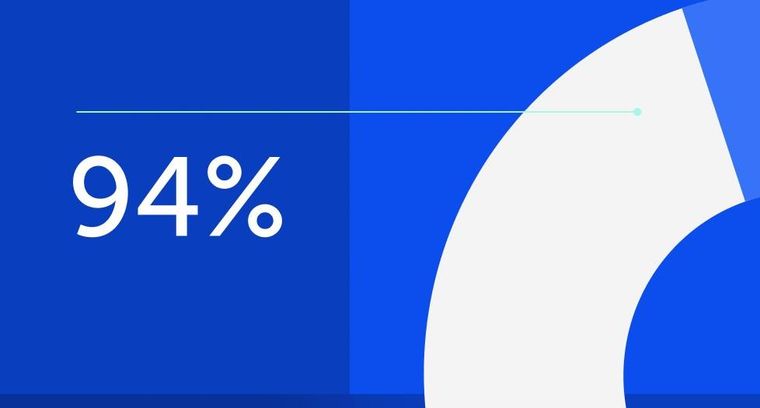
94% of researchers rate our articles as excellent or good
Learn more about the work of our research integrity team to safeguard the quality of each article we publish.
Find out more
ORIGINAL RESEARCH article
Front. Med., 11 October 2021
Sec. Intensive Care Medicine and Anesthesiology
Volume 8 - 2021 | https://doi.org/10.3389/fmed.2021.762724
This article is part of the Research TopicAcute Respiratory Distress Syndrome and Mechanical VentilationView all 15 articles
Background: To prospectively observe the early alterations of lymphocyte subsets in ARDS caused by Acinetobacter baumannii.
Methods: ARDS patients admitted to our ICU between January 1, 2017 and May 30, 2020 were selected. We enrolled all the pulmonary ARDS caused by Acinetobacter baumannii pneumonia who required mechanical ventilation or vasopressors. All the available clinical data, follow up information and lymphocyte subsets were recorded.
Results: Eighty-seven of all the 576 ARDS patients were enrolled. The 28-day mortality of the enrolled patients was 20.7% (18/87). The T lymphocyte count (452 vs. 729 cells/ul, P = 0.004), especially the CD8+ T lymphocyte count (104 vs. 253 cells/ul, P = 0.002) was significantly lower in non-survivors, as were counts of the activated T cell subsets (CD8+CD28+ and CD8+CD38+). The CD8+ T cell count was an independent risk factor for 28-day mortality, and a cutoff value of 123 cells/ul was a good indicator to predict the prognosis of ARDS caused by Acinetobacter baumannii pneumonia, with sensitivity of 74.6% and specificity of 83.3% (AUC 0.812, P < 0.0001).
Conclusions: Lower CD8+ T cell count was associated with higher severity and early mortality in ARDS patients caused by Acinetobacter baumannii pneumonia, which could be valuable for outcome prediction.
Acinetobacter baumannii (A. baumannii) is an opportunistic pathogen and one of the most common causes of hospital-acquired pneumonia, resulting from the increasingly serious occurrence of antibiotic resistance (1). Acute respiratory distress syndrome (ARDS) caused by A. baumannii pneumonia has significantly high mortality (2). It follows that there has been growing interest in identifying biological sub-phenotypes of ARDS patients (3). Measuring plasma biomarkers in ARDS can help find subgroups of patients those share important host-response features and/or those have worse clinical outcomes. Li et al. (4) found that neutrophil to lymphocyte ratio (NLR) was significantly associated with 28-day mortality in patients with ARDS, and NLR was related to the severity of ARDS. However, how the lymphocyte counts changed in NLR and their correlation with prognosis were not clearly illustrated.
Traditionally, A. baumannii was thought to cause extracellular infection, and innate immunity played a vital role in the defense against A. baumannii infection. Monocytes release tumor necrosis factor (TNF) to recruit granulocytes, which phagocytize bacteria or produce reactive oxygen species. Immature dendritic cells (DCs) capture and process antigens with high efficiency. CD4+ T cells differentiate toward a Th1-polarizing phenotype through the activation of DCs (5). However, A. baumannii has been shown to cause facultative intracellular infection recently, and many studies have confirmed its ability to invade lung epithelial cells and macrophages (1, 6–8). Therefore, many immune cells and cytokines that act against intracellular and extracellular infection might be involved in the immune response against A. baumannii infection, which is worth of further study. There have been few clinical studies on the changes and specific roles of lymphocyte subsets in the pathogenesis of ARDS caused by A. baumannii pneumonia. In this study we aimed to explore the role of lymphocyte subsets in ARDS caused by A. baumannii and its correlation with prognosis.
We screened all the ARDS patients according to the 2012 Berlin definition (9) admitted to the intensive care unit (ICU) of Peking Union Medical College Hospital (PUMCH) between January 1, 2017 and May 30, 2020. Pulmonary ARDS caused by A. baumannii pneumonia and required mechanical ventilation or vasopressors were enrolled in our study. All eligible patients needed to be over 18 years old, ICU stays for 48 h and met none of the exclusion criteria. Exclusion criteria were: (1) any condition causing neutropenia as receiving corticosteroids or immunosuppression; (2) pregnancy or lactation; (3) any condition causing primary or acquired immunodeficiency, such as HIV infection, active autoimmune disease, hematopathy, or malignant tumors receiving chemotherapy within the previous 3 months; and (4) life expectancy <48 h. This study was approved by the Institutional Review Board of PUMCH (approval number: JS-1170), and all methods were performed in accordance with the relevant guidelines and regulations. Informed consent was obtained from all patients, and the study was registered at chictr.org.cn (identifier ChiCTR-ROC-17010750).
The Berlin definition of ARDS included 4 aspects (9): (1) Timing: newly onset or worsening respiratory syndrome within 1 week of known clinical insults; (2) Chest imaging: Bilateral opacities—not fully explained by effusion, lobar/lung collapse, or nodules; (3) Origin of edeme: respiratory failure that can not be fully explained by cardiac failure or fluid overload and need objective assessment to exclude hydrostatic edema if no risk factors present, such as echocardiography; (4) Oxygenation: Mild ARDS 200 mm Hg < PaO2/FIO2 ≤300 mm Hg with PEEP or CPAP ≥5 cm H2O, Moderate ARDS 100 mm Hg < PaO2/FIO2 ≤200 mm Hg with PEEP≥5 cm H2O, Severe ARDS PaO2/FIO2 ≤100 mm Hg with PEEP ≥5 cm H2O.
Clinical diagnostic criteria of pneumonia (10–12): Pneumonia was diagnosed pulmonary infiltrates caused by infection, and at least two of the following findings: fever with a body temperature >38°C or hypothermia with a temperature <36°C; leukocytosis (>12,000 cells/mm3) or leukopenia (<4,000 cells/mm3); presence of newly purulent tracheal secretions; and a decrease in oxygenation.
Microbiological methods to diagnose pneumonia (12): lower respiratory tract specimens were obtained immediately after ICU admission and were sent to the PUMCH Clinical Microbiology Laboratory. Samples were obtained using non-invasive sampling and cultured semi-quantitatively. Endotracheal aspirates with >25 neutrophils on Gram's stain with <10 epithelial cells per high-power field were required for culture. If qualified samples of suputum were difficult to obtain or diagnose pneumonia, invasive respiratory sampling with bronchoalveolar lavage, protected specimen brush and blind bronchial sampling could be used. The Clinical Microbiology Laboratory determined antimicrobial susceptibility of isolated bacteria by means of the microdilution method (MicroScan System; Baxter health Care, West Sacramento, CA, USA). Results were interpreted according to breakpoints defined by the National Committee for Clinical Laboratory Standards (13). A. baumannii had to be the only pathogenic bacterium isolated from the enrolled patients. Bacterial colonization and ventilator-associated tracheobronchitis was carefully excluded by more than two intensivists, and other infections were cautiously excluded.
After enrollment, the following baseline information was collected: age, sex, comorbidities, treatment strategies, ventilator parameters, antibiotics, lymphocyte subsets, cytokines, and other laboratory data. SOFA score, Acute Physiology and Chronic Health Evaluation II (APACHE II) score and Clinical Pulmonary Infection Score (CPIS) within 24 h of admission were calculated, as well as the duration of mechanical ventilation, ICU stay and hospital stay, and 28-day mortality.
Lymphocyte subsets were evaluated in the Infection Laboratory. Peripheral blood mononuclear cells were stained with fluorescent monoclonal antibodies, then subjected to flow cytometric analysis (3-Color EPICS-XL Flow Cytometer; Beckman Coulter, Brea, CA, USA) to detect T cells (CD3+), CD4+ T cell subgroups, CD8+ T cell subgroups, B cells (CD19+), and NK cells (CD3+CD16+CD56+). Rate nephelometry (Array 360; Beckman Coulter) was used to measure serum levels of IgA, IgG, and IgM and complement factors C3 and C4.
Initial and targeted antibiotics: initial antibiotics were those given empirically at admission. Targeted antibiotics were those sensitive to A. baumannii in in vitro sensitivity tests, commonly including ampicillin/sulbactam, cefoperazone sulbactam, amikacin, ceftazidime averbatan, minocycline, carbapenems, tigecycline, and polymyxins.
The study protocol did not call for a standardized approach to critical care, and all treatment measures and drug selections were decided by the intensivists. As circulatory failure happened in most of patients, ECMO was not commonly used in this group.
Normally distributed data were expressed as the mean and standard deviation and were compared using Students' t-test. Non-normally distributed data were presented as median and interquartile range (IQR) and were analyzed using the non-parametric Mann–Whitney U-test. Categorical variables were expressed as number and percentage and were compared with the chi-square or Fisher's exact test. A Cox proportional hazards model were performed successively to determine the association between lymphocyte subsets and outcome. Receiver operating characteristic (ROC) curve analysis was performed to determine the discriminatory ability of parameters for predicting 28-day mortality. Youden's index was defined for points along the ROC curve, and the reliability was assessed by sensitivity and specificity. P < 0.05 was considered statistically significant. The results were expressed as P-value and hazard ratio (OR) with 95% confidence interval (CI). IBM SPSS 23.0 software was used for all statistical analyses (IBM Corp., Armonk, NY, USA).
A total of 576 ARDS patients based on the Berlin definition were admitted to our ICU during the study period. There were 364 ARDS patients caused by pulmonary infection; 347 of which were severe enough to receive mechanical ventilation or vasopressor treatment. Cultures from the lower respiratory tract of 131 patients were positive for A. baumannii. Among the 131 patients with pulmonary ARDS, 39 were infected with more than one pathogen, three died within 24 h after admission, and two were lost to follow-up. Finally, 87 pulmonary ARDS patients caused by A. baumannii pneumonia were enrolled in the study (Figure 1).
The median age of these ARDS patients caused by A. baumannii was 66 years (IQR 57–71 years), with males accounting for 70.1% (61/87). The 28-day mortality of these patients was 20.7% (18/87), and there were no significant differences in sex, age, and comorbidities between survivors and non-survivors. According to the 28-day mortality, the severity of the disease was slightly higher in non-survival group than that of survival group, as the differences in SOFA core, APACHE II score, and CPIS score at admission were not significant. The ICU and hospital stays were significantly longer in the survivors (20 vs. 10 days, P = 0.004, and 21 vs. 11.5 days, P = 0.005, respectively) (Table 1).
According to the 28-day mortality, there were no significant differences in vital signs and laboratory results between survivors and non-survivors. In terms of respiratory parameters, the PaO2: FiO2 (P/F) ratio (198 vs. 178, P = 0.173) was lower, the driving pressure (12 vs. 13 cmH2O, P = 0.083) was higher, and the peak airway pressure (21 vs. 24 cmH2O, P = 0.016) was significantly higher in the non-survivor group. As for treatment, the non-survivors received more renal replacement therapy and glucocorticoids (23.2 vs. 55.6%, P = 0.008 and 11.6 vs. 33.3%, P = 0.025, respectively). There was no significant difference between the two groups in the initial empirical antibiotic treatment, including the use of effective antibiotics against A. baumannii and the subsequent targeted anti-infective treatment. There was also no significant difference in the time interval from the empirical treatment to the targeted treatment. One patient in each group changed antibiotics because of adverse effects, which had no effect on the final statistical analysis (Table 2).
According to the 28-day mortality, there was no significant differences in procalcitonin, (1,3)-β-D Glucan, interleukin, Tumor necrosis factor-α and other inflammatory markers, as well as complement and immunoglobulin between the two groups. As for lymphocyte subsets: there was no significant difference in the total number of leukocytes and neutrophils between the two groups. The number of T cells (452 ± 322 vs. 729 ± 364/μl, P = 0.004), especially CD8+ T cells (104 ± 94 vs. 253 ± 191/μl, P = 0.002) was significantly lower in non-survivors than in survivors. The total number of lymphocytes and CD4+ T cells was also lower in non-survivors (765 ± 525 vs. 1,016 ± 526/μl, P = 0.084 and 332 ± 245 vs. 729 ± 364/μl, P = 0.011 respectively) (Table 3).
To clarify the factors independently associated with 28-day mortality, A cox proportional hazards model were performed. SOFA score, lactate level, P/F ratio and driving pressure that had been confirmed to be related to survival were included in the model as well as the parameters that were significantly different between the survivors and non-survivors in our study (Ppeak, RRT and Glucocorticoids use). Lymphocyte related parameters were also included in the model separately. CD8+ T cell count and its subtypes were independent factors associated with 28-day mortality of ARDS caused by A. baumannii pneumonia (CD8+ T cell count: OR 3.667, 95% CI 1.553–8.659, P = 0.003; CD8+CD28+ T cell count: OR 2.043, 95% CI 1.871–31.801, P = 0.005; CD8+CD38+ T cell count: OR 2.705, 95% CI 1.152–6.353, P = 0.022) (Table 4).
To further analyze the influence of lymphocyte subsets related parameters that differed significantly between survivors and non-survivors in the univariate analysis on prognosis, ROC curve analysis was performed. The CD8+ T cell count had the greatest discriminatory ability, with an area under the curve of 0.812. A cutoff value of 123 cells/μl at ICU admission was predictive of 28-day mortality for ARDS caused by A. baumannii with a sensitivity of 74.6% and specificity of 83.3% (P < 0.0001) (Figure 2).
Figure 2. ROC curve of CD8+ T cell count (A), CD8+CD28+ T cell count (B), and CD8+CD38+ T cell count (C) for the prediction of 28-day mortality.
Furthermore, we divided these ARDS patients into two groups according to the CD8+ T cell count (<123 and ≥123 cells/μl). the accumulative survival rate from the Kaplan-Meier curve was significantly lower and ventilation free day was significantly shorter (14.5 vs. 21 days, P = 0.001) in patients with a lower CD8+ T cell count, while 28-day mortality was significantly higher than that in patients with a higher CD8+ T cell count (46.9 vs. 5.7%, P < 0.0001). The patients with lower CD8+ T cell counts had lower P/F ratio, higher driving pressure at admission. The NLR, PCT level and CPIS at admission were also higher in patients with a lower CD8+ T cell count (Table 5, Figures 3A–E). We divided the cohort into different severity of ARDS according to admission P/F ratio and found that patients with increasing severity of ARDS had progressively lower CD8+ T cell counts (Figure 4).
Figure 3. (A–E) relationship between CD8+ T cell count and (A) P/F ratio; (B) Driving Pressure; (C) Neutrophil to lymphocyte ratio; (D) CPIS. (E) the Kaplan-Meier curve of patients with different CD8+ T cell counts. P/F ratio at D1 PaO2:FiO2 at admission; CPIS, clinical pulmonary infection score.
Figure 4. The relationship between the severity of ARDS and the CD8+ T cell counts. ARDS, acute respiratory distress syndrome.
To our knowledge, this is the first clinical study exploring the early alterations of lymphocyte subsets in ARDS caused by A. baumannii pneumonia. 28-day mortality of these patients was 20.7%. The T cell count, especially for CD8+ T cells, at admission was independently associated with 28-day mortality. The CD8+ T cell count was an early predictive marker for prognosis. So far, studies about A. baumannii and immunity have been limited to basic research, and there are limited data for clinical use. Our study first confirmed that T cell immunity might play an important role in ARDS caused by A. baumannii pneumonia, which could be important for clinical practice.
Immune disorder is the characteristic manifestation of sepsis, and increasing evidence shows that the immune system plays a bridging role between severe infection, organ damage and prognosis. However, the current view of how the two most complex syndromes, systemic inflammatory response syndrome and immune response syndrome, affect each other is still rudimentary (14, 15). The first step is to clarify the relationship between certain diseases and immune responses. There is a profound relationship between NLR and sepsis, NLR and ARDS, the CD4+ T cell count and carbapenem-resistant Enterobacteriaceae (CRE) infection (16), and there has been growing interest in identifying biological sub-phenotypes of ARDS patients with similar host-response features for prognostic enrichment (3). Basic immunological research into A. baumannii infection is in full swing, along with research into the mechanism of host defense against the infection. For example, there has been in-depth study of outer membrane protein A (5, 17). However, there has been little clinical research on the relationship between immunity to A. baumannii infection and prognosis. In this study, we found that the CD8+ and CD8+CD28+ T cell counts were independently associated with 28-day mortality in ARDS patients caused by A. baumannii. Clinically, this is the first-time providing evidence of Acinetobacter baumannii causing intracellular infection, which makes the understanding of this aspect go further.
A variety of immune cells are involved in resistance to ARDS caused by A. baumannii pneumonia (18). Large numbers of A. baumannii are taken up by alveolar macrophages as early as 4 h after infection. NK T cells are another cell type that act during the immune response against A. baumannii. Depletion of NK T cells in a murine pneumonia model caused impaired bacterial clearance and increased mortality. We found that the NK T cell count in non-survivors was lower than in survivors, which was consistent with previous research (19). DCs are the bridge between innate and adaptive immune responses, CD4+ T cells differentiate toward a Th1-polarizing phenotype through the activation of DCs (5). Subsequently, CD4+ T-helper cells support the production of specific antibodies by B cells and promote the bactericidal activity of phagocytes that together clear the infection. Our previous study also illustrated that the CD4+CD28+ T cell count was a useful marker for early diagnosis of CRE infection and outcome prediction (16). It was not surprising to see that innate immune cells were involved in the defense against A. baumannii, but with the ability to cause intracellular infection, A. baumannii pneumonia should induce cellular immunity response. However, the relationship between the CD8+ T cell count and A. baumannii has rarely been studied before (6). For the first time, our study illustrated the association between CD8+ T cells and ARDS caused by A. baumannii pneumonia. The possible mechanisms were as follows: (1) A. baumannii directly invaded alveolar epithelial cells, and cytotoxic CD8+ T cells directly recognized and killed infected epithelial cells (8); and (2) in addition to CD8+ T cells, the CD8+CD38+ T cells also differed significantly between survivors and non-survivors with A. baumannii infection. Inflammatory reaction, antigen exposure and environmental factors have been shown to allow differentiation of the NK-like CD8+ T cells. Therefore, CD8+ cells may also act indirectly against A. baumannii infection, which could be important for improving protective immunity (20). Further laboratory and clinical studies are needed to explore the specific mechanism of CD8+ T cells in A. baumannii infection.
Our study showed that the CD8+ T cell count was an independent risk factor for 28-day mortality of ARDS caused by A. baumannii pneumonia, which could be explained by the difference in physical and biological sub-phenotypes of ARDS patients (3). From the perspective of biological phenotype, multiple large clinical ARDS trials used P/F ratio and driving pressure for prognostic enrichment. The CD8+ T cell count was significantly associated with P/F ratio in ARDS caused by A. baumannii pneumonia and stratified according to P/F ratio. The proportion of patients with a lower CD8+ T cell count was significantly higher in severe ARDS patients. The CD8+ T cell count was also significantly associated with driving pressure, and patients with a lower T cell count needed higher driving pressure. Both severity of ARDS and driving pressure were strongly associated with survival rate (2, 21). From the perspective of biological phenotype, this might be related to the intensity of local inflammatory response. T cells, especially CD8+ T cells, were significantly decreased in non-survivors, resulting in a significant increase in NLR. The adaptive immune response to A. baumannii was reduced, while the non-specific innate immunity mainly composed of neutrophils was enhanced, which led to enhancement of the local pro-inflammatory response, manifesting as higher CPIS.
There were several limitations to this study. First, the study lasted almost 3 years with extremely strict inclusion criteria. Only septic patients with ARDS whose only pathogen was A. baumannii were enrolled, and they needed to receive mechanical ventilation or vasopressor treatment for enrollment. Despite the relatively small sample size, it was large enough to illustrate the role of lymphocytes, especially CD8+ T cells in patients with ARDS caused by A. baumannii. Second, the serum lymphocyte count might not be able to reflect the local infection situation. We need to further study the cell subgroup analysis of bronchoalveolar lavage fluid (BALF). However, there are challenges because, at present, the cell classification of BALF cannot be quantified as in routine blood tests, and there is no normal reference range for the cell classification; both of which need further research. Last, one key element that stands out from the existing studies is how much strain-to-strain variation of A. baumannii influences the interaction with host cells and observed phenotypes in vivo. A deeper understanding of the virulence factors of A. baumannii that are important for in vivo pathogenesis is needed (1).
Lower CD8+ T cell count was associated with higher severity and early mortality in ARDS patients caused by A. baumannii pneumonia, which could be valuable for outcome prediction.
The raw data supporting the conclusions of this article will be made available by the authors, without undue reservation.
The studies involving human participants were reviewed and approved by the Institutional Review Board of PUMCH (approval number: JS-1170). The patients/participants provided their written informed consent to participate in this study.
WC and NC contributed to the conception of the study, data interpretation, and drafted the manuscript. HW, JZ, DL, GB, WH, and JC contributed to data collection and critically revised the manuscript for important intellectual content. All authors approved the final version of the manuscript.
This work was supported by National Natural Science Foundation of China (Nos. 82072226 and 81601657), Beijing Municipal Science and Technology Commission (No. Z201100005520049), Non-profit Central Research Institute Fund of Chinese Academy of Medical Sciences (No. 2019XK320040), Tibet Natural Science Foundation [No. XZ2019ZR-ZY12(Z)], and Excellence Program of Key Clinical Specialty of Beijing in 2020 (No. ZK128001).
The authors declare that the research was conducted in the absence of any commercial or financial relationships that could be construed as a potential conflict of interest.
All claims expressed in this article are solely those of the authors and do not necessarily represent those of their affiliated organizations, or those of the publisher, the editors and the reviewers. Any product that may be evaluated in this article, or claim that may be made by its manufacturer, is not guaranteed or endorsed by the publisher.
ARDS, acute respiratory distress syndrome; A. baumannii, Acinetobacter baumannii; ICU, intensive care unit; NLR, neutrophil to lymphocyte ratio; TNF, tumor necrosis factor; DCs, dendritic cells; NK, natural killer cells; SOFA, sequential organ failure assessment score; CPIS, clinical pulmonary infection score; Ig, immunoglobulin; IQR, interquartile range; ROC, Receiver operating characteristic curve; OR, odds ratio; AUC, area under the curve; P/F, ratio PaOa: FiO2; BALF, bronchoalveolar lavage fluid.
1. Pires S, Parker D. Innate immune responses to Acinetobacter baumannii in the airway. J Interferon Cytokine Res. (2019) 39:441–4. doi: 10.1089/jir.2019.0008
2. Thompson BT, Chambers RC, Liu KD. Acute respiratory distress syndrome. N Engl J Med. (2017) 377:562–72. doi: 10.1056/NEJMra1608077
3. Wilson JG, Calfee CS. ARDS subphenotypes: understanding a heterogeneous syndrome. Crit Care. (2020) 24:102. doi: 10.1186/s13054-020-2778-x
4. Li WJ, Xiaolin A, Yuenan N, Zengpanpan Y, Zongan L. The association between the neutrophil-to-lymphocyte ratio and mortality in patients with acute respiratory distress syndrome: a retrospective cohort study. Shock. (2019) 51:161–7. doi: 10.1097/SHK.0000000000001136
5. Lee JS, Lee JC, Lee CM, Jung ID, Jeong YI, Seong EY, et al. Outer membrane protein A of Acinetobacter baumannii induces differentiation of CD4+ T cells toward a Th1 polarizing phenotype through the activation of dendritic cells. Biochem Pharmacol. (2007) 74:86–97. doi: 10.1016/j.bcp.2007.02.012
6. Qiu H, Zack L, Rhonda KL, Greg H, Xiaoling G, Hongbin Y, et al. Host resistance to intranasal Acinetobacter baumannii reinfection in mice. Pathog Dis. (2016) 74:ftw048. doi: 10.1093/femspd/ftw048
7. Qiu H, Kuolee R, Harris G, Chen W. Role of NADPH phagocyte oxidase in host defense against acute respiratory Acinetobacter baumannii infection in mice. Infect Immun. (2009) 77:1015–21. doi: 10.1128/IAI.01029-08
8. Choi CH, Lee JS, Lee YC, Park TI, Lee JC. Acinetobacter baumannii invades epithelial cells and outer membrane protein A mediates interactions with epithelial cells. BMC Microbiol. (2008) 8:216. doi: 10.1186/1471-2180-8-216
9. Force ARDT, Ranieri VM, Rubenfeld GD, Thompson BT, Ferguson ND, Caldwell E. Acute respiratory distress syndrome: the berlin definition. JAMA. (2012) 307:2526–33. doi: 10.1001/jama.2012.5669
10. American Thoracic Society Infectious Diseases Society of America. Infectious diseases society of america: guidelines for the management of adults with hospital-acquired, ventilator-associated, and healthcare- associated pneumonia. Am J Respir Crit Care Med. (2005) 171:388–416. doi: 10.1164/rccm.200405-644ST
11. Ceccato A, Panagiotarakou M, Ranzani OT, Martin-Fernandez M, Almansa-Mora R, Gabarrus A, et al. Lymphocytopenia as a predictor of mortality in patients with ICU-acquired pneumonia. J Clin Med. (2019) 8:843. doi: 10.3390/jcm8060843
12. Kalil AC, Metersky ML, Klompas M, Muscedere J, Sweeney DA, Palmer LB, et al. Management of adults with hospital-acquired and ventilator-associated pneumonia: 2016 clinical practice guidelines by the infectious diseases society of america and the american thoracic society. Clin Infect Dis. (2016) 3:e61–111. doi: 10.1093/cid/ciw353
13. Melvin PW. Methods for Dilution Antimicrobial Susceptibility Tests for Bacteria That Grow Aerobically, 11th edition. Clinical and Laboratory Standards Institute (2018). Available online at: https://clsi.org/standards/products/microbiology/documents/m07/
14. Rubio I, Osuchowski MF, Shankar-Hari M, Skirecki T, Winkler MS, Lachmann G, et al. Current gaps in sepsis immunology: new opportunities for translational research. Lancet Infect Dis. (2019) 19:E422–36. doi: 10.1016/S1473-3099(19)30567-5
15. Singer M, Deutschman CS, Seymour CW, Shankar-Hari M, Annane D, Bauer M, et al. The third international consensus definitions for sepsis and septic shock (Sepsis-3). JAMA. (2016) 315:801–10. doi: 10.1001/jama.2016.0287
16. Cheng W, Wang H, Zhang J, Bai G, Han W, Chen J, et al. Lymphocyte subset counts as diagnostic and prognostic markers for carbapenem-resistant Enterobacteriaceae (CRE) infection in critically ill patients. Int J Infect Dis. (2020) 96:315–22. doi: 10.1016/j.ijid.2020.04.072
17. Gaddy JA, Tomaras AP, Actis LA. The Acinetobacter baumannii 19606 OmpA protein plays a role in biofilm formation on abiotic surfaces and in the interaction of this pathogen with eukaryotic cells. Infect Immun. (2009) 77:3150–60. doi: 10.1128/IAI.00096-09
18. Qiu H, KuoLee R, Harris G, Van Rooijen N, Patel GB, Chen W. Role of macrophages in early host resistance to respiratory Acinetobacter baumannii infection. PLoS ONE. (2012) 7:e40019. doi: 10.1371/journal.pone.0040019
19. Tsuchiya T, Nakao N, Yamamoto S, Hirai Y, Miyamoto K, Tsujibo H. NK1.1(+) cells regulate neutrophil migration in mice with Acinetobacter baumannii pneumonia. Microbiol Immunol. (2012) 56:107–16. doi: 10.1111/j.1348-0421.2011.00402.x
20. Pita-López ML, Pera A, Solana R. Adaptive memory of human NK-like CD8(+) T-cells to aging, and viral and tumor antigens. Front Immunol. (2016) 7:616. doi: 10.3389/fimmu.2016.00616
Keywords: Acinetobacter baumannii, lymphocyte subset counts, acute respiratory distress syndrome, prognosis, ARDS
Citation: Cheng W, Zhang J, Li D, Bai G, Han W, Chen J, Wang H and Cui N (2021) Early Alterations of Lymphocyte Subsets in Acute Respiratory Distress Syndrome Caused by Acinetobacter baumannii Pneumonia: A Prospective Observational Study. Front. Med. 8:762724. doi: 10.3389/fmed.2021.762724
Received: 22 August 2021; Accepted: 13 September 2021;
Published: 11 October 2021.
Edited by:
Ling Liu, Southeast University, ChinaReviewed by:
Zhigang Chang, Beijing Hospital, ChinaCopyright © 2021 Cheng, Zhang, Li, Bai, Han, Chen, Wang and Cui. This is an open-access article distributed under the terms of the Creative Commons Attribution License (CC BY). The use, distribution or reproduction in other forums is permitted, provided the original author(s) and the copyright owner(s) are credited and that the original publication in this journal is cited, in accordance with accepted academic practice. No use, distribution or reproduction is permitted which does not comply with these terms.
*Correspondence: Hao Wang, bmV3d2FuZ2hhb0Bob3RtYWlsLmNvbQ==; Na Cui, cHVtY2hjbkAxNjMuY29t
Disclaimer: All claims expressed in this article are solely those of the authors and do not necessarily represent those of their affiliated organizations, or those of the publisher, the editors and the reviewers. Any product that may be evaluated in this article or claim that may be made by its manufacturer is not guaranteed or endorsed by the publisher.
Research integrity at Frontiers
Learn more about the work of our research integrity team to safeguard the quality of each article we publish.