- Department of Ophthalmology, Korea University College of Medicine, Seoul, South Korea
Enhanced depth imaging optical coherence tomography (EDI-OCT) and swept-source OCT (SS-OCT) have emerged as essential diagnostic tools in the study and management of various chorioretinal diseases. Evidence from early clinical studies using EDI-OCT and SS-OCT indicates that choroidal dysfunction plays a major role in the pathogenesis of chorioretinal diseases. Measurement of choroidal thickness (CT) has already become a major research and clinical method, and CT is considered as an indicator of choroidal status in a variety of ophthalmic diseases. Recently, CT measurement has also been proposed as a non-invasive marker for the early detection and monitoring of various systemic diseases. Among the several possible CT measurement locations, subfoveal CT has rapidly become a reliable parameter for measuring CT in healthy and diseased eyes. Moreover, recent advancements in OCT technology have enabled faster and wider imaging of the posterior part of the eye, allowing the various changes in CT as measured outside the macula to be shown accordingly. In this review, we first provide an overview of the results of clinical studies that have analyzed the healthy macular choroid and that in various chorioretinal diseases, and then summarize the current understanding of the choroid outside the macula. We also examine the CT profile as an index that encompasses both within and outside of the macula. Furthermore, we describe the clinical applications of ultrawide OCT, which enables visualization of the far periphery, and discuss the prospects for the development of more reliable choroidal parameters that can better reflect the choroid's characteristics.
Introduction
The choroid is a vascular coat that supports the retina to maintain stable blood flow, nutrition, and temperature (1, 2). Choroidal dysfunction contributes to the structural and functional abnormalities of the overlying retinal pigment epithelium and retina, and these relationships have been suggested to be implicated in the pathogenesis of various chorioretinal diseases (3–8). Indocyanine green angiography revealed that abnormal choroidal vascular flow was involved in the development of chorioretinal diseases such as central serous chorioretinopathy (CSC), polypoidal choroidal vasculopathy, or uveitis (4–9). With the advent of spectral domain optical coherence tomography (SD-OCT) technology, a method to measure choroidal thickness (CT) in vivo was introduced in ophthalmology (10, 11). Subsequently, the introduction of enhanced depth imaging (EDI) techniques and swept source optical coherence tomography (SS-OCT) have made it possible to observe topographic variations in CT (12, 13).
Variations in subfoveal CT in various chorioretinal diseases have been more extensively reported in the corresponding pathologic state than in the physiologic state (14), and these variations have been suggested to be related to the onset and progression of these chorioretinal diseases (15, 16). Recently, an association between CT variation and various systemic diseases has also been indicated (16, 17). Consequently, CT measurement has become a major research method, and CT is considered an indicator of choroidal status not only in chorioretinal diseases but also in various systemic diseases (18, 19). Subsequently, subfoveal CT has become a primary parameter to measure CT in normal and diseased eyes (14, 20–22). In addition to the macular choroid, it has been suggested that the peripapillary choroid could also be representative of the choroid of individuals (23–25). In early studies, variation in nasal peripapillary was reported in chorioretinal diseases, and measurement of the peripapillary CT showed the potential to serve as another reference point for CT measurements (26–28). Advancements in scan speeds and optics of OCT systems provided simple and easy opportunities to image a wider range of posterior segment of the eye (12, 14, 18). Several recent studies found CT variations in normal and diseased eyes both in the posterior pole and the peripheral area (29–31). In addition to CT measurement at a single representative point, the use of combinations of CT measurements at different points has been suggested as another reliable strategy for characterizing the human choroid both with and without diseases (32, 33).
In this study, we reviewed how the CT profile has progressively evolved into one of the most representative biomarkers for characterizing the human choroid in recent studies using OCT. To understand how CT measurement has become an important non-invasive indicator, we first reviewed the process of obtaining measurement reliability in healthy subjects, followed by an overview of the geographic characteristics of CT in the macula. The characteristics and variations of the CT outside the macula, particularly the peripapillary CT, were described in various chorioretinal and systemic diseases. We also presented recent clinical studies on CT measurement up to the periphery using wide-field OCT and discussed the need for and application of a novel parameter based on choroidal thickness.
Development of Choroidal Thickness Measurement
Early studies on CT were conducted using ultrasonography (34, 35). In 1979, Coleman and Lizzi (34) estimated CT to be 420 μm at the posterior pole in vivo and found even thicker regions outside the macula. However, it was not easy to precisely determine the specific topographic location of the CT measurement point with ultrasonography. Advancement of OCT technology has enabled the collection of detailed topographic volume data from the posterior pole. OCT also enables imaging of the histologic structure of the fovea in vivo, which makes it possible to measure retinal thickness in the subfoveal and parafoveal areas.
In 2008, the introduction of EDI method using commercially available SD-OCT by Spaide et al. made it possible to reasonably visualize the deeper layer of the choroid and choroidoscleral junction (12). In early human studies using SD-OCT, reliable CT measurements could be obtained for both healthy and diseased eyes (10, 11). Branchini et al. (36) showed good reproducibility of CT measurements in healthy individuals using three different SD-OCT systems. Furthermore, SS-OCT systems using longer wavelengths added more detailed information about the outer choroidal boundary than SD-OCT (37–39). For reliable CT measurements, it is important to accurately identify the choroid-scleral junction as an outer boundary. In some studies, SS-OCT permitted accurate identification of the choroid-scleral junction in 100% of normal eyes (37, 38). Lee et al. (39) showed that SD-OCT devices had lower reliability than SS-OCT devices in eyes with CT ≥ 400 μm and subfoveal active lesions. Despite these differences, both SS-OCT and SD-OCT with the EDI technique demonstrated good reproducibility in terms of CT measurement, and both systems have become standard methods for measuring CT, especially subfoveal CT (14, 22, 39).
Choroidal Thickness Measurement at the Macula
From Subfovea to Perifovea
Extensive studies measuring CT focused on the choroid at the macula (14), especially the subfoveal choroid, where the overlying retina is most important in preserving visual function. We reviewed the published literature on CT using OCT in humans, with a focus on the topographic location of CT measurements. A literature search in the PubMed database (RRID:SCR_004846) in August 2021 using the terms “choroidal thickness” and “optical coherence tomography” with no restrictions identified a total of 2,734 citations published with the cut-off publication date of July 31, 2021. In Table 1, we summarize the results of the articles that specifically compare various locations of CT measurements and various chorioretinal diseases between different publication time periods. We also used combinations of relevant keywords, MeSH terms, and Boolean operators (AND and OR) to conduct literature searches. For example, in Table 1, we used the search query; subfoveal OR macular AND age related macular degeneration AND “choroidal thickness” AND optical coherence tomography to present the number of studies on subfoveal CT measurement using OCT in age-related macular degeneration.
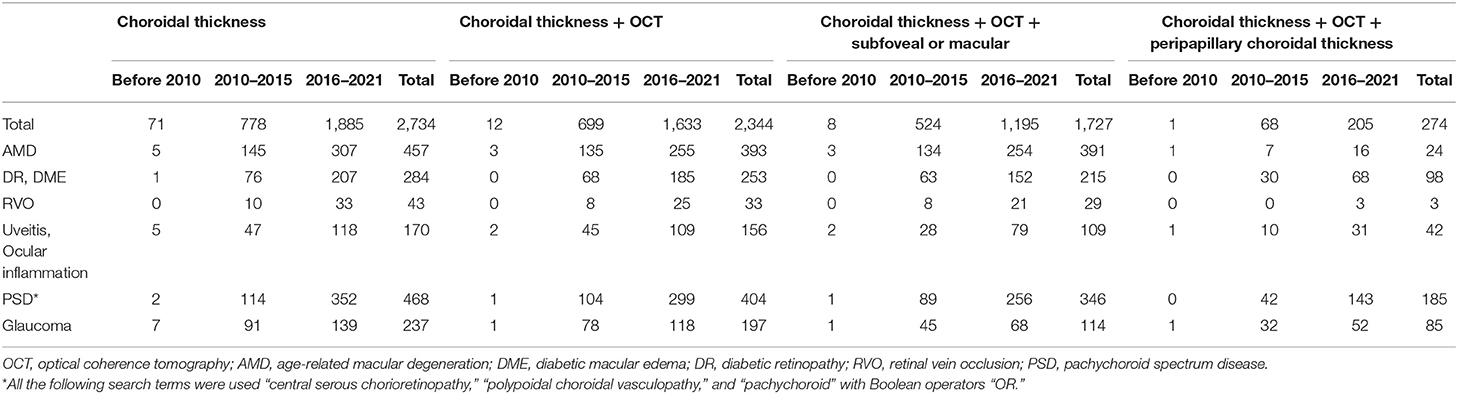
Table 1. The number of citations on choroidal thickness measurements depending on the different search terms and the date of publication in PubMed database.
To investigate geographic CT characteristics within the macular area, other locations and the subfoveal choroid on the horizontal meridian of the macula were employed for CT measurement using SD-OCT in horizontal raster scans (Figure 1). Most studies reported that within the macula, the subfoveal choroid was the thickest and decreased in thickness as the distance from the fovea increased in either direction. Manjunath et al. (10) performed high-definition raster scanning using EDI SD-OCT with frame enhancement software to measure the CT at the macula in eyes without chorioretinal disease. In this study, wherein measurements were performed at the subfovea and at 500-μm intervals from the fovea to 2,500 μm temporal and nasal to the fovea, the choroid was found to have a topographic CT pattern, with the nasal perifovea being the thinnest, the subfovea being the thickest, and the temporal perifovea being again on the thinner side. Chen et al. (40) also found that the choroid was thickest at the subfovea and thicker at the temporal perifovea than at the nasal perifovea in normal eyes. Attempts to measure CT at the macula have extended to the eyes of patients with various ocular and systemic diseases. Regatieri et al. (11) measured CT at the macula in patients with and without diabetes using the same measurement protocol and confirmed that the variation pattern of CT progressively decreased with increasing distance from the fovea and it became markedly thinner on the nasal side than on the temporal side of the fovea. In eyes without high myopia, Ikuno et al. (41) also reported that subfoveal CT was significantly greater than nasal and temporal CT using SS-OCT. However, in a study of highly myopic patients with a spherical equivalent refractive error of six diopters or more, Fujiwara et al. (20) found that the temporal perifoveal choroid was thickest, and that CT progressively decreased underneath the fovea and nasal perifoveal choroid along a 6-mm horizontal scan line. Ho et al. (42) also reported comparable results in terms of CT variations in myopic eyes. These results highlight that even in the absence of retinal disease, variations in CT may exist secondary to chorioscleral structural changes such as axial elongation of the eyeball and stretching of the choroid in myopic eyes. Therefore, the refractive error or axial length must be considered in studies of choroidal variations.
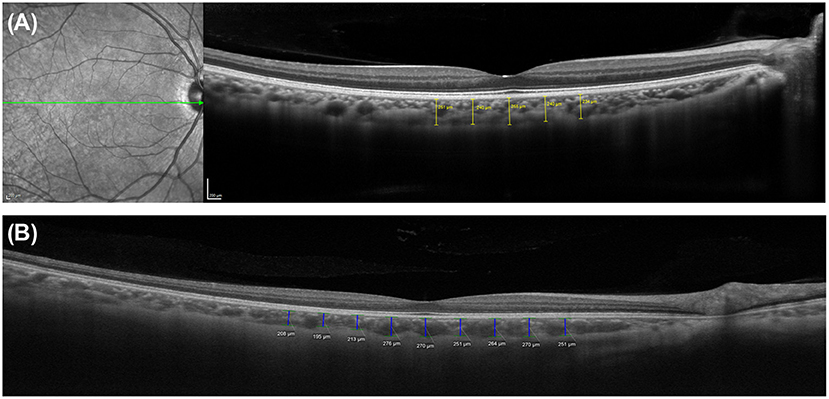
Figure 1. Measurements of choroidal thickness (CT) within the macula. A horizontal line scan image centered on the fovea obtained using (A) spectral domain optical coherence tomography with enhanced depth imaging technique (Spectralis OCT2; Heidelberg Engineering, Heidelberg, Germany) and (B) swept-source optical coherence tomography system (PLEX Elite 9000; Carl Zeiss Meditec, Inc, Dublin, California, USA). The choroidoscleral junction and the detail of choroid is clearly visible in both images. CT was determined by measuring the vertical distance from the retinal pigment epithelium/Bruch's membrane complex to the choroidoscleral junction. Subfoveal and macular CT was measured manually by caliper tool at the subfovea and at 500-μm intervals up to (A) 1,000 μm and (B) 2,000 μm temporal and nasal to the fovea, respectively.
Measurement at Superior and Inferior Perifovea
In addition to the horizontal meridian based on the foveal center, various attempts have been made to widen the measurement in other directions at the macula. Several studies have measured CT along the vertical meridian superior and inferior to the fovea (40, 41). In a study using SS-OCT, Ikuno et al. (41) reported that the superior choroid was significantly thicker than the inferior choroid. Using EDI SD-OCT choroidal imaging, Chen et al. (40) also reported significant topographic variations in CT within the macular region. They found that the choroid was thickest at the subfovea, followed by that in the superior perifovea, and then the choroid was least thick in the in inferior perifovea. They also pointed out that there is no consensus on what a “normal” CT profile of the macula is in the current literature. As knowledge of the regional variations in CT accumulate, it is possible to improve the understanding of the choroidal profile of the macula in healthy subjects and its variations in patients with various diseases.
Three-Dimensional Thickness Map
Since EDI-OCT method requires the averaging of many B-scans to acquire good choroidal images, fewer averaged raster scans can be obtained using EDI-OCT than by using SS-OCT (12, 43). Hirata et al. (43) indicate that CT measurements through only a few scans are more susceptible to ill-defined inner choroid-scleral borders, as well as focal thickening or thinning of the choroid. Using SS-OCT, they produced a CT map of the macular area using a three-dimensional (3D) volumetric scan protocol in healthy eyes and applied an early treatment diabetic retinopathy study (ETDRS) grid (Figure 2). They showed that CT in the nasal quadrant was lower than that in the other quadrants. In addition, the choroid was thicker in the outer superior field than in the outer inferior field of the ETDRS grid. They also showed that the subfoveal CT was similar to that in the superior temporal quadrant, however, it was greater than that in the inferior nasal quadrant. Heirani et al. (44) demonstrated that the choroid was thickest at 1 mm superior to the fovea and thinnest at 3 mm nasal to the fovea. Recently, using SS-OCT system with a 12 × 12-mm 3D volume scan centered on the fovea, Breher et al. (45) showed that CT gradually decreased with an increasing distance from the fovea. A topographic pattern with thicker choroids was observed in the order of upper, temporal, lower, and nasal side of the choroid. These studies generally found that the superior and temporal choroid was thicker than the inferior and nasal choroid, but they also revealed that choroidal geography varied greatly among healthy subjects.
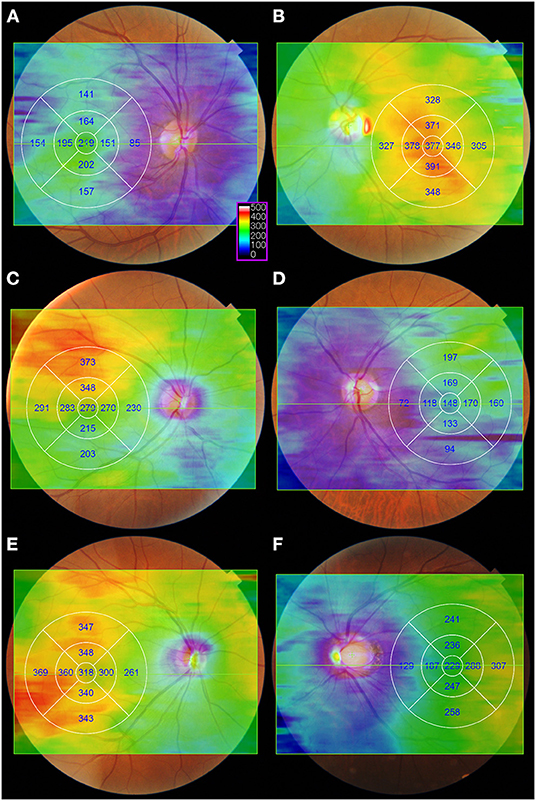
Figure 2. Examples of the color-coded choroidal thickness (CT) map in healthy eyes. The CT maps were generated automatically from a 12 × 9 mm three-dimensional volume scan consisting of a 256 B-scan with 512 A-scans per B-scan. Images were obtained using swept-source optical coherence tomography system (DRI OCT Triton, software version 10.17.003.01; Topcon Corp., Tokyo, Japan). A color-coded CT map and early treatment diabetic retinopathy study (ETDRS) grid were superimposed on the fundus photography using the built-in software. After the automatic layer segmentation, a color-coded CT map was obtained with the Bruch's membrane and choroidoscleral interface selected as the inner and outer limits of segmentation lines, respectively. The color scale (purple box) shows the choroidal thickness in microns. On a color-coded CT map, the warmer colors represent the thicker areas, while the cooler colors represent the thinner regions. (A,B) Representative cases of eyes with the greatest CT at the fovea. (C,D) Representative cases of eyes with the greatest CT it the superotemporal region. (E) Representative case of eyes with the greatest CT it the inferotemporal region. (F) Representative cases of highly myopic eyes with the greatest CT in the temporal region.
Furthermore, not all OCT systems provide a volumetric CT map with a built-in software, and it is difficult to obtain a reliable CT map automatically in diseased eyes due to segmentation errors. The newer image processing techniques, such as variety automatic segmentation and edge detection algorithms, along with the increased application of artificial intelligence, have allowed ophthalmologists to access a vast amount of 3D volumetric OCT data, which would otherwise be time-consuming for manual analysis (46, 47). These advances have enabled more accurate and reliable CT measurements over a wider range, rather than just one part of the macula.
Limitation of Measurement at Macula
The normal range of subfoveal CT has still not been definitively established (14, 48). Additionally, many factors, including age (20, 41, 43, 44, 49), refractive error (20, 41, 44, 49), and axial length (20, 40, 43, 44), have been postulated to affect subfoveal CT, and the physiologic diurnal variations (50–56) in CT have also been described. Moreover, several studies observe that CT changes even after light exposure and daily activities such as physical exertion, drinking water, and smoking (13, 56–58). As a result, evaluating a patient based only on the basis of the absolute value of CT measured at one site and one time point has limitations, and confounding factors such as age, axial length, refractive error, and diurnal variation must be considered. To compensate for the limitations of the subfoveal CT, an increase in the number of ophthalmology studies using CT measurements has led to the measurement of CT not only at the subfovea, but also at various locations in the posterior part of the eye.
Choroidal Thickness Measurement Beyond the Macula
CT measurement is not limited to the macular areas (42, 59–62). When literature searches were conducted in August 2021 using the PubMed database (RRID:SCR_004846) with no restrictions and the search terms “subfoveal choroidal thickness” and “OCT,” a total of 1,727 citations were identified accordingly. When the terms “peripapillary choroidal thickness” and “OCT” were used, a total of 274 citations were identified further. Spaide (63) was the first to measure temporal peripapillary CT in eyes with age-related choroidal atrophy in his early study. Oh et al. (64) proposed a method to measure peripapillary CT using retinal nerve fiber layer scanning in patients with glaucoma (Figure 3). It is known that both age and myopia are correlated with variations in peripapillary CT (63–67), and many studies have been conducted to analyze these variations in normal subjects and glaucoma patients (62, 68). Most of these studies focus on determining the relationship between peripapillary CT and optic nerve damage, such as glaucoma and optic neuropathy (68–71).
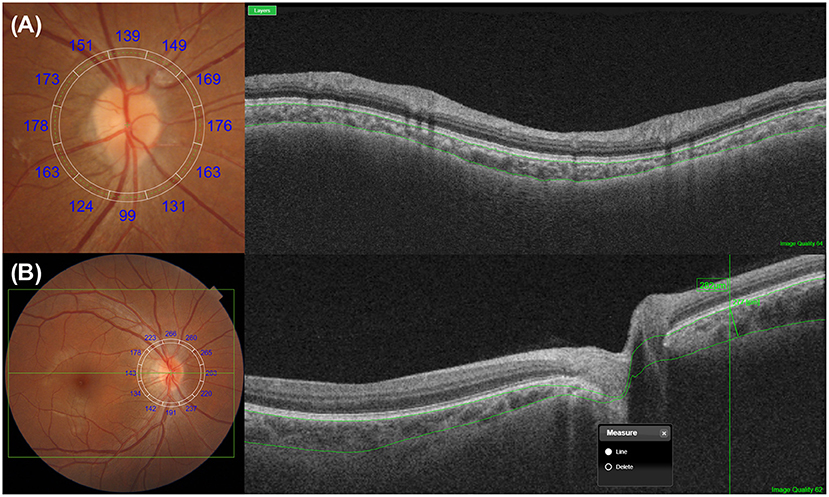
Figure 3. Representative methods for the measurements of peripapillary choroidal thickness (CT). (A) Peripapillary CT can be measured over 360° along a 3.4-mm circular grid, which is used for retinal nerve fiber layer analysis. Depending on the devices, the upper and lower segmentation lines are either automatically or manually selected as the Bruch's membrane and the choroidoscleral junction, respectively. After segmentation, peripapillary CT is determined automatically using built-in manufacturer's software, either by the sectorial or overall average. (B) Peripapillary CT can be measured manually at a point of interest with a similar method for macular CT measurement. A circular RNFL grid centered on the optic disc can be used to improve the consistency of the measurement position.
Peripapillary Choroidal Thickness
At Chorioretinal Diseases
Several investigators have investigated peripapillary CT in various diseases and have attempted to determine its significance in the development of chorioretinal diseases (Table 2) (26, 27, 32, 33, 62, 65, 66, 72–82). The choroid covers the posterior two-thirds of the eye, whereas the subfoveal choroid is only one part of the choroid. Many chorioretinal diseases can either affect regions outside the macula or they may affect both regions within the macula and those outside of it (73, 83–86). Peripapillary CT measurement has been proposed as one of the useful methods for assessing CT outside of the macula. Yun et al. (26) raised the question of whether measuring only subfoveal or parafoveal CT could be representative of the choroid as a whole. CSC is well-known to be associated with subfoveal choroidal thickening on OCT (Figure 4) (15, 87). They measured nasal peripapillary CT outside the macula, as well as subfoveal CT in patients with CSC. They found that both nasal peripapillary and subfoveal CT were thicker in the affected eyes and fellow eyes of the CSC patient group than in the normal controls.
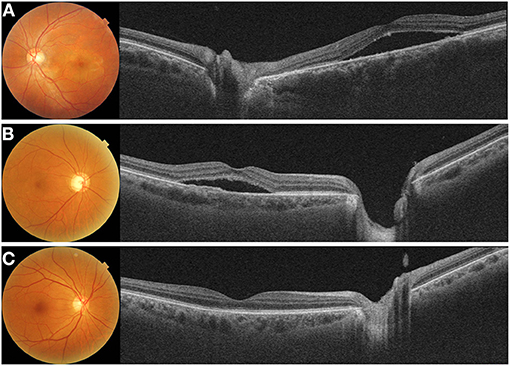
Figure 4. Representative cases with (A) acute central chorioretinopathy (CSC), (B) chronic CSC, and (C) resolved CSC. (A) A 38-year-old male with acute CSC showed diffuse thickening of both the macular and peripapillary choroid with dilated large choroidal vessels on a cross-sectional swept-source optical coherence tomography (SS-OCT) image. (B) A 69-year-old male with chronic CSC had increased subfoveal choroidal thickness (CT) and showed shallow irregular pigment epithelial detachment on and SS-OCT image. Peripapillary choroid was also thickened with dilated pachyvessels. (C) A 69-year-old male with resolved CSC showed diffuse thickening of the macular choroid. But, this patient had a relatively thinner peripapillary choroid.
A trial to measure CT outside the macula has been extended to age-related macular degeneration (Figure 5). Switzer et al. (22) found that eyes with reduced subfoveal CT were more likely to have reticular pseudodrusen. Yun et al. (27) measured the CT outside the macular area and showed that peripapillary CT outside the macula was lower in eyes with reticular pseudodrusen than in those eyes without reticular pseudodrusen, in addition to that in the macula (Figure 5B). These findings were confirmed by Nam et al. (72), who reported that peripapillary CT in patients with non-exudative age-related macular degeneration showed variations according to the accompanying drusen type. Kim et al. (32) also showed that the peripapillary CT of eyes with pachydrusen was higher than that of eyes with soft drusen (Figure 5C). Kim et al. (75) also showed that nasal peripapillary CT outside the macula was different between pachychoroid neovasculopathy and classic exudative age-related macular degeneration. These studies found similar trends in the alteration of the peripapillary and subfoveal CTs in each chorioretinal disease. These findings suggest that peripapillary CT can be useful in disorders where measuring subfoveal CT is difficult, such as in patients with CSC or exudative AMD.
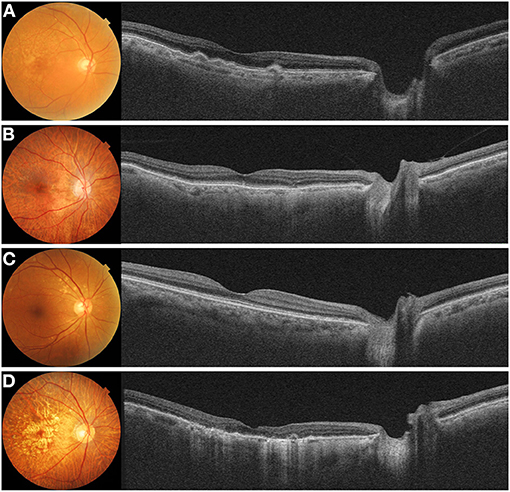
Figure 5. Color fundus photograph and swept-source optical coherence tomography (SS-OCT) image in eyes with (A) soft drusen, (B) reticular pseudodrusen, (C) pachydrusen, and (D) geographic atrophy. (A) Fundus photograph of an 85-year-old male showed multiple soft drusen in the macular. SS-OCT image showed several dome-shaped retinal pigment epithelium (RPE) elevations with medium internal reflectivity and diffuse choroidal thinning in the macula and peripapillary area. (B) Fundus photograph of an 83-year-old female showed numerous reticular pseudodrusen in the macula. A cross-sectional SS-OCT image demonstrated multiple hyperreflective material lies above the RPE. Both the macular and peripapillary choroid demonstrated generalized thinning. (C) Fundus photograph of a 65-year-old female showed pachydrusen with various sizes scattered along the vascular arcade. The choroid in the macular and peripapillary regions was not thinned significantly on SS-OCT image. (D) Fundus photograph of an 85-year-old female demonstrated multiple confluent well-demarcated atrophic lesions in the macula. A cross-sectional SS-OCT image showed thinning of the outer retina and hypertransmission. A marked thinning of the macula and peripapillary choroid is observed.
In addition, several studies have reported changes in peripapillary CT that differ from subfoveal CT. Phasukkijwatana et al. (73) reported that peripapillary choroidal syndrome is caused by a disproportionate thickening of the peripapillary choroid relative to the macular choroid. Recently, Kim et al. (76) reported that peripapillary CT increased in patients with acute and chronic CSC; however, this was not observed in patients with resolved CSC (Figure 4). As further research proceeds, it is expected that peripapillary CT will be more helpful.
The usefulness of peripapillary CT measurements extends to retinal vascular diseases. Kang et al. (77) demonstrated that peripapillary CT decreased significantly over 12 months in eyes with branch retinal vein occlusion (BRVO) and in fellow eyes in patients with unilateral BRVO. Lee et al. (78) also analyzed the peripapillary CT in eight sectors and showed that it significantly decreased in all sectors over 6 months in eyes with BRVO. Sirakaya and Kucuk (79) showed that the average, superior, and inferior peripapillary CT in both eyes of patients with unilateral BRVO were significantly lower and nasal peripapillary CT in the affected eyes was lower than that in the control eyes. Kang et al. (80) found that the mean peripapillary CT in patients with BRVO and normal-tension glaucoma was significantly lower in eyes with focal laminar cribrosa defects than in those without it. In a recent study, Cetin et al. (81) extended the measurement of peripapillary CT in patients with retinitis pigmentosa. Balci and Turan-Vural measured peripapillary CT in eyes with ocular sarcoidosis and glaucoma (82). These studies highlighted the application of CT measurements outside the macula in a variety of retinal disorders. Although the pathophysiological and clinical significance of these CT regional variations observed outside the macula has not yet been established, it is suggested that analyzing a wider field of view could be useful for investigating CT abnormalities in diseased eyes (Figure 6).
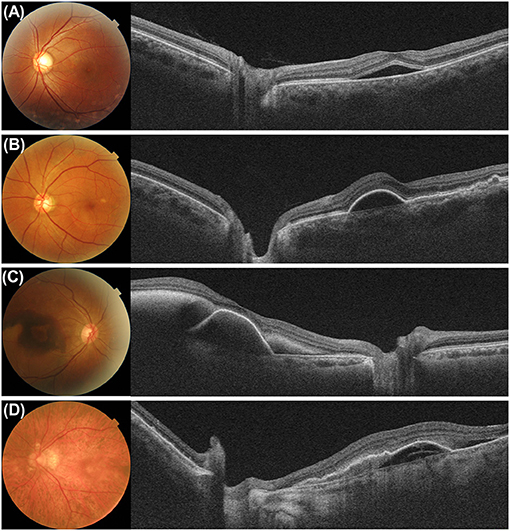
Figure 6. Examples of eyes with active macular disease of (A) central chorioretinopathy, (B) pachychoroid neovasculopathy, (C) polypoidal choroidal neovasculopathy, and (D) exudative age-related macular degeneration. Because the outer boundary of the subfoveal choroid is not readily visible in cases of active diseases such as accumulation of subretinal fluid, blood, and pigment epithelial detachment in the macula, measuring the subfoveal choroidal thickness are not always possible. Even in these cases, the peripapillary choroidal thickness can be measured.
At Systemic Diseases
The choroid is composed mostly of a dense network of blood vessels with the highest blood flow per unit weight in the human body (2). This highly vascularized nature of the choroid, which can be assessed by non-invasive imaging, has drawn attention to the choroid in a variety of systemic diseases, such as the metabolic, inflammatory, and the systemic vascular disease (88). Peripapillary CT measurements have also been attempted in patients with systemic illnesses (24, 89–93) such as diabetes (23, 94–97), thyroid diseases (98, 99), and pulmonary diseases (25, 100) (Table 3). Yazgan et al. (94) showed that the macular and peripapillary choroid was significantly thicker in pre-diabetic patients than in healthy controls at all 15 measuring points. Vujosevic et al. (95) showed that both macular and peripapillary CT decreased with advancement of the diabetic retinopathy stage. Peripapillary CT was lower in type 2 diabetic patients with impaired renal function in a study by Liu et al. (23), and it was positively correlated with the estimated glomerular filtration rate. Loureiro et al. (89) reported that peripapillary CT in the temporal and inferotemporal sectors was significantly lower in patients with metabolic syndrome than in normal controls. Changes in CT not only in the macula but also outside the macula support the hypothesis that choroidopathy occurs in patients with systemic vascular diseases.
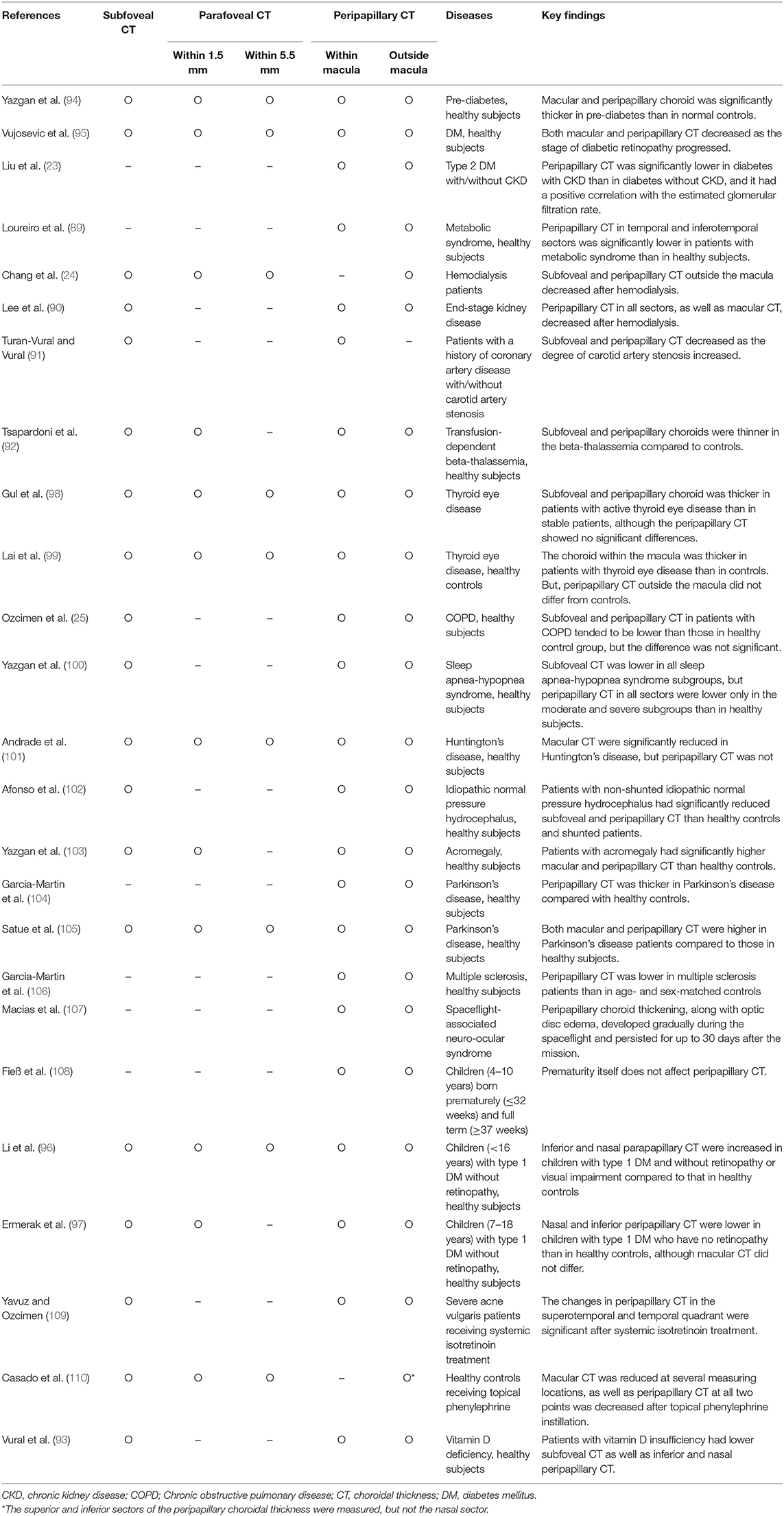
Table 3. Findings of peripapillary choroidal thickness measurement in systemic disease or after medication administration.
Correlations between hemodynamic changes and CT variations have also been suggested in literature. Chang et al. (24) showed that peripapillary CT in all sectors, as well as macular CT, decreased after hemodialysis. Lee et al. (90) also showed a decrease in macular and peripapillary CT shortly after hemodialysis, however, the decrease was not significantly correlated with changes in mean ocular perfusion pressure. Turan-Vural et al. (91) studied the relationship between CT variation and ocular perfusion in patients with carotid artery stenosis. Their results showed that thinner subfoveal and peripapillary CT were associated with worsening of the degree of carotid artery stenosis. Tsapardoni et al. (92) studied choroidal variation in patients with transfusion-dependent beta-thalassemia and reported that subfoveal and peripapillary CT were lower in patients with beta-thalassemia than in healthy controls. They suggested that chronic anemia and underlying choroidal hemodynamic changes possibly result in choroidal thinning in patients with beta-thalassemia. These studies thus suggested that subfoveal and peripapillary CT measurements could indirectly and non-invasively reflect ocular and systemic perfusion status.
The association between thyroid eye diseases and changes in the choroid has been studied with respect to autoimmune inflammatory disease. Gul et al. (98) measured macular and peripapillary CT in patients with thyroid eye diseases associated with Graves' disease, nodular goiter, and Hashimoto's thyroiditis and found that the subfoveal choroid was thicker in patients with active thyroid eye disease than in those with a stable state. Peripapillary CT tended to be higher in the active group, however, the difference was not statistically significant. Lai et al. (99) reported that the choroid at the subfovea and at the macular area within 2 mm from the foveal center was thicker in patients with thyroid-associated orbitopathy than in controls, however, the peripapillary choroid did not differ from that in normal controls. Choroid thickness has been proposed as an inflammatory marker for a variety of systemic inflammatory diseases, but little is known about its clinical utility.
Regarding peripapillary CT in patients with pulmonary diseases, Ozcimen et al. (25) showed that subfoveal and peripapillary CT in patients with chronic obstructive pulmonary disease tended to be lower than those in the healthy control group, however, the difference was not statistically significant. Yazgan et al. (100) measured subfoveal and peripapillary CT in patients with sleep apnea-hypopnea syndrome and normal controls and found that subfoveal CT was lower in all sleep apnea-hypopnea syndrome subgroups than in the control group. However, peripapillary CT in all sectors was lower only in the moderate and severe subgroups than in the healthy controls.
At Neurodegenerative Diseases
Anatomically and developmentally, the retina is known as an extension of the CNS (111); therefore, studies using OCT have been actively conducted in many neurodegenerative diseases (19). This close relationship highlights the optic nerve, and meaningful quantitative alterations in retinal thickness and volume have been demonstrated in patients with cognitive impairment and neurodegenerative diseases (19, 112). In addition, a growing number of studies have analyzed peripapillary CT in neurologic diseases (101–106). Andrade et al. (101) demonstrated that macular CT was significantly reduced in patients with Huntington's disease compared to that in controls. However, no differences were observed in the peripapillary choroidal measurements. Afonso et al. (102) showed that patients with non-shunted idiopathic normal pressure hydrocephalus had significantly reduced subfoveal and peripapillary CT than healthy controls and shunted patients. However, the subfoveal and peripapillary CT did not differ significantly between shunted patients and healthy controls. Yazgan et al. (103) reported that patients with acromegaly had significantly higher macular and peripapillary CT than healthy controls. Garcia-Martin et al. (104) reported that the peripapillary choroid was significantly thicker in patients with Parkinson's disease than in age- and sex-matched controls. Satue et al. (105) also showed that both macular and peripapillary CT were higher in patients with Parkinson's disease than in healthy controls. Garcia-Martin et al. (106) reported that peripapillary CT was lower in patients with multiple sclerosis than in age- and sex-matched controls. Macias et al. (107) studied the choroidal changes in astronauts with space-flight-associated neuro-ocular syndrome and found that peripapillary choroid thickening, along with disc edema, developed gradually during spaceflight and persisted for up to 30 days after the mission. Although CT within and outside the macula has been studied in various neurodegenerative diseases, further studies are needed before CT can be used as a marker for the early detection and progression of these diseases.
At Other Conditions
Children have also been subjected to peripapillary CT measurements. Fieß et al. (108) reported that premature birth itself does not affect peripapillary CT, however they found that infants born small for their gestational age had peripapillary choroidal thinning when compared to those infants born at the appropriate gestational age. Li et al. (96) measured macular and parapapillary CT in children with diabetes and reported that inferior and nasal parapapillary CT were increased in children with type 1 diabetes and without retinopathy or visual impairment as compared to healthy controls. However, Ermerak et al. (97) also showed that nasal and inferior peripapillary CT were lower in children with type 1 diabetes who had no retinopathy than in normal controls, although macular CT did not differ between the groups.
In addition to systemic diseases, peripapillary CT measurements have also been used in studies on the effects of drugs or nutritional deficiencies. Yavuz and Ozcimen (109) reported that there were significant changes in peripapillary CT in the superotemporal and temporal quadrants after systemic isotretinoin treatment for severe acne vulgaris. Casado et al. (110) investigated the effect of topical phenylephrine instillation on macular and peripapillary CT. Peripapillary CT was significantly reduced after phenylephrine instillation. In a horizontal macular OCT scan, CT was reduced at the subfovea and at 500, 1,000, and 1,500 μm temporal to the fovea, but not inferior to the fovea. CT at the subfovea and only the area within 500 μm from the fovea was reduced in a vertical scan. Vural et al. (93) reported that patients with vitamin D deficiency had lower subfoveal CT, as well as inferior and nasal peripapillary CT.
These findings in current studies of ocular and systemic diseases indicate that both macular and peripapillary CT have been employed to account for variations in CT. This could suggest that researchers are expanding the number of landmarks used for CT measurements.
Choroidal Thickness Measurement to Far Periphery
Several efforts to evaluate CT in the peripheral retina have been made in addition to posterior pole and peripapillary area (113, 114). Development of faster OCT systems and wide-viewing technologies have enabled easier scanning of the far periphery (Figure 7) (31, 115–117). Mohler et al. (118) described choroidal images and CT maps in normal and diseased eyes over an ~60° field of view using prototype SS-OCT. However, this method using a special lens and device is difficult to apply in a general clinical environment. Using commercially available SD-OCT devices, Rasheed et al. (119) analyzed CT up to the mid-equator with manual montage and showed that the choroid outside the macula was significantly thinner in all quadrants than the choroid within the macula, with the greatest reduction observed in the inferior quadrant. Hoseini-Yazdi et al. (120) used a wide-field lens module and EDI-OCT to assess the CT throughout a 55 × 45° region centered on the fovea and found that the choroid thinned substantially toward the periphery, with minimum and maximum peripheral thinning superiorly and nasally, respectively. They also found that myopia-related choroidal thinning was more prominent in the macular than in the extra-macular areas. In addition to normal eyes, Singh et al. (29) conducted wide-field choroidal vessel analysis in CSC and fellow eyes and measured CT in extremes of gazes in all quadrants. They reported that the absolute value of CT was higher in CSC eyes than in normal eyes, however the topographical variation was similar to that of the normal eyes. In studies using SD-OCT, it is difficult to obtain multiple images at consecutive and parallel positions for manual montage, and image analysis requires substantial effort and time. Additionally, this method has the disadvantage of being difficult to acquire images from the same location in a longitudinal study.
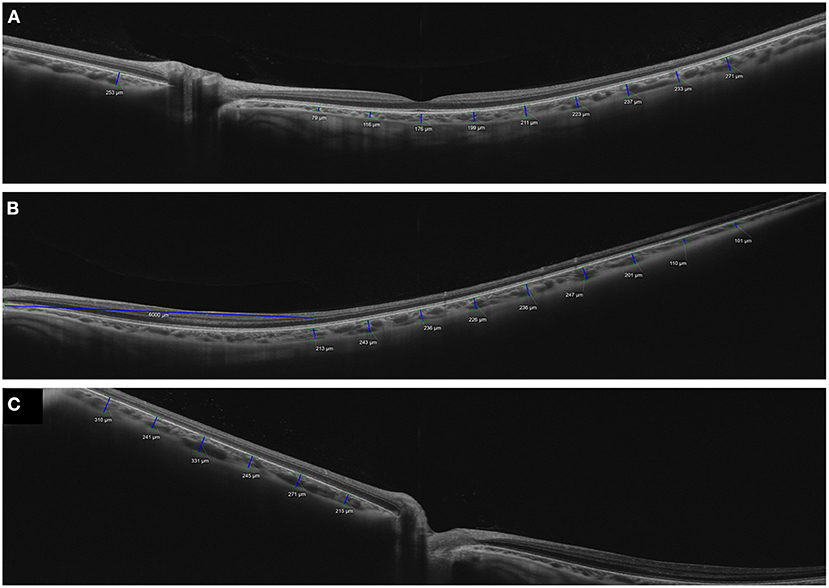
Figure 7. Measurements of choroidal thickness in the macular and peripheral areas using wide-field optical coherence tomography. A wide-field 16-mm high-definition horizontal line scans image of a healthy volunteer was acquired using swept-source optical coherence tomography system (PLEX Elite 9000; Carl Zeiss Meditec, Inc, Dublin, California, USA). (A) A horizontal line scans passing through the fovea was acquired in primary gaze. (B) Temporal and (C) nasal horizontal line scans were obtained in temporal and nasal gazes. Choroidal thicknesses were measured using a caliper tool at 1,000-μm intervals in the macular area and the peripheral areas in each direction. Irregular distribution of large choroidal vessels was observed frequently in the peripheral areas (B,C).
Using SS-OCT, Breher et al. (121) generated a CT map of 13 extended wide-field ETDRS sectors with radii of 0.5, 1.5, 3, and 6 mm in myopic eyes and showed a topographic variation of CT with thicker choroid in the central, superior, and temporal regions. They also suggested that the elongation of the myopic eye influenced the CT. Kim et al. (30) used SS-OCT and a wide-field 16 mm line scan to measure the CT in the macular, nasal peripapillary, and peripheral areas. Although CT demonstrated bilateral symmetry in both the central and peripheral regions, the degree of CT asymmetry increased toward the nasal peripapillary and peripheral regions. Their results suggested that the nasal peripapillary and peripheral choroid had greater anatomic variation than the macula. In another study, Lim et al. (31) compared CT between the pachychoroid and normo-choroid groups using the same imaging protocol. They showed that the pachychoroid group had thicker choroid in both the macular and peripheral areas than the normo-choroid group, however the difference was smaller in the peripheral area. In their study, regional variations in the distribution of pachyvessels were observed more frequently in the nasal peripapillary and peripheral areas than in the macula (Figure 7). They speculated that these variations caused the topographic CT pattern of the nasal peripapillary and peripheral areas to differ from that of the subfovea, implying the fact that only the subfoveal CT could not represent the entire choroid of the eye. Wide-field OCT studies are still in their infancy, and more research on the characteristics and importance of the peripheral choroid is required. Peripheral distortion in OCT images is an important factor to consider when conducting and analyzing wide-field OCT research.
Discussion
Emerging Issues in Choroidal Thickness Measurements
The introduction of OCT has revolutionized the current practice of ophthalmology, and OCT imaging is now a standard method for investigating macular diseases. OCT devices using a faster and wider range of technologies have enabled the measurement of CT at various locations in the posterior eye. Similar to its contribution to the evaluation of macular thickness, wider and deeper choroidal imaging using the current OCT systems is now expected to open new avenues in CT measurement.
Most studies on CT measurement have focused on the measurement of subfoveal CT; however, CT measurement should not be limited to the macula for several reasons. First, most choroidal diseases are not confined to the macular area. Second, the anatomy and physiology of the choroid differ greatly from those of the retina (2). Although both the choroid and retina receive blood supply from the ophthalmic artery, the afferent and efferent vasculature are separated and located in different areas (1, 2, 122, 123). In the vascular system of the retina, the entry and exit points of vascular flow are located close to the optic nerve, whereas in the vascular system of the choroid, the entry and exit points are far apart. Moreover, the retinal vascular system has prominent avascular zones, such as the foveal avascular zone and peripheral avascular area, and many retinal vascular diseases are associated with flow defects in these areas. Meanwhile, the choroidal vascular bed has watershed zones situated between the various posterior ciliary arteries (PCA), short PCA, peripheral retrograde choroidal arteries, arterioles, and vortex veins (1, 122–124). Although the major posterior watershed zone of the choroid is usually situated between the nasal edge of the optic disc and the fovea in a vertical pattern (123, 125), it has been known to have considerable variations in location and configuration. Furthermore, a peripheral watershed zone between PCA and peripheral retrograde choroidal arteries has been identified in the temporal peripheral choroid near the equator (124). These various watershed zones, which can be found in various locations, from the macular to peripheral areas, have been associated with various types of chorioretinal disease (126–132). Despite these differences, subfoveal CT is a unique parameter representing the CT of an individual, similar to the central subfield thickness of the macula. Although one of the critical roles of the choroid is to supply blood to the retina in the foveal avascular zone and maintain the temperature of the macula (2), whether subfoveal CT is representative of the entire choroid of an individual still remains unclear. Third, another concern relates to the greater amplitude of physiological variation in subfoveal CT compared to that of the central subfield thickness of the macula (51, 133, 134), which is mainly derived from the diurnal change in the luminal area of the choroid (55). These findings have forced investigators to consider the time of the day when comparing subfoveal CT at different time points. In addition to diurnal variation, many factors, including age, sex, ethnicity, smoking, physical exertion, illumination, and alcohol consumption, can cause short-term changes in subfoveal CT (56–58, 135, 136). The finding that macular CT is vulnerable to physiological changes identifies the need to find a new way to calibrate CT measurements. Moreover, it remains questionable whether these variations are predictors of macular diseases or whether they are associated with them (137–139), though macular CT measurement has provided new insights into the understanding of macular diseases.
Choroidal Thickness Profile
Choroidal blood flow changes dynamically in different physiological states (56–58, 136), and diurnal variations in CT are observed in the submacular choroid (50–56). Therefore, in order to characterize the choroid in specific conditions, it is necessary to take into account not only the primary factors influencing CT variation, but also the secondary factors as well. Moreover, rather than taking a single CT measurement at one point, taking multiple measurements at different locations which represent choroidal characteristics may provide more details about the choroid of a patient. Several efforts have been made to overcome the limitations of subfoveal CT measurements in isolation. In some studies, CT measurements at locations other than the macula or subfoveal CT have been attempted in various diseases (23, 79, 82, 89, 104, 108). In others, CT was measured both at the macula and outside the macular region (26, 32, 77, 81). These studies showed that variations in CT outside the macula can be different from those within it (81, 97–101). Another approach was to calculate the ratio of different CT measurements (32, 33, 75). Park et al. (140) used the CT ratio, defined as the ratio of peripapillary CT in a quadrant or clock-hour position to the average peripapillary CT of the individual, and they suggested that the CT ratio, rather than the absolute peripapillary CT value, could be more helpful in assessing regional peripapillary CT differences in eyes with normal tension glaucoma. Hwang et al. (141) proposed the choroidal spatial distribution index (CSDI) to characterize the topographic features or variations of the choroid. After measuring the choroidal volume in each subfield of the ETDRS grid, to account for the topographic variations, they calculated the vertical and horizontal CSDIs as the natural logarithm of the superior and temporal choroidal volumes divided by the inferior and nasal choroidal volumes, respectively. Yun et al. (26) suggested that the characteristics of nasal peripapillary CT outside the macula could differ from those of macular CT, since the blood flow of the nasal peripapillary differs from that of the macular area. Kim et al. (33) reported that the ratio of subfoveal to nasal CT was the best predictor of early response in the treatment of polypoidal choroidal vasculopathy. In another study (76), the authors showed that the ratio of subfoveal to nasal CT in eyes with chronic CSC was significantly lower than that in eyes with normal fundus, acute CSC, and resolved CSC.
Profiling is the term used to describe the activity of collecting important and useful details about someone or something, and profile is the term for a short description of someone's life, work, character, etc. Profiling chorioretinal diseases or systemic illnesses using a CT profile could prove useful for understanding the role of the choroid in the pathophysiology of these diseases. Several parameters for the CT profile have been suggested in previous studies. In addition to subfoveal CT, parafoveal CT has also been included in the CT profile (142–149). While macular CT varies with physiological state, CT outside the macula has been suggested to have a tendency to be less affected by macular diseases and treatments (31–33, 119). Regional differences in CT, such as the ratio of CT at the macula and outside the macula could be candidates for surrogates of the choroid (32, 33).
Future Perspectives
The development of EDI-OCT and SS-OCT has ushered in a new era of in vivo and non-invasive CT measurements in humans. CT measurement is now a standard tool for characterizing the choroid in patients with ocular and systemic diseases. In addition to macular CT, CT outside the macula is expected to provide novel insights into the choroid. New parameters measured using OCT or OCT angiography, such as the choroidal vascularity index (150) and choriocapillaris flow void area (151), have recently been introduced for the characterization of the choroid, and these have helped us to better understand the choroid in normal and diseased eyes. Nevertheless, although more parameters can provide more information about the choroid, fewer parameters representing the choroid are easier to be adapted to busy daily clinics. In the future, we expect to determine which of the various choroidal parameters can more accurately and reliably represent the characteristics of the choroid in a particular disease. We also expect to determine which of these parameter combinations will help in the profiling of diseased eyes and patients.
Author Contributions
JO designed the study and wrote the first draft of the manuscript. YK and JO searched and collected the data and contributed to manuscript revision. All authors read and approved final manuscript.
Conflict of Interest
The authors declare that the research was conducted in the absence of any commercial or financial relationships that could be construed as a potential conflict of interest.
Publisher's Note
All claims expressed in this article are solely those of the authors and do not necessarily represent those of their affiliated organizations, or those of the publisher, the editors and the reviewers. Any product that may be evaluated in this article, or claim that may be made by its manufacturer, is not guaranteed or endorsed by the publisher.
References
1. Hayreh SS. In vivo choroidal circulation and its watershed zones. Eye. (1990) 4:273–89. doi: 10.1038/eye.1990.39
2. Nickla DL, Wallman J. The multifunctional choroid. Prog Retin Eye Res. (2010) 29:144–68. doi: 10.1016/j.preteyeres.2009.12.002
3. Yannuzzi LA, Slakter JS, Sorenson JA, Guyer DR, Orlock DA. Digital indocyanine green videoangiography and choroidal neovascularization. Retina. (1992) 12:191–223. doi: 10.1097/00006982-199212030-00003
4. Guyer DR, Yannuzzi LA, Slakter JS, Sorenson JA, Ho A, Orlock D. Digital indocyanine green videoangiography of central serous chorioretinopathy. Arch Ophthalmol. (1994) 112:1057–62. doi: 10.1001/archopht.1994.01090200063023
5. Prunte C, Flammer J. Choroidal capillary and venous congestion in central serous chorioretinopathy. Am J Ophthalmol. (1996) 121:26–34. doi: 10.1016/S0002-9394(14)70531-8
6. Spaide RF, Yannuzzi LA, Slakter JS, Sorenson J, Orlach DA. Indocyanine green videoangiography of idiopathic polypoidal choroidal vasculopathy. Retina. (1995) 15:100–10. doi: 10.1097/00006982-199515020-00003
7. Yannuzzi LA, Wong DW, Sforzolini BS, Goldbaum M, Tang KC, Spaide RF, et al. Polypoidal choroidal vasculopathy and neovascularized age-related macular degeneration. Arch Ophthalmol. (1999) 117:1503–10. doi: 10.1001/archopht.117.11.1503
8. Khan S, Engelbert M, Imamura Y, Freund KB. Polypoidal choroidal vasculopathy: simultaneous indocyanine green angiography and eye-tracked spectral domain optical coherence tomography findings. Retina. (2012) 32:1057–68. doi: 10.1097/IAE.0b013e31823beb14
9. Borruat FX, Auer C, Piguet B. Choroidopathy in multiple evanescent white dot syndrome. Arch Ophthalmol. (1995) 113:1569–71. doi: 10.1001/archopht.1995.01100120101021
10. Manjunath V, Taha M, Fujimoto JG, Duker JS. Choroidal thickness in normal eyes measured using Cirrus HD optical coherence tomography. Am J Ophthalmol. (2010) 150:325–9.e1. doi: 10.1016/j.ajo.2010.04.018
11. Regatieri CV, Branchini L, Carmody J, Fujimoto JG, Duker JS. Choroidal thickness in patients with diabetic retinopathy analyzed by spectral-domain optical coherence tomography. Retina. (2012) 32:563–8. doi: 10.1097/IAE.0B013E31822F5678
12. Spaide RF, Koizumi H, Pozzoni MC. Enhanced depth imaging spectral-domain optical coherence tomography. Am J Ophthalmol. (2008) 146:496–500. doi: 10.1016/j.ajo.2008.05.032
13. Mansouri K, Medeiros FA, Marchase N, Tatham AJ, Auerbach D, Weinreb RN. Assessment of choroidal thickness and volume during the water drinking test by swept-source optical coherence tomography. Ophthalmology. (2013) 120:2508–16. doi: 10.1016/j.ophtha.2013.07.040
14. Singh SR, Vupparaboina KK, Goud A, Dansingani KK, Chhablani J. Choroidal imaging biomarkers. Surv Ophthalmol. (2019) 64:312–33. doi: 10.1016/j.survophthal.2018.11.002
15. Kim SW, Oh J, Kwon SS, Yoo J, Huh K. Comparison of choroidal thickness among patients with healthy eyes, early age-related maculopathy, neovascular age-related macular degeneration, central serous chorioretinopathy, and polypoidal choroidal vasculopathy. Retina. (2011) 31:1904–11. doi: 10.1097/IAE.0b013e31821801c5
16. Tan KA, Gupta P, Agarwal A, Chhablani J, Cheng CY, Keane PA, et al. State of science: Choroidal thickness and systemic health. Surv Ophthalmol. (2016) 61:566–81. doi: 10.1016/j.survophthal.2016.02.007
17. Yeung SC, You Y, Howe KL, Yan P. Choroidal thickness in patients with cardiovascular disease: a review. Surv Ophthalmol. (2020) 65:473–86. doi: 10.1016/j.survophthal.2019.12.007
18. Mrejen S, Spaide RF. Optical coherence tomography: imaging of the choroid and beyond. Surv Ophthalmol. (2013) 58:387–429. doi: 10.1016/j.survophthal.2012.12.001
19. Doustar J, Torbati T, Black KL, Koronyo Y, Koronyo-Hamaoui M. Optical coherence tomography in Alzheimer's disease and other neurodegenerative diseases. Front Neurol. (2017) 8:701. doi: 10.3389/fneur.2017.00701
20. Fujiwara T, Imamura Y, Margolis R, Slakter JS, Spaide RF. Enhanced depth imaging optical coherence tomography of the choroid in highly myopic eyes. Am J Ophthalmol. (2009) 148:445–50. doi: 10.1016/j.ajo.2009.04.029
21. Spaide RF, Ledesma-Gil G. Choriocapillaris vascular parameters in normal eyes and those with pachychoroid with and without disease. Retina. (2021) 41:679–85. doi: 10.1097/IAE.0000000000002988
22. Switzer DW Jr, Mendonca LS, Saito M, Zweifel SA, Spaide RF. Segregation of ophthalmoscopic characteristics according to choroidal thickness in patients with early age-related macular degeneration. Retina. (2012) 32:1265–71. doi: 10.1097/IAE.0b013e31824453ac
23. Liu S, Wang W, Tan Y, He M, Wang L, Li Y, et al. Correlation between renal function and peripapillary choroidal thickness in treatment-naive diabetic eyes using swept-source optical coherence tomography. Curr Eye Res. (2020) 45:1526–33. doi: 10.1080/02713683.2020.1753213
24. Chang IB, Lee JH, Kim JS. Changes in choroidal thickness in and outside the macula after hemodialysis in patients with end-stage renal disease. Retina. (2017) 37:896–905. doi: 10.1097/IAE.0000000000001262
25. Ozcimen M, Sakarya Y, Kurtipek E, Bekci TT, Goktas S, Sakarya R, et al. Peripapillary choroidal thickness in patients with chronic obstructive pulmonary disease. Cutan Ocul Toxicol. (2016) 35:26–30. doi: 10.3109/15569527.2015.1004079
26. Yun C, Oh J, Han JY, Hwang SY, Moon SW, Huh K. Peripapillary choroidal thickness in central serous chorioretinopathy: is choroid outside the macula also thick? Retina. (2015) 35:1860–6. doi: 10.1097/IAE.0000000000000539
27. Yun C, Oh J, Ahn SE, Hwang SY, Kim SW, Huh K. Peripapillary choroidal thickness in patients with early age-related macular degeneration and reticular pseudodrusen. Graefes Arch Clin Exp Ophthalmol. (2016) 254:427–35. doi: 10.1007/s00417-015-3054-7
28. Yun C, Oh J, Ahn J, Hwang SY, Lee B, Kim SW, et al. Comparison of intravitreal aflibercept and ranibizumab injections on subfoveal and peripapillary choroidal thickness in eyes with neovascular age-related macular degeneration. Graefes Arch Clin Exp Ophthalmol. (2016) 254:1693–702. doi: 10.1007/s00417-015-3260-3
29. Singh SR, Invernizzi A, Rasheed MA, Cagini C, Goud A, Gujar R, et al. Wide-field choroidal vascular analysis in central serous chorioretinopathy. Eur J Ophthalmol. (2020) 31:2520–7. doi: 10.1177/1120672120963456
30. Kim MS, Lim HB, Lee WH, Kim KM, Nam KY, Kim JY. Wide-field swept-source optical coherence tomography analysis of interocular symmetry of choroidal thickness in healthy young individuals. Invest Ophthalmol Vis Sci. (2021) 62:5. doi: 10.1167/iovs.62.3.5
31. Lim HB, Kim K, Won YK, Lee WH, Lee MW, Kim JY, et al. comparison of choroidal thicknesses between pachychoroid and normochoroid eyes acquired from wide-field swept-source OCT. Acta Ophthalmol. (2021) 99:e117–e23. doi: 10.1111/aos.14522
32. Kim YH, Lee B, Kang E, Oh J. Clustering of eyes with age-related macular degeneration or pachychoroid spectrum diseases based on choroidal thickness profile. Sci Rep. (2021) 11:4999. doi: 10.1038/s41598-021-84650-7
33. Kim YH, Lee B, Kang E, Oh J. Choroidal thickness profile and clinical outcomes in eyes with polypoidal choroidal vasculopathy. Graefes Arch Clin Exp Ophthalmol. (2021) 259:1711–21. doi: 10.1007/s00417-020-05051-6
34. Coleman DJ, Lizzi FL. In vivo choroidal thickness measurement. Am J Ophthalmol. (1979) 88:369–75. doi: 10.1016/0002-9394(79)90635-4
35. Nickla DL, Wildsoet C, Wallman J. Visual influences on diurnal rhythms in ocular length and choroidal thickness in chick eyes. Exp Eye Res. (1998) 66:163–81. doi: 10.1006/exer.1997.0420
36. Branchini L, Regatieri CV, Flores-Moreno I, Baumann B, Fujimoto JG, Duker JS. Reproducibility of choroidal thickness measurements across three spectral domain optical coherence tomography systems. Ophthalmology. (2012) 119:119–23. doi: 10.1016/j.ophtha.2011.07.002
37. Adhi M, Liu JJ, Qavi AH, Grulkowski I, Lu CD, Mohler KJ, et al. Choroidal analysis in healthy eyes using swept-source optical coherence tomography compared to spectral domain optical coherence tomography. Am J Ophthalmol. (2014) 157:1272–81.e1. doi: 10.1016/j.ajo.2014.02.034
38. Copete S, Flores-Moreno I, Montero JA, Duker JS, Ruiz-Moreno JM. Direct comparison of spectral-domain and swept-source OCT in the measurement of choroidal thickness in normal eyes. Br J Ophthalmol. (2014) 98:334–8. doi: 10.1136/bjophthalmol-2013-303904
39. Lee MW, Park HJ, Shin YI, Lee WH, Lim HB, Kim JY. Comparison of choroidal thickness measurements using swept source and spectral domain optical coherence tomography in pachychoroid diseases. PLoS ONE. (2020) 15:e0229134. doi: 10.1371/journal.pone.0229134
40. Chen FK, Yeoh J, Rahman W, Patel PJ, Tufail A, Da Cruz L. Topographic variation and interocular symmetry of macular choroidal thickness using enhanced depth imaging optical coherence tomography. Invest Ophthalmol Vis Sci. (2012) 53:975–85. doi: 10.1167/iovs.11-8771
41. Ikuno Y, Kawaguchi K, Nouchi T, Yasuno Y. Choroidal thickness in healthy Japanese subjects. Invest Ophthalmol Vis Sci. (2010) 51:2173–6. doi: 10.1167/iovs.09-4383
42. Ho M, Liu DT, Chan VC, Lam DS. Choroidal thickness measurement in myopic eyes by enhanced depth optical coherence tomography. Ophthalmology. (2013) 120:1909–14. doi: 10.1016/j.ophtha.2013.02.005
43. Hirata M, Tsujikawa A, Matsumoto A, Hangai M, Ooto S, Yamashiro K, et al. Macular choroidal thickness and volume in normal subjects measured by swept-source optical coherence tomography. Invest Ophthalmol Vis Sci. (2011) 52:4971–8. doi: 10.1167/iovs.11-7729
44. Heirani M, Shandiz JH, Shojaei A, Narooie-Noori F. Choroidal thickness profile in normal iranian eyes with different refractive status by spectral-domain optical coherence tomography. J Curr Ophthalmol. (2020) 32:58–68. doi: 10.1016/j.joco.2019.08.005
45. Breher K, Terry L, Bower T, Wahl S. Choroidal biomarkers: a repeatability and topographical comparison of choroidal thickness and choroidal vascularity index in healthy eyes. Transl Vis Sci Technol. (2020) 9:8. doi: 10.1167/tvst.9.11.8
46. Kugelman J, Alonso-Caneiro D, Read SA, Hamwood J, Vincent SJ, Chen FK, et al. Automatic choroidal segmentation in OCT images using supervised deep learning methods. Sci Rep. (2019) 9:13298. doi: 10.1038/s41598-019-49816-4
47. Baghaie A, Yu Z, D'Souza RM. State-of-the-art in retinal optical coherence tomography image analysis. Quant Imaging Med Surg. (2015) 5:603–17. doi: 10.3978/j.issn.2223-4292.2015.07.02
48. Spaide RF. The ambiguity of pachychoroid. Retina. (2021) 41:231–7. doi: 10.1097/IAE.0000000000003057
49. Wei WB, Xu L, Jonas JB, Shao L, Du KF, Wang S, et al. Subfoveal choroidal thickness: the Beijing eye study. Ophthalmology. (2013) 120:175–80. doi: 10.1016/j.ophtha.2012.07.048
50. Nickla DL, Wildsoet CF, Troilo D. Diurnal rhythms in intraocular pressure, axial length, and choroidal thickness in a primate model of eye growth, the common marmoset. Invest Ophthalmol Vis Sci. (2002) 43:2519–28.
51. Brown JS, Flitcroft DI, Ying GS, Francis EL, Schmid GF, Quinn GE, et al. In vivo human choroidal thickness measurements: evidence for diurnal fluctuations. Invest Ophthalmol Vis Sci. (2009) 50:5–12. doi: 10.1167/iovs.08-1779
52. Chakraborty R, Read SA, Collins MJ. Diurnal variations in axial length, choroidal thickness, intraocular pressure, and ocular biometrics. Invest Ophthalmol Vis Sci. (2011) 52:5121–9. doi: 10.1167/iovs.11-7364
53. Tan CS, Ouyang Y, Ruiz H, Sadda SR. Diurnal variation of choroidal thickness in normal, healthy subjects measured by spectral domain optical coherence tomography. Invest Ophthalmol Vis Sci. (2012) 53:261–6. doi: 10.1167/iovs.11-8782
54. Usui S, Ikuno Y, Akiba M, Maruko I, Sekiryu T, Nishida K, et al. Circadian changes in subfoveal choroidal thickness and the relationship with circulatory factors in healthy subjects. Invest Ophthalmol Vis Sci. (2012) 53:2300–7. doi: 10.1167/iovs.11-8383
55. Kinoshita T, Mitamura Y, Shinomiya K, Egawa M, Iwata A, Fujihara A, et al. Diurnal variations in luminal and stromal areas of choroid in normal eyes. Br J Ophthalmol. (2017) 101:360–4. doi: 10.1136/bjophthalmol-2016-308594
56. Ahn J, Ahn SE, Yang KS, Kim SW, Oh J. Effects of a high level of illumination before sleep at night on chorioretinal thickness and ocular biometry. Exp Eye Res. (2017) 164:157–67. doi: 10.1016/j.exer.2017.09.001
57. Sizmaz S, Kucukerdonmez C, Pinarci EY, Karalezli A, Canan H, Yilmaz G. The effect of smoking on choroidal thickness measured by optical coherence tomography. Br J Ophthalmol. (2013) 97:601–4. doi: 10.1136/bjophthalmol-2012-302393
58. Sayin N, Kara N, Pekel G, Altinkaynak H. Choroidal thickness changes after dynamic exercise as measured by spectral-domain optical coherence tomography. Indian J Ophthalmol. (2015) 63:445–50. doi: 10.4103/0301-4738.159884
59. Huang W, Wang W, Zhou M, Chen S, Gao X, Fan Q, et al. Peripapillary choroidal thickness in healthy Chinese subjects. BMC Ophthalmol. (2013) 13:23. doi: 10.1186/1471-2415-13-23
60. Gupta P, Jing T, Marziliano P, Baskaran M, Cheung GC, Lamoureux EL, et al. Peripapillary choroidal thickness assessed using automated choroidal segmentation software in an Asian population. Br J Ophthalmol. (2015) 99:920–6. doi: 10.1136/bjophthalmol-2014-306152
61. Jiang R, Wang YX, Wei WB, Xu L, Jonas JB. Peripapillary choroidal thickness in adult Chinese: the Beijing eye study. Invest Ophthalmol Vis Sci. (2015) 56:4045–52. doi: 10.1167/iovs.15-16521
62. Wang YX, Yang H, Luo H, Hong SW, Gardiner SK, Jeoung JW, et al. Peripapillary scleral bowing increases with age and is inversely associated with peripapillary choroidal thickness in healthy eyes. Am J Ophthalmol. (2020) 217:91–103. doi: 10.1016/j.ajo.2020.03.050
63. Spaide RF. Age-related choroidal atrophy. Am J Ophthalmol. (2009) 147:801–10. doi: 10.1016/j.ajo.2008.12.010
64. Oh J, Yoo C, Yun CM, Yang KS, Kim SW, Huh K. Simplified method to measure the peripapillary choroidal thickness using three-dimensional optical coherence tomography. Korean J Ophthalmol. (2013) 27:172–7. doi: 10.3341/kjo.2013.27.3.172
65. Sung MS, Heo H, Piao H, Guo Y, Park SW. Parapapillary atrophy and changes in the optic nerve head and posterior pole in high myopia. Sci Rep. (2020) 10:4607. doi: 10.1038/s41598-020-61485-2
66. Sun D, Du Y, Chen Q, Ye L, Chen H, Li M, et al. Imaging features by machine learning for quantification of optic disc changes and impact on choroidal thickness in young myopic patients. Front Med. (2021) 8:657566. doi: 10.3389/fmed.2021.657566
67. Shibata H, Sawada Y, Ishikawa M, Yoshitomi T, Iwase T. Peripapillary choroidal thickness assessed by spectral-domain optical coherence tomography in normal Japanese. Jpn J Ophthalmol. (2021) 65:666–71. doi: 10.1007/s10384-021-00843-7
68. Zhang Z, Yu M, Wang F, Dai Y, Wu Z. Choroidal thickness and open-angle glaucoma: a meta-analysis and systematic review. J Glaucoma. (2016) 25:e446–54. doi: 10.1097/IJG.0000000000000275
69. Fenolland JR, Giraud JM, May F, Mouinga A, Seck S, Renard JP. Enhanced depth imaging of the choroid in open-angle glaucoma: a preliminary study. J Fr Ophtalmol. (2011) 34:313–7. doi: 10.1016/j.jfo.2011.02.004
70. Maul EA, Friedman DS, Chang DS, Boland MV, Ramulu PY, Jampel HD, et al. Choroidal thickness measured by spectral domain optical coherence tomography: factors affecting thickness in glaucoma patients. Ophthalmology. (2011) 118:1571–9. doi: 10.1016/j.ophtha.2011.01.016
71. Fard MA, Abdi P, Kasaei A, Soltani Mogaddam R, Afzali M, Moghimi S. Peripapillary choroidal thickness in nonarteritic anterior ischemic optic neuropathy. Invest Ophthalmol Vis Sci. (2015). doi: 10.1167/iovs.14-15661
72. Nam KT, Chung HW, Jang S, Kim SW, Oh J, Yun C. Features of the macular and peripapillary choroid and choriocapillaris in eyes with nonexudative age-related macular degeneration. Retina. (2020) 40:2270–6. doi: 10.1097/IAE.0000000000002758
73. Phasukkijwatana N, Freund KB, Dolz-Marco R, Al-Sheikh M, Keane PA, Egan CA, et al. Peripapillary pachychoroid syndrome. Retina. (2018) 38:1652–67. doi: 10.1097/IAE.0000000000001907
74. Baek J, Dansingani KK, Lee JH, Lee WK, Freund KB. Choroidal morphology in eyes with peripapillary polypoidal choroidal vasculopathy. Retina. (2019) 39:1571–9. doi: 10.1097/IAE.0000000000002188
75. Kim YH, Lee B, Kang E, Oh J. Comparison of regional differences in the choroidal thickness between patients with pachychoroid neovasculopathy and classic exudative age-related macular degeneration. Curr Eye Res. (2021) 46:1–8. doi: 10.1080/02713683.2021.1887269
76. Kim YH, Lee B, Kang E, Oh J. Peripapillary choroidal vascularity outside the macula in patients with central serous chorioretinopathy. Transl Vis Sci Technol. (2021) 10:9. doi: 10.1167/tvst.10.8.9
77. Kang HM, Choi JH, Koh HJ, Lee CS, Lee SC. Significant reduction of peripapillary choroidal thickness in patients with unilateral branch retinal vein occlusion. Retina. (2018) 38:72–8. doi: 10.1097/IAE.0000000000001495
78. Lee NE, Kang HM, Choi JH, Koh HJ, Lee SC. Sectoral changes of the peripapillary choroidal thickness in patients with unilateral branch retinal vein occlusion. Int J Ophthalmol. (2019) 12:472–9. doi: 10.18240/ijo.2019.03.19
79. Sirakaya E, Kucuk B. Thickness of the lamina cribrosa, retinal-nerve fiber layer, and peripapillary choroid in patients with branch retinal vein occlusion. Ophthalmologica. (2020) 243:288–96. doi: 10.1159/000505100
80. Kang HM, Kim EW, Choi JH, Koh HJ, Lee SC. Focal lamina cribrosa defects and significant peripapillary choroidal thinning in patients with unilateral branch retinal vein occlusion. PLoS ONE. (2020) 15:e0230293. doi: 10.1371/journal.pone.0230293
81. Cetin EN, Parca O, Akkaya HS, Pekel G. Association of retinal biomarkers and choroidal vascularity index on optical coherence tomography using binarization method in retinitis pigmentosa. Graefes Arch Clin Exp Ophthalmol. (2020) 258:23–30. doi: 10.1007/s00417-019-04516-7
82. Balci S, Turan-Vural E. Evaluation of lamina cribrosa by using enhanced depth imaging optical coherence tomography in ocular sarcoidosis during quiescent phase. Optom Vis Sci. (2021) 98:137–42. doi: 10.1097/OPX.0000000000001644
83. Silvestri G, Archer DB, Johnston PB. Peripapillary subretinal neovascular membranes: the natural history. Eye. (1993) 7:398–402. doi: 10.1038/eye.1993.78
84. Lafaut BA, Leys AM, Snyers B, Rasquin F, De Laey JJ. Polypoidal choroidal vasculopathy in Caucasians. Graefes Arch Clin Exp Ophthalmol. (2000) 238:752–9. doi: 10.1007/s004170000180
85. Browning DJ, Fraser CM. Ocular conditions associated with peripapillary subretinal neovascularization, their relative frequencies, and associated outcomes. Ophthalmology. (2005) 112:1054–61. doi: 10.1016/j.ophtha.2004.11.062
86. Coscas G, Lupidi M, Coscas F, Benjelloun F, Zerbib J, Dirani A, et al. Toward a specific classification of polypoidal choroidal vasculopathy: idiopathic disease or subtype of age-related macular degeneration. Invest Ophthalmol Vis Sci. (2015) 56:3187–95. doi: 10.1167/iovs.14-16236
87. Imamura Y, Fujiwara T, Margolis R, Spaide RF. Enhanced depth imaging optical coherence tomography of the choroid in central serous chorioretinopathy. Retina. (2009) 29:1469–73. doi: 10.1097/IAE.0b013e3181be0a83
88. Steiner M, Esteban-Ortega MDM, Muñoz-Fernández S. Choroidal and retinal thickness in systemic autoimmune and inflammatory diseases: a review. Surv Ophthalmol. (2019) 64:757–69. doi: 10.1016/j.survophthal.2019.04.007
89. Loureiro M, Braga AC, Meira D, Sepulveda P, Agrelos L, Torres P. Peripapillary choroidal thickness by enhanced depth imaging optical coherence tomography: the impact of metabolic syndrome. Eur J Ophthalmol. (2017) 27:428–32. doi: 10.5301/ejo.5000911
90. Lee WJ, Hong R, Kang MH, Cho H, Han SW Yi JH, et al. Effect of hemodialysis on peripapillary choroidal thickness measured by swept-source optical coherence tomography. J Glaucoma. (2021) 30:459–64. doi: 10.1097/IJG.0000000000001762
91. Turan-Vural E, Vural U. Evaluation of peripapillary and subfoveal choroid thickness in asymptomatic carotid artery stenosis. Clin Ophthalmol. (2020) 14:1641–50. doi: 10.2147/OPTH.S237403
92. Tsapardoni F, Makri OE, Lazaris V, Labropoulou V, Lygeros S, Mastronikolis S, et al. Choroidal thickness evaluation in a transfusion-dependent beta-thalassemia Greek population. Clin Ophthalmol. (2020) 14:4511–8. doi: 10.2147/OPTH.S285312
93. Vural E, Hazar L, Caglayan M, Seker O, Celebi ARC. Peripapillary choroidal thickness in patients with vitamin D deficiency. Eur J Ophthalmol. (2021) 31:578–83. doi: 10.1177/1120672120902025
94. Yazgan S, Arpaci D, Celik HU, Dogan M, Isik I. Macular choroidal thickness may be the earliest determiner to detect the onset of diabetic retinopathy in patients with prediabetes: a prospective and comparative study. Curr Eye Res. (2017) 42:1039–47. doi: 10.1080/02713683.2016.1264606
95. Vujosevic S, Martini F, Cavarzeran F, Pilotto E, Midena E. Macular and peripapillary choroidal thickness in diabetic patients. Retina. (2012) 32:1781–90. doi: 10.1097/IAE.0b013e31825db73d
96. Li T, Jia Y, Wang S, Xu Y, Yin Y, Wang A, et al. Change in peripapillary and macular choroidal thickness change in children with type 1 diabetes mellitus without visual impairment or diabetic retinopathy. Acta Ophthalmol. (2020) 98:e203–e11. doi: 10.1111/aos.14225
97. Ermerak BC, Yalcinbayir O, Eren E, Sobu E, Erseven C, Yucel AA. Evaluation of choroidal thickness in children with type 1 diabetes: the role of optical coherence tomography in diabetic retinopathy screening. Clin Pediatr Endocrinol. (2021) 30:41–7. doi: 10.1297/cpe.30.41
98. Gul A, Basural E, Ozturk HE. Comparison of choroidal thickness in patients with active and stable thyroid eye disease. Arq Bras Oftalmol. (2019) 82:124–8. doi: 10.5935/0004-2749.20190019
99. Lai FHP, Iao TWU, Ng DSC, Young AL, Leung J, Au A, et al. Choroidal thickness in thyroid-associated orbitopathy. Clin Exp Ophthalmol. (2019) 47:918–24. doi: 10.1111/ceo.13525
100. Yazgan S, Erboy F, Celik HU, Ornek T, Ugurbas SH, Kokturk F, et al. Peripapillary choroidal thickness and retinal nerve fiber layer in untreated patients with obstructive sleep apnea-hypopnea syndrome: a case-control study. Curr Eye Res. (2017) 42:1552–60. doi: 10.1080/02713683.2016.1266661
101. Andrade C, Beato J, Monteiro A, Costa A, Penas S, Guimaraes J, et al. Spectral-domain optical coherence tomography as a potential biomarker in Huntington's disease. Mov Disord. (2016) 31:377–83. doi: 10.1002/mds.26486
102. Afonso JM, Falcao M, Schlichtenbrede F, Falcao-Reis F, Silva SE, Schneider TM. Spectral domain-optical coherence tomography as a new diagnostic marker for idiopathic normal pressure hydrocephalus. Front Neurol. (2017) 8:172. doi: 10.3389/fneur.2017.00172
103. Yazgan S, Arpaci D, Celik HU, Isik I. Evaluation of macular and peripapillary choroidal thickness, macular volume and retinal nerve fiber layer in acromegaly patients. Int Ophthalmol. (2018) 38:617–25. doi: 10.1007/s10792-017-0500-z
104. Garcia-Martin E, Pablo LE, Bambo MP, Alarcia R, Polo V, Larrosa JM, et al. Comparison of peripapillary choroidal thickness between healthy subjects and patients with Parkinson's disease. PLoS ONE. (2017) 12:e0177163. doi: 10.1371/journal.pone.0177163
105. Satue M, Obis J, Alarcia R, Orduna E, Rodrigo MJ, Vilades E, et al. Retinal and choroidal changes in patients with Parkinson's disease detected by swept-source optical coherence tomography. Curr Eye Res. (2018) 43:109–15. doi: 10.1080/02713683.2017.1370116
106. Garcia-Martin E, Jarauta L, Pablo LE, Bambo MP, Ara JR, Martin J, et al. Changes in peripapillary choroidal thickness in patients with multiple sclerosis. Acta Ophthalmol. (2019) 97:e77–83. doi: 10.1111/aos.13807
107. Macias BR, Patel NB, Gibson CR, Samuels BC, Laurie SS, Otto C, et al. Association of long-duration spaceflight with anterior and posterior ocular structure changes in astronauts and their recovery. JAMA Ophthalmol. (2020) 138:553–9. doi: 10.1001/jamaophthalmol.2020.0673
108. Fieß A, Christian L, Kolb-Keerl R, Knuf M, Kirchhof B, Muether PS, et al. Peripapillary choroidal thickness in former preterm and full-term infants aged from 4 to 10 years. Invest Ophthalmol Vis Sci. (2016) 57:6548–53. doi: 10.1167/iovs.16-20128
109. Yavuz C, Ozcimen M. An evaluation of peripapillar choroidal thickness in patients receiving systemic isotretinoin treatment. Cutan Ocul Toxicol. (2019) 38:25–8. doi: 10.1080/15569527.2018.1503289
110. Casado A, Lopez-de-Eguileta A, Gaitan J, Fonseca S, Gordo-Vega MA. Peripapillary and macular choroidal thickness before and after phenylephrine instillation. Eye. (2019) 33:1741–7. doi: 10.1038/s41433-019-0478-z
111. London A, Benhar I, Schwartz M. The retina as a window to the brain-from eye research to CNS disorders. Nat Rev Neurol. (2013) 9:44–53. doi: 10.1038/nrneurol.2012.227
112. Chan VTT, Sun Z, Tang S, Chen LJ, Wong A, Tham CC, et al. Spectral-domain OCT measurements in Alzheimer's disease: a systematic review and meta-analysis. Ophthalmology. (2019) 126:497–510. doi: 10.1016/j.ophtha.2018.08.009
113. Mori K, Kanno J, Gehlbach PL, Yoneya S. Montage images of spectral-domain optical coherence tomography in eyes with idiopathic macular holes. Ophthalmology. (2012) 119:2600–8. doi: 10.1016/j.ophtha.2012.06.027
114. Chu RL, Pannullo NA, Adam CR, Rafieetary MR, Sigler EJ. Morphology of peripheral vitreoretinal interface abnormalities imaged with spectral domain optical coherence tomography. J Ophthalmol. (2019) 2019:3839168. doi: 10.1155/2019/3839168
115. Pichi F, Carrai P, Bonsignore F, Villani E, Ciardella AP, Nucci P. Wide-field spectral domain optical coherence tomography. Retina. (2015) 35:2584–92. doi: 10.1097/IAE.0000000000000611
116. Choudhry N, Golding J, Manry MW, Rao RC. Ultra-widefield steering-based spectral-domain optical coherence tomography imaging of the retinal periphery. Ophthalmology. (2016) 123:1368–74. doi: 10.1016/j.ophtha.2016.01.045
117. McNabb RP, Grewal DS, Mehta R, Schuman SG, Izatt JA, Mahmoud TH, et al. Wide field of view swept-source optical coherence tomography for peripheral retinal disease. Br J Ophthalmol. (2016) 100:1377–82. doi: 10.1136/bjophthalmol-2015-307480
118. Mohler KJ, Draxinger W, Klein T, Kolb JP, Wieser W, Haritoglou C, et al. Combined 60 degrees wide-field choroidal thickness maps and high-definition en face vasculature visualization using swept-source megahertz OCT at 1050 nm. Invest Ophthalmol Vis Sci. (2015) 56:6284–93. doi: 10.1167/iovs.15-16670
119. Rasheed MA, Singh SR, Invernizzi A, Cagini C, Goud A, Sahoo NK, et al. Wide-field choroidal thickness profile in healthy eyes. Sci Rep. (2018) 8:17166. doi: 10.1038/s41598-018-35640-9
120. Hoseini-Yazdi H, Vincent SJ, Collins MJ, Read SA, Alonso-Caneiro D. Wide-field choroidal thickness in myopes and emmetropes. Sci Rep. (2019) 9:3474. doi: 10.1038/s41598-019-39653-w
121. Breher K, Ohlendorf A, Wahl S. Myopia induces meridional growth asymmetry of the retina: a pilot study using wide-field swept-source OCT. Sci Rep. (2020) 10:10886. doi: 10.1038/s41598-020-67940-4
122. Hayreh SS. Segmental nature of the choroidal vasculature. Br J Ophthalmol. (1975) 59:631–48. doi: 10.1136/bjo.59.11.631
123. Hayreh SS. Posterior ciliary artery circulation in health and disease: the Weisenfeld lecture. Invest Ophthalmol Vis Sci. (2004) 45:749–57. doi: 10.1167/iovs.03-0469
124. Takahashi K, Muraoka K, Kishi S, Shimizu K. Watershed zone in the human peripheral choroid. Ophthalmology. (1996) 103:336–42. doi: 10.1016/S0161-6420(96)30695-7
125. Giuffre G. Main posterior watershed zone of the choroid. Variations of its position in normal subjects. Doc Ophthalmol. (1989) 72:175–80. doi: 10.1007/BF00156707
126. Mendrinos E, Pournaras CJ. Topographic variation of the choroidal watershed zone and its relationship to neovascularization in patients with age-related macular degeneration. Acta Ophthalmol. (2009) 87:290–6. doi: 10.1111/j.1755-3768.2008.01247.x
127. Alten F, Clemens CR, Heiduschka P, Eter N. Localized reticular pseudodrusen and their topographic relation to choroidal watershed zones and changes in choroidal volumes. Invest Ophthalmol Vis Sci. (2013) 54:3250–7. doi: 10.1167/iovs.13-11923
128. Ross RD, Barofsky JM, Cohen G, Baber WB, Palao SW, Gitter KA. Presumed macular choroidal watershed vascular filling, choroidal neovascularization, and systemic vascular disease in patients with age-related macular degeneration. Am J Ophthalmol. (1998) 125:71–80. doi: 10.1016/S0002-9394(99)80237-2
129. Shin MK, Lee JE, Byon IS, Park SW. Choroidal watershed zone and growth of polypoidal choroidal vasculopathy. Curr Eye Res. (2017) 42:252–9. doi: 10.1080/02713683.2016.1183794
130. Lee JE, Shin MK, Chung IY, Lee JE, Kim HW, Lee SJ, et al. Topographical relationship between the choroidal watershed zone and submacular idiopathic choroidal neovascularisation. Br J Ophthalmol. (2016) 100:652–9. doi: 10.1136/bjophthalmol-2015-306678
131. Bae K, Cho K, Kang SW, Kim SJ, Kim JM. Peripheral reticular pigmentary degeneration and choroidal vascular insufficiency, studied by ultra wide-field fluorescein angiography. PLoS One. (2017) 12:e0170526. doi: 10.1371/journal.pone.0170526
132. Mantel I, Schalenbourg A, Zografos L. Peripheral exudative hemorrhagic chorioretinopathy: polypoidal choroidal vasculopathy and hemodynamic modifications. Am J Ophthalmol. (2012) 153:910–22.e2. doi: 10.1016/j.ajo.2011.10.017
133. Toyokawa N, Kimura H, Fukomoto A, Kuroda S. Difference in morning and evening choroidal thickness in Japanese subjects with no chorioretinal disease. Ophthalmic Surg Lasers Imaging. (2012) 43:109–14. doi: 10.3928/15428877-20120102-06
134. Han YS, Lim HB, Lee SH, Kim JY. Diurnal variation in choroidal and retinal thickness of the early treatment of diabetic retinopathy study macular subfields determined using swept-source optical coherence tomography. Ophthalmologica. (2015) 233:192–7. doi: 10.1159/000375538
135. Song Y, Tham YC, Chong C, Ong R, Fenner BJ, Cheong KX, et al. Patterns and determinants of choroidal thickness in a multiethnic Asian population: the Singapore epidemiology of eye diseases study. Ophthalmol Retina. (2021) 5:458–67. doi: 10.1016/j.oret.2020.08.012
136. Kang HM, Woo YJ, Koh HJ, Lee CS, Lee SC. The effect of consumption of ethanol on subfoveal choroidal thickness in acute phase. Br J Ophthalmol. (2016) 100:383–8. doi: 10.1136/bjophthalmol-2015-306969
137. Bouteleux V, Kodjikian L, Mendes M, Agard E, Machkour-Bentaleb Z, El-Chehab H, et al. Increased choroidal thickness: a new feature to monitor age-related macular degeneration recurrence. Graefes Arch Clin Exp Ophthalmol. (2019) 257:699–707. doi: 10.1007/s00417-018-04216-8
138. Mathis T, Mendes M, Dot C, Bouteleux V, Machkour-Bentaleb Z, El Chehab H, et al. Increased choroidal thickness: a new indicator for monitoring diabetic macular oedema recurrence. Acta Ophthalmol. (2020) 98:e968–e74. doi: 10.1111/aos.14447
139. Mathis T, Dot C, Mauget-Faysse M, Kodjikian L. Variation of choroidal thickness in diabetic macular edema: friend or foe? Acta Ophthalmol. (2021) 99:e282–e3. doi: 10.1111/aos.14530
140. Park JH, Yoo C, Kim YY. Peripapillary choroidal thickness in untreated normal-tension glaucoma eyes with a single-hemifield retinal nerve fiber layer defect. Medicine. (2018) 97:e11001. doi: 10.1097/MD.0000000000011001
141. Hwang S, Kong M, Song YM, Ham DI. Choroidal spatial distribution indexes as novel parameters for topographic features of the choroid. Sci Rep. (2020) 10:574. doi: 10.1038/s41598-019-57211-2
142. Ayton LN, Guymer RH, Luu CD. Choroidal thickness profiles in retinitis pigmentosa. Clin Exp Ophthalmol. (2013) 41:396–403. doi: 10.1111/j.1442-9071.2012.02867.x
143. Kuroda S, Ikuno Y, Yasuno Y, Nakai K, Usui S, Sawa M, et al. Choroidal thickness in central serous chorioretinopathy. Retina. (2013) 33:302–8. doi: 10.1097/IAE.0b013e318263d11f
144. Park KA, Oh SY. Choroidal thickness in healthy children. Retina. (2013) 33:1971–6. doi: 10.1097/IAE.0b013e3182923477
145. Ruiz-Medrano J, Flores-Moreno I, Pena-Garcia P, Montero JA, Duker JS, Ruiz-Moreno JM. Macular choroidal thickness profile in a healthy population measured by swept-source optical coherence tomography. Invest Ophthalmol Vis Sci. (2014) 55:3532–42. doi: 10.1167/iovs.14-13868
146. Chhablani J, Nayaka A, Rani PK, Jalali S. Choroidal thickness profile in inherited retinal diseases in Indian subjects. Indian J Ophthalmol. (2015) 63:391–3. doi: 10.4103/0301-4738.159862
147. Chhablani JK, Deshpande R, Sachdeva V, Vidya S, Rao PS, Panigati A, et al. Choroidal thickness profile in healthy Indian children. Indian J Ophthalmol. (2015) 63:474–7. doi: 10.4103/0301-4738.162577
148. Ruiz-Medrano J, Flores-Moreno I, Pena-Garcia P, Montero JA, Duker JS, Ruiz-Moreno JM. Asymmetry in macular choroidal thickness profile between both eyes in a healthy population measured by swept-source optical coherence tomography. Retina. (2015) 35:2067–73. doi: 10.1097/IAE.0000000000000590
149. Ruiz-Medrano J, Flores-Moreno I, Pena-Garcia P, Montero JA, Garcia-Feijoo J, Duker JS, et al. Analysis of age-related choroidal layers thinning in healthy eyes using swept-source optical coherence tomography. Retina. (2017) 37:1305–13. doi: 10.1097/IAE.0000000000001347
150. Agrawal R, Salman M, Tan KA, Karampelas M, Sim DA, Keane PA, et al. Choroidal vascularity index (CVI)–a novel optical coherence tomography parameter for monitoring patients with panuveitis? PLoS ONE. (2016) 11:e0146344. doi: 10.1371/journal.pone.0146344
Keywords: choroid, choroidal thickness, profile, choroidal thickness profile, choroidal profile, retinal disease, optical coherence tomography
Citation: Kim YH and Oh J (2021) Choroidal Thickness Profile in Chorioretinal Diseases: Beyond the Macula. Front. Med. 8:797428. doi: 10.3389/fmed.2021.797428
Received: 18 October 2021; Accepted: 30 November 2021;
Published: 20 December 2021.
Edited by:
Yitian Zhao, Ningbo Institute of Materials Technology & Engineering, Chinese Academy of Sciences (CAS), ChinaReviewed by:
Meng Wang, Soochow University, ChinaJianyang Xie, Ningbo Institute of Materials Technology & Engineering, Chinese Academy of Sciences (CAS), China
Copyright © 2021 Kim and Oh. This is an open-access article distributed under the terms of the Creative Commons Attribution License (CC BY). The use, distribution or reproduction in other forums is permitted, provided the original author(s) and the copyright owner(s) are credited and that the original publication in this journal is cited, in accordance with accepted academic practice. No use, distribution or reproduction is permitted which does not comply with these terms.
*Correspondence: Jaeryung Oh, b2pyNDk5MUBrb3JlYS5hYy5rcg==