- 1Department of Radiology, An Nan Hospital, China Medical University, Tainan, Taiwan
- 2School of Medicine, College of Medicine, China Medical University, Taichung, Taiwan
- 3Department of Medical Imaging and Radiology, Shu-Zen Junior College of Medicine and Management, Kaohsiung, Taiwan
- 4Division of Chest Medicine, Department of Internal Medicine, National Yang Ming Chiao Tung University Hospital, Yilan, Taiwan
- 5Department of Critical Care Medicine, National Yang Ming Chiao Tung University Hospital, Yilan, Taiwan
- 6Faculty of Medicine, College of Medicine, National Yang Ming Chiao Tung University, Taipei, Taiwan
- 7Department of Information Management, Meiho University, Pingtung, Taiwan
- 8Department of Emergency Medicine, An Nan Hospital, China Medical University, Tainan, Taiwan
- 9Graduate Institute of Medical Sciences, Chang Jung Christian University, Tainan, Taiwan
- 10Management Office for Health Data, China Medical University Hospital, Taichung, Taiwan
- 11Graduate Institute of Biomedical Sciences, College of Medicine, China Medical University, Taichung, Taiwan
- 12Center of Augmented Intelligence in Healthcare, China Medical University Hospital, Taichung, Taiwan
- 13Department of Nuclear Medicine and PET Center, China Medical University Hospital, Taichung, Taiwan
- 14Department of Bioinformatics and Medical Engineering, Asia University, Taichung, Taiwan
Objective: To evaluate the association between radiation exposure from repeated nuclear medicine (NM) examinations and the subsequent risk of neoplasm in pediatric patients.
Methods: From 2000 to 2017, participants under 18 years of age who underwent NM scanning were identified using the Health and Welfare Data Science Center (HWDC) dataset, which was extracted from the Taiwan National Health Insurance Research Database (NHIRD). Both the exposed cohort and unexposed subjects were followed up with until the presence of any malignancy arose, including malignant brain, lymphoid and hematopoietic tumors and benign brain or other central nervous tumors.
Results: There were 35,292 patients in the exposed cohort and 141,152 matched subjects in the non-exposed group. The exposed cohort had an overall higher IR (IR: incidence rate, per 100,000 person-years) of any malignancy and benign central nervous tumor than the non-exposed group [IR, 16.9 vs. 1.54; adjusted hazard ratio (HR), 10.9; 95% CI, 6.53–18.2]. Further stratifying the number of NM examinations into 1-2, 3-4, and 5 or more times revealed that the IR of pediatric neoplasms increased gradually with the increased frequency of NM examinations (IR, 11.5; adjusted HR, 7.5; 95% CI, 4.29–13.1; IR, 25.8; adjusted HR, 15.9; 95% CI, 7.00–36.1; IR, 93.8; adjusted HR, 56.4; 95% CI, 28.8–110.3).
Conclusion: NM examination is significantly associated with a higher risk of pediatric neoplasms, according to our population-based data. Thorough radiation protection and dose reduction in pediatric NM procedures should be an issue of concern.
Introduction
Radiation hazards to patients and medical workers exposed to radiological examinations have raised worldwide concerns in recent decades (1–3). The ICRP 2015 Proceedings indicate that 32.7 million global diagnostic nuclear medicine (NM) examinations are performed annually, with an increase of 0.2 million examinations per year since 1991 (4). According to the National Council on Radiation Protection and Measurements (NCRP) 160 report, NM procedures have increased from 6.3 million in 1984 to 18 million in 2006, with ~1% of these procedures performed on children in the United States (5). NM imaging provides essential physiological and pathological information in oncology, urology, and orthopedics, and such imaging is particularly valuable in children and infants, in whom a rapid and accurate diagnosis is crucial for developmental health and reducing disease progression. Children have a higher risk for adverse effects from radiation exposure than adults, and the subsequent lifelong estimated cancer risks due to repeated radiological examination should not be ignored (6, 7). NM procedures are traditionally thought to be safe and non-invasive without serious complications. For example, 99mTc-DMSA renal scintigraphy can be used acutely to confirm the presence of acute pyelonephritis or, 4–6 months later, the sequelae of renal cortical scarring in pediatric patients with recurrent urinary tract infections. The estimated effective dose administered for a common 99mTc-DMSA scan is low, varying between 0.6 and 0.8 millisieverts (mSv). Although the majority of NM exams performed on children require a low effective dose of <1 mSv, radiation exposure and cancer risk from repeated scans in the acute stage and disease follow-up should never be overlooked (8). Some NM examinations, especially in oncology and orthopedics, can result in an intermediate radiation dose, between 3 and 6 mSv, which is close to the radiation dose received from one modern CT scan (9, 10). Several studies have revealed evidence of higher cancer risk after repeated CT scan exposure in pediatric patients (11–15). It is conceivable that the cancer risk associated with NM procedures in the pediatric population is a topic that warrants further assessment. Our encrypted identification and medical records of participants were representatively extracted from the NHIRD, which has the advantage of being one of the largest national databases in the world. This population-based study was designed to investigate the possible association between pediatric neoplasms and diagnostic NM procedures.
Materials and Methods
Data Source
This study used data from the Health and Welfare Data Science Center (HWDC), which contains over 99% of 23 million Taiwanese residents' national electronic medical records. The International Classification of Diseases, Clinical Modification (ICD-9-CM) and International Classification of Diseases, Tenth Revision, Clinical Modification (ICD-10-CM) were used as diagnostic tools for diseases. This study was approved by the Central Regional Research Ethics Committee, China Medical University, Taichung, Taiwan (CMUH109–109-REC2–031).
Study Population
The study period of this retrospective population-based cohort study was between 2000 and 2017. We selected participants under 18 years of age who underwent nuclear medicine (NM) scans (Taiwan National Health Insurance payment code 26001B~26070B). Positron emission tomography (PET) and I131−related therapy were not included due to cancer selection bias. For each NM scan patient, we selected four patients who never underwent NM scans as unexposed comparison group based on sex, age, and index year. The exclusion criteria for both the NM scan group and the unexposed group were as follows: patients who ever underwent high radiation dose examination or therapy, such as CT scan and radiation therapy, and those with disorders that may have increased cancer risk, such as multiple endocrine neoplasia (ICD-9-CM code 258.01–258.03; ICD-10-CM code E31.20-E31.23); neurofibromatosis; phakomatosis; and tuberous sclerosis (ICD-9-CM code 237.70–72; 759.5, 759.6; ICD-10-CM code Q85.0–85.9). In addition, we excluded patients if they had a prior history of cancer before the index date and cancer development within the first 2 years of follow-up (lag period of 2 years for cancer diagnosis). The date for the first NM examination was defined as the index date and the start of follow-up set at 2 years after the index date. All participants were followed from cohort entry until the presence of the primary outcome, death, censoring for loss or the end of the study period (December 31, 2018), whichever came first (Figure 1).
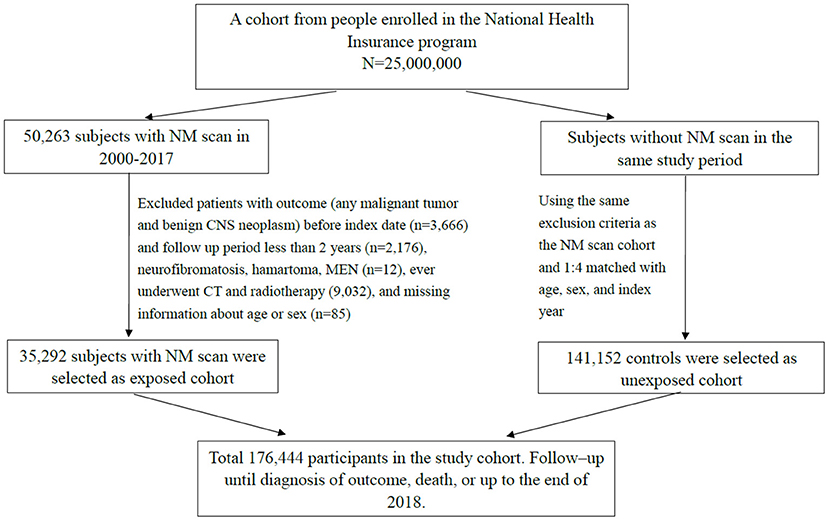
Figure 1. Flowchart for selecting study participants. NM, nuclear medicine; CNS, central nervous system; MEN, multiple endocrine neoplasia.
Outcomes
The major categories of leukemias, lymphomas and malignant brain tumors represent close to 70% of all pediatric cancers (16).
A previous study revealed that repeated head CT scans might increase the risk of benign pediatric brain tumors (12). Therefore, our primary outcome measure included any malignant tumor (ICD-9-CM code 140.0–208.92; ICD-10-CM code C00.1-C80.1), malignant lymphoid and hematopoietic tissue (ICD-9-CM code 200.00–208.92; ICD-10-CM code C81.00-C96.9), malignant brain and other central nervous tumor (ICD-9-CM code 191.0, 192, 194.2, 194.3, 194.4; ICD-10-CM code C71.0-C72.9), and benign brain and other central nervous neoplasm (ICD-9-CM code 225.0–225.9, 227.3, 227.4; ICD-10-CM code D32.0-D33.9).
Statistical Analysis
We computed the categorical variables as numbers and percentages and the continuous variables as the mean and standard deviation (SD). For the difference in the categorical and continuous demographic variables between the NM scan group and unexposed comparison group, statistical significance was determined with the chi-square test and Student's t-test. To address the concern of constant proportionality, we examined the proportional hazard model assumption using a test of scaled Schoenfeld residuals. Results showed that there was no significant relationship between Schoenfeld residuals for radiation exposure and follow-up time (p-value = 0.71) in the model evaluating the neoplasm risk. Univariable and multivariable Cox proportional hazards models were used to estimate the risk of pediatric neoplasms in children with and without NM scans. With adjustment for sex, age, and urbanization in multivariable analysis, we obtained the adjusted hazard ratio. The Kaplan–Meier method was applied to obtain the cumulative incidence curve. Statistical analysis was performed using SAS software, version 9.4, and we generated survival curves in R software. The significance criterion was a two-sided p-value < 0.05.
Results
Characteristics of Study Population
The comparisons of demographic characteristics in participants with and without NM scans of the study are listed in Table 1. From 2000 to 2017, the cohort consisted of 35,292 patients with NM scans and 141,152 patients without NM scans. After matching, the distribution of sex, age and urbanization was similar in the NM scan-exposed group and the unexposed comparison group. The average age in both groups was ~6 years old. There were more female patients (50.4%) than male patients (49.6%) in both groups. The average follow-up time was ~9 years.
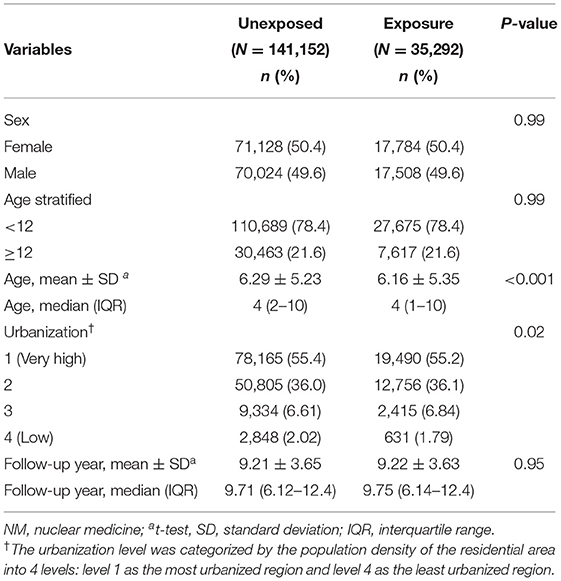
Table 1. Demographic characteristics and incidence rate in cohorts with and without NM examination exposure.
Higher Cancer Incidence Rates in the NM Scan Exposure Cohort
As shown in Table 2, the overall incidence rates (IR: incidence rate, per 100,000 person-years) of any malignant tumor and benign central nervous neoplasm in the NM-exposed cohort were significantly higher than those in the non-exposed group [IR, 16.9 vs. 1.54; adjusted hazard ratio (HR), 10.9; 95% CI, 6.53–18.2]. The incidence rate of malignant lymphoid and hematopoietic cancer in the exposed cohort was significantly higher than that in the non-exposed group (IR, 6.46 vs. 0.92; adjusted HR, 6.77; 95% CI, 3.33–13.8). The incidence rate of malignant brain and other central nervous tumors in the exposed cohort was significantly higher than that in the non-exposed group (IR, 2.77 vs. 0.38; adjusted HR, 6.78; 95% CI, 2.27–20.3). The incidence rate of benign brain and other central nervous tumors in the exposed cohort was significantly higher than that in the non-exposed group (IR, 1.54 vs. 0.08; adjusted HR, 25.0; 95% CI, 3.01–207.7).
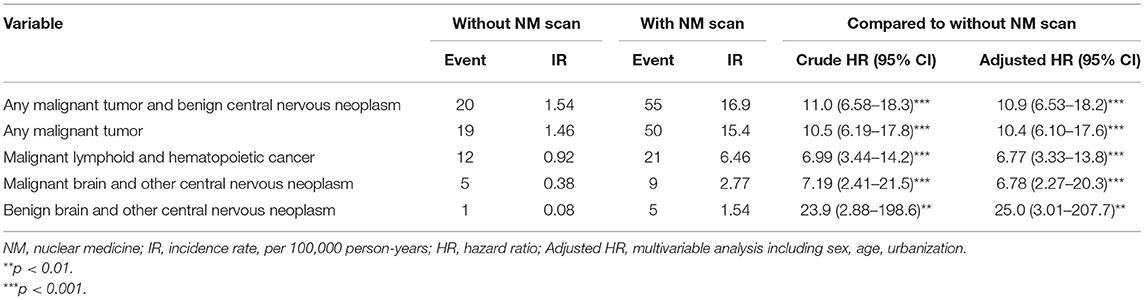
Table 2. Comparison of incidence rate of radiosensitive tumor and hazard ratio between cohorts with or without NM procedures.
Trend in Increased NM Frequency Associated With Higher Cancer Risk
Table 3 shows the stratification of the number of NM exams in the exposed cohort into 1-2, 3-4, and 5 or more times and revealed that the incidence rates of pediatric neoplasms increased gradually with the increased frequency of NM exams (IR, 11.5; adjusted HR, 7.5; 95% CI, 4.29–13.1; IR, 25.8; adjusted HR, 15.9; 95% CI, 7.00–36.1; IR, 93.8; adjusted HR, 56.4; 95% CI, 28.8–110.3). Figure 2 shows that the cumulative incidence of pediatric neoplasms in children who underwent NM scanning was significantly higher than that in children who did not undergo scanning (log-rank test p < 0.001).
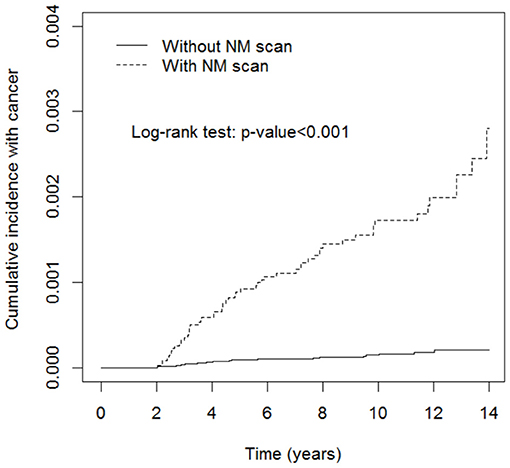
Figure 2. Cumulative incidence rate of pediatric neoplasms in patients with or without nuclear medicine (NM) scans. Pediatric neoplasms had a significantly higher incidence rate in the NM scan exposure group (log-rank test p < 0.001).
Discussion
This current study shows that pediatric patients who are exposed to NM examinations have a significantly higher risk of developing neoplasms than non-exposed subjects. The incidence rates of any malignant tumor and benign brain and central nervous neoplasm in the NM scanning cohort were significantly higher than those of patients without scanning (16.9 vs. 1.54; adjusted HR, 10.9; 95% CI, 6.53–18.2). Subsequent analysis revealed the trend that with an increasing number of NM scans, the risk of pediatric neoplasms was aggregated (increase in adjusted HR from 7.50 to 56.4 with the increase in frequency from 1–2 to ≥5).
The most significant consequence of low-level radiation exposure in humans is cancer. The carcinogenic risk of radiation may be two to three times higher in children than in adults, although this risk varies with organ and tissue type (8). Cancer induction resulting from radiation exposure occurs in a stochastic manner: there is no threshold point, and the risk increases proportionally with the dose (17). The vulnerability of the pediatric population to ionizing radiation is due not only to the enhanced radiosensitivity of their tissues but also to a longer time period for the manifestation of stochastic radiation effects. First, our study results revealed that the incidence rate of pediatric neoplasms was significantly higher in the NM exposure group from the beginning of the follow-up period, which is consistent with the lack of a stochastic effect threshold. Furthermore, a higher risk of cancer is significantly associated with a higher frequency of NM exams, which indicates higher radiation dose exposure. This proportional probability of cancer occurrence is also compatible with the dose-dependent stochastic effect (Table 2; Figure 2). The abovementioned two results demonstrate the traditionally accepted linear and no-threshold model of the stochastic radiation effect.
Hereditary deformity attributed to low-dose radiation is the other example of a stochastic effect. Earlier studies linked prenatal medical radiation exposure to pediatric cancer in offspring, but there was no solid evidence for these conclusions (18, 19). Recent articles reviewed animal studies or applied different risk estimation models to discuss low-dose radiation exposure in nuclear medicine and the associated risk of cancer (20, 21). A PubMed-based literature search revealed a lack of large-scale investigations into childhood NM radiation exposure and subsequent cancer risk. One of the possible reasons is difficulties in pediatric NM procedure dose estimation. NM radiation dosimetry is challenging due to diversities in the uptake, retention, and clearance of variable radiopharmaceuticals. Furthermore, individual administered doses in children also differ depending on the patient's body mass, type of examination, available SPECT model, examination time, and patient cooperation (22, 23). Nevertheless, updated nuclear medicine drug decay half-lives are now available in ICRP Publication 107 (24). Based on these contemporary radiopharmaceutical data and the new biokinetic model, more accurate absorbed dose estimations are available for patients examined with NM procedures (25–27). With advances in absorbed dose estimation, we expect more quantitative knowledge about the risk of cancer associated with pediatric NM procedures in the future. At present, parents of pediatric patients are always anxious about the lifelong cancer risk associated with NM procedures. According to our study results, cancer risk associated with pediatric NM examinations should never be ignored. As a result of this issue, we advise physicians to take a more comprehensive approach and to consider the benefits of nuclear medicine as well as the potential risk associated with radiation exposure when deciding the best option for pediatric patients.
Limitation
The HWDC datasets do not provide information about the clinical purpose of NM examinations. Thus, a screening effect and selection bias in the NM exposure cohort may have been present in this study. Pediatric patients who underwent NM procedures might receive additional radiation exposure, such as through routine plain films or other special examinations. We excluded high radiation dose procedures such as CT scan, radiotherapy, PET scan, and therapeutic NM procedures in both the exposure and unexposed groups, and this confounding factor might not be strong enough to affect the significance of our result. In addition, the NHIRD (National Health Insurance Research Database) cannot provide radiopharmaceuticals' dosage from each NM examination, the lack of the accurate data about radiopharmaceuticals decay (related to the accurate absorbed radiation and exposure) is our major study limitation.
Therefore, due to the lack of data about the actual absorbed radiation dose at each NM examination, our results indicating higher pediatric cancer risk associated with NM exposure should be interpreted with caution. Besides above mentioned limitation, prenatal information and family history of our participants were not included in the HWDC datasets, and hereditary or genetic effects of developing pediatric neoplasms could not be assessed.
In conclusion, this study benefits from a long follow-up period (2000–2018) and a large sample size (35,292 subjects with NM exposure) and shows that low-dose radiation exposure from NM examinations is significantly associated with a higher risk of cancer and benign central nervous tumors in pediatric patients. Nuclear medicine practitioners, including physicians and technologists, should show more initiative to protect patients from radiation and should be prudent in optimizing the radiation dose in pediatric NM procedures.
Data Availability Statement
The dataset used in this study is held by the Taiwan Ministry of Health and Welfare (MOHW). The Ministry of Health and Welfare must approve our application to access this data. Any researcher interested in accessing this dataset can submit an application form to the Ministry of Health and Welfare requesting access. Please contact the staff of MOHW (Email: stcarolwu@mohw.gov.tw) for further assistance. All relevant data are within the paper. Requests to access the datasets should be directed to stcarolwu@mohw.gov.tw.
Ethics Statement
The studies involving human participants were reviewed and approved by the Central Regional Research Ethics Committee, China Medical University, Taichung, Taiwan (CMUH109–109-REC2–031). Written informed consent from the participants' legal guardian/next of kin was not required to participate in this study in accordance with the national legislation and the institutional requirements.
Author Contributions
M-KY and C-HK: conception and design. C-HK: administrative support. All authors contributed significantly, agreement with the content of the manuscript, collection and assembly of data, data analysis and interpretation, manuscript writing, and final approval of manuscript.
Funding
This study was supported in part by Taiwan Ministry of Health and Welfare Clinical Trial Center (MOHW110-TDU-B-212-124004), China Medical University Hospital (DMR-109-231, DMR-110-089, DMR-111-105), Ministry of Science and Technology (MOST 110-2321-B-039-003). The funders had no role in the study design, data collection and analysis, the decision to publish, or preparation of the manuscript.
Conflict of Interest
The authors declare that the research was conducted in the absence of any commercial or financial relationships that could be construed as a potential conflict of interest.
Publisher's Note
All claims expressed in this article are solely those of the authors and do not necessarily represent those of their affiliated organizations, or those of the publisher, the editors and the reviewers. Any product that may be evaluated in this article, or claim that may be made by its manufacturer, is not guaranteed or endorsed by the publisher.
Acknowledgments
We are grateful to Health Data Science Center, China Medical University Hospital for providing administrative, technical and funding support.
References
1. Yuan MK, Tsai DC, Chang SC, Yuan MC, Chang SJ, Chen HW, et al. The risk of cataract associated with repeated head and neck CT studies: a nationwide population-based study. AJR Am J Roentgenol. (2013) 201:626–30. doi: 10.2214/AJR.12.9652
2. Schonfeld SJ, Lee C, Berrington de González A. Medical exposure to radiation and thyroid cancer. Clin Oncol. (2011) 23:244–50. doi: 10.1016/j.clon.2011.01.159
3. Lima F, Swift JM, Greene ES, Allen MR, Cunningham DA, Braby LA, et al. Exposure to low-dose X-ray radiation alters bone progenitor cells and bone microarchitecture. Radiat Res. (2017) 188:433–42. doi: 10.1667/RR14414.1
4. Song HC. Current global and Korean issues in radiation safety of nuclear medicine procedures. Ann ICRP. (2016) 45(Suppl. 1):122–37. doi: 10.1177/0146645315624048
5. Schauer DA, Linton OW. NCRP Report No. 160, Ionizing Radiation Exposure of the Population of the United States, Medical Exposure—Are We (2009).
6. Kutanzi KR, Lumen A, Koturbash I, Miousse IR. Pediatric exposures to ionizing radiation: carcinogenic considerations. Int J Environ Res Public Health. (2016) 13:1057. doi: 10.3390/ijerph13111057
7. Bibbo G. Effective doses and standardised risk factors from paediatric diagnostic medical radiation exposures: Information for radiation risk communication. J Med Imaging Radiat Oncol. (2018) 62:43–50. doi: 10.1111/1754-9485.12628
8. Linet MS, Kim KP, Rajaraman P. Children's exposure to diagnostic medical radiation and cancer risk: epidemiologic and dosimetric considerations. Pediatr Radiol. (2009) 39:S4–26. doi: 10.1007/s00247-008-1026-3
9. Fahey FH, Goodkind AB, Plyku D, Khamwan K, O'Reilly SE, Cao X, et al. Dose estimation in pediatric nuclear medicine. Semin Nucl Med. (2017) 47:118–25. doi: 10.1053/j.semnuclmed.2016.10.006
10. Biassoni L, Easty M. Paediatric nuclear medicine imaging. Br Med Bull. (2017) 123:127–48. doi: 10.1093/bmb/ldx025
11. Miglioretti DL, Johnson E, Williams A, Greenlee RT, Weinmann S, Solberg LI, et al. The use of computed tomography in pediatrics and the associated radiation exposure and estimated cancer risk. JAMA Pediatr. (2013) 167:700–7. doi: 10.1001/jamapediatrics.2013.311
12. Huang WY, Muo CH, Lin CY, Jen YM, Yang MH, Lin JC, et al. Paediatric head CT scan and subsequent risk of malignancy and benign brain tumour: a nation-wide population-based cohort study. Br J Cancer. (2014) 110:2354–60. doi: 10.1038/bjc.2014.103
13. Vallin C, Blouin P, Venel Y, Maurin L, Sirinelli D. The use of computed tomography and nuclear medicine examinations in paediatric oncology: an analysis of practice in a university hospital. Diagn Interv Imaging. (2014) 95:411–9. doi: 10.1016/j.diii.2013.12.016
14. Chen JX, Kachniarz B, Gilani S, Shin JJ. Risk of malignancy associated with head and neck CT in children: a systematic review. Otolaryngol Head Neck Surg. (2014) 151:554–66. doi: 10.1177/0194599814542588
15. Meulepas JM, Ronckers CM, Smets AMJB, Nievelstein RAJ, Gradowska P, Lee C, et al. Radiation exposure from pediatric CT scans and subsequent cancer risk in the Netherlands. J Natl Cancer Inst. (2019) 111:256–63. doi: 10.1093/jnci/djy104
16. Howlader N, Noone, AM, Krapcho, M, Miller, D, Brest, A, Yu, M, . SEER Cancer Statistics Review, 1975-2018. Bethesda, MD: National Cancer Institute (2021). Available online at: https://seer.cancer.gov/csr/1975_2018/, based on November 2020 SEER data submission, posted to the SEER web site.
17. Martin CJ,. Effective Dose in Medicine. Effective ICRP 2019, SESSION 8 (2019). Available online at: https://www.icrp.org/page.asp?id=456 (accessed December 8, 2021).
18. Wakeford R. The risk of childhood cancer from intrauterine and preconceptional exposure to ionizing radiation. Environ Health Perspect. (1995) 103:1018–25. doi: 10.1289/ehp.951031018
19. Doll R, Wakeford R. Risk of childhood cancer from fetal irradiation. Br J Radiol. (1997) 70:130–9. doi: 10.1259/bjr.70.830.9135438
20. Adelstein SJ. Radiation risk in nuclear medicine. Semin Nucl Med. (2014) 44:187–92. doi: 10.1053/j.semnuclmed.2014.03.003
21. Andersson M, Eckerman K, Mattsson S. Lifetime attributable risk as an alternative to effective dose to describe the risk of cancer for patients in diagnostic and therapeutic nuclear medicine. Phys Med Biol. (2017) 62:9177–88. doi: 10.1088/1361-6560/aa959c
22. Vermeulen K, Vandamme M, Bormans G, Cleeren F. Design and challenges of radiopharmaceuticals. Semin Nucl Med. (2019) 49:339–56. doi: 10.1053/j.semnuclmed.2019.07.001
23. Treves ST, Lassmann M, EANM/SNMMI Pediatric Dosage Harmonization Working Group. International guidelines for pediatric radiopharmaceutical administered activities. J Nucl Med. (2014) 55:869–70. doi: 10.2967/jnumed.114.139980
24. Eckerman K, Endo A. ICRP publication 107. Nuclear decay data for dosimetric calculations. Ann ICRP. (2008) 38:7–96. doi: 10.1016/j.icrp.2008.10.005
25. Andersson M, Johansson L, Eckerman K, Mattsson S. IDAC-Dose 2.1, an internal dosimetry program for diagnostic nuclear medicine based on the ICRP adult reference voxel phantoms. EJNMMI Res. (2017) 7:88. doi: 10.1186/s13550-017-0339-3
26. Andersson M, Johansson L, Minarik D, Mattsson S, Leide-Svegborn S. An internal radiation dosimetry computer program, IDAC2.0, for estimation of patient dose for radiopharmaceuticals. Radiat Prot Dosim. (2014) 162:299–305. doi: 10.1093/rpd/nct337
Keywords: nuclear medicine (NM), pediatric neoplasms, radiation protection, malignancy, National Health Insurance Research Database (NHIRD)
Citation: Yuan M-K, Chang S-C, Yuan M-C, Foo N-P, Chan S-H, Wang S-Y, Lin C-L, Hsu C-Y and Kao C-H (2021) Pediatric Nuclear Medicine Examinations and Subsequent Risk of Neoplasm: A Nationwide Population-Based Cohort Study. Front. Med. 8:764849. doi: 10.3389/fmed.2021.764849
Received: 26 August 2021; Accepted: 01 December 2021;
Published: 20 December 2021.
Edited by:
Yuh-Feng Wang, Dalin Tzu Chi Hospital, TaiwanReviewed by:
Malcolm Koo, Tzu Chi University of Science and Technology, TaiwanPrasanta Kumar Pradhan, Sanjay Gandhi Post Graduate Institute of Medical Sciences (SGPGI), India
Copyright © 2021 Yuan, Chang, Yuan, Foo, Chan, Wang, Lin, Hsu and Kao. This is an open-access article distributed under the terms of the Creative Commons Attribution License (CC BY). The use, distribution or reproduction in other forums is permitted, provided the original author(s) and the copyright owner(s) are credited and that the original publication in this journal is cited, in accordance with accepted academic practice. No use, distribution or reproduction is permitted which does not comply with these terms.
*Correspondence: Chia-Hung Kao, d10040@mail.cmuh.org.tw; dr.kaochiahung@gmail.com