- 1Foodborne and Waterborne Diseases Research Center, Research Institute for Gastroenterology and Liver Diseases, Shahid Beheshti University of Medical Sciences, Tehran, Iran
- 2Gastroenterology and Liver Diseases Research Center, Research Institute for Gastroenterology and Liver Diseases, Shahid Beheshti University of Medical Sciences, Tehran, Iran
- 3Basic and Molecular Epidemiology of Gastrointestinal Disorders Research Center, Research Institute for Gastroenterology and Liver Diseases, Shahid Beheshti University of Medical Sciences, Tehran, Iran
- 4Internal Medicine and Emergency Medicine, St'Andrea Hospital, Sapienza University, Rome, Italy
- 5Department of Clinical and Molecular Medicine, Sapienza University, Rome, Italy
- 6Infectious Diseases Service, University Hospital Campus Bio-Medico, Rome, Italy
Coronavirus disease 2019 (COVID-19) has rapidly spread all over the world with a very high rate of mortality. Different symptoms developed by COVID-19 infection and its impacts on various organs of the human body have highlighted the importance of both coinfections and superinfections with other pathogens. The gastrointestinal (GI) tract is vulnerable to infection with COVID-19 and can be exploited as an alternative transmission route and target for virus entry and pathogenesis. The GI manifestations of COVID-19 disease are associated with severe disease outcomes and death in all age groups, in particular, elderly patients. Empiric antibiotic treatments for microbial infections in hospitalized patients with COVID-19 in addition to experimental antiviral and immunomodulatory drugs may increase the risk of antibiotic-associated diarrhea (AAD) and Clostridioides difficile infection (CDI). Alterations of gut microbiota are associated with depletion of beneficial commensals and enrichment of opportunistic pathogens such as C. difficile. Hence, the main purpose of this review is to explain the likely risk factors contributing to higher incidence of CDI in patients with COVID-19. In addition to lung involvement, common symptoms observed in COVID-19 and CDI such as diarrhea, highlight the significance of bacterial infections in COVID-19 patients. In particular, hospitalized elderly patients who are receiving antibiotics might be more prone to CDI. Indeed, widespread use of broad-spectrum antibiotics such as clindamycin, cephalosporins, penicillin, and fluoroquinolones can affect the composition and function of the gut microbiota of patients with COVID-19, leading to reduced colonization resistance capacity against opportunistic pathogens such as C. difficile, and subsequently develop CDI. Moreover, patients with CDI possibly may have facilitated the persistence of SARS-CoV-2 viral particles in their feces for approximately one month, even though the nasopharyngeal test turned negative. This coinfection may increase the potential transmissibility of both SARS-CoV-2 and C. difficile by fecal materials. Also, CDI can complicate the outcome of COVID-19 patients, especially in the presence of comorbidities or for those patients with prior exposure to the healthcare setting. Finally, physicians should remain vigilant for possible SARS-CoV-2 and CDI coinfection during the ongoing COVID-19 pandemic and the excessive use of antimicrobials and biocides.
Introduction
Coronavirus disease 2019 (COVID-19) is a respiratory infection caused by the newly identified beta-coronavirus known as the severe acute respiratory syndrome coronavirus 2 (SARS-CoV-2) (1, 2). Although COVID-19 outbreak first occurred in Wuhan, a city in Hubei province of China on December 2019, it rapidly spread via human-human contact to the rest of the world (3, 4). When the virus infected people of 114 countries, COVID-19 was called a “pandemic” by the World Health Organization (WHO) (5, 6). On 8 November 2021, Center for Systems Science and Engineering (CSSE) at John Hopkins University confirmed around 250,799,409 cases so far throughout the world including 5,063,295 global deaths (https://coronavirus.jhu.edu/). COVID-19 patients may present with various clinical manifestations from an asymptomatic infection to a mild condition or acute respiratory distress syndrome (ARDS) and dysfunction, in different organs mainly the liver, bowel and kidneys (7, 8). Within most cases, fever, sore throat, cough, fatigue, dyspnea, sputum production, myalgia, fatigue, and headache occur. While such patients most commonly present with severe respiratory disease, it has been reported that about 19% of COVID-19 patients display gastrointestinal (GI) symptoms (1) including 2–10% diarrhea, 1–3.6% loss of appetite, nausea, vomiting, and abdominal pain that are increasingly being recognized as important symptoms of COVID-19 (9, 10). The virus has been detected in different parts of the patients' GI tract as well as the feces, suggesting the importance of the gut tropism in the pathogenesis and dissemination of this horrible pandemic (11). The overuse of empirical antimicrobial agents in COVID-19 patients increase the risk of microbiota alterations, antibiotic-associated diarrhea and Clostridioides difficile infection (CDI). Therefore, involvement of the GI tract in COVID-19 warrants attention of clinicians and researchers. This article also gives an update of the recent evidences on the gut microbiota disruption during COVID-19 and on the occurrence of CDI.
SARS-CoV-2 Structure
SARS-CoV-2 is an enveloped, non-segmented, positive-sense single-stranded RNA (+ssRNA) virus that belongs to the genus Coronavirus, the family Coronaviridae, and the order Nidovirales (12, 13). Genome sequencing has indicated that the virus shows ~80% similarity to severe acute respiratory syndrome (SARS)-like coronaviruses (SARS-like CoVs) and 50% similarity with Middle East respiratory syndrome coronavirus (MERS-CoV) (14, 15). Structurally, the genome of SARS-CoV-2, with full length of 29,903 bp, is composed of four major structural protein-coding genes namely, spike (S) glycoprotein, envelope (E) glycoprotein, membrane (M) glycoprotein, and nucleocapsid (N) proteins (16). Non-structural proteins are encoded by the open reading frame (ORF) region that consist of replicase complex, which includes components such as 3-chymotrypsin-like protease, papain-like protease, and RNA-dependent RNA polymerase (17) (Figure 1).
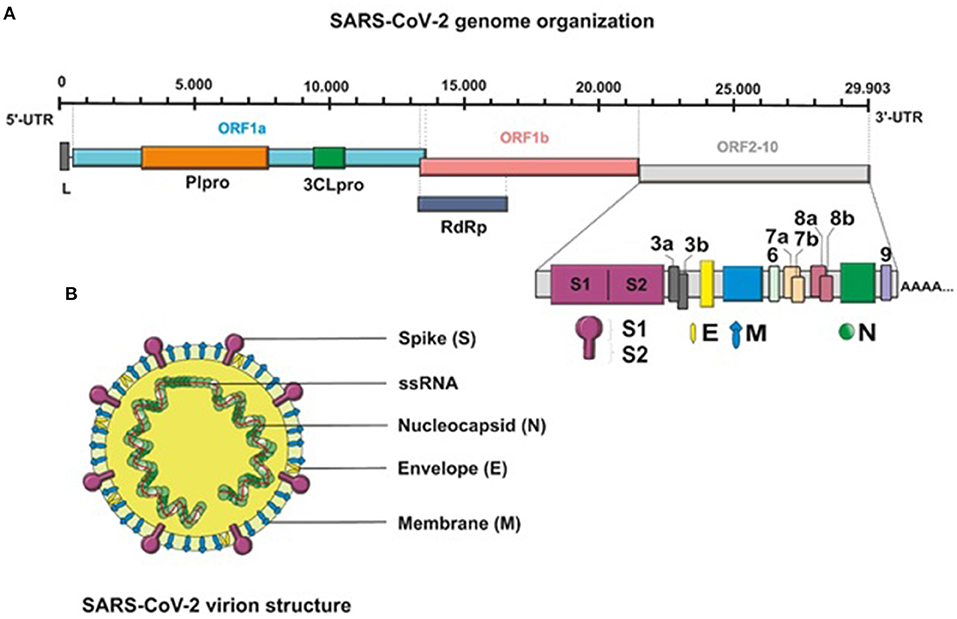
Figure 1. (A) Schematic presentation of the full-length genomic RNA (29,903 nt), and transcribed canonical subgenomic RNAs (sgRNA) of SARS-CoV-2 virus. In addition to the genomic RNA that also serves as an mRNA encoding two overlapping ORFs, ORF1a and ORF1b, nine major subgenomic RNAs are produced. (B) Schematic structure of the SARS-CoV-2 virion and its major structural proteins.
COVID-19 Pathogenesis
Extensive research around the world has led to a better understanding of the pathogenesis of COVID-19, and development of potential treatments and vaccines against it. Several studies have established that SARS-CoV-2 requires the angiotensin converting enzyme 2 (ACE2) as a major entry receptor (14, 15, 18–20). ACE2 protein, a type I integral metallocarboxypeptidase, is mainly expressed in epithelial and endothelial cells in renal, kidney, lung parenchyma and the GI tract (21). It was shown that glycosylated S proteins, as the most significant transmembrane protein in the outer portion of the virus, bind to the host cell receptor ACE2 and mediate direct binding of virus to the host cells (22, 23). This initial step is an important part of the pathogenesis of COVID-19 infection. Besides, the cleavage of the S proteins by transmembrane protease serine type 2 (TMPRSS2) to subunit S1 and S2 regulates viral uptake and allows the cellular membranes fusion of virus and host cell (24–27). Thus, co-expression of ACE2 and TMPRSS2 is thought to be essential for viral entry into the host cells. Binding to the cellular receptor and inducing membrane fusion lead to the release of uncoated viral RNA genome into the host cell followed by transcription of the sub-genomic RNAs and subsequently, initiation of viral genome replication cycle (12, 28, 29) (Figure 2).
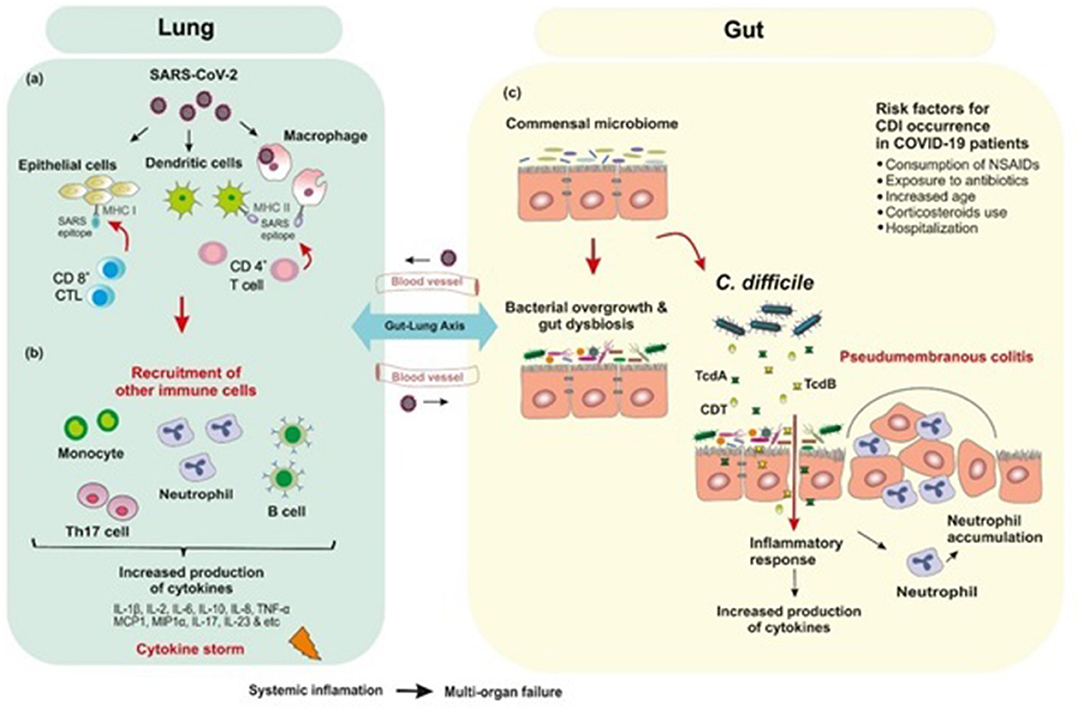
Figure 2. Postulated schematic diagram of the interplay of human gut microbiota and SARS-CoV-2 with bacterial coinfections. (a) SARS-CoV-2 binds to ACE2 receptor which mediates direct binding of virus to the human lungs and evokes an immune response. (b) Inflammation occurs through the immune responses of both innate and adaptive immune system. CD8+ cytotoxic T cells which are able to secret a cluster of molecules are essential in the eradication of virus infected cells. CD4+ helper T cells facilitate the overall adaptive response by assisting cytotoxic T cells. B-cell mediated humoral immune response plays a protective role by producing neutralizing antibodies, and also impedes re-infection. SARS-CoV-2 infection triggers the release of inflammatory cytokines and chemokines, which lead to the recruitment of neutrophils and other immune cells. (c) Empirical antibiotic therapy for bacterial infections in hospitalized COVID-19 patients are associated with depletion of beneficial commensals and enrichment of opportunistic pathogens such as Clostridioides difficile infection. The immune response to C. difficile is similar to that produced against SARS-CoV-2 in which inflammatory cytokines are upregulated.
Immune Response to SARS-CoV-2 Infection
Evidence from genetic analyses indicates that inflammation plays a key role in COVID-19 severity (30). Inflammation occurs through the immune responses of both innate and adaptive immune system; however, it is not clear how this response occurs in SARS-CoV-2 infections. Initially, alveolar epithelial cells, macrophages, and circulating monocytes utilize pattern recognition receptors (PRRs) to detect virus-associated products, this step is followed by recruitment of more immune cells and production of high levels of pro-inflammatory cytokines and chemokines (31). Researchers have hypothesized that the overproduction of early-response pro-inflammatory cytokines such as Interleukin-1β (IL1β), IL-2, IL-6, IL-8, IL-10, tumor necrosis factor-α (TNF-α), interferon γ (IFN-γ), granulocyte colony stimulating factor (G-CSF), monocyte chemoattractant protein-1 (MCP1, also known as CCL2), macrophage inflammatory protein 1 alpha (MIP1α, also known as CCL3), CXC-chemokine ligand 10 (CXCL10), D-dimer, erythrocyte sedimentation rate (ESR), and C-reactive peptide (CRP), in the majority of non-surviving COVID-19 patients contribute to cytokine release syndrome (CRS) or “cytokine storm-induced ARDS”, sepsis, multiple-organ failure and death (20, 30, 32). Among the inflammatory mediators increased in COVID-19 patients, IL-6 blood levels are noticeably highly correlated with mortality (20). Furthermore, there are some reports about the role of T helper 17 (Th17) type cytokine storm in patients with severe COVID-19 (33). Strong Th17 and T-helper 1 (Th1) responses or induced IL-17-related pathways have also been reported in other pulmonary viral infections including MERS-CoV, SARS-CoV, and H1N1 influenza (33, 34) (Figure 2).
COVID-19 and Gut Microbiota
Alteration of fecal microbiota composition in COVID-19 patients are known to be associated with the enrichment of opportunistic pathogens and depletion of beneficial commensals. Zuo et al. revealed that hospitalized patients with COVID-19 can experience a prolonged dysbiosis in their gut microbiome which was associated with SARS-CoV-2 fecal shedding and disease severity (35). This study showed that several opportunistic pathogens such as Coprobacillus, Clostridium ramosum, and Clostridium hathewayi were enriched in the gut microbiome of patients with COVID-19. Also, higher abundance of C. hathewayi and inverse correlation between abundance of Faecalibacterium prausnitzii at the baseline of disease was associated with COVID-19 severity. Nevertheless, over the course of hospitalization, existence of beneficial commensals such as Bacteroides dorei, Bacteroides thetaiotaomicron, Bacteroides massiliensis, and Bacteroides ovatus, which led to downregulation of ACE2 expression in murine gut, inversely correlated with SARS-CoV-2 load in patients' fecal samples. Thus, it may be speculated that GI tract disease symptoms could be related to the changes in the intestinal microbiome during COVID-19 (35–38). In addition to the bacteria listed above, the prevalence of Clostridioides difficile is also dependent on the balance of intestinal microbiota. Recently, it has been shown that disturbing the balance of the gut microbiome called “intestinal dysbiosis” is directly associated with increased risk of C. difficile infection (CDI), which in turn, leads to development of intestine-related diseases (39, 40). Furthermore, it has been documented that long-lasting conventional antibiotic administration such as clindamycin, cephalosporins, penicillin, and fluoroquinolones affects the biomass, composition and function of the gut microbiota and consequently reduce colonization resistance capacity against opportunistic pathogens such as C. difficile, and subsequently develop CDI (41, 42). Thus, during the current pandemic, clinicians should be vigilant of coinfections in COVID-19 patients with C. difficile (Figure 2).
CDI vs. COVID-19: Comparison of Clinical Manifestations
CDI is an important global infectious disease caused by a toxin-releasing obligate anaerobic organism after long-term use of broad-spectrum antibiotics, absence of antimicrobial stewardship and hospital overcrowding (43–45). Besides, C. difficile bacteria produce two major exotoxins, toxin A (TcdA) and toxin B (TcdB), which are both glucosylating toxins responsible for CDI symptoms, including intestinal injury, mucosal inflammation and marked neutrophil recruitment. TcdA and TcdB cause actin cytoskeleton disaggregation, caspases activation, and intestinal cell damage by inactivation of Rho family GTPases (Rho, Rac and Cdc42) (46, 47). CDI spectrum varies from asymptomatic carriage, mild and moderate diarrhea to pseudomembranous colitis, severe fulminant colitis, toxic megacolon, and death, particularly in elderly patients (48, 49).
Annual mortality rate of CDI is high in Europe (8,382 deaths in year) and the United States (29,000 deaths in year) (50). Various risk factors for CDI have been reported such as consumption of different antibiotics, elderly (>65 years), long-term hospitalization, chemotherapy, comorbidities, and chronic kidney and GI disorders (51, 52). It should be noted that these are also factors for COVID-19 which may cause an overlap, and highlights the importance of considering coinfections. The severity of COVID-19 is under the influence of factors such as advanced age (over 65 years) and underlying chronic conditions including chronic respiratory diseases, hypertension, diabetes mellitus, malignancy, and cardiovascular disease (9, 53). Similar GI symptoms including diarrhea, nausea, vomiting and abdominal pain observed in the two diseases would increase the importance of bacterial coinfection in COVID-19 patients.
The cytokines released from immune system during CDI is almost similar to that produced in patients with the severe form of COVID-19. Upregulation of many cytokines mainly IL-1β, IL-6, IL-8, IL-17A, and IL-16, were shown in CDI patients (54). Moreover, the IL-1β/Th17 axis plays a key role in producing inflammatory responses in CDI (54). Notably, elevated Th17 responses affect CDI-associated mortality by increasing IL-17 production. A previous report revealed an association between Th17 cytokines IL-6 and IL-23 and CDI severity (33, 55). These findings demonstrate the key effects of Th17 cells on CDI severity. The role of Th17 cells in patients with severe COVID-19 has also been demonstrated (33).
Risk Factors Affecting the Occurrence of CDI in COVID-19 Patients
Possible Risk Factors for C. difficile Coinfection
Several studies have confirmed that secondary bacterial infections can increase mortality of the respiratory diseases. During the influenza epidemics, bacterial coinfection was shown to increase the mortality rate of infected-patients (56, 57). Moreover, bacterial infections in intensive care unit (ICU) patients with MERS-CoV were also observed (56). Nonetheless, a limited number of studies have discussed the coinfection of SARS-CoV-2 with bacterial pathogens. This has led to neglect, or underestimation, of the role of bacteria in COVID-19 patients due to the complexity of the symptoms caused by the virus (58).
Some studies have suggested COVID-19 as a possible risk factor for the development of nosocomial infections from C. difficile and other multidrug-resistant (MDR) bacteria. Bentivegna et al. (59, 60) showed that during 2020 the use of infection prevention and control (IPC) measures gave rise to a significant decreased of this type of infections in medical departments. Nevertheless, this was not observed in COVID-19 patients where, although not reaching statistical significance, CDI and other nosocomial MDR bacterial infections increased. Since IPC measures were even stricter in the COVID-19 group this apparently conflicting finding opens up several possible interpretations. Some authors, as already mentioned, have suggested the alteration of the intestinal flora in COVID-19 patients as a possible motivation for the increase of CDI (35).
Other possible interpretations suggest the widespread use of broad-spectrum antibiotics in COVID-19 patients as the possible cause of selections and spread of MDR bacteria (61). Impaired immune system, other peculiarities of subjects who develop severe forms of COVID-19 that require hospitalization (62–65), could be another important risk factor for developing CDI. Furthermore, in the hospital unit from which the study data came, COVID-19 patients are mostly managed by infectious disease specialists, who are more likely to perform CD research and culture tests in case of clinical suspicion. Other explanation finds the cause in the intrinsic characteristics of the hospitalized COVID-19 patients itself that are often with pluri-organic comorbidities and several previous hospitalization that could have led to colonization by MDR bacteria. Here, we describe in detail possible risk factors for C. difficile coinfection during COVID-19.
Consumption of NSAIDs
Non-steroidal anti-inflammatory drugs (NSAIDs) such as aspirin, ibuprofen, celecoxib, and indomethacin are widely used for mitigation of pain, inflammation, and fever (48, 66–68). At the early stages of COVID-19, consumption of NSAIDs can help to reduce the fever as the most common symptom of the infected patients. The use of anti-inflammatory drugs and immunomodulators may potentially enhance the prevalence of opportunistic bacteria and predispose development of bacterial coinfections (69, 70). Additionally, it is most likely that elderly (above 65 years old), as an important target population for CDI, are often on multiple medications such as NSAIDs (71). These medications exert their pharmacological effects through reducing the production of prostaglandins (PGs) via inhibition of cyclooxygenase enzymes (COX-1 and COX-2), and modulation of host inflammatory response (72, 73). There are increasing reports indicating the plausibility of an association between use of NSAIDs and increased risk of severe manifestations in CDI (74). Muñoz-Miralles et al. reported that indomethacin-administered, antibiotic-treated mice infected with C. difficile demonstrated enhanced intestinal inflammation, weight loss, and presented the signs of severe infection and worsened histopathological damage (75). They concluded that NSAIDs can have a negative impact on antibiotic-associated CDI, and proposed that targeting the biosynthesis or signaling pathways of prostaglandins might be a promising approach to ameliorate the severity of CDI. Moreover, similar results were obtained by Mesada et al. regarding the deteriorate effects of indomethacin exposure on alteration of the gut microbiota and exacerbation of C. difficile colitis (76). It was also hypothesized by Noori et al. that the NSAID indomethacin is able to induce the expression of prostaglandin E2-inactivating enzyme named 15-hydroxyprostaglandin dehydrogenase (Hpgd, 15-PGDH), which may result in enhanced PGE2 production (77). Furthermore, the therapeutic efficacy, disposition (absorption, distribution, metabolism, and excretion), toxicity and enteropathy of NSAIDs are influenced by the dynamic crosstalk between host cells and gut microbiota (69, 78, 79). On the other hand, NSAIDs can directly alter the structure and functional profiles of the gut microbiota or indirectly impact the physiological status of the host which may, in turn, cause intestinal dysbiosis (80).
Besides, a possible association was reported between NSAIDs, especially diclofenac, and diarrhea among patients with Clostridium difficile associated disease (CDAD) that were not hospitalized or exposed to antimicrobial agents (81–83). Suissa et al. found consumption of diclofenac is related with 35% increase in the risk of developing CDAD (81).
Antibiotic Therapy
The rapid spread of SARS-CoV-2 increased the need to develop effective antiviral agents against COVID-19. Given that there are no clinically approved drugs available for SARS-CoV-2 infection, clinicians have to consider various agents such as antiviral peptides (remidesivir, ribavirin, and favipiravir), antibiotics (azithromycin, doxycycline, clindamycin, cephalosporins, and fluoroquinolone), and different treatment strategies like convalescent plasma transfusion therapy, heparin therapy for improving hypoxia, and also corticosteroids to modulate the inflammation response (84–86). Patients with COVID-19 mostly present with clinical manifestations including fever, cough and lung infiltrates, which resemble bacterial pneumonia. Since cough, fever and radiological infiltrates are common symptoms of pneumonia, appropriate antimicrobial therapy may be required during the hospital stay (87, 88). However, the rate of bacterial coinfection at admission in patients with COVID-19 is extremely low, thus not justifying the extensive use of antibiotics. Therefore, antibiotics should be given only if there is a suspicion of bacterial pneumonia, not because of fever and radiological infiltrates during COVID-19. One of the main causes of bacterial coinfection is the disturbance of microbial balance of the GI tract due to antibiotic exposure and the growth of opportunistic pathogens, such as Staphylococcus aureus, Streptococcus pyogenes, and Pseudomonas aeruginosa (89).
Generally, maintaining the microbial balance of the GI tract plays an important role in the health of each individual. Furthermore, the indiscriminate use of antibiotics changes the overall diversity and composition of the gut microbiota and disrupts the microbial balance. Disruption of the gut microbiota caused by antibiotics is a risk factor for CDI; however, the entry of C. difficile is due to poor infection control measures in the healthcare setting. This is the cause of outbreaks (90, 91). Given that the antibiotic use is a triggering factor associated with the development of CDI, it might be one of the probable consequences of antimicrobial agent's consumption against COVID-19. Also, symptoms of diarrhea caused by C. difficile were reported in some patients with COVID-19 (92). This study reported 9 cases of coinfection with COVID-19 and CDI in elderly hospitalized patients who had received antibiotics. In addition, the results of this work showed that the rate of CDI increased from 3.32/10,000 to 3.60/10,000 patient-days during January–April 2020. Examination of patients with C. difficile and COVID-19 coinfection showed that both can cause GI symptoms, thus, it is recommended to screen diarrheal patients for CDI during the coronavirus disease pandemic (1). Furthermore, it has been reported that CDI complicates COVID-19 severity, mainly in patients with co-morbidities and previous healthcare exposures (87). These findings once again highlight the significance of infection prevention and control measures, and also antimicrobial stewardship programmers, especially in the management of COVID-19 crisis.
Older Age
Current epidemiological studies denote comorbidities are significantly associated to increased virus susceptibility and burden of COVID-19 disease (93). As comorbidities often increase with aging, elderly patients are more susceptible to COVID-19 (median age at death 75 years) and may in turn experience a more severe COVID-19 (94). It is also noteworthy that elderly patients over 65 years of age represent approximately 80% of hospitalizations and higher death rates (23-fold) than younger people (95–97). According to the current epidemiological data, the risk of death among SARS-CoV-2-infected patients with comorbidities over 80 years old was greater than younger-aged groups (20, 87, 98–100). A study in China reported that the COVID-19 mortality rate in older patients (>60 years) was significantly higher than that of the younger patients (5.3 vs. 1.4%) (86). A similar study in Italy, as a country most severely affected by COVID-19 pandemic, confirmed the effect of age on the mortality rate of COVID-19 (1). Age-related structural, physiological, and immunological changes of the respiratory system showed that aging itself has been strongly associated with worse health outcomes in COVID-19 patients (94). Moreover, COVID-19 pandemic demonstrated that older people (over 60 years) are not only at a higher risk for severe disease, but they may also be at risk of ignoring their antecedent chronic conditions or being lost to follow up (101). Previous studies suggested that possible decrease in ACE2 expression induced by the SARS-CoV-2 infection in the majority of elderly patients especially those with hypertension may up-regulate angiotensin II, and exacerbate pro-inflammatory conditions, leading to greater COVID-19 severity and mortality (102, 103). Given the disproportionate impact of COVID-19 on older people, age is clearly the most important factor for death due to COVID-19 (104–106). Antibiotic exposure and advanced age (age >65 years old) are two established risk factors for developing CDI (107, 108). It is well known that CDI, is the most important cause of acute diarrhea in all age groups, but it disproportionately affects elderly resulting in increased risk of morbidity and mortality among this compromised population (107, 109). The predisposing risk factors which may be specific to older adults are multifactorial, and include frequent contacts with healthcare systems and age-associated alterations in host physiology such as waning immunity (immunosenescence) and probably altered intestinal microbiota (110, 111).
Discussion
Despite well-defined recommendation from the WHO for the use of broad-spectrum empirical antibiotics in hospitalized patients with COVID-19 who suffer from bacterial co/superinfections, there had been unjustifiable use of antimicrobials in these patients, especially among those with asymptomatic or mild-to-moderate illness (112). Since pulmonary bacterial super-infections seems to be mostly nosocomial and were associated with receipt of empirical antimicrobial therapy (113), disequilibrium in the composition of human gut microbiota following inappropriate antibiotic consumption might play a prominent role in the emergence of several opportunistic pathogens specially C. difficile in longitudinal and high-quality respiratory samples. This is a matter of worrisome when the majority of asymptomatic or hospitalized symptomatic patients with COVID-19 (nearly 72%) were treated with broad-spectrum antibiotics mostly quinolones to prevent bacterial co/superinfections (20, 57, 114). Also, approximately 75% of long-term care facility (LTCF) residents for 6 months or longer will receive at least one course of antibiotics (115). Indeed, alteration of the intestinal microbiome of COVID-19 patients who are treated with broad-spectrum antibiotics, is reported to be associated with escalated clinical status such as proinflammatory conditions, disease severity, fecal shedding of the virus, plasma concentrations of several cytokines and finally drastic long-term effects on host health (35, 116, 117). These traceable alterations of the microbiome of COVID-19 patients following the use of antibiotics can lead to dysbiosis of the microbiome and CDI (118). The use of antibiotics in COVID-19 pneumonia increases the risk of antibiotic-associated diarrhea (AAD) and CDI. Sandhu et al. recently reported a case series of nine patients with coinfection of SARS-CoV-2 and C. difficile in hospitalized elderly female patients which were treated with antibiotics. This study emphasizes the unintended consequences of judicious administration of antibiotics throughout the disease course of COVID-19 and bacterial infection such as CDI (92). Additionally, COVID-19 is an illness with frequent GI symptoms such as nausea, vomiting and diarrhea that induces changes in the gut microbiota composition of infected patients, which can complicate the diagnosis of CDI owing to lack of clinical suspicion (119). Not only these patients are more susceptible to CDI, but also antibiotics may induce emergence of new virulent strains of C. difficile, which in turn will produce severe consequences in such patients (1). The onset of GI symptoms in COVID-19 patients can be related to the presence of ACE2 receptor and TMPRSS2 in the esophageal upper epithelial, ileum and colon (1), which as previously mentioned, are involved in cell entry of SARS-CoV-2 and its pathogenesis. Studies have shown that ACE2 is the target of SARS-CoV-2 (27, 120) which is highly expressed in colonocytes of patients with inflammatory bowel disease and can regulate amino acid transport, microbial ecology, and inflammation in the gut and create conditions favorable for coronaviruses infection (11, 120). It seems that for prevention of delayed diagnosis of CDI, both CDI and COVID-19 should be considered in patients with GI symptoms (92). Even if GI symptoms are not seen, the possibility of CDI should be considered because of the extensive use of antibiotics. In addition, damage to the gut caused by COVID-19 may facilitate bacterial infection, especially in patients who are susceptible to CDI. Interestingly, some reports showed that beneficial bacterial species are negatively correlated with COVID-19 severity. It has been shown that in bacterial and viral co-infection, commensal species which are able to downregulate ACE2 expression may become potential targets for alleviating immune alterations leading to less severe cases of COVID-19 (121).
Previous data presented that gut microbiome dysbiosis in high-risk groups like those with diabetes, kidney, obesity, autoimmune, and aging-related diseases is often an underlying condition and that GI symptoms are often indicative of severe COVID-19 complication (122). Interestingly, hypovitaminosis D was linked to adverse events in patients with lung, heart, kidney diseases, CDI, and SARS-CoV-2 infection (123–125). Immunological findings confirmed vitamin D involvement in the entire cascade of host responses toward infectious diseases (124, 125). In this regard previous studies have indicated that vitamin D inhibits TNF-α, IFN-γ, IL-1, IL-6, and IL-17, by reducing the p38 MAP kinase activation in human monocytes/macrophages and enhancing the expression level of T-regulatory cells, Th2, M2 macrophages, and IL-10 (126–131). Notably, C. difficile stimulate production of inflammatory cytokines such as IL-8, IL-6, IL-1β, TNF-α, COX-2, and PE2, which are suggested playing crucial roles in CDI pathogenesis as well as SARS-CoV-2 infection (132–134). Given that, vitamin D can help prevent upper respiratory tract infections or reduce the severity of them through increasing the immune modulatory activity by inhibition of transcription level of proinflammatory cytokines such as TNF-α, IFN-γ, IL-17, IL-2, and IL-21 (125, 135, 136).
The fecal-oral transmission is one way of shedding C. difficile into the environment, which has been confirmed in previous studies (137, 138). Noteworthy, the presence of coronaviruses in GI tract of infected humans has been also proven, so that there are some studies about isolation of live SARS-CoV-2 virus from fecal sample of patients with SARS-CoV-2 respiratory tract infection (139). CDI patients possibly may have facilitated the persistence of SARS-CoV-2 in feces almost a month, even after the nasopharyngeal test turned negative. This coinfection might exacerbate the GI signs and symptoms and raise possibility of fecal-oral transmission of SARS-CoV-2 and C. difficile (119, 140). Since SARS-CoV-2 virus can be potentially transmitted through fecal matters even in asymptomatic patients, CDI may complicate COVID-19 and lead to higher rate of mortality (119). Thus, it is needed to clarify the interactions between COVID-19 and CDI. Notably, adherence to health principles including health education and publicity, environmental health and personal hygiene, frequent hand washing, disinfection of surfaces, might become more important in COVID-19 pandemic to prevent occurrence of co-infection (87) and this concern has been addressed by some studies in which, it was emphasized that when CDI is present as a co-infection with COVID-19 monitoring of CDI therapy can be difficult if diarrhea persists because of COVID-19 (59). In addition to the aforementioned points, it is important to note that patients taking proton pump inhibitors (PPIs) are exposed to severe risk of clinical outcomes of COVID-19 (98). Furthermore, there is a statistically significant association between the use of gastric acid suppressors, including PPIs and histamine-2 receptor antagonists (H2RAs) and CDI (47, 99, 100). Since scarce data are available in this context, paying attention to patients receiving PPIs during COVID-19 pandemic may help prevention of CDI coinfection. All of these pieces of evidence highlight the importance of paying attention to CDI during the COVID-19 pandemic. Also, there must be high suspicion for CDI in COVID-19 patients with persistent diarrhea.
Conclusion
The evidence is available on COVID-19 and CDI coinfection; CDI should be monitored in COVID-19 patients for a better management of the disease. In this regard, we highlight the following risk factors for CDI in COVID-19 patients: (1) The detrimental effect on the gut microbiota caused by antibody therapy in COVID-19 patients should be monitored. (2) Patients with COVID-19 infection who take antibiotics for a long time in the hospital settings or at home may be susceptible to develop CDI. (3) Patients with acute COVID-19 infections who have to be hospitalized for a long time should be monitored for CDI. (4) Adherence to good healthcare practices in medical centers should be considered for COVID-19 patients presenting diarrhea. (5) The importance of CDI diagnosis in patients with GI symptoms to prevent the misdiagnosis of COVID-19 infection. (6) CDI should be considered in COVID-19 patients treated with anti-inflammatory drugs.
Taken together, this review underlines the importance of infection prevention and rational use of antibiotics in the management of patients with COVID-19. Antibiotic treatment can be one of the main causes of this problem, thus, surveillance on antibiotic administration should be incorporated in COVID-19 management.
Author Contributions
MA, MN, HR, and AY prepared the draft of the manuscript. MN designed the figures. AY, EB, PM, NP, SS, HAA, and MRZ reviewed and revised the manuscript. All authors read and approved the final version of the manuscript and the author list.
Funding
This work was supported by Foodborne and Waterborne Diseases Research Center, Research Institute for Gastroenterology and Liver Diseases, Shahid Beheshti University of Medical Sciences, Tehran, Iran.
Conflict of Interest
The authors declare that the research was conducted in the absence of any commercial or financial relationships that could be construed as a potential conflict of interest.
Publisher's Note
All claims expressed in this article are solely those of the authors and do not necessarily represent those of their affiliated organizations, or those of the publisher, the editors and the reviewers. Any product that may be evaluated in this article, or claim that may be made by its manufacturer, is not guaranteed or endorsed by the publisher.
Acknowledgments
The authors would like to thank the staff of Foodborne and Waterborne Diseases Research Center at the Research Institute for Gastroenterology and Liver Diseases, Shahid Beheshti University of Medical Sciences, Tehran, Iran.
References
1. Spigaglia P. COVID-19 and clostridioides difficile infection (CDI): possible implications for elderly patients. Anaerobe. (2020) 64:102233. doi: 10.1016/j.anaerobe.2020.102233
2. Neurath MF. Covid-19 and immunomodulation in IBD. Gut. (2020) 69:1335–42. doi: 10.1136/gutjnl-2020-321269
3. Zhu N, Zhang D, Wang W, Li X, Yang B, Song J, et al. A novel coronavirus from patients with pneumonia in China, 2019. N Eng J Med. (2020) 382:727–33. doi: 10.1056/NEJMoa2001017
4. Nakase H, Matsumoto T, Matsuura M, Iijima H, Matsuoka K, Ohmiya N, et al. Expert opinions on the current therapeutic management of inflammatory bowel disease during the covid-19 pandemic: Japan ibd covid-19 taskforce, intractable diseases, the health and labor sciences research. Digestion. (2021) 102:814–22. doi: 10.1159/000510502
5. WHO Organisation. WHO Director-General's Opening Remarks at the Media Briefing on COVID-19-11 March 2020. Retrieved from: https://www.who.int/dg/speeches/detail/who-director-general-s-opening-remarks-atthemedia-briefing-on-covid-19-−11-march-2020 (accessed March 11, 2020).
6. Iacucci M, Cannatelli R, Labarile N, Mao R, Panaccione R, Danese S, et al. Endoscopy in inflammatory bowel diseases during the COVID-19 pandemic and post-pandemic period. Lancet Gastroenterol Hepatol. (2020) 5:598–606. doi: 10.1016/S2468-1253(20)30119-9
7. Naderpour Z, Saeedi M. A primer on COVID-19 for clinicians: clinical manifestation and natural course. Adv J Emerg Med. (2020) 44:e62. doi: 10.22114/ajem.v4i2s.418
8. Shirani K, Sheikhbahaei E, Torkpour Z, Nejad MG, Moghadas BK, Ghasemi M, et al. A narrative review of COVID-19: the new pandemic disease. Iran J Med Sci. (2020) 45:233–49. doi: 10.30476/ijms.2020.85869.1549
9. Ramachandran P, Onukogu I, Ghanta S, Gajendran M, Perisetti A, Goyal H, et al. Gastrointestinal symptoms and outcomes in hospitalized coronavirus disease 2019 patients. Dig Dis. (2020) 38:373–9. doi: 10.1159/000509774
10. Zhang H, Kang Z, Gong H, Xu D, Wang J, Li Z, et al. Digestive system is a potential route of COVID-19: an analysis of single-cell coexpression pattern of key proteins in viral entry process. Gut. (2020) 69:1010–8. doi: 10.1136/gutjnl-2020-320953
11. Xiao F, Tang M, Zheng X, Liu Y, Li X, Shan H. Evidence for gastrointestinal Infection of SARS-CoV-2. Gastroenterology. (2020) 158:1831–3.e3. doi: 10.1053/j.gastro.2020.02.055
12. Guo YR, Cao QD, Hong ZS, Tan YY, Chen SD, Jin HJ, et al. The origin, transmission and clinical therapies on coronavirus disease 2019 (COVID-19) outbreak–an update on the status. Mil Med Res. (2020) 7:11. doi: 10.1186/s40779-020-00240-0
13. Astuti I. Ysrafil. Severe acute respiratory syndrome coronavirus 2 (SARS-CoV-2): an overview of viral structure and host response. Diabetes Metab Syndr. (2020) 14:407–12. doi: 10.1016/j.dsx.2020.04.020
14. Zhou P, Lou YX, Wang X, Hu B, Zhang L, Zhang W, et al. Pneumonia outbreak associated with a new coronavirus of probable bat origin. Nature. (2020) 579:270–3. doi: 10.1038/s41586-020-2012-7
15. Lu R, Zhao X, Li J, Niu P, Yang B, Wu H, et al. Genomic characterisation and epidemiology of 2019 novel coronavirus: implications for virus origins and receptor binding. Lancet. (2020) 395:565–74. doi: 10.1016/S0140-6736(20)30251-8
16. Jiang S, Hillyer C, Du L. Neutralizing antibodies against SARS-CoV-2 and other human coronaviruses. Trends Immunol. (2020) 41:545. doi: 10.1016/j.it.2020.04.008
17. Chan JF, Kok KH, Zhu Z, Chu H, To KK, Yuan S, et al. Genomic characterization of the 2019 novel human-pathogenic coronavirus isolated from a patient with atypical pneumonia after visiting Wuhan. Emerg Microbes Infect. (2020) 9:221–36. doi: 10.1080/22221751.2020.1719902
18. Hoffmann M, Kleine-Weber H, Schroeder S, Krüger N, Herrler T, Erichsen S, et al. SARS-CoV-2 cell entry depends on ACE2 and TMPRSS2 and is blocked by a clinically proven protease inhibitor. Cell. (2020) 181:271–80.e8. doi: 10.1016/j.cell.2020.02.052
19. Letko M, Marzi A, Munster V. Functional assessment of cell entry and receptor usage for SARS-CoV-2 and other lineage B betacoronaviruses. Nat Microbiol. (2020) 5:562–9. doi: 10.1038/s41564-020-0688-y
20. Zhou F, Yu T, Du R, Fan G, Liu Y, Liu Z, et al. Clinical course and risk factors for mortality of adult inpatients with COVID-19 in Wuhan, China: a retrospective cohort study. Lancet. (2020) 395:1054–62. doi: 10.1016/S0140-6736(20)30566-3
21. Gheblawi M, Wang K, Viveiros A, Nguyen Q, Zhong JC, Turner AJ, et al. Angiotensin-converting enzyme 2: SARS-CoV-2 receptor and regulator of the renin-angiotensin system: celebrating the 20th anniversary of the discovery of ACE2. Circ Res. (2020) 126:1456–74. doi: 10.1161/CIRCRESAHA.120.317015
22. Fehr AR, Perlman S. Coronaviruses: an overview of their replication and pathogenesis. Methods Mol Biol. (2015) 128:1–23. doi: 10.1007/978-1-4939-2438-7_1
23. Duan L, Zheng Q, Zhang H, Niu Y, Lou Y, Wang H. The SARS-CoV-2 spike glycoprotein biosynthesis, structure, function, and antigenicity: implications for the design of spike-based vaccine immunogens. Front Immunol. (2020) 11:576622. doi: 10.3389/fimmu.2020.576622
24. Wu F, Zhao S, Yu B, Chen YM, Wang W, Song ZG, et al. A new coronavirus associated with human respiratory disease in China. Nature. (2020) 579:265–9. doi: 10.1038/s41586-020-2008-3
25. Harrison AG, Lin T, Wang P. Mechanisms of SARS-CoV-2 transmission and pathogenesis. Trends Immunol. (2020) 41:1100–15. doi: 10.1016/j.it.2020.10.004
26. Gallagher TM, Buchmeier MJ. Coronavirus spike proteins in viral entry and pathogenesis. Virology. (2001) 279:371–4. doi: 10.1006/viro.2000.0757
27. Wang Q, Zhang Y, Wu L, Niu S, Song C, Zhang Z, et al. Structural and functional basis of SARS-CoV-2 entry by using human ACE2. Cell. (2020) 181:894–904.e9. doi: 10.1016/j.cell.2020.03.045
28. Belouzard S, Millet JK, Licitra BN, Whittaker GR. Mechanisms of coronavirus cell entry mediated by the viral spike protein. Viruses. (2012) 4:1011–33. doi: 10.3390/v4061011
29. Masters PS, Perlman S. Coronaviridae. In: Knipe DM, Howley PM, Cohen JI, editors. Fields Virology, 6th ed. Philadelphia: Lippincott Williams and Wilkins (2013). p.825.
30. Shen B, Yi X, Sun Y, Bi X, Du J, Zhang C, et al. Proteomic and metabolomic characterization of COVID-19 patient sera. Cell. (2020) 182:59–72.e15. doi: 10.1016/j.cell.2020.05.032
31. Mortaz E, Tabarsi P, Varahram M, Folkerts G, Adcock IM. The immune response and immunopathology of COVID-19. Front Immunol. (2020) 11:2037. doi: 10.3389/fimmu.2020.02037
32. Meduri GU, Headley S, Kohler G, Stentz F, Tolley E, Umberger R, et al. Persistent elevation of inflammatory cytokines predicts a poor outcome in ARDS: plasma IL-1β and IL-6 levels are consistent and efficient predictors of outcome over time. Chest. (1995) 107:1062–73. doi: 10.1378/chest.107.4.1062
33. Wu D, Yang XO. TH17 responses in cytokine storm of COVID-19: an emerging target of JAK2 inhibitor Fedratinib. J Microbiol Immunol Infect. (2020) 53:368–70. doi: 10.1016/j.jmii.2020.03.005
34. Josset L, Menachery VD, Gralinski LE, Agnihothram S, Sova P, Carter VS, et al. Cell host response to infection with novel human coronavirus EMC predicts potential antivirals and important differences with SARS coronavirus. MBio. (2013) 4:e00165–13. doi: 10.1128/mBio.00165-13
35. Zuo T, Zhang F, Lui GC, Yeoh YK, Li AY, Zhan H, et al. Alterations in gut Microbiota of patients with COVID-19 during time of hospitalization. Gastroenterology. (2020) 159:944–55.e8. doi: 10.1053/j.gastro.2020.05.048
36. Yamamoto S, Saito M, Tamura A, Prawisuda D, Mizutani T, Yotsuyanagi H. The human microbiome and COVID-19: A systematic review. PLoS ONE. (2021) 16:e0253293. doi: 10.1371/journal.pone.0253293
37. Chattopadhyay I, Shankar EM. SARS-CoV-2-indigenous microbiota nexus: does gut microbiota contribute to inflammation and disease severity in COVID-19? Front Cell Infect Microbiol. (2021) 11:590874. doi: 10.3389/fcimb.2021.590874
38. Geva-Zatorsky N, Sefik E, Kua L, Pasman L, Tan TG, Ortiz-Lopez A, et al. Mining the human gut microbiota for immunomodulatory organisms. Cell. (2017) 168:928–43.e11. doi: 10.1016/j.cell.2017.01.022
39. Collins J, Auchtung JM. Control of clostridium difficile infection by defined microbial communities. Microbiol Spectr. (2017) 5:10. doi: 10.1128/microbiolspec.BAD-0009-2016
40. Yoon MY, Yoon SS. Disruption of the gut ecosystem by antibiotics. Yonsei Med J. (2018) 59:4–12. doi: 10.3349/ymj.2018.59.1.4
41. Horvat S, Rupnik M. Interactions between clostridioides difficile and fecal microbiota in in vitro batch model: growth, sporulation, and microbiota changes. Front Microbiol. (2018) 9:1633. doi: 10.3389/fmicb.2018.01633
42. Pérez-Cobas AE, Moya A, Gosalbes MJ, Latorre A. Colonization resistance of the gut microbiota against clostridium difficile. Antibiotics. (2015) 4:337–57. doi: 10.3390/antibiotics4030337
43. DePestel DD. Aronoff DM. Epidemiology of Clostridium difficile infection. J Pharm Pract. (2013) 26:464–75. doi: 10.1177/0897190013499521
44. Cataldo MA, Granata G. Petrosillo N. Clostridium difficile infection: new approaches to prevention, non-antimicrobial treatment, and stewardship. Expert Rev Anti Infect Ther. (2017) 15:1027–40. doi: 10.1080/14787210.2017.1387535
45. Azimirad M, Krutova M, Yadegar A, Shahrokh S, Olfatifar M, Aghdaei HA, et al. Clostridioides difficile ribotypes 001 and 126 were predominant in Tehran healthcare settings from 2004 to 2018: a 14-year-long cross-sectional study. Emerg Microbes Infect. (2020) 9:1432–43. doi: 10.1080/22221751.2020.1780949
46. Shen A. Clostridium difficile toxins: mediators of inflammation. J Innate Immun. (2012) 4:149–58. doi: 10.1159/000332946
47. Voth DE, Ballard JD. Clostridium difficile toxins: mechanism of action and role in disease. Clin Microbiol Rev. (2005) 18:247–63. doi: 10.1128/CMR.18.2.247-263.2005
48. Smith AB, Soto Ocana J, Zackular JP. From nursery to nursing home: emerging concepts in Clostridioides difficile pathogenesis. Infect Immun. (2020) 88:e00934–19. doi: 10.1128/IAI.00934-19
49. Farooq PD, Urrunaga NH, Tang DM, von Rosenvinge EC. Pseudomembranous colitis. Dis Mon. (2015) 61:181–206. doi: 10.1016/j.disamonth.2015.01.006
50. Lessa FC, Mu Y, Bamberg WM, Beldavs ZG, Dumyati GK, Dunn JR, et al. Burden of Clostridium difficile Infection in the United States. N Eng J Med. (2015) 372:825–34. doi: 10.1056/NEJMoa1408913
51. Bagdasarian N, Rao K, Malani PN. Diagnosis and treatment of Clostridium difficile in adults: a systematic review. JAMA. (2015) 313:398–408. doi: 10.1001/jama.2014.17103
52. Eze P, Balsells E, Kyaw MH, Nair H. Risk factors for Clostridium difficile infections - an overview of the evidence base and challenges in data synthesis. J Glob Health. (2017) 7:010417. doi: 10.7189/jogh.07.010417
53. Lopetuso LR, Scaldaferri F, Ianiro G, Bibbò S, Settanni CR, Papa A, et al. The impact of COVID-19 pandemic on IBD endoscopic procedures in a high-volume IBD Center. Endosc Int Open. (2020) 8:E980–84. doi: 10.1055/a-1183-3073
54. Yu H, Chen K, Sun Y, Carter M, Garey KW, Savidge TC, et al. Cytokines are markers of the clostridium difficile-induced inflammatory response and predict disease severity. Clin Vaccine Immunol. (2017) 24:e00037–17. doi: 10.1128/CVI.00037-17
55. Saleh MM, Frisbee AL, Leslie JL, Buonomo EL, Cowardin CA, Ma JZ, et al. Colitis-induced Th17 cells increase the risk for severe subsequent clostridium difficile infection. Cell Host Microbe. (2019) 25:756–65.e5. doi: 10.1016/j.chom.2019.03.003
56. Morris DE, Cleary DW, Clarke SC. Secondary bacterial infections associated with influenza pandemics. Front Microbiol. (2017) 8:1041. doi: 10.3389/fmicb.2017.01041
57. Rawson TM, Moore LS, Zhu N, Ranganathan N, Skolimowska K, Gilchrist M, et al. Bacterial and fungal coinfection in individuals with coronavirus: a rapid review to support COVID-19 antimicrobial prescribing. Clin Infect Dis. (2020) 71:2459–68. doi: 10.1093/cid/ciaa530
58. Hendaus MA, Jomha FA. Covid-19 induced superimposed bacterial infection. J Biomol Struct Dyn. (2021):39:4185–91. doi: 10.1080/07391102.2020.1772110
59. Bentivegna E, Alessio G, Spuntarelli V, Luciani M, Santino I, Simmaco M, et al. Impact of COVID-19 prevention measures on risk of health care-associated Clostridium difficile infection. Am J Infect Control. (2021) 49:640–2. doi: 10.1016/j.ajic.2020.09.010
60. Bentivegna E, Luciani M, Arcari L, Santino I, Simmaco M, Martelletti P. Reduction of multidrug-resistant (MDR) bacterial infections during the COVID-19 pandemic: a retrospective study. Int J Environ Res Public Health. (2021) 18:1003. doi: 10.3390/ijerph18031003
61. Liew Y, Lee WHL, Tan L, Kwa ALH, Thien SY, Cherng BPZ, et al. Antimicrobial stewardship programme: a vital resource for hospitals during the global outbreak of coronavirus disease 2019 (COVID-19). Int J Antimicrob Agents. (2020) 56:106145. doi: 10.1016/j.ijantimicag.2020.106145
62. Luciani M, Bentivegna E, Spuntarelli V, Lamberti PA, Guerritore L, Chiappino D, et al. Coinfection of tuberculosis pneumonia and COVID-19 in a patient vaccinated with Bacille Calmette-Guérin (BCG): case report. SN Compr Clin Med. (2020) 2:2419–22. doi: 10.1007/s42399-020-00601-9
63. Bentivegna E, Luciani M, Spuntarelli V, Speranza M, Guerritore L, Sentimentale A, et al. Extremely severe case of COVID-19 pneumonia recovered despite bad prognostic indicators: a didactic report. SN Compr Clin Med. (2020) 2:1204–7. doi: 10.1007/s42399-020-00383-0
64. Bentivegna E, Sentimentale A, Luciani M, Speranza ML, Guerritore L, Martelletti P. New IgM seroconversion and positive RT-PCR test after exposure to the virus in recovered COVID-19 patient. J Med Virol. (2021) 93:97–8. doi: 10.1002/jmv.26160
65. Luciani M, Bentivegna E, Spuntarelli V, Lamberti PA, Cacioli G, Del Porto F, et al. Recurrent COVID-19 pneumonia in the course of chemotherapy: consequence of a weakened immune system? J Med Virol. (2021) 93:1882–4. doi: 10.1002/jmv.26701
66. Little P. Non-steroidal anti-inflammatory drugs and covid-19. BMJ. (2020) 368:m1185. doi: 10.1136/bmj.m1185
67. Conaghan PG. A turbulent decade for NSAIDs: update on current concepts of classification, epidemiology, comparative efficacy, and toxicity. Rheumatol Int. (2012) 32:1491–502. doi: 10.1007/s00296-011-2263-6
68. Micallef J, Soeiro T, Annie-Pierre JB. Non-steroidal anti-inflammatory drugs, pharmacology, and COVID-19 infection. Therapie. (2020 75:355–62. doi: 10.1016/j.therap.2020.05.003
69. Maseda D, Ricciotti E. NSAID-gut microbiota interactions. Front Pharmacol. (2020) 11:1153. doi: 10.3389/fphar.2020.01153
70. Kirchgesner J, Lemaitre M, Carrat F, Zureik M, Carbonnel F, Dray-Spira R. Risk of serious and opportunistic infections associated with treatment of inflammatory bowel diseases. Gastroenterology. (2018) 155:337–46.e10. doi: 10.1053/j.gastro.2018.04.012
71. Wongrakpanich S, Wongrakpanich A, Melhado K, Rangaswami J. A comprehensive review of non-steroidal anti-inflammatory drug use in the elderly. Aging Dis. (2018) 9:143–50. doi: 10.14336/AD.2017.0306
72. Gunaydin C, Bilge SS. Effects of nonsteroidal anti-inflammatory drugs at the molecular level. Eurasian J Med. (2018) 50:116–21. doi: 10.5152/eurasianjmed.2018.0010
73. FitzGerald G, Ricciotti E. Prostaglandins and Inflammation. Arterioscler Thromb Vasc Biol. (2011) 31:986–1000. doi: 10.1161/ATVBAHA.110.207449
74. Esba LCA, Alqahtani RA, Thomas A, Shamas N, Alswaidan L, Mardawi G. Ibuprofen and NSAID use in COVID-19 infected patients is not associated with worse outcomes: a prospective cohort study. Infect Dis Ther. (2021) 10:253–68. doi: 10.1007/s40121-020-00363-w
75. Muñoz-Miralles J, Trindade BC, Castro-Córdova P, Bergin IL, Kirk LA, Gil F, et al. Indomethacin increases severity of Clostridium difficile infection in mouse model. Future Microbiol. (2018) 13:1271–81. doi: 10.2217/fmb-2017-0311
76. Maseda D, Zackular JP, Trindade B, Kirk L, Roxas JL, Rogers LM, et al. Nonsteroidal anti-inflammatory drugs alter the microbiota and exacerbate clostridium difficile colitis while dysregulating the inflammatory response. mBio. (2019) 10:e02282–18. doi: 10.1128/mBio.02282-18
77. Noori M, Yadegar A, Zali MR. A complex scenario of nonsteroidal anti-inflammatory drugs induced prostaglandin E2 production and gut microbiota alteration in clostridium difficile-infected mice. mBio. (2020) 11:e02596–19. doi: 10.1128/mBio.02596-19
78. Rekatsina M, Paladini A, Cifone MG, Lombardi F, Pergolizzi JV, Varrassi G. Influence of microbiota on NSAID enteropathy: a systematic review of current knowledge and the role of probiotics. Adv Ther. (2020) 37:1933–45. doi: 10.1007/s12325-020-01338-6
79. Otani K, Tanigawa T, Watanabe T, Shimada S, Nadatani Y, Nagami Y, et al. Microbiota plays a key role in non-steroidal anti-inflammatory drug-induced small intestinal damage. Digestion. (2017) 95:22–8. doi: 10.1159/000452356
80. Rogers MAM, Aronoff DM. The influence of non-steroidal anti-inflammatory drugs on the gut microbiome. Clin Microbiol Infect. (2016) 22:178.e1–9. doi: 10.1016/j.cmi.2015.10.003
81. Suissa D, Delaney JA, Dial S, Brassard P. Non-steroidal anti-inflammatory drugs and the risk of Clostridium difficile-associated disease. Br J Clin Pharmacol. (2012) 74:370–5. doi: 10.1111/j.1365-2125.2012.04191.x
82. Gentric A, Pennec YL. Diclofenac-induced pseudomembranous colitis. Lancet. (1992) 340:126–7. doi: 10.1016/0140-6736(92)90459-G
83. Romero-Gómez M, Suárez García E, Castro Fernández M. Pseudomembranous colitis induced by diclofenac. J Clin Gastroenterol. (1998) 26:228. doi: 10.1097/00004836-199804000-00018
84. Mycroft-West CJ, Su D, Pagani I, Rudd TR, Elli S, Gandhi NS, et al. Heparin inhibits cellular invasion by SARS-CoV-2: structural dependence of the interaction of the spike s1 receptor-binding domain with Heparin. Thromb Haemost. (2020) 120:1700–15. doi: 10.1055/s-0040-1721319
85. Chary MA, Barbuto AF, Izadmehr S, Hayes BD, Burns MM. COVID-19: therapeutics and their toxicities. J Med Toxicol. (2020) 16:284–94. doi: 10.1007/s13181-020-00777-5
86. Yacouba A, Olowo-Okere A, Yunusa I. Repurposing of antibiotics for clinical management of COVID-19: a narrative review. Ann Clin Microbiol Antimicrob. (2021) 20:37. doi: 10.1186/s12941-021-00444-9
87. Granata G, Bartoloni A, Codeluppi M, Contadini I, Cristini F, Fantoni M, et al. The burden of clostridioides difficile infection during the COVID-19 pandemic: a retrospective case-control study in italian hospitals (CloVid). J Clini Med. (2020) 9:3855. doi: 10.3390/jcm9123855
88. Huttner BD, Catho G, Pano-Pardo JR, Pulcini C, Schouten J. COVID-19: don't neglect antimicrobial stewardship principles! Clin Microbiol Infect. (2020) 26:808–10. doi: 10.1016/j.cmi.2020.04.024
89. Fan BE, Lim KGE, Chong VCL, Chan SSW, Ong KH, Kuperan P. COVID-19 and mycoplasma pneumoniae coinfection. Am J Hematol. (2020) 95:723–4. doi: 10.1002/ajh.25785
90. Ferreira EO, Penna B, Yates EA. Should we be worried about clostridioides difficile during the SARS-CoV2 pandemic? Front Microbiol. (2020) 11:581343. doi: 10.3389/fmicb.2020.581343
91. Reeves AE, Theriot CM, Bergin IL, Huffnagle GB, Schloss PD, Young VB. The interplay between microbiome dynamics and pathogen dynamics in a murine model of Clostridium difficile Infection. Gut Microbes. (2011) 2:145–58. doi: 10.4161/gmic.2.3.16333
92. Sandhu A, Tillotson G, Polistico J, Salimnia H, Cranis M, Moshos J, et al. Clostridioides difficile in COVID-19 patients, Detroit, Michigan, USA, March–April 2020. Emerg Infect Dis. (2020) 26:2272–4. doi: 10.3201/eid2609.202126
93. Libertini G, Corbi G, Cellurale M, Ferrara N. Age-related dysfunctions: evidence and relationship with some risk factors and protective drugs. Biochemistry. (2019) 84:1442–50. doi: 10.1134/S0006297919120034
94. Wang W, Tang J, Wei F. Updated understanding of the outbreak of 2019 novel coronavirus (2019-nCoV) in Wuhan, China. J Med Virol. (2020) 92:441–7. doi: 10.1002/jmv.25689
95. Leung C. Clinical features of deaths in the novel coronavirus epidemic in China. Rev Med Virol. (2020) 30:e2103. doi: 10.1002/rmv.2103
96. Kang SJ, Jung SI. Age-related morbidity and mortality among patients with COVID-19. Infect Chemother. (2020) 52:154–64. doi: 10.3947/ic.2020.52.2.154
97. Mueller AL, McNamara MS. Sinclair DA. Why does COVID-19 disproportionately affect older people? Aging. (2020) 12:9959–81. doi: 10.18632/aging.103344
98. Lionakis N, Mendrinos D, Sanidas E, Favatas G. Georgopoulou M. Hypertension in the elderly. World J Cardiol. (2012) 4: 135–47. doi: 10.4330/wjc.v4.i5.135
99. Suastika K, Dwipayana P, Saraswati IMR, Kuswardhani T, Astika N, Putrawan IB, et al. Relationship between age and metabolic disorders in the population of Bali. J Clin Gerontol Geriatr. (2011) 2:47–52. doi: 10.1016/j.jcgg.2011.03.001
100. Grasselli G, Zangrillo A, Zanella A, Antonelli M, Cabrini L, Castelli A, et al. Baseline characteristics and outcomes of 1591 patients infected with SARS-CoV-2 admitted to ICUs of the Lombardy Region, Italy. JAMA. (2020) 323:1574–81. doi: 10.1001/jama.2020.5394
101. Landi F, Barillaro C, Bellieni A, Brandi V, Carfì A, D'Angelo M, et al. The new challenge of geriatrics: saving frail older people from the SARS-COV-2 pandemic infection. J Nutr Health Aging. (2020) 24:466–70. doi: 10.1007/s12603-020-1356-x
102. Yazdanpanah F, Hamblin MR, Rezaei N. The immune system and COVID-19: friend or foe? Life Sci. (2020) 256:117900. doi: 10.1016/j.lfs.2020.117900
103. AlGhatrif M, Cingolani O, Lakatta EG. The dilemma of coronavirus disease 2019, aging, and cardiovascular disease: insights from cardiovascular aging science. JAMA Cardiol. (2020) 5:747–8. doi: 10.1001/jamacardio.2020.1329
104. Williamson E, Walker AJ, Bhaskaran K, Bacon S, Bates C, Morton CE, et al. OpenSAFELY: factors associated with COVID-19-related hospital death in the linked electronic health records of 17 million adult NHS patients. Nature. (2020) 584:430–6. doi: 10.1038/s41586-020-2521-4
105. Santesmasses D, Castro J, Zenin A, Shindyapina A, Gerashchenko M, Zhang B, et al. COVID-19 is an emergent disease of aging. Aging Cell. (2020) 81:e13230. doi: 10.1111/acel.13230
106. Geller C, Varbanov M, Duval RE. Human coronaviruses: insights into environmental resistance and its influence on the development of new antiseptic strategies. Viruses. (2012) 4:3044–68. doi: 10.3390/v4113044
107. Jump RL. Clostridium difficile infection in older adults. Aging Health. (2013) 9:403–14. doi: 10.2217/ahe.13.37
108. Loo VG, Bourgault AM, Poirier L, Lamothe F, Michaud S, Turgeon N, et al. Host and pathogen factors for Clostridium difficile infection and colonization. N Engl J Med. (2011) 365:1693–703. doi: 10.1056/NEJMoa1012413
110. Dharmarajan T, Sipalay M, Shyamsundar R, Norkus E, Pitchumoni C. Co-morbidity, not age predicts adverse outcome in Clostridium difficile colitis. World J Gastroenterol. (2000) 6:198–201. doi: 10.3748/wjg.v6.i2.198
111. Donskey CJ. Clostridium difficile in older adults. Infect Dis Clin North Am. (2017) 31:743–56. doi: 10.1016/j.idc.2017.07.003
112. Getahun H, Smith I, Trivedi K, Paulin S. Balkhy HH. Tackling antimicrobial resistance in the COVID-19 pandemic. Bull World Health Organ. (2020) 98:442–A. doi: 10.2471/BLT.20.268573
113. Buehler PK, Zinkernagel AS, Hofmaenner DA, Wendel Garcia PD, Acevedo CT, Gómez-Mejia A, et al. Bacterial pulmonary superinfections are associated with longer duration of ventilation in critically ill COVID-19 patients. Cell Rep Med. (2021) 2:100229. doi: 10.1016/j.xcrm.2021.100229
114. Ding Q, Lu P, Fan Y, Xia Y, Liu M. The clinical characteristics of pneumonia patients coinfected with 2019 novel coronavirus and influenza virus in Wuhan, China. J Med Virol. (2020) 92:1549–55. doi: 10.1002/jmv.25781
115. Jump RLP, Crnich CJ, Mody L, Bradley SF, Nicolle LE, Yoshikawa TT. Infectious diseases in older adults of long-term care facilities: update on approach to diagnosis and management. J Am Geriatr Soc. (2018) 66:789–803. doi: 10.1111/jgs.15248
116. Adelman MW, Woodworth MH, Langelier C, Busch LM, Kempker JA, Kraft CS, et al. The gut microbiome's role in the development, maintenance, and outcomes of sepsis. Crit Care. (2020) 24:278. doi: 10.1186/s13054-020-02989-1
117. Howell MC, Green R, McGill AR, Dutta R, Mohapatra S, Mohapatra SS. SARS-CoV-2-induced gut microbiome dysbiosis: implications for colorectal cancer. Cancers. (2021) 13:2676. doi: 10.3390/cancers13112676
118. Davies K, Lawrence J, Berry C, Davis G, Yu H, Cai B, et al. Risk factors for primary clostridium difficile infection; results from the observational study of risk factors for clostridium difficile infection in hospitalized patients with infective diarrhea (ORCHID). Front Public Health. (2020) 8:293. doi: 10.3389/fpubh.2020.00293
119. Khanna S, Kraft CS. The interplay of SARS-CoV-2 and Clostridioides difficile infection. Future Microbiol. (2021) 16:439–43. doi: 10.2217/fmb-2020-0275
120. Wang J, Zhao S, Liu M, Zhao Z, Xu Y, Wang P, et al. ACE2 expression by colonic epithelial cells is associated with viral infection, immunity and energy metabolism. medRxiv [Preprints]. (2020). doi: 10.1101/2020.02.05.20020545
121. Gasbarrini G, Dionisi T, Franceschi F, Gasbarrini A. Editorial-COVID-19 and the microbiota: new kids on the block. Eur Rev Med Pharmacol Sci. (2020) 24:5189–91. doi: 10.26355/eurrev_202005_21218
122. Ma WT, Pang M, Fan QL, Chen DK. The commensal microbiota and viral infection: a comprehensive review. Front Immunol. (2019) 10:1551. doi: 10.3389/fimmu.2019.01551
123. Lau FH, Majumder R, Torabi R, Saeg F, Hoffman R, Cirillo JD, et al. Vitamin D insufficiency is prevalent in severe COVID-19. medRxiv [Preprints]. (2020). doi: 10.1101/2020.04.24.20075838
124. Wang WJ, Gray S, Sison C, Arramraju S, John BK, Hussain SA, et al. Low vitamin D level is an independent predictor of poor outcomes in Clostridium difficile-associated diarrhea. Therap Adv Gastroenterol. (2014) 7:14–9. doi: 10.1177/1756283X13502838
125. Balzanelli MG, Distratis P, Lazzaro R, Cefalo A, Catucci O, Aityan SK, et al. The Vitamin D, IL-6 and the eGFR markers a possible way to elucidate the lung–heart–kidney cross-talk in COVID-19 disease: a foregone conclusion. Microorganisms. (2021) 9:1903. doi: 10.3390/microorganisms9091903
126. Grant WB, Lahore H, McDonnell SL, Baggerly CA, French CB, Aliano JL, et al. Evidence that vitamin D supplementation could reduce risk of influenza and COVID-19 Infections and deaths. Nutrients. (2020) 12:988. doi: 10.3390/nu12040988
127. Tay MZ, Poh CM, Rénia L, MacAry PA, Ng LFP. The trinity of COVID-19: immunity, inflammation and intervention. Nat Rev Immunol. (2020) 20:363–74. doi: 10.1038/s41577-020-0311-8
128. van der Wielen RPJ, de Groot LCPGM, van Staveren WA, Löwik MRH, van den Berg H, Haller J, et al. Serum vitamin D concentrations among elderly people in Europe. Lancet. (1995) 346:207–10. doi: 10.1016/S0140-6736(95)91266-5
129. Zhang Y, Leung DYM, Richers BN, Liu Y, Remigio LK, Riches DW, et al. Vitamin D inhibits monocyte/macrophage proinflammatory cytokine production by targeting MAPK phosphatase-1. J Immunol. (2012) 188:2127–35. doi: 10.4049/jimmunol.1102412
130. Calton EK, Keane KN, Newsholme P, Soares MJ. The impact of vitamin D levels on inflammatory status: a systematic review of immune cell studies. PLoS ONE. (2015) 10:e0141770. doi: 10.1371/journal.pone.0141770
131. Wöbke TK, Sorg BL, Steinhilber D. Vitamin D in inflammatory diseases. Front Physiol. (2014) 5:244. doi: 10.3389/fphys.2014.00244
132. Foschetti D, Braga-Neto M, Bolick D, Moore J, Alves L, Martins C, et al. Clostridium difficile toxins or infection induce upregulation of adenosine receptors and IL-6 with early pro-inflammatory and late anti-inflammatory pattern. Braz J Med Biol Res. (2020) 53:e9877. doi: 10.1590/1414-431x20209877
133. Alcantara C, Stenson WF, Steiner TS, Guerrant RL. Role of inducible cyclooxygenase and prostaglandins in Clostridium difficile toxin A-induced secretion and inflammation in an animal model. J Infect Dis. (2001) 184:648–52. doi: 10.1086/322799
134. Koon HW, Shih DQ, Hing TC, Yoo JH, Ho S, Chen X, et al. Human monoclonal antibodies against Clostridium difficile toxins A and B inhibit inflammatory and histologic responses to the toxins in human colon and peripheral blood monocytes. Antimicrob Agents Chemother. (2013) 57:3214–23. doi: 10.1128/AAC.02633-12
135. Marshall B, Bennett N, Smith A, Oh R, Burket J. PURLS: can vitamin D prevent acute respiratory infections? J Fam Pract. (2019) 68:230–1.
136. Kim H, Baek S, Hong SM, Lee J, Jung SM, Lee J, et al. 1,25-dihydroxy vitamin D3 and interleukin-6 blockade synergistically regulate rheumatoid arthritis by suppressing interleukin-17 production and osteoclastogenesis. J Korean Med Sci. (2020) 35:e40. doi: 10.3346/jkms.2020.35.e40
137. Durovic A, Widmer AF, Tschudin-Sutter S. New insights into transmission of Clostridium difficile infection—narrative review. Clin Microbiol Infect. (2018) 24:483–92. doi: 10.1016/j.cmi.2018.01.027
138. Bouza E. Consequences of Clostridium difficile infection: understanding the healthcare burden. Clin Microbiol Infect. (2012) 18:5–12. doi: 10.1111/1469-0691.12064
139. Wang W, Xu Y, Gao R, Lu R, Han K, Wu G, et al. Detection of SARS-CoV-2 in different types of clinical specimens. JAMA. (2020) 323:1843–4. doi: 10.1001/jama.2020.3786
140. Yadav SS, Sinha S, Das V. Infection of gastro-intestinal tract by SARS-CoV-2 and its potential for faecal-oral transmission: a literature review. J Crit Rev. (2020) 7:1061–5. Retrieved from: https://researchonline.lshtm.ac.uk/id/eprint/4657390
Keywords: coronavirus disease 2019, Clostridioides difficile infection, antibiotic therapy, NSAIDs, gut microbiota, elderly, COVID-19
Citation: Azimirad M, Noori M, Raeisi H, Yadegar A, Shahrokh S, Asadzadeh Aghdaei H, Bentivegna E, Martelletti P, Petrosillo N and Zali MR (2021) How Does COVID-19 Pandemic Impact on Incidence of Clostridioides difficile Infection and Exacerbation of Its Gastrointestinal Symptoms? Front. Med. 8:775063. doi: 10.3389/fmed.2021.775063
Received: 13 September 2021; Accepted: 22 November 2021;
Published: 13 December 2021.
Edited by:
Sofia Viana, University of Coimbra, PortugalReviewed by:
Maria Contaldo, University of Campania Luigi Vanvitelli, ItalyFlávio Reis, University of Coimbra, Portugal
Copyright © 2021 Azimirad, Noori, Raeisi, Yadegar, Shahrokh, Asadzadeh Aghdaei, Bentivegna, Martelletti, Petrosillo and Zali. This is an open-access article distributed under the terms of the Creative Commons Attribution License (CC BY). The use, distribution or reproduction in other forums is permitted, provided the original author(s) and the copyright owner(s) are credited and that the original publication in this journal is cited, in accordance with accepted academic practice. No use, distribution or reproduction is permitted which does not comply with these terms.
*Correspondence: Abbas Yadegar, YS55YWRlZ2FyQHNibXUuYWMuaXI=; YmFiYWtfeTE5ODNAeWFob28uY29t
†ORCID: Masoumeh Azimirad orcid.org/0000-0002-2983-7117
Maryam Noori orcid.org/0000-0001-7191-4895
Hamideh Raeisi orcid.org/0000-0001-8334-4180
Abbas Yadegar orcid.org/0000-0002-2135-7581