased tear osmotic pressure in patients with dry eye can induce ocular surface inflammation. VanDerMeid et al. (42) found that increased tear osmotic pressure is positively correlated with cytokines (IL-1α, IL-6, TNF-α) and matrix metalloproteinase 2, 9 and 10. The hyperosmotic state of DED can disrupt this defense system by activating various signaling pathways; for instance, mitogen-activated protein kinase (MAPK), c-Jun N-terminal kinases (JNKs), extracellular regulated protein kinases (ERKs) pathways and in particular, the p38 pathway, which in turn activates nuclear transcription factor-κB (NF-κB), leading to the release of interleukin (IL)-1 and tumor necrosis factor-α (TNF-α). This inflammatory mechanism can then induce the release of other downstream mediators and the activation of cellular signaling that amplify inflammatory response (43, 44). In addition, the activation of pathogen-related pattern recognition receptors (PRR) is also involved in the inflammatory response of DED (45). In innate immune cells, Toll-like receptors (TLRs)-3,7 & 9 and RNA sensors-mediated signaling can lead to the activation of inflammatory caspases within signaling platforms called inflammasomes, with subsequent maturation and secretion of a variety of pro-inflammatory cytokines such as IL-1β and IL-18 [38].
The expression of inflammatory IL-1β, TNF-α and IL-6 of the ocular surface epithelium is critical for the inflammatory response in DED. Knockdown of IL-1 receptors in mice results in a significant reduction in inflammatory factors produced by the cornea and conjunctiva (46). In the ocular surface, chemokines such as CC chemokine ligand (CCL)3, CCL4, CCL5, CXCL9, C-X-C motif chemokine ligand (CXCL)10 and CX3CL1 can recruit macrophages, dendritic cells, neutrophils, activate T cells and upregulate the corresponding chemokine receptors (47, 48). Another key factor is the expression of intercellular adhesion molecule 1 (ICAM-1) in corneal and conjunctival epithelium, which binds to its ligand lymphocyte function associated antigen 1 (LFA-1) at sites of inflammation and lymph (49). Innate and adaptive immune responses occur in different regions of the ocular surface, but they share common key molecular interactions that promote cell migration. The interaction between ICAM-1 and LFA-1 is critical for immune cell proliferation and infiltration, and can be a potential therapeutic target.
The cells involved in the innate immune response in DED are mainly neutrophils, NK cells and monocytes/macrophages. Neutrophils constitute the first line of defense for the host innate immune response. Although they are low in number in the normal conjunctiva, they are abundant on the ocular surface of patients with severe aqueous-deficient dry eye (50). Neutrophil depletion leads to the increased CD4+ Th cell activation and the increased extent of corneal staining, suggesting that neutrophils may play certain protective role (51). A recent study has suggested that NK cells may play an important role in the pathogenesis of DED (22). The recruitment or activation of NK cells at the ocular surface enhances the production of inflammatory cytokines such as interferon-γ (IFN-γ), IL-6 and Th17-related IL-17, which stimulate macrophages, antigen presenting cells (APC) and autoreactive T lymphocytes. IFN-γ is responsible for T helper 1 (Th1) cell activation and differentiation, and can cause cell-mediated conjunctival epithelial damage and goblet cell loss (26). Monocytes infiltrated to the conjunctiva can be differentiated into two subtypes of macrophages: M1 cells associated with pro-inflammatory responses and M2 cells responsible for immunoregulation. Studies have shown that DED mainly induces the differentiation of monocytes toward the M1 phenotype (52). The innate immune system also includes γδ T cells and the complement system. The γδ T cells are often present near epithelial cells, and they can produce IL-17 at the ocular surface, but their specific role for DED remains unknown. Studies on the role of complement in ocular surface inflammation in DED have been limited to the observations in animal models, where nude mice receiving serum from mice with DED can develop DED with the recruitment of inflammatory cells and production of cytokines induced by complement C3a/C5a and C3b/C5b activation (53). In addition to the above factors, inflammatory mediators associated with DED include IL-2, IL-4, IL-5, CXCL8, matrix metalloproteinase 3, macrophage inflammatory protein 2, epidermal growth factor, lactoferrin, transforming growth factor (TGF), mucin MUC5AC and S100 protein. Changes in these inflammatory factors can be detected in the ocular surface of DED patients. Most of the above inflammatory factors are found in dry syndrome type of DED and the changes are more pronounced on the ocular surface (54).
The presence of CD4+ Th cells on the ocular surface in DED and the efficacy of topical cyclosporine in treating ocular surface inflammation provide valid evidence for the involvement of the adaptive immune response in DED. Adaptive immunity mainly involves the production and recruitment of effector T cells (55, 56). The initiation of an adaptive immune response requires antigens at the site of inflammation to be processed and presented by APC, which then migrate to the lymphoid tissue to activate and induce the proliferation of specific effector T cells (57). Although the antigen that initiates this response remains unknown, study has suggested that the expression of autoantigens is critical for triggering the inflammatory epithelial lesions in DED (58). Since the ocular surface in inflammatory states is characterized by the upregulation of major histocompatibility complex class II (MHCII) and other stimulatory signals (IL-6 and IL-17 etc.), the recruitment of activated T cells to the conjunctiva and cornea of patients with DED may be an alternative pathway for antigen presentation in the adaptive immune response (53). After mature APC and CD4+ T cells combine in the lymph nodes through immune synapses (59), they secrete IL-6, IL-12, IL-17, IL-23, TGF-β and IFN-γ, together with pro-inflammatory cytokines from the corneal epithelial cells, promote the differentiation of CD4+ T cells into Th1, T helper 2 (Th2), T helper 17 (Th17) and Treg cells (60). Effector T cells then undergo migration and recruitment to the conjunctival stroma (61) for the activation of residual APC, in turn induce the damage to the ocular surface epithelium and dysfunction through the release of inflammatory cytokines IL-1β, TNF-α and IL-6 (62–65). Th1 and Th17 are the main Th lymphocyte subsets that account for ocular surface dysfunction (66, 67). IFN-γ secreted by Th1 cells promotes the apoptosis of ocular surface epithelial cells and the loss of conjunctival goblet cells (68, 69). IL-17 secreted by Th17 cells promotes corneal epithelial cells and fibroblasts to produce matrix metalloproteinases (MMPs), which leads to the destruction of the corneal epithelial barrier and further damage of the ocular surface (70–72).
Although the spleen is the primary lymphoid tissue responsible for intraocular immune regulation, its role in ocular surface inflammation is not predominant. The role of the thymus in the regulation of ocular surface immune responses is unknown. However, animal models of DED and the observation of the ocular surface status of patients with graft-vs.-host disease causing thymic damage prior to hematopoietic stem cell transplantation suggest that the central tolerance regulated by the thymic environment is important for ocular surface immunity (73). Conjunctival-associated lymphoid tissue that reaches the epithelial cell surface will respond according to local antigen exposure to form germinal centers, which can take part in mucosal tolerance as well as immune defense against ocular surface inflammation (74).
Pyroptosis, also known as cellular inflammatory necrosis, is a newly discovered form of cell death that is important to innate immune response. It is manifested by the continuous expansion of cells until the cell membrane ruptures, leading to the release of cellular contents and thus the activation of an intense inflammatory response (75). Cell death is dependent on caspase-1 and its PRR, NLR family pyrin domain containing 3 (NLRP3) inflammasome, which cleaves the inactive caspase-1 precursor to be active caspase-1 (76). Caspase-1 can induce cell membrane perforation to cause cell lysis and death, with the subsequent release of intracellular materials through the disrupted cell membrane to induce an inflammatory response. Caspase-1 can also cleave the IL-1β and IL-18 precursors to produce and secrete the active mature IL-1β and IL-18. IL-18 then induce the synthesis and release of other inflammatory cytokines and amplify local and systemic inflammatory responses (77). It has been demonstrated that the expression of mRNA and protein of NLRP3, caspase-1, IL-1β and IL-18 is elevated in the ocular surface of patients with DED (78).
A distinguishing feature of the human meibomian gland is its resistance to inflammation and infection. When keratoconjunctival epithelial cells are exposed to bacterial toxins, they cause the significant upregulation of immune-related genes, cytokine and chemokine expression, TLR signaling pathways, and inflammatory and immune responses. However, epithelial cells of the meibomian gland do not upregulate pro-inflammatory gene expression and TLR signaling upon the exposure to bacterial toxins (79), leading to the speculation that the meibomian gland may have inherent anti-inflammatory and anti-infective factors. The most highly expressed gene in the meibomian gland is the leukocyte-associated immunoglobulin-like receptor, an inhibitory receptor that inhibits immune cell activation and reduces the production of pro-inflammatory cytokines (80). Moreover, the immunoglobulin-like receptor gene expression is upregulated upon epithelial cell differentiation in the human meibomian gland (81). Recently, researchers have also found that human meibomian gland epithelial cell lysates inhibit the growth rate of gram-negative bacteria and pseudomonas aeruginosa (57).
Ocular surface desiccating stress can induce autoreactive T cells. When the patient has immunodeficiency, it can cause Sjogren's syndrome-like inflammation in the lacrimal gland, cornea and conjunctiva, but it does not interfere with other organs (82). The desiccating stress acting on the ocular surface activates intracellular signal transduction pathways as a trigger condition to release pro-inflammatory factors that promote the maturation and activation of immature APC. The infiltration of APC to lymph nodes through lymphatic vessels, together with the subsequent migration of Th1 and Th17 cells to the ocular surface. IFN-γ and IL-17 together exert pathogenic properties and cause squamous metaplasia of ocular surface epithelial cells. IFN-γ decreases goblet cell differentiation and IL-17 disrupts corneal epithelial barrier function, while IFN-γ and IL-17 also enhance the production of pro-inflammatory cytokines, chemokines, MMPs, cell adhesion molecules and vascular endothelial growth factor. These inflammatory mediators again promote the maturation and migration of APC. This creates a vicious cycle of inflammation that ultimately leads to the destabilization of the ocular surface and tear film, as well as an increase in tear osmolarity (83, 84).
The complications of dryness in DED may vary from a serious abnormal feeling sensation to contact lens intolerance (85). Currently, the methods of treating DED worldwide mainly include the application of ocular surface lubricants to protect the mucous membrane and the use of anti-ocular surface inflammation drugs, punctual plug placement to reduce tear loss, and physical therapy of the eyelids to restore the meibomian glands (86). However, various methods can only reduce but not completely eliminate the symptoms of DED. Artificial tears (87), silicone eye shields (88), gas lenses (89) and corneal contact lenses (90) are the basic treatment for DED, but in moderate-to-severe DED with ocular surface inflammation, anti-inflammatory and immunosuppressive treatments are essential (Table 2) (128).
Tetracycline and its derivatives are broad-spectrum antibiotics with anti-inflammatory properties. Tetracycline can reduce the activity of collagenase and phospholipase A2, inhibit the activity of MMP-9 in the ocular surface tissues, and downregulate the expression of inflammatory cytokine IL-1β and TNF-α. Doxycycline and minocycline are commonly used ocular surface anti-inflammatory drugs, they are both tetracycline derivatives. In an experimental mouse model of DED, topical application of doxycycline has soothing effects, function as a barrier to the corneal epithelium and reduces ocular surface inflammation (129). Tetracycline and its derivatives are commonly used in the treatment of DED caused by meibomian gland dysfunction (MGD) and blepharitis. There is no consensus standard dosage at present, but doctors can make adjustments on the dosage according to the patient's condition.
Non-steroidal anti-inflammatory drugs overcome the defects of many adverse reactions and strong drug dependency of corticosteroid drugs. NSAIDs such as ketorolac tromethamine can inhibit the activity of cyclooxygenase, block the synthesis of prostaglandin, reduce the migration and phagocytosis of granulocytes and monocytes, and achieve the purpose of controlling ocular surface inflammation. However, for DED caused by autoimmune diseases such as Sjogren's syndrome, the immunomodulatory activity of NSAIDs is much lower than that of glucocorticoids (130). Moreover, some NSAIDs have reduced corneal sensitivity in both normal subjects and patients with DED (131). Therefore, NSAIDs are not recommended for patients with mild DED dominated by environmental factors (low humidity and smoke exposure, etc.) (132).
Pranoprofen is a propionic acid-derived NSAID that can relieve the symptoms of DED. It is currently used widely in ophthalmology clinics. Liu et al. (99) found that the combination of pranoprofen and sodium hyaluronate can quickly control the symptoms of DED, effectively stimulate the secretion of tears, and achieve significant clinical effects. It may be related to the fact that pranoprofen reduces the level of inflammatory mediators and prevents the accumulation of leukocytes in eyes. Diclofenac sodium is a typical non-steroidal anti-inflammatory drug, which has antipyretic, analgesic and anti-inflammatory effects to improve vision, reduce photophobia and alleviate the clinical symptoms of DED. It can be completely absorbed, with full infiltration into the eye tissue after 30 min of medication (133). Studies have shown that diclofenac eye drops combined with sodium hyaluronate in the treatment of DED can improve eye symptoms significantly, with good safety without irritation and induction of infection upon long-term use (101). Bromfenac sodium eye drops have good penetrability, the abilities to maintain the blood concentration of the ocular surface for a long time and improve the inflammatory response of patients with DED (108). It has been shown that while bromfenac sodium eye drops not only relieve the symptoms of DED but also promote the improvement of the patient's lacrimal gland function and prevent the development of inflammation (134).
Glucocorticoids have a rapid onset of action, including the inhibition of the inflammatory factor production, downregulation of pro-inflammatory mediator IL-1 and TNF-α by inhibiting the NF-kB signal pathway, and induction of lymphocyte apoptosis. Methylprednisolone is a synthetic corticosteroid and Methylprednisolone (1%) can inhibit the expression of MMP-9 and MAPK activity in the corneal epithelium of mice with DED. The use of glucocorticoids for 2–4 weeks can significantly improve the symptoms of DED, but its long-term use can cause complications such as high intraocular pressure and cataracts (135).
Flumetholone is a glucocorticoid drug with a high rate of clinical use. Flumetholone can effectively neutralize inflammatory mediators, improve non-infectious inflammatory symptoms, and decrease lymphocytes and macrophages activation, thereby enhancing the anti-inflammatory effect. Fluorometholone eye drops (0.1%) is safe and has low incidence of adverse effects (136). Loteprednol is a new type of glucocorticoid drug (137) that has recently been approved by the FDA for the treatment of short-term DED (138). Loteprednol can fully exert therapeutic effects in DED by inducing the inhibition of phospholipase A. Loteprednol has low toxicity with high fat-solubility and good corneal permeability.
Korenfeld et al. (139) found that loteprednol has a significant clinical effect in the treatment of moderate-to-severe DED. For examples, it can effectively improve dry eye symptoms, promote the recovery of corneal damage and stabilize the tear film of DED patients.
Cyclosporine A (CsA), an immunosuppressant, is a fungal metabolite that inhibits Th1-related IL-2 activation. It has been used in anti-rejection therapy for organ transplantation, autoimmune diseases, local allergy, corneal limbal stem cell dysfunction and autoimmune uveitis. CsA (0.05%) was approved by the FDA in 2003 for the treatment of moderate-to-severe DED (140), as it can reduce inflammatory cells and inflammatory mediators on the ocular surface, inhibit the apoptosis of lacrimal cells and conjunctival goblet cells, promote lymphocyte apoptosis, and reduce the ocular surface inflammatory response. It has been shown that CsA eye drops combined with vitamin A can significantly increase the amount of tear secretion in dry eye rats, prolong the tear film rupture time, and alleviate the apoptosis of corneal cells (141). Tacrolimus is a new type of immunosuppressant with the same action as CsA, but with stronger anti-inflammatory effects and fewer side effects than CsA. It has been shown that tacrolimus can effectively alleviate the pathological changes of conjunctival epithelial cell hyperplasia and goblet cell reduction caused by Sjogren's syndrome in mice (142). Moscovici et al. (120) used tacrolimus (0.03%) to treat patients with Sjogren's syndrome, suggesting that tacrolimus in the form of eye drops may have positive effects when administered locally for treating severe autoimmune DED caused by Sjogren's syndrome. It has also been shown that tacrolimus is effective in treating dry eyes caused by Sjogren's syndrome, Stevens-Johnson syndrome, graft-vs.-host disease and diabetes (140, 143).
The main ligand of LFA-1 is ICAM-1, which is expressed on the cell surface of endothelial cells, epithelial cells and APC (144). The binding of LFA-1 to ICAM-1 is known as the “immune synapse” and is the key step in T-cell activation. Lifitegrast is a new type of small molecule T lymphocyte inhibitor, which can effectively suppress the migration of APC, as well as the migration and recruitment of T lymphocytes to the ocular surface by inhibiting the interaction between LFA-1 and ICAM-1 (145). Lifitegrast is structurally similar to ICAM-1 and acts as a competitive antagonist to block LFA-1 and ICAM-1 binding and hence reduce the release of inflammatory factors (145). Lifitegrast (5%) reduces dry eye scores and ocular discomfort with a good safety and tolerability profile (146). Experimental studies have shown that Lifitegrast can inhibit the levels of IL-1β, IFN-γ and IL-10, and can increase the number and spread of conjunctival goblet cells (147). It was approved by the FDA in 2016 to be a novel drug for the treatment of DED (146).
RGN-259 is a natural polypeptide composed of 43 amino acids, which is the main protein of wound repairing cells (platelets, macrophages and polymorphonuclear cells) (148). For the treatment of DED and neurotrophic keratopathy, RGN-259 is a new preservative-free peptide eye drops with Thymosin β4 (Tβ4) as the active ingredient developed by RegeneRx. Preclinical studies have shown that Tβ4 can promote the migration of corneal epithelial cells, increase cell-interstitial contact, reduce cell apoptosis and reduce corneal inflammation. The Phase II clinical trial (ARISE-2) ended in October 2017 showed that RGN-259 (0.1%) provides ocular comfort, is safe and highly tolerable (149). The symptoms of DED were significantly improved 15 days after treatment, and corneal staining was also significantly improved 15 days and 29 days after treatment (150).
In recent years, several biological agents have also been shown to reduce ocular surface inflammation, improve dry eye symptoms and corneal staining. These biologicals include lubricating agents, recombinant human nerve growth factor, IL-1 receptor antagonists, anti-TNF-α agents, anti-IL-17 agents, calcitonin gene-related peptides and neuropeptide Y (140). Although many biologic agents have been thoroughly studied in animal models, clinical studies in humans are still scarce and human clinical trials with randomized, double-blind and placebo-controlled Good Clinical Practice are needed to further evaluate their therapeutic effects.
China has a long history of using traditional Chinese medicine to treat DED. Long-term clinical practice has confirmed that TCM has a pronounced systemic therapeutic effect on DED. In recent years, in-depth research has also proven the multi-targeted efficacy of TCM extracts in treating a variety of DED endotypes. The reported TCM for the treatment of the dry eye disease is summarized in the Table 3. Zhang et al. investigated the effect of extracts of Bidens pilosa L. on tear secretion in dry eye rats induced by finasteride, they found that Bidens pilosa L. extracts could increase tear production and prolong breakup time (BUT). Moreover, Bidens pilosa L. extracts can increase the tear film stability, inhibit the inflammatory response of the lacrimal gland and reduce the pro-inflammatory cytokines (151). A series of animal experiments confirmed that the extract of Buddleja offcinalis is effective for the treatment of rabbit dry eye model, and the extract of Buddleja offcinalis has the effect of inhibiting the expression of inflammatory cytokines TNF-α and IL-1β. The extract of Buddleja offcinalis can significantly improve the pathological changes of the lacrimal gland tissue, increase the production of tears and the stability of the tear film. The extract of Buddleja offcinalis can also inhibit the apoptosis of lacrimal gland cells and inhibit the expression of IL-1β and TNF-α in the lacrimal gland tissue (152). The main component of Buddleja offcinalis is flavonoids, which have a similar effect to androgens, so its extracts can improve the ocular surface symptoms in castrated male rats with dry eyes (153). Yao XL et al. studied the efficacy of total flavonoids from chrysanthemum on castration-induced dry eye in male rabbits and its effect on the expression of Bax and Bcl-2 in lacrimal gland cells and found that total flavonoids from chrysanthemum can significantly inhibit the occurrence of the dry eye accompanied with the apoptosis of lacrimal gland cells and maintain the stability of the tear film (154). The total flavonoids of chrysanthemum have an effect on the pro-apoptotic factor Bax in lacrimal gland cells and the activation of the anti-apoptotic factor Bcl-2, thereby inhibiting the apoptosis of lacrimal epithelial cells and improving ocular surface symptoms (155). In a rat model of dry eye induced by particulate matter 2.5 (PM2.5), Lee et al. found that the water extract of Corni Fructus can alleviate the decrease in tear secretion and corneal epithelial damage. In addition, the water extract of Corni Fructus can inhibit the apoptosis of conjunctival goblet cells and down-regulate the expression of inflammatory factors in the lacrimal gland. Corni Fructus water extracts help to improve retinal function and lipid metabolism disorders (156). Park et al. (158) used polydatin eye drops to treat dry eye models in rats with lacrimal gland excision and found that polydatin eye drops can improve tear reduction and repair corneal damage. Polydatin eye drops can reduce tear film BUT and reduce goblet cell loss. In addition, polydatin can reduce the expression of inflammatory cytokines and NLRP3 in conjunctival tissues (159). According to Chinese medicine, middle-aged and elderly people are prone to the symptoms of liver and kidney “Yin” deficiency, which are manifested as dryness in the eyes and foreign body sensation. There are many descriptions for middle-aged and elderly dry eye syndrome, especially in menopausal women. Prunella vulgaris exerts the effect of lowering blood pressure and cleaning the liver. It has been shown that the administration of Prunella vulgaris can significantly improve the diagnostic indicators of dry eye in menopausal women. Prunella vulgaris can reduce the cytokine production of TNF-α and IL-1β, and reduce ocular surface inflammation (161). Lycium barbarum belongs to the genus Lycium barbarum of the Solanaceae family which is the mature fruit of its plant. Chinese medicine believes that Lycium barbarum has the effects of nourishing “Yin”, repairing the liver and improving eyesight. Lycium barbarum is rich in vitamin A and carotene, which are the components of rhodopsin in the visual cells that sense slight light, and play an important role in maintaining normal visual function. Liu et al. found that in human retinal epithelial cells and dry eye rat models, Lycium barbarum can not only improve the stability of the tear film, promote tear secretion, but also repair the cornea, reduce oxidative stress, and improve the secretion function of the meibomian glands (168, 169).
Based on our previous research findings, the water extract of Dendrobium officinale can relieve the symptoms of DED in rats and inhibit the expression of IL-1β and TNF-α (171, 172). Aquaporins (AQPs) participate in the water transport across cell membranes driven by osmotic pressure, so as to maintain the balance of the volume of body cavity fluid and saline. Various studies have shown that the expression of AQP5 in ocular tissues was reduced in the state of dry eye. Our results demonstrated that the water extract of Dendrobium officinale possess therapeutic effects in treating DED via activating and upregulating AQP5 and MUC5AC in conjunctival cells (172). Dry eye disease not only induces symptoms on the ocular surface but also systemic symptoms. With the continuous in-depth study of dry eye syndrome in traditional Chinese medicine, besides monomeric Chinese herbal extracts, Chinese herbal formulas and decoctions are also commonly used methods. Therefore, when modern medicine has not achieved a particularly ideal treatment for dry eye disease, we should give full play to the advantages of traditional Chinese medicine to assist modern medicine in the joint treatment of dry eye disease. The combination of traditional Chinese medicine and Western medicine can often achieve better results.
The pathogenesis of DED is complex and multifactorial. Currently, inflammation at the ocular surface is the main cause of DED. The corresponding anti-inflammatory pathways are the key targets in DED treatment. On the other hand, current animal models and in vitro studies have confirmed that inflammatory cells and inflammatory factors are closely associated with the tear film instability and the elevated tear osmolarity. Differential activation of distinct intracellular signal transduction pathways will lead to the release of inflammatory factors from inflammatory immune cells. These inflammatory mediators will then promote the infiltration of more pro-inflammatory cells to the local inflammatory sites, thus forming a vicious cycle of inflammation. Although a more detailed mechanisms of ocular surface inflammation requires further investigation, blocking the vicious cycle of ocular inflammation by suppressing various signaling pathways, or the application of multi-targeted TCM could be the tactics in the treatment of DED.
JL and BC reviewed the literature and wrote the manuscript. MT, XG, PL, CL, and J-MH reviewed and revised the manuscript. CW reviewed the literature and contributed to the conceptualization of the manuscript. All authors contributed to the article and approved the submitted version.
This study was supported by State Key Laboratory of Research on Bioactivities and Clinical Applications of Medicinal Plants, CUHK from Innovation and Technology Commission, Hong Kong and funding from Li Dak Sum Yip Yio Chin R&D Center for Chinese Medicine, the Chinese University of Hong Kong.
The authors declare that the research was conducted in the absence of any commercial or financial relationships that could be construed as a potential conflict of interest.
All claims expressed in this article are solely those of the authors and do not necessarily represent those of their affiliated organizations, or those of the publisher, the editors and the reviewers. Any product that may be evaluated in this article, or claim that may be made by its manufacturer, is not guaranteed or endorsed by the publisher.
APC/mAPC, antigen presenting cell/mature antigen presenting cell; AQP, aquaporin; BUT, break-up time; CsA, cyclosporine A; CCL, CC chemokine ligand; CXCL, C-X-C motif chemokine ligand; DED, dry eye disease; ERK, extracellular regulated protein kinase; ICAM-1, intercellular adhesion molecule 1; IFN-γ, interferon-γ; IL, interleukin; JNK, jun N-terminal kinase; LFA-1, lymphocyte function associated antigen 1; LFU, lacrimal functional unit; MAPK, mitogen-activated protein kinase; MGD, meibomian gland dysfunction; MHCII, major histocompatibility complex class II; MMP, matrix metalloproteinase; NF-κB, nuclear transcription factor-κB; NK cells, natural killer cells; NLRP3, NLR family pyrin domain containing 3; NSAIDs, non-steroidal anti-inflammatory drugs; PRR, pattern recognition receptor; TCM, Traditional Chinese Medicine; TGF, transforming growth factor; Th, T helper; TLR, Toll-like receptor; TNF-α, tumor necrosis factor-α; Tβ4, thymosin β4.
1. Galor A, Feuer W, Lee DJ, Florez H, Carter D, Pouyeh B, et al. Prevalence and risk factors of dry eye syndrome in a United States veterans affairs population. Am J Ophthalmol. (2011) 152:377–84 e2. doi: 10.1016/j.ajo.2011.02.026
2. Le Q, Ge L, Li M, Wu L, Xu J, Hong J, et al. Comparison on the vision-related quality of life between outpatients and general population with dry eye syndrome. Acta Ophthalmol. (2014) 92:e124–32. doi: 10.1111/aos.12204
3. Stapleton F, Alves M, Bunya VY, Jalbert I, Lekhanont K, Malet F, et al. TFOS DEWS II Epidemiology Report. Ocul Surf. (2017) 15:334–65. doi: 10.1016/j.jtos.2017.05.003
4. Gomes JAP, Santo RM. The impact of dry eye disease treatment on patient satisfaction and quality of life: A review. Ocul Surf. (2019) 17:9–19. doi: 10.1016/j.jtos.2018.11.003
5. Hantera MM. Trends in dry eye disease management worldwide. Clin Ophthalmol. (2021) 15:165–73. doi: 10.2147/OPTH.S281666
6. Pflugfelder SC, Stern ME. The cornea in keratoconjunctivitis sicca. Exp Eye Res. (2020) 201:108295. doi: 10.1016/j.exer.2020.108295
7. Mochizuki M, Sugita S, Kamoi K. Immunological homeostasis of the eye. Prog Retin Eye Res. (2013) 33:10–27. doi: 10.1016/j.preteyeres.2012.10.002
8. Stern ME, Schaumburg CS, Siemasko KF, Gao J, Wheeler LA, Grupe DA, et al. Autoantibodies contribute to the immunopathogenesis of experimental dry eye disease. Invest Ophthalmol Vis Sci. (2012) 53:2062–75. doi: 10.1167/iovs.11-9299
9. Guzman M, Keitelman I, Sabbione F, Trevani AS, Giordano MN, Galletti JG. Mucosal tolerance disruption favors disease progression in an extraorbital lacrimal gland excision model of murine dry eye. Exp Eye Res. (2016) 151:19–22. doi: 10.1016/j.exer.2016.07.004
10. Pflugfelder SC, de Paiva CS. The pathophysiology of dry eye disease: what we know and future directions for research. Ophthalmology. (2017) 124:S4–S13. doi: 10.1016/j.ophtha.2017.07.010
11. Barbosa FL, Xiao Y, Bian F, Coursey TG, Ko BY, Clevers H, et al. Goblet cells contribute to ocular surface immune tolerance-implications for dry eye disease. Int J Mol Sci. (2017) 18. doi: 10.3390/ijms18050978
12. Bertoni A, Alabiso O, Galetto AS, Baldanzi G. Integrins in T cell physiology. Int J Mol Sci. (2018) 19. doi: 10.3390/ijms19020485
13. Yamaguchi T. Inflammatory response in dry eye. Invest Ophthalmol Vis Sci. (2018) 59:DES192–DES9. doi: 10.1167/iovs.17-23651
14. Zhang X, Volpe EA, Gandhi NB, Schaumburg CS, Siemasko KF, Pangelinan SB, et al. NK cells promote Th-17 mediated corneal barrier disruption in dry eye. PLoS ONE. (2012) 7:e36822. doi: 10.1371/journal.pone.0036822
15. Stevenson W, Chauhan SK, Dana R. Dry eye disease: an immune-mediated ocular surface disorder. Arch Ophthalmol. (2012) 130:90–100. doi: 10.1001/archophthalmol.2011.364
16. Strauss-Albee DM, Horowitz A, Parham P, Blish CA. Coordinated regulation of NK receptor expression in the maturing human immune system. J Immunol. (2014) 193:4871–9. doi: 10.4049/jimmunol.1401821
17. Hu D, Tjon EC, Andersson KM, Molica GM, Pham MC, Healy B, et al. Aberrant expression of USF2 in refractory rheumatoid arthritis and its regulation of proinflammatory cytokines in Th17 cells. Proc Natl Acad Sci U S A. (2020) 117:30639–48. doi: 10.1073/pnas.2007935117
18. Bian F, Pelegrino FS, Henriksson JT, Pflugfelder SC, Volpe EA, Li DQ, et al. Differential effects of dexamethasone and doxycycline on inflammation and mmp production in murine alkali-burned corneas associated with dry eye. Ocul Surf. (2016) 14:242–54. doi: 10.1016/j.jtos.2015.11.006
19. Manoussakis MN, Boiu S, Korkolopoulou P, Kapsogeorgou EK, Kavantzas N, Ziakas P, et al. Rates of infiltration by macrophages and dendritic cells and expression of interleukin-18 and interleukin-12 in the chronic inflammatory lesions of Sjogren's syndrome: correlation with certain features of immune hyperactivity and factors associated with high risk of lymphoma development. Arthritis Rheum. (2007) 56:3977–88. doi: 10.1002/art.23073
20. Sisto M, Lisi S, Ingravallo G, Lofrumento DD, D'Amore M, Ribatti D. Neovascularization is prominent in the chronic inflammatory lesions of Sjogren's syndrome. Int J Exp Pathol. (2014) 95:131–7. doi: 10.1111/iep.12061
21. You IC, Coursey TG, Bian F, Barbosa FL, de Paiva CS, Pflugfelder SC. Macrophage Phenotype in the Ocular Surface of Experimental Murine Dry Eye Disease. Arch Immunol Ther Exp (Warsz). (2015) 63:299–304. doi: 10.1007/s00005-015-0335-0
22. Chen Y, Chauhan SK, Saban DR, Sadrai Z, Okanobo A, Dana R. Interferon-gamma-secreting NK cells promote induction of dry eye disease. J Leukoc Biol. (2011) 89:965–72. doi: 10.1189/jlb.1110611
23. De Paiva CS, Raince JK, McClellan AJ, Shanmugam KP, Pangelinan SB, Volpe EA, et al. Homeostatic control of conjunctival mucosal goblet cells by NKT-derived IL-13. Mucosal Immunol. (2011) 4:397–408. doi: 10.1038/mi.2010.82
24. Lee MH, Choi JW, Jang WR, Kim JM, Kim JH. Activation of eosinophils is more closely linked with interleukin-5 and nitric oxide production than tumor necrosis factor-alpha and immunoglobulin E levels. Acta Haematol. (2013) 130:238–41. doi: 10.1159/000350474
25. Galletti JG, Guzman M, Giordano MN. Mucosal immune tolerance at the ocular surface in health and disease. Immunology. (2017) 150:397–407. doi: 10.1111/imm.12716
26. Alam J, de Paiva CS, Pflugfelder SC. Desiccation induced conjunctival monocyte recruitment and activation - implications for keratoconjunctivitis. Front Immunol. (2021) 12:701415. doi: 10.3389/fimmu.2021.701415
27. Yoon KC, Park CS, You IC, Choi HJ, Lee KH, Im SK, et al. Expression of CXCL9,−10,−11, and CXCR3 in the tear film and ocular surface of patients with dry eye syndrome. Invest Ophthalmol Vis Sci. (2010) 51:643–50. doi: 10.1167/iovs.09-3425
28. Pflugfelder SC. Tear dysfunction and the cornea: LXVIII Edward Jackson Memorial Lecture. Am J Ophthalmol. (2011) 152:900–9 e1. doi: 10.1016/j.ajo.2011.08.023
29. Bettelli E, Oukka M, Kuchroo VK. T(H)-17 cells in the circle of immunity and autoimmunity. Nat Immunol. (2007) 8:345–50. doi: 10.1038/ni0407-345
30. De Paiva CS, Chotikavanich S, Pangelinan SB, Pitcher JD. 3rd, Fang B, Zheng X, et al. IL-17 disrupts corneal barrier following desiccating stress. Mucosal Immunol. (2009) 2:243–53. doi: 10.1038/mi.2009.5
31. Barabino S, Montaldo E, Solignani F, Valente C, Mingari MC, Rolando M. Immune response in the conjunctival epithelium of patients with dry eye. Exp Eye Res. (2010) 91:524–9. doi: 10.1016/j.exer.2010.07.008
32. McClellan AJ, Volpe EA, Zhang X, Darlington GJ, Li DQ, Pflugfelder SC, et al. Ocular surface disease and dacryoadenitis in aging C57BL/6 mice. Am J Pathol. (2014) 184:631–43. doi: 10.1016/j.ajpath.2013.11.019
33. Subbarayal B, Chauhan SK, Di Zazzo A, Dana R. IL-17 augments B cell activation in ocular surface autoimmunity. J Immunol. (2016) 197:3464–70. doi: 10.4049/jimmunol.1502641
34. Tsubota K, Pflugfelder SC, Liu Z, Baudouin C, Kim HM, Messmer EM, et al. Defining dry eye from a clinical perspective. Int J Mol Sci. (2020) 21:9271. doi: 10.3390/ijms21239271
35. Yu L, Yu C, Dong H, Mu Y, Zhang R, Zhang Q, et al. Recent Developments About the Pathogenesis of Dry Eye Disease: Based on Immune Inflammatory Mechanisms. Front Pharmacol. (2021) 12:732887. doi: 10.3389/fphar.2021.732887
36. Sekhon AS, He B, Iovieno A, Yeung SN. Pathophysiology of corneal endothelial cell loss in dry eye disease and other inflammatory ocular disorders. Ocul Immunol Inflamm. (2021) 1–11. doi: 10.1080/09273948.2021.1980808
37. Ponzini E, Scotti L, Grandori R, Tavazzi S, Zambon A. Lactoferrin concentration in human tears and ocular diseases: a meta-analysis. Invest Ophthalmol Vis Sci. (2020) 61:9. doi: 10.1167/iovs.61.12.9
38. Zhang Y, Lu C, Zhang J. Lactoferrin and its detection methods: a review. Nutrients. (2021) 13:2492. doi: 10.3390/nu13082492
39. van Setten GB. Osmokinetics: A new dynamic concept in dry eye disease. J Fr Ophtalmol. (2019) 42:221–5. doi: 10.1016/j.jfo.2018.11.001
40. Tomlinson A, Khanal S, Ramaesh K, Diaper C, McFadyen A. Tear film osmolarity: determination of a referent for dry eye diagnosis. Invest Ophthalmol Vis Sci. (2006) 47:4309–15. doi: 10.1167/iovs.05-1504
41. Suzuki M, Massingale ML, Ye F, Godbold J, Elfassy T, Vallabhajosyula M, et al. Tear osmolarity as a biomarker for dry eye disease severity. Invest Ophthalmol Vis Sci. (2010) 51:4557–61. doi: 10.1167/iovs.09-4596
42. VanDerMeid KR, Su SP, Ward KW, Zhang JZ. Correlation of tear inflammatory cytokines and matrix metalloproteinases with four dry eye diagnostic tests. Invest Ophthalmol Vis Sci. (2012) 53:1512–8. doi: 10.1167/iovs.11-7627
43. Luo L, Li DQ, Doshi A, Farley W, Corrales RM, Pflugfelder SC. Experimental dry eye stimulates production of inflammatory cytokines and MMP-9 and activates MAPK signaling pathways on the ocular surface. Invest Ophthalmol Vis Sci. (2004) 45:4293–301. doi: 10.1167/iovs.03-1145
44. Li DQ, Luo L, Chen Z, Kim HS, Song XJ, Pflugfelder SC, et al. and ERK MAP kinases mediate induction of IL-1beta, TNF-alpha and IL-8 following hyperosmolar stress in human limbal epithelial cells. Exp Eye Res. (2006) 82:588–96. doi: 10.1016/j.exer.2005.08.019
45. Shimizu T, Nakamura H, Kawakami A. Role of the innate immunity signaling pathway in the pathogenesis of sjogren's syndrome. Int J Mol Sci. (2021) 22:3090. doi: 10.3390/ijms22063090
46. Simmons KT, Xiao Y, Pflugfelder SC, de Paiva CS. Inflammatory response to lipopolysaccharide on the ocular surface in a murine dry eye model. Invest Ophthalmol Vis Sci. (2016) 57:2443–51. doi: 10.1167/iovs.15-18396
47. Karin N. CXCR3 ligands in cancer and autoimmunity, chemoattraction of effector t cells, and beyond. Front Immunol. (2020) 11:976. doi: 10.3389/fimmu.2020.00976
48. Perez VL, Stern ME, Pflugfelder SC. Inflammatory basis for dry eye disease flares. Exp Eye Res. (2020) 201:108294. doi: 10.1016/j.exer.2020.108294
49. Haber SL, Benson V, Buckway CJ, Gonzales JM, Romanet D, Scholes B. Lifitegrast: a novel drug for patients with dry eye disease. Ther Adv Ophthalmol. (2019) 11:2515841419870366. doi: 10.1177/2515841419870366
50. An S, Raju I, Surenkhuu B, Kwon JE, Gulati S, Karaman M, et al. Neutrophil extracellular traps (NETs) contribute to pathological changes of ocular graft-vs-host disease (oGVHD) dry eye: Implications for novel biomarkers and therapeutic strategies. Ocul Surf. (2019) 17:589–614. doi: 10.1016/j.jtos.2019.03.010
51. Galletti JG, de Paiva CS. The ocular surface immune system through the eyes of aging. Ocul Surf. (2021) 20:139–62. doi: 10.1016/j.jtos.2021.02.007
52. Ho TC, Yeh SI, Chen SL, Tsao YP. Integrin alphav and vitronectin prime macrophage-related inflammation and contribute the development of dry eye disease. Int J Mol Sci. (2021) 22:8410. doi: 10.3390/ijms22168410
53. Steven P, Schwab S, Kiesewetter A, Saban DR, Stern ME, Gehlsen U. Disease-specific expression of conjunctiva associated lymphoid tissue (CALT) in mouse models of dry eye disease and ocular allergy. Int J Mol Sci. (2020) 21:7514. doi: 10.3390/ijms21207514
54. Willcox MDP, Argueso P, Georgiev GA, Holopainen JM, Laurie GW, Millar TJ, et al. TFOS DEWS II tear film report. Ocul Surf. (2017) 15:366–403. doi: 10.1016/j.jtos.2017.03.006
55. Pflugfelder SC, Corrales RM, de Paiva CS. T helper cytokines in dry eye disease. Exp Eye Res. (2013) 117:118–25. doi: 10.1016/j.exer.2013.08.013
56. Chen Y, Dana R. Autoimmunity in dry eye disease - An updated review of evidence on effector and memory Th17 cells in disease pathogenicity. Autoimmun Rev. (2021) 102933. doi: 10.1016/j.autrev.2021.102933
57. Bron AJ, de Paiva CS, Chauhan SK, Bonini S, Gabison EE, Jain S, et al. TFOS DEWS II pathophysiology report. Ocul Surf. (2017) 15:438–510. doi: 10.1016/j.jtos.2017.05.011
58. Ushio A, Arakaki R, Eguchi H, Hotta F, Yamada A, Kudo Y, et al. Pathological analysis of ocular lesions in a murine model of sjogren's syndrome. Int J Mol Sci. (2017) 18:1209. doi: 10.3390/ijms18061209
59. Teijeira A, Hunter MC, Russo E, Proulx ST, Frei T, Debes GF, et al. T cell migration from inflamed skin to draining lymph nodes requires intralymphatic crawling supported by ICAM-1/LFA-1 interactions. Cell Rep. (2017) 18:857–65. doi: 10.1016/j.celrep.2016.12.078
60. Chivasso C, Sarrand J, Perret J, Delporte C, Soyfoo MS. The involvement of innate and adaptive immunity in the initiation and perpetuation of sjogren's syndrome. Int J Mol Sci. (2021) 22:658. doi: 10.3390/ijms22020658
61. Reyes JL, Vannan DT, Eksteen B, Avelar IJ, Rodriguez T, Gonzalez MI, et al. Innate and adaptive cell populations driving inflammation in dry eye disease. Mediators Inflamm. (2018) 2018:2532314. doi: 10.1155/2018/2532314
62. Muller WA. How endothelial cells regulate transmigration of leukocytes in the inflammatory response. Am J Pathol. (2014) 184:886–96. doi: 10.1016/j.ajpath.2013.12.033
63. Rouzaut A, Garasa S, Teijeira A, Gonzalez I, Martinez-Forero I, Suarez N, et al. Dendritic cells adhere to and transmigrate across lymphatic endothelium in response to IFN-alpha. Eur J Immunol. (2010) 40:3054–63. doi: 10.1002/eji.201040523
64. Jackson DG. Leucocyte Trafficking via the Lymphatic Vasculature- Mechanisms and Consequences. Front Immunol. (2019) 10:471. doi: 10.3389/fimmu.2019.00471
65. Periman LM, Perez VL, Saban DR, Lin MC, Neri P. The immunological basis of dry eye disease and current topical treatment options. J Ocul Pharmacol Ther. (2020) 36:137–46. doi: 10.1089/jop.2019.0060
66. El Annan J, Chauhan SK, Ecoiffier T, Zhang Q, Saban DR, Dana R. Characterization of effector T cells in dry eye disease. Invest Ophthalmol Vis Sci. (2009) 50:3802–7. doi: 10.1167/iovs.08-2417
67. Rios-Rios WJ, Sosa-Luis SA, Torres-Aguilar H. T cells subsets in the immunopathology and treatment of sjogren's syndrome. Biomolecules. (2020) 10:1539. doi: 10.3390/biom10111539
68. Zhang X, De Paiva CS, Su Z, Volpe EA, Li DQ, Pflugfelder SC. Topical interferon-gamma neutralization prevents conjunctival goblet cell loss in experimental murine dry eye. Exp Eye Res. (2014) 118:117–24. doi: 10.1016/j.exer.2013.11.011
69. Alam J, de Paiva CS, Pflugfelder SC. Immune - Goblet cell interaction in the conjunctiva. Ocul Surf. (2020) 18:326–34. doi: 10.1016/j.jtos.2019.12.006
70. Chauhan SK, Dana R. Role of Th17 cells in the immunopathogenesis of dry eye disease. Mucosal Immunol. (2009) 2:375–6. doi: 10.1038/mi.2009.21
71. Zheng X, de Paiva CS, Li DQ, Farley WJ, Pflugfelder SC. Desiccating stress promotion of Th17 differentiation by ocular surface tissues through a dendritic cell-mediated pathway. Invest Ophthalmol Vis Sci. (2010) 51:3083–91. doi: 10.1167/iovs.09-3838
72. Rasool M, Malik A, Abdul Basit Ashraf M, Arooj M, Kiran A, Waquar S, et al. Role of diagnostic factors associated with antioxidative status and expression of matrix metalloproteinases (MMPs) in patients with cancer therapy induced ocular disorders. Saudi J Biol Sci. (2018) 25:1724–8. doi: 10.1016/j.sjbs.2018.08.009
73. Perez VL, Barsam A, Duffort S, Urbieta M, Barreras H, Lightbourn C, et al. Novel scoring criteria for the evaluation of ocular graft-versus-host disease in a preclinical allogeneic hematopoietic stem cell transplantation animal model. Biol Blood Marrow Transplant. (2016) 22:1765–72. doi: 10.1016/j.bbmt.2016.07.012
74. Mastropasqua R, Agnifili L, Fasanella V, Nubile M, Gnama AA, Falconio G, et al. The conjunctiva-associated lymphoid tissue in chronic ocular surface diseases. Microsc Microanal. (2017) 23:697–707. doi: 10.1017/S1431927617000538
75. Singh M, Tyagi SC. Hyperhomocysteinemia and Age-related Macular Degeneration: Role of Inflammatory Mediators and Pyroptosis; A Proposal. Med Hypotheses. (2017) 105:17–21. doi: 10.1016/j.mehy.2017.06.012
76. Vakrakou AG, Boiu S, Ziakas PD, Xingi E, Boleti H, Manoussakis MN. Systemic activation of NLRP3 inflammasome in patients with severe primary Sjogren's syndrome fueled by inflammagenic DNA accumulations. J Autoimmun. (2018) 91:23–33. doi: 10.1016/j.jaut.2018.02.010
77. Zhang J, Dai Y, Yang Y, Xu J. Calcitriol alleviates hyperosmotic stress-induced corneal epithelial cell damage via inhibiting the nlrp3-asc-caspase-1-gsdmd pyroptosis pathway in dry eye disease. J Inflamm Res. (2021) 14:2955–62. doi: 10.2147/JIR.S310116
78. Zheng Q, Ren Y, Reinach PS, Xiao B, Lu H, Zhu Y, et al. Reactive oxygen species activated NLRP3 inflammasomes initiate inflammation in hyperosmolarity stressed human corneal epithelial cells and environment-induced dry eye patients. Exp Eye Res. (2015) 134:133–40. doi: 10.1016/j.exer.2015.02.013
79. Yang S, Kam WR, Liu Y, Ding J, Li Y, Sullivan DA. Comparative influence of differentiation and proliferation on gene expression in human meibomian gland epithelial cells. Exp Eye Res. (2021) 205:108452. doi: 10.1016/j.exer.2021.108452
80. Ellenbroek G, de Haan JJ, van Klarenbosch BR, Brans MAD, van de Weg SM, Smeets MB, et al. Leukocyte-Associated Immunoglobulin-like Receptor-1 is regulated in human myocardial infarction but its absence does not affect infarct size in mice. Sci Rep. (2017) 7:18039. doi: 10.1038/s41598-017-13678-5
81. Rotzer V, Melega F, Garreis F, Paulsen F, Waschke J. E-Cadherin Is Important for Meibomian Gland Function as Revealed by a New Human ex Vivo Slice Culture Model. Am J Pathol. (2019) 189:1559–68. doi: 10.1016/j.ajpath.2019.04.015
82. Yoon KC, Ahn KY, Choi W, Li Z, Choi JS, Lee SH, et al. Tear production and ocular surface changes in experimental dry eye after elimination of desiccating stress. Invest Ophthalmol Vis Sci. (2011) 52:7267–73. doi: 10.1167/iovs.11-7231
83. Messmer EM. The pathophysiology, diagnosis, and treatment of dry eye disease. Dtsch Arztebl Int. (2015) 112:71–81. doi: 10.3238/arztebl.2015.0071
84. Mashaghi A, Hong JX, Chauhan SK, Dana R. Ageing and ocular surface immunity. Brit J Ophthalmol. (2017) 101:1–5. doi: 10.1136/bjophthalmol-2015-307848
86. Mittal R, Patel S, Galor A. Alternative therapies for dry eye disease. Curr Opin Ophthalmol. (2021) 32:348–61. doi: 10.1097/ICU.0000000000000768
87. Kim M, Lee Y, Mehra D, Sabater AL, Galor A. Dry eye: why artificial tears are not always the answer. BMJ Open Ophthalmol. (2021) 6:e000697. doi: 10.1136/bmjophth-2020-000697
88. Reichstein D. Current treatments and preventive strategies for radiation retinopathy. Curr Opin Ophthalmol. (2015) 26:157–66. doi: 10.1097/ICU.0000000000000141
89. Jackson AJ, Wolsley CJ. Rigid gas permeable contact lenses: out in the cold. Cont Lens Anterior Eye. (2009) 32:204–6. doi: 10.1016/j.clae.2009.06.006
90. Wu X, Ma Y, Chen X, He S, Lin X, Yu X, et al. Efficacy of bandage contact lens for the management of dry eye disease after cataract surgery. Int Ophthalmol. (2021) 41:1403–13. doi: 10.1007/s10792-021-01692-6
91. Nichols JJ, Bickle KM, Zink RC, Schiewe MD, Haque RM, Nichols KK. Safety and efficacy of topical azithromycin ophthalmic solution 10% in the treatment of contact lens-related dry eye. Eye Contact Lens. (2012) 38:73–9. doi: 10.1097/ICL.0b013e31823ff229
92. Foulks GN, Borchman D, Yappert M, Kakar S. Topical azithromycin and oral doxycycline therapy of meibomian gland dysfunction: a comparative clinical and spectroscopic pilot study. Cornea. (2013) 32:44–53. doi: 10.1097/ICO.0b013e318254205f
93. Xue AL, Downie LE, Ormonde SE, Craig JP. A comparison of the self-reported dry eye practices of New Zealand optometrists and ophthalmologists. Ophthalmic Physiol Opt. (2017) 37:191–201. doi: 10.1111/opo.12349
94. Doughty MJ. On the prescribing of oral doxycycline or minocycline by UK optometrists as part of management of chronic Meibomian Gland Dysfunction (MGD). Cont Lens Anterior Eye. (2016) 39:2–8. doi: 10.1016/j.clae.2015.08.002
95. Hagen KB, Bedi R, Blackie CA, Christenson-Akagi KJ. Comparison of a single-dose vectored thermal pulsation procedure with a 3-month course of daily oral doxycycline for moderate-to-severe meibomian gland dysfunction. Clin Ophthalmol. (2018) 12:161–8. doi: 10.2147/OPTH.S150433
96. Aronowicz JD, Shine WE, Oral D, Vargas JM, McCulley JP. Short term oral minocycline treatment of meibomianitis. Br J Ophthalmol. (2006) 90:856–60. doi: 10.1136/bjo.2006.091579
97. Schechter BA. Ketorolac tromethamine 0.4% as a treatment for allergic conjuctivitis. Expert Opin Drug Metab Toxicol. (2008) 4:507–11. doi: 10.1517/17425255.4.4.507
98. Torres-Luna C, Hu N, Tammareddy T, Domszy R, Yang J, Wang NS, et al. Extended delivery of non-steroidal anti-inflammatory drugs through contact lenses loaded with Vitamin E and cationic surfactants. Cont Lens Anterior Eye. (2019) 42:546–52. doi: 10.1016/j.clae.2019.04.011
99. Liu X, Wang S, Kao AA, Long Q. The effect of topical pranoprofen 01% on the clinical evaluation and conjunctival HLA-DR expression in dry eyes. Cornea. (2012) 31:1235–9. doi: 10.1097/ICO.0b013e31824988e5
100. Chen J, Dong F, Chen W, Sun X, Deng Y, Hong J, et al. Clinical efficacy of 01% pranoprofen in treatment of dry eye patients: a multicenter, randomized, controlled clinical trial. Chin Med J (Engl). (2014) 127:2407–12.
101. Kilic S, Kulualp K. Efficacy of several therapeutic agents in a murine model of dry eye syndrome. Comp Med. (2016) 66:112–8.
102. Kato K, Miyake K, Kondo N, Asano S, Takeda J, Takahashi A, et al. Conjunctival goblet cell density following cataract surgery with diclofenac versus diclofenac and rebamipide: a randomized trial. Am J Ophthalmol. (2017) 181:26–36. doi: 10.1016/j.ajo.2017.06.016
103. Honkanen RA, Huang L, Xie G, Rigas B. Phosphosulindac is efficacious in an improved concanavalin A-based rabbit model of chronic dry eye disease. Transl Res. (2018) 198:58–72. doi: 10.1016/j.trsl.2018.04.002
104. Huang W, Wen Z, Saglam MS, Huang L, Honkanen RA, Rigas B. Phospho-Sulindac (OXT-328) inhibits dry eye disease in rabbits: a dose-, formulation- and structure-dependent effect. J Ocul Pharmacol Ther. (2021) 37:321–30. doi: 10.1089/jop.2019.0025
105. Avunduk AM, Avunduk MC, Varnell ED, Kaufman HE. The comparison of efficacies of topical corticosteroids and nonsteroidal anti-inflammatory drops on dry eye patients: a clinical and immunocytochemical study. Am J Ophthalmol. (2003) 136:593–602. doi: 10.1016/S0002-9394(03)00326-X
106. Zhu L, Zhang C, Chuck RS. Topical steroid and non-steroidal anti-inflammatory drugs inhibit inflammatory cytokine expression on the ocular surface in the botulinum toxin B-induced murine dry eye model. Mol Vis. (2012) 8:1803–12.
107. Kawahara A, Utsunomiya T, Kato Y, Takayanagi Y. Comparison of effect of nepafenac and diclofenac ophthalmic solutions on cornea, tear film, and ocular surface after cataract surgery: the results of a randomized trial. Clin Ophthalmol. (2016) 10:385–91. doi: 10.2147/OPTH.S101836
108. Yanai K, Huang J, Kadonosono K, Uchio E. Corneal sensitivity after topical bromfenac sodium eye-drop instillation. Clin Ophthalmol. (2013) 7:741–4. doi: 10.2147/OPTH.S41801
109. Fujishima H, Fuseya M, Ogata M, Murat D. Efficacy of bromfenac sodium ophthalmic solution for treatment of dry eye disease. Asia Pac J Ophthalmol (Phila). (2015) 4:9–13. doi: 10.1097/APO.0000000000000032
110. Okanobo A, Chauhan SK, Dastjerdi MH, Kodati S, Dana R. Efficacy of topical blockade of interleukin-1 in experimental dry eye disease. Am J Ophthalmol. (2012) 154:63–71. doi: 10.1016/j.ajo.2012.01.034
111. Lee JH, Min K, Kim SK, Kim EK, Kim TI. Inflammatory cytokine and osmolarity changes in the tears of dry eye patients treated with topical 1% methylprednisolone. Yonsei Med J. (2014) 55:203–8. doi: 10.3349/ymj.2014.55.1.203
112. Kumari S, Dandamudi M, Rani S, Behaeghel E, Behl G, Kent D, et al. Dexamethasone-loaded nanostructured lipid carriers for the treatment of dry eye disease. Pharmaceutics. (2021) 13. doi: 10.3390/pharmaceutics13060905
113. Pinto-Fraga J, Enriquez-de-Salamanca A, Calonge M, Gonzalez-Garcia MJ, Lopez-Miguel A, Lopez-de la Rosa A, et al. Severity, therapeutic, and activity tear biomarkers in dry eye disease: An analysis from a phase III clinical trial. Ocul Surf. (2018) 16:368–76. doi: 10.1016/j.jtos.2018.05.001
114. Nattinen J, Jylha A, Aapola U, Enriquez-de-Salamanca A, Pinto-Fraga J, Lopez-Miguel A, et al. Topical fluorometholone treatment and desiccating stress change inflammatory protein expression in tears. Ocul Surf. (2018) 16:84–92. doi: 10.1016/j.jtos.2017.09.003
115. Taniguchi J, Sharma A. Fluorometholone modulates gene expression of ocular surface mucins. Acta Ophthalmol. (2019) 97:e1082–e8. doi: 10.1111/aos.14113
116. Sheppard JD, Donnenfeld ED, Holland EJ, Slonim CB, Solomon R, Solomon KD, et al. Effect of loteprednol etabonate 05% on initiation of dry eye treatment with topical cyclosporine 005%. Eye Contact Lens. (2014) 40:289–96. doi: 10.1097/ICL.0000000000000049
117. Chen W, Shi XL, He XH, Mao YH, Li C, Dong N. Loteprednol combined with sodium hyaluronate in the treatment of dry eye disease and its effect on TNF-alpha and CXCL10 in tears. J Biol Regul Homeost Agents. (2020) 34:1825–9.
118. Burade V, Zalawadia R, Patel A, Ogundele A. Preclinical efficacy comparison of cyclosporine ophthalmic solution 009% vs cyclosporine ophthalmic emulsion 005% vs ciclosporin ophthalmic emulsion 01% in a NOD mouse model of dry eye disease. Clin Ophthalmol. (2020) 14:2747–55. doi: 10.2147/OPTH.S259331
119. Cubuk MO, Ucgul AY, Ozgur A, Ozulken K, Yuksel E. Topical cyclosporine a (005%) treatment in dry eye patients: a comparison study of Sjogren's syndrome versus non-Sjogren's syndrome. Int Ophthalmol. (2021) 41:1479–85. doi: 10.1007/s10792-021-01708-1
120. Moscovici BK, Holzchuh R, Sakassegawa-Naves FE, Hoshino-Ruiz DR, Albers MB, Santo RM, et al. Treatment of Sjogren's syndrome dry eye using 003% tacrolimus eye drop: Prospective double-blind randomized study. Cont Lens Anterior Eye. (2015) 38:373–8. doi: 10.1016/j.clae.2015.04.004
121. Moawad P, Shamma R, Hassanein D, Ragab G, El Zawahry O. Evaluation of the effect of topical tacrolimus 0.03% versus cyclosporine 0.05% in the treatment of dry eye secondary to Sjogren syndrome. Eur J Ophthalmol. (2021) 1120672121992680. doi: 10.1177/1120672121992680
122. Alam J, de Souza RG, Yu Z, Stern ME, de Paiva CS, Pflugfelder SC. Calcineurin inhibitor voclosporin preserves corneal barrier and conjunctival goblet cells in experimental dry eye. J Ocul Pharmacol Ther. (2020) 36:679–85. doi: 10.1089/jop.2020.0005
123. Narain S, Berman N, Furie R. Biologics in the treatment of Sjogren's syndrome, systemic lupus erythematosus, and lupus nephritis. Curr Opin Rheumatol. (2020) 32:609–16. doi: 10.1097/BOR.0000000000000754
124. Gonzalez AL. Safety and efficacy of lifitegrast 5% ophthalmic solution in contact lens discomfort. Clin Ophthalmol. (2018) 12:2079–85. doi: 10.2147/OPTH.S184776
125. Tong AY, Passi SF, Gupta PK. Clinical outcomes of lifitegrast 5% ophthalmic solution in the treatment of dry eye disease. Eye Contact Lens. (2020) 46 Suppl 1:S20–S4. doi: 10.1097/ICL.0000000000000601
126. Sosne G, Kim C, Kleinman HK. Thymosin beta4 significantly reduces the signs of dryness in a murine controlled adverse environment model of experimental dry eye. Expert Opin Biol Ther. (2015) 15 Suppl 1:S155–61. doi: 10.1517/14712598.2015.1019858
127. Jin R, Li Y, Li L, Kim DH, Yang CD, Son HS, et al. Anti-inflammatory effects of glycine thymosin beta4 eye drops in experimental dry eye. Biomed Rep. (2020) 12:319–25. doi: 10.3892/br.2020.1296
128. Wolffsohn JS, Trave Huarte S, Jones L, Craig JP, Wang MTM. Ambassadors T. clinical practice patterns in the management of dry eye disease: A TFOS international survey. Ocul Surf. (2021) 21:78–86. doi: 10.1016/j.jtos.2021.04.011
129. Zhang Z, Yang WZ, Zhu ZZ, Hu QQ, Chen YF, He H, et al. Therapeutic effects of topical doxycycline in a benzalkonium chloride-induced mouse dry eye model. Invest Ophth Vis Sci. (2014) 55:2963–74. doi: 10.1167/iovs.13-13577
130. Coursey TG, de Paiva CS. Managing Sjogren's Syndrome and non-Sjogren Syndrome dry eye with anti-inflammatory therapy. Clinical Ophthalmology. (2014) 8:1447–58. doi: 10.2147/OPTH.S35685
131. Singer DD, Kennedy J, Wittpenn JR. Topical NSAIDs effect on corneal sensitivity. Cornea. (2015) 34:541–3. doi: 10.1097/ICO.0000000000000309
133. Hayasaka Y, Hayasaka S, Zhang XY, Nagaki Y. Effects of topical corticosteroids and nonsteroidal anti-inflammatory drugs on prostaglandin e2-induced aqueous flare elevation in pigmented rabbits. Ophthalmic Res. (2003) 35:341–4. doi: 10.1159/000074074
134. Walters TR, Goldberg DF, Peace JH, Gow JA. Bromfenac Ophthalmic Solution 0.07% Once Daily Study G. Bromfenac ophthalmic solution 007% dosed once daily for cataract surgery: results of 2 randomized controlled trials. Ophthalmology. (2014) 121:25–33. doi: 10.1016/j.ophtha.2013.07.006
135. Jones L, Downie LE, Korb D, Benitez-Del-Castillo JM, Dana R, Deng SX, et al. TFOS DEWS II Management and Therapy Report. Ocular Surface. (2017) 15:575–628. doi: 10.1016/j.jtos.2017.05.006
136. Pinto-Fraga J, Lopez-Miguel A, Gonzalez-Garcia MJ, Fernandez I, Lopez-de-la-Rosa A, Enriquez-de-Salamanca A, et al. Topical fluorometholone protects the ocular surface of dry eye patients from desiccating stress: a randomized controlled clinical trial. Ophthalmology. (2016) 123:141–53. doi: 10.1016/j.ophtha.2015.09.029
137. Beckman K, Katz J, Majmudar P, Rostov A. Loteprednol etabonate for the treatment of dry eye disease. J Ocul Pharmacol Ther. (2020) 36:497–511. doi: 10.1089/jop.2020.0014
139. Korenfeld M, Nichols KK, Goldberg D, Evans D, Sall K, Foulks G, et al. Safety of KPI-121 ophthalmic suspension 025% in patients with dry eye disease: a pooled analysis of 4 multicenter, randomized, vehicle-controlled studies. Cornea. (2021) 40:564–70. doi: 10.1097/ICO.0000000000002452
140. Zhang XY, Zhao L, Deng SJ, Sun XG, Wang NL. Dry eye syndrome in patients with diabetes mellitus: prevalence, etiology, and clinical characteristics. J Ophthalmol. (2016) 2016. doi: 10.1155/2016/8201053
141. Fajnkuchen F, Barritault D, Giocanti-Auregan A. Evaluation of a new matrix regenerating agent in patients with Sjogren syndrome and superficial ulcerative keratitis resistant to conventional therapy: A report of 3 cases. Medicine (Baltimore). (2018) 97:e9935. doi: 10.1097/MD.0000000000009935
142. Lin BW, Chen MZ, Fan SX, Chuck RS, Zhou SY. Effect of 0025% FK-506 eyedrops on botulinum toxin B-induced mouse dry eye. Invest Ophthalmol Vis Sci. (2014) 56:45–53. doi: 10.1167/iovs.13-12925
143. Mu J, Zeng D, Fan J, Liu M, Yu S, Ding W, et al. Associations between air pollution exposure and daily pediatric outpatient visits for dry eye disease: a time-series study in shenzhen, China. Int J Public Health. (2021) 66:1604235. doi: 10.3389/ijph.2021.1604235
144. Pflugfelder SC, Stern M, Zhang S, Shojaei A. LFA-1/ICAM-1 interaction as a therapeutic target in dry eye disease. J Ocul Pharmacol Ther. (2017) 33:5–12. doi: 10.1089/jop.2016.0105
145. Chan CC, Prokopich CL. Lifitegrast Ophthalmic Solution 50% for Treatment of Dry Eye Disease: Overview of Clinical Trial Program. J Pharm Pharm Sci. (2019) 22:49–56. doi: 10.18433/jpps29895
146. Hovanesian JA, Nichols KK, Jackson M, Katz J, Chan A, Glassberg MB, et al. Real-world experience with lifitegrast ophthalmic solution (Xiidra((R))) in the US and Canada: retrospective study of patient characteristics, treatment patterns, and clinical effectiveness in 600 patients with dry eye disease. Clin Ophthalmol. (2021) 15:1041–54. doi: 10.2147/OPTH.S296510
147. Guimaraes de Souza R, Yu Z, Stern ME, Pflugfelder SC, de Paiva CS. Suppression of Th1-mediated keratoconjunctivitis sicca by lifitegrast. J Ocul Pharmacol Ther. (2018) 34:543–9. doi: 10.1089/jop.2018.0047
148. Sosne G, Qiu P, Ousler GW. 3rd, Dunn SP, Crockford D. Thymosin beta4: a potential novel dry eye therapy. Ann N Y Acad Sci. (2012) 1270:45–50. doi: 10.1111/j.1749-6632.2012.06682.x
149. Sosne G, Ousler GW. Thymosin beta 4 ophthalmic solution for dry eye: a randomized, placebo-controlled, Phase II clinical trial conducted using the controlled adverse environment (CAE) model. Clin Ophthalmol. (2015) 9:877–84. doi: 10.2147/OPTH.S80954
150. Kim CE, Kleinman HK, Sosne G, Ousler GW, Kim K, Kang S, et al. RGN-259 (thymosin beta4) improves clinically important dry eye efficacies in comparison with prescription drugs in a dry eye model. Sci Rep. (2018) 8:10500. doi: 10.1038/s41598-018-28861-5
151. Zhang C, Li K, Yang Z, Wang Y, Si H. The effect of the aqueous extract of bidens pilosa L. on androgen deficiency dry eye in rats. Cell Physiol Biochem. (2016) 39:266–77. doi: 10.1159/000445622
152. Qin G, Zhou Y, Peng J, Zhang Y, Peng X, Peng Q, et al. The effect of buddleja officinalis maxim eye drops on morphology and apoptosis in lacrimal gland of experimental dry eye rabbit model. J Ophthalmol. (2019) 2019:5916243. doi: 10.1155/2019/5916243
153. Peng QH, Yao XL, Wu QL, Tan HY, Zhang JR. Effects of extract of Buddleja officinalis eye drops on androgen receptors of lacrimal gland cells of castrated rats with dry eye. Int J Ophthalmol. (2010) 3:43–8. doi: 10.3736/jcim20100308
154. Yao XL, Peng QH, Chen QL, Tang YH, Zhong Q. Effects of total flavonoid of chrysanthemum on Fas and Fasl expression in lacrimal gland cells of male castrated rabbits with dry eye. Guoji Yanke Zazhi. (2014) 14:1749–54. doi: 10.3980/j.issn.1672-5123.2014.10.03
155. Jian S, Li-Hao C, Qian-Hong L, Jun P, Yu T, Xiao-Lei Y, et al. Inflammatory mechanism of total flavonoids of chrysanthemum and medicated serum on castrated dry eye animal and cell models. Digital Chinese Medicine. (2020) 3:283–96. doi: 10.1016/j.dcmed.2020.12.007
156. Lee H, Kim MY, Ji SY, Kim DH, Kim SY, Hwangbo H, et al. The protective effect of oral application of corni fructus on the disorders of the cornea, conjunctiva, lacrimal gland and retina by topical particulate matter 2.5. Nutrients. (2021) 13:2986. doi: 10.3390/nu13092986
157. Liu Z, Jin M, Li Y, Liu J, Xiao X, Bi H, et al. Efficacy and safety of houttuynia eye drops atomization treatment for meibomian gland dysfunction-related dry eye disease: a randomized, double-blinded, placebo-controlled clinical trial. J Clin Med. (2020) 9:4022. doi: 10.3390/jcm9124022
158. Park B, Lee IS, Hyun SW, Jo K, Lee TG, Kim JS, et al. The protective effect of Polygonum cuspidatum (PCE) aqueous extract in a dry eye model. Nutrients. (2018) 10:1550. doi: 10.3390/nu10101550
159. Park B, Jo K, Lee TG, Hyun SW, Kim JS, Kim CS. Polydatin inhibits NLRP3 inflammasome in dry eye disease by attenuating oxidative stress and inhibiting the NF-kappaB pathway. Nutrients. (2019) 11:2792. doi: 10.3390/nu11112792
160. Park B, Lee TG, Hyun S, Jo K, Lee IS, Jin SK, et al. The effect of Polydatin in dry eye disease in vivo and in vitro. The FASEB J. (2019) 33. doi: 10.1096/fasebj.2019.33.1_supplement.lb388
161. Wang SJ, Wang XH, Dai YY, Ma MH, Rahman K, Nian H, et al. Prunella vulgaris: a comprehensive review of chemical constituents, pharmacological effects and clinical applications. Curr Pharm Design. (2019) 25:359–69. doi: 10.2174/1381612825666190313121608
162. Jarvinen RL, Larmo PS, Setala NL, Yang B, Engblom JR, Viitanen MH, et al. Effects of oral sea buckthorn oil on tear film Fatty acids in individuals with dry eye. Cornea. (2011) 30:1013–9. doi: 10.1097/ICO.0b013e3182035ad9
163. Wang B, Lin L, Ni Q, Su CL. Hippophae rhamnoides Linn. for treatment of diabetes mellitus: A review. J Med Plants Res. (2011) 5:2599–607.
164. Larmo P, Jarvinen R, Laihia J, Loyttyniemi E, Maavirta L, Yang B, et al. Effects of a sea buckthorn oil spray emulsion on dry eye. Cont Lens Anterior Eye. (2019) 42:428–33. doi: 10.1016/j.clae.2018.11.011
165. Kim CS, Jo K, Lee IS, Kim J. Topical application of apricot kernel extract improves dry eye symptoms in a unilateral exorbital lacrimal gland excision mouse. Nutrients. (2016) 8:750. doi: 10.3390/nu8110750
166. Hyun SW, Kim J, Park B, Jo K, Lee TG, Kim JS, et al. Apricot kernel extract and amygdalin inhibit urban particulate matter-induced keratoconjunctivitis sicca. Molecules. (2019) 24:650. doi: 10.3390/molecules24030650
167. Kang SW, Kim KA, Lee CH, Yang SJ, Kang TK, Jung JH, et al. A standardized extract of Rhynchosia volubilis Lour. exerts a protective effect on benzalkonium chloride-induced mouse dry eye model. J Ethnopharmacol. (2018) 215:91–100. doi: 10.1016/j.jep.2017.12.041
168. Liu L, Lao W, Ji QS, Yang ZH, Yu GC, Zhong JX. Lycium barbarum polysaccharides protected human retinal pigment epithelial cells against oxidative stress-induced apoptosis. Int J Ophthalmol. (2015) 8:11–6. doi: 10.3980/j.issn.2222-3959.2015.01.02
169. Chien KJ, Horng CT, Huang YS, Hsieh YH, Wang CJ, Yang JS, et al. Effects of Lycium barbarum (goji berry) on dry eye disease in rats. Mol Med Rep. (2018) 17:809–18. doi: 10.3892/mmr.2017.7947
170. Xu S, Liu S, Yan G. Lycium barbarum exerts protection against glaucoma-like injury via inhibition of MMP-9 signaling in vitro. Med Sci Monit. (2019) 25:9794–800. doi: 10.12659/MSM.919187
171. Zeng Q, Ko CH, Siu WS, Li KK, Wong CW, Han XQ, et al. Inhibitory effect of different Dendrobium species on LPS-induced inflammation in macrophages via suppression of MAPK pathways. Chin J Nat Medicines. (2018) 16:481–9. doi: 10.1016/S1875-5364(18)30083-9
172. Zeng Q, Siu WS, Ko CH, Wong CW, Bik-San Lau C, Wu ZZ, et al. Dendrobium officinale Kimura et Migo Improved Dry Eye Symptoms via Promoting Tear Production in an Experimental Dry Eye Rat Model. Pharmacogn Mag. (2020) 16:294–302.
Keywords: inflammation, signaling pathways, immunomodulatory therapy, traditional Chinese medicine, dry eye disease
Citation: Ling J, Chan BC-L, Tsang MS-M, Gao X, Leung PC, Lam CW-K, Hu J-M and Wong CK (2022) Current Advances in Mechanisms and Treatment of Dry Eye Disease: Toward Anti-inflammatory and Immunomodulatory Therapy and Traditional Chinese Medicine. Front. Med. 8:815075. doi: 10.3389/fmed.2021.815075
Received: 17 November 2021; Accepted: 27 December 2021;
Published: 17 January 2022.
Edited by:
Vito Romano, University of Brescia, ItalyReviewed by:
Lei Zhou, Singapore Eye Research Institute (SERI), SingaporeCopyright © 2022 Ling, Chan, Tsang, Gao, Leung, Lam, Hu and Wong. This is an open-access article distributed under the terms of the Creative Commons Attribution License (CC BY). The use, distribution or reproduction in other forums is permitted, provided the original author(s) and the copyright owner(s) are credited and that the original publication in this journal is cited, in accordance with accepted academic practice. No use, distribution or reproduction is permitted which does not comply with these terms.
*Correspondence: Chun Kwok Wong, Y2std29uZ0BjdWhrLmVkdS5oaw==
Disclaimer: All claims expressed in this article are solely those of the authors and do not necessarily represent those of their affiliated organizations, or those of the publisher, the editors and the reviewers. Any product that may be evaluated in this article or claim that may be made by its manufacturer is not guaranteed or endorsed by the publisher.
Research integrity at Frontiers
Learn more about the work of our research integrity team to safeguard the quality of each article we publish.