- 1Center for Reproductive Medicine, Cheeloo College of Medicine, Shandong University, Jinan, China
- 2Key Laboratory of Reproductive Endocrinology of Ministry of Education, Shandong University, Jinan, China
- 3Shandong Key Laboratory of Reproductive Medicine, Jinan, China
- 4Shandong Provincial Clinical Research Center for Reproductive Health, Jinan, China
- 5National Research Center for Assisted Reproductive Technology and Reproductive Genetics, Shandong University, Jinan, China
Polycystic ovary syndrome (PCOS) is one of the most common endocrine disorders in women of reproductive age, which is characterized by ovulatory dysfunction, clinical and/or biochemical androgen excess, polycystic ovaries on ultrasound and genetic heterogeneity. It was well-accepted that many lncRNAs and mRNAs were associated with PCOS, however, remain unclear. Therefore, the purpose of our study was to examine different expression profiles of lncRNAs and mRNAs in ovarian granulosa cells (GCs) in PCOS and Controls, and identify the correlation between lncRNAs, mRNAs and clinical parameters. Sixty five PCOS patients and 65 Controls were enrolled in this study and adopted standard long agonist protocols or GnRH antagonist protocols. Then 6 GCs samples in each group were subjected to high-thoughput sequencing and the remaining samples were used for the further verification by quantitative real-time PCR (qRT-PCR). Gene Oncology (GO), Kyoto Encyclopedia Genes and Genomes (KEGG) enrichment analysis were performed. We predicted the relationship between lncRNAs and mRNAs by Cytoscape software. According to the expression level of lncRNAs, mRNAs and the clinical parameters, we also explored their relationship and evaluate their predictive values for embryos quality and PCOS. We identified 1,049 differential expressed lncRNAs and 3,246 mRNAs (fold-change ≥2, p-value < 0.05). Seven lncRNAs (NONHSAT101926.2, NONHSAT136825.2, NONHSAT227177.1, NONHSAT010538.2, NONHSAT191377.1, NONHSAT230904.1, ENST00000607307) and 3 mRNAs (EREG, ENTPD6, YAP1) were validated consistent with sequence profile. Seven lncRNAs were related to hormone level and follicle counts, 3 mRNAs had connections with lipid metabolism. The area under curve (AUC) of 7 lncRNAs were valuable in distinguishing patients with PCOS from Controls. The AUC of NONHSAT230904.1 and NONHSAT227177.1 were 0.6807 and 0.6410, respectively, for distinguishing whether the rate of high-quality embryos exceeds 50%. Our study showed that the GCs lncRNAs and mRNAs were involved in the occurrence and development of PCOS, which contribute to clarify the pathogenesis mechanism of PCOS.
Introduction
Polycystic ovary syndrome (PCOS) is a common endocrine disorder affecting 8–13% of women of reproductive age (1). It is primarily characterized by ovulatory dysfunction, clinical and/or biochemical androgen excess, polycystic ovaries on ultrasound, and genetic heterogeneity (2). There are many other symptoms or signs that accompany PCOS, such as hyperandrogenemia, sleep apnea, and metabolic problems including insulin resistance, diabetes, acanthosis nigricans, and non-alcoholic fatty liver (3). In addition, certain psychological problems can also arise in such cases (4). It is now believed that environmental and genetic factors cause PCOS (5), but its exact etiology remains unclear.
Long non-coding RNAs (lncRNAs) are a type of non-coding RNA with a length exceeding 200 nucleotides. They contribute to transcriptional and post-transcriptional regulation, and are broadly classified as signaling molecules, decoy molecules, guide molecules, or scaffold molecules (6). LncRNAs, as “transcriptional noise,” have relatively low expression levels compared with mRNAs and lack protein-coding capacity (7). In recent years, many studies have revealed that lncRNAs exhibit certain characteristics of mRNAs. For instance, lncRNAs are transcribed by RNA polymerase II, equipped with a 3′ poly (A) tail and a 5′ cap, and contain a promoter and multiple exons (8, 9). These features enable lncRNAs to interact with DNA, RNA, and proteins to directly regulate several biological processes, including chromatin modification, RNA transcription, pre-mRNA splicing, mRNA translation, and gene expression (10, 11). In addition, some studies have been found that lncRNAs have target-mimetic, sponge/decoy functions on microRNAs (12). Many studies also have revealed the roles of microRNA in various diseases (13, 14). At the same time, several studies have shown a wide range of interactions among mRNAs, microRNAs, and lncRNAs (15).
Several studies on lncRNAs have described the association with PCOS. The results of lncRNAs profiles in cumulus cells revealed that lncRNAs may contribute to the occurrence of PCOS and affect oocyte development (16). In addition, Liu et al. reported that lncRNAs may play a role in the proliferation and steroidogenesis of human granulosa cells (GCs) (17). The profiles of lncRNAs in GCs from women with PCOS with or without hyperandrogenism showed that lncRNAs may play key roles in steroidogenesis and metabolism (18). The lncRNA PVT1 regulates the secretion of estradiol (E2) and progesterone (P4), and the proliferation and apoptosis of GCs in PCOS via the lncRNA PVT1/microRNA-17-5p/PTEN axis (19). By constructing gene co-expression networks, it was revealed that there were many co-regulatory relationships among lncRNAs, mRNAs, and PCOS phenotypes from lncRNAs and mRNAs profiles in follicular fluid from mature and immature ovarian follicles (6). Furthermore, lncRNAs may have a certain impact on embryo quality. It was reported in the literature that lncRNAs could influence zebra embryonic development by regulating the Yax gene (20). However, so far, the mechanism of lncRNAs affect embryo quality is not very clear.
In addition, lncRNAs also played an important role in lipid metabolism. Low level of plasm high-density lipoprotein cholesterol (HDL-C) was a risk factor in cardiovascular (21). Xiao-Hua Yu et al. found that lncRNA kcnq1ot1 overexpression markedly decreased plasma HDL-C level, and kcnq1ot1 could promote lipid accumulation and accelerate the development of atherosclerosis through the miR-452-3p/HDAC3/ABCA1 pathway (22). Moreover, Xinping Wang et al. performed microarray analysis of long non-coding RNA expression profiles in low high-density lipoprotein cholesterol disease and found that certain lncRNAs could cause low high-density lipoprotein cholesterol disease (23). LncRNAs could modulate the transfer of lipid molecules. Apolipoprotein A1 (APOA1) is the primary protein in HDL complexes, which are in charge of the transport of excess cholesterol to the liver. The lncRNA APOA1-AS was observed negatively regulated APO A1 transcription in both in vivo and in vitro liver models (24).
Therefore, to explore the potential pathogenetic role of lncRNAs in patients with PCOS, we also carried out high-throughput sequencing of mRNAs expression profiles to conduct a network including mRNAs, lncRNAs and possible microRNAs.
However, the regulatory mechanisms and biological functions of lncRNAs in PCOS are still not fully understood. Hence, the aim of this study is to investigate the differences of lncRNA and mRNAs profiles between PCOS and Controls, and to discover novel prognostic markers and therapeutic targets of this condition.
Materials and Methods
Participants and Group Criteria
Ovarian GCs were collected from 65 PCOS patients (PCOS group) and 65 patients with an indication of male factor infertility serving as Controls (Control group), all of whom underwent in vitro fertilization (IVF) or intracytoplasmic sperm injection (ICSI) at the Center for Reproductive Medicine, Shandong University, between December 2018 and December 2019. These patients agreed to undergo long protocol and/or GnRH antagonist protocol to promote follicle development (25, 26). The PCOS patients were diagnosed based on Rotterdam revised criteria (27, 28) after excluding patients with Cushing's syndrome, congenital adrenal hyperplasia and androgen-secreting tumors. The exclusion criteria of the two groups were age ≥40 years old; body mass index (BMI) ≥30 kg/m2; basal follicle stimulating hormone (FSH) level >12 mIU/L; and systemic diseases, endometriosis, abnormal prolactin levels or thyroid function, immune diseases, recurrent abortion, abnormal chromosomal. This study was approved by the ethics committee of the Reproductive Hospital Affiliated to Shandong University. Written informed consent was obtained from each patient.
Clinical and Endocrine Parameters of PCOS Patients and Controls Who Were Subjected to High-Throughput Sequencing
We selected 6 GCs of PCOS patients and 6 GCs of Controls subjected to high-throughput sequencing. The clinical characteristics of the PCOS patients and Controls are shown in Table 1, including age, body mass index (BMI, kg/m2), fasting plasma glucose (FPG), plasma luteinizing hormone (LH), plasma follicle-stimulating hormone (FSH), plasma free testosterone (T), anti-Müllerian hormone (AMH). In addition, the antral follicle count (AFC) for follicles with a diameter of 2–9 mm was determined by transvaginal ultrasound. It should be note that increasing BMI appears to be causal for PCOS (29), and PCOS patients with high BMI could be frequently observed in clinical practice. For this reason, the difference in BMI found between PCOS and control groups was not taken into account.
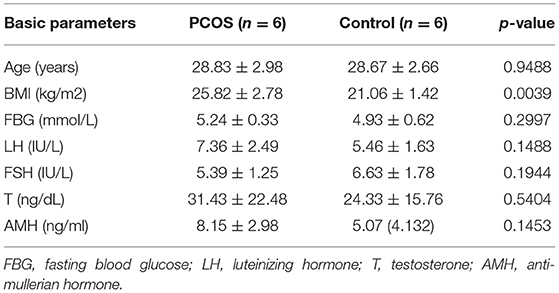
Table 1. Clinical and endocrine parameter of PCOS patients and controls enrolled in high throughput sequencing.
Follicular Fluid Collection and Retrieval of Ovarian Granulosa Cells
The two groups underwent controlled ovarian stimulation (COS) as described above. All patients were administered chorionic gonadotropin (hCG) at a dose of 4,000–8,000 IU was intramuscularly injected when at least two dominant follicles reached 18–20 mm in diameter. Oocytes were retrieved 36 h after hCG injection, while GCs were collected from the follicular fluid without blood contamination (30). GCs were purified by magnetic-activated cell sorting (Miltenyi Biotec, Bergisch Gladbach, Germany), in accordance with the manufacturer's instructions. GCs and follicular fluid samples were stored at −80°C. Total RNA was extracted from GCs using TRIzol Reagent (Life Technologies, Shanghai, China), in accordance with the manufacturer's instructions.
Library Construction for RNA-Seq and Sequencing Procedures
To assess the total RNA, Agilent Bioanalyzer 2,100 (Agilent Technologies, CA, USA) was used to evaluate the RNA integrity number (RIN). Qubit®3.0 Fluorometer (Thermo Fisher, USA) was used to assess total RNA levels and purity. We used the TruSeq®Stranded Total Sample Preparation kit (Illumina, USA) to prepare the strand-specific libraries strictly following the manufacturer's instructions. Purified libraries were quantified using Qubit® 2.0 Fluorometer (Life Technologies, USA) and confirmed using Agilent 2,100 Bioanalyzer (Agilent Technologies, USA) to confirm the insert size and calculate the molar concentration. Then, cluster was generated by cBot with the library diluted to 10 pM, followed by sequencing on HiSeq X Ten (Illumina, USA). The library construction and sequencing were performed at Shanghai Sinomics Corporation.
LncRNA Microarray Analysis
GCs selected for microarray analysis were collected from 6 PCOS patients and 6 Controls. To preprocess the sequenced raw reads, we filtered out rRNA reads, sequencing adapters, short-fragment reads, and other low-quality reads. We mapped the cleaned reads to the human GRCh 38 reference genome using Tophat v2.0.9, allowing two mismatches. After genome mapping, Cufflinks v2.1.1 was run with a reference annotation to generate FPKM values for known gene models, and FPKM values refer to fragments per kilobase of exon model per million mapped fragments. We used Cuffdiff to identify these differentially expressed genes and set the p-value significance threshold in multiple tests. According to the FPKM of each sample, the fold changes were estimated. Finally, hierarchical clustering was performed to display the genes with distinct expression patterns among the samples. All raw sequencing data have been submitted to the Gene Expression Omnibus (GEO) database (https://www.ncbi.nlm.nih.gov/geo/).
Pathway Analysis
Gene Ontology (GO; www.geneontology.org/) analysis was mainly used to analyze the main function of putative target genes. And Kyoto Encyclopedia Genes and Genomes (KEGG; www.genome.jp/kegg) analysis was performed to identify molecular pathways that were potentially altered.
Quantitative Real-Time PCR (qRT-PCR)
To confirm the microarray results, we chose some lncRNAs and mRNAs to test their expression in more GCs samples, in line with the screening criteria, including |fold-change| ≥2, p-value < 0.05, sequence length <3,000 bp, and count >10. We used about 30 PCOS patients and 30 Control patients to confirm the expression trends of every lncRNAs and mRNAs that we chose. Due to the limited size of RNA and cDNA from every GCs, a total of 59 PCOS GCs and 59 Control GCs were enrolled, which were enough to validate these lncRNAs and mRNAs we screened. Total RNA was extracted from GCs using TRIzol Reagent (Life Technologies, Shanghai, China) and reversed-transcribed into cDNA using the PrimeScript™ RT Reagent Kit with gDNA Eraser (Takara, China), in accordance with the manufacturer's instructions. We quantified the levels of lncRNAs and mRNAs that we chose by qRT-PCR on a LightCycler 480 system, in accordance with the manufacturer's instructions with TB Green™ Premix Ex Taq™ II (Takara, China). We normalized the levels of lncRNAs and mRNAs using GAPDH expression. Finally, we calculated the relative expression level of each lncRNA by the 2−ΔΔCT method. The clinical and endocrine parameters of the PCOS group and Control group are presented in Table 2, while the primers used for qRT-PCR are presented in Table 3.
Predict the Relationship Between LncRNAs and MRNAs After Verification
We used Cytoscape software to predict the relationship between lncRNAs and mRNAs which have been verified by expanding number of samples in each group. By integrating the information of the lncRNAs and the relationship between the mRNAs, we could obtain a regulatory network diagram between the lncRNAs and mRNAs.
Statistical Analysis
We used SPSS 26.0 (SPSS, Chicago, IL, USA), GraphPad Prism 8.0 (GraphPad Software, CA, USA), and R studio for the statistical analyses. Cytoscape was used to construct a lncRNA–microRNA-mRNA interaction network. Data are reported as mean ± standard deviation. Student's t-test was used for between-group comparisons, parameters not normally distributed were presented as median and compared by non-parametric test. The Pearson's correlation analysis was used for analyzing linear associations. Receiver operating characteristic (ROC) curve analysis was used for assessing the efficiency of lncRNAs in distinguishing PCOS samples from Controls and different quality of embryos. A p-value of < 0.05 was regarded as indicating statistical significance.
Results
Expression Profiles of LncRNAs and MRNAs in PCOS Patients and Controls
In our study, 54,615 lncRNAs and 123,141 mRNAs were detected in six PCOS patients and six Control patients by the high-throughput sequencing of lncRNAs. A Volcano plot was used to present the p-values and fold-changes of lncRNAs and mRNAs that were differentially expressed between the two groups. A heatmap was used to show the expression profiles of lncRNAs and mRNAs in PCOS patients and Controls. To obtain more detailed results, hierarchical clustering analysis was used to reveal the distribution of each of the lncRNAs and mRNAs (Figures 1A,B).
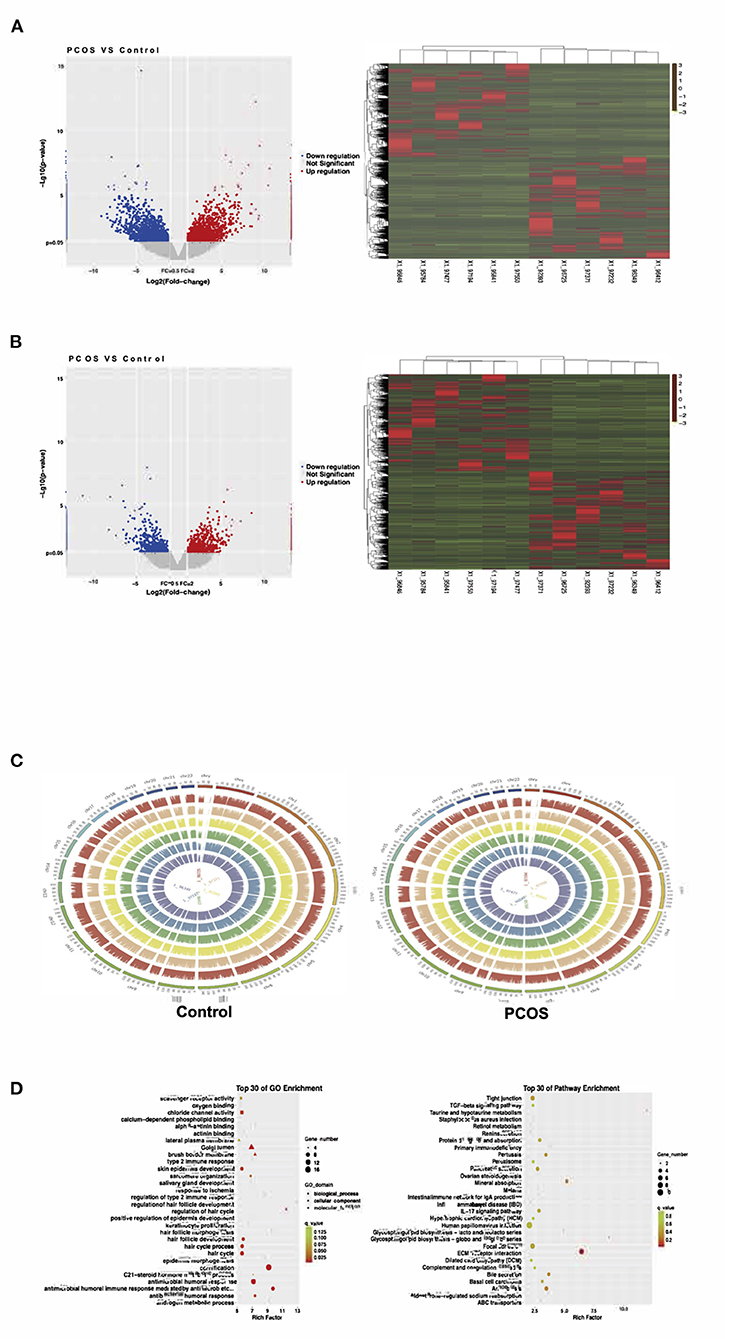
Figure 1. The expression of ovarian granulosa cells mRNAs and lncRNAs from polycystic ovary syndrome (PCOS) patients and controls. (A) Volcano map and Heat map of differentially expressed mRNAs (p < 0.05). (B) Volcano map and Heat map of differentially expressed lncRNAs (p < 0.05). (C) The distribution of differentially expressed lncRNAs in human chromosomes. (D) Gene Ontology (GO) and Kyoto Encyclopedia of Genes and Genomes (KEGG) pathway analysis for all target genes.
Compared with the levels in the Controls, the expression of 1,049 lncRNAs differed (|fold-change| ≥2, p-value < 0.05), of which 517 were upregulated and 532 downregulated. According to the same screening criteria, 3,246 mRNAs presented differential expression, with 1,551 upregulated and 1,695 downregulated in PCOS. Tables 4, 5 showed the top 10 most upregulated and downregulated lncRNAs and mRNAs according to the fold changes and p-values. The distribution of differentially expressed lncRNAs in the human chromosomes is illustrated in Figure 1C. These differentially expressed lncRNAs mainly derived from Chr.1 (10.20%, 107/1049), followed by Chr.2 (7.24%, 76/1049) and Chr.17 (6.39%, 67/1049). It should be note that the X and Y chromosome in human have both homologous and non-homologous part, and the alleles only present on homologous chromosome part, which lead to a small part of alleles were aligned to the Y chromosome.
Gene Ontology and Kyoto Encyclopedia of Genes and Genomes Pathway Analyses
GO and KEGG were used to predict the functions of genes. The top 30 GO and KEGG enriched categories were determined (Figure 1D). The prominent GO enriched categories were associated with androgen and steroid hormone metabolism. The pathways closely linked to PCOS in the KEGG analysis were ovarian steroid production, lipid metabolism, immune signaling pathways, and the PI3K–Akt signaling pathway.
Confirmation of LncRNA and mRNA Expression
In order to conduct follow-up study, 34 lncRNAs and 10 mRNAs were randomly selected to verify their expression trend in PCOS group and control group by qRT-PCR. These lncRNAs and mRNAs were verified with 30 ovarian GC samples in each two groups. The screening criteria include p < 0.05, |fold-change| ≥2, sequence length <3,000bp and counts >10. After verification, it was found that the expression of 7 lncRNAs (NONHSAT101926.2, NONHSAT136825.2, NONHSAT227177.1, NONHSAT010538.2, NONHSAT191377.1, NONHSAT230904.1, ENST00000607307) and 3 mRNAs (YAP1, ENTPD6 and EREG) among the lncRNAs and mRNAs that have been screened were consistent with the sequencing results. The results in this paper showed that the expression trends of these lncRNAs and mRNAs were consistent with the sequencing results (Figure 2). The expression levels of 4 lncRNAs (NONHSAT101926.2, NONHSAT136825.2, NONHSAT227177.1, NONHSAT010538.2) among the 7 lncRNAs were consistent with the sequencing results with a significant difference between the two groups (p < 0.05). Meanwhile, the changes of the levels of the other 3 lncRNAs (NONHSAT191377.1, NONHSAT230904.1, ENST00000607307) lost statistical significance (p > 0.05). The expression levels of YAP1 among the 3 mRNAs were significant (p < 0.05), and the changes of the levels of the other 2 mRNAs (ENTPD6, EREG) did not reach statistical significance (p > 0.05) (Figure 2). The details of the fold changes and p-values of these lncRNAs and mRNAs as mentioned above are shown in Table 6.
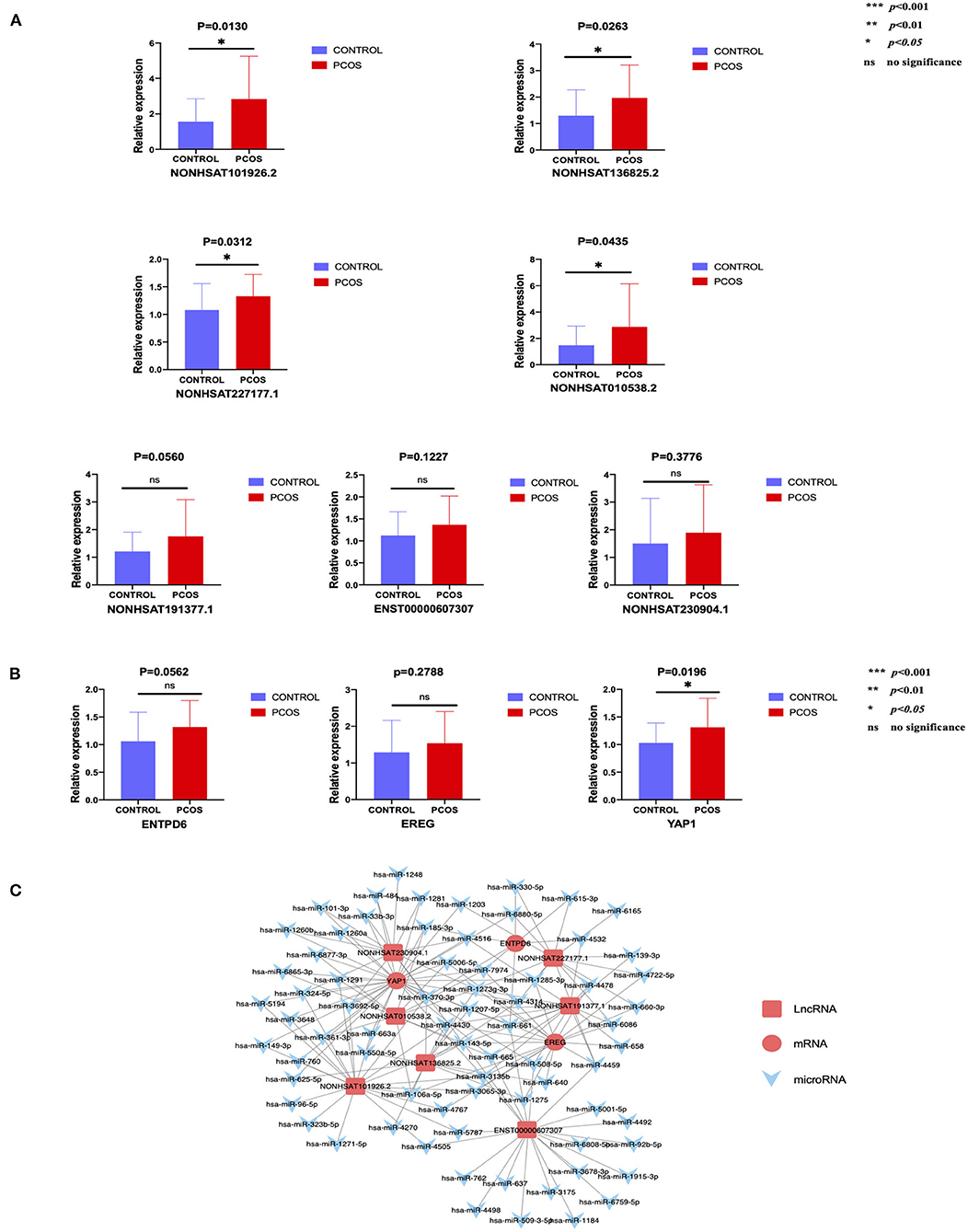
Figure 2. The expression level of lncRNAs and mRNAs conforming to the sequencing results and the predicted network regulatory relationship between them. (A) 7 lncRNAs that meet the trend of sequencing results. (B) 3 mRNAs that meet the trend of sequencing results. (C) Possible regulatory relationship between lncRNAs and mRNAs.
Construction of the LncRNA–MicroRNA-MRNA Interaction Network
According to our verification results, Cytoscape software was used to construct a lncRNA–microRNA-mRNA interaction network (Figure 2) to further speculate on the possible mutual regulatory relationship between lncRNA, microRNA and mRNA.
Analysis of the Correlation Between LncRNAs and Clinical Parameters
Pearson's correlation analysis was performed between several PCOS phenotypes and the expression of mRNAs (YAP1, EREG, ENTPD6) and lncRNAs (NONHSAT101926.2, NONHSAT136825.2, NONHSAT227177.1, NONHSAT010538.2, NONHSAT191377.1, ENST00000607307, NONHSAT230904.1), which have been confirmed consistent with sequencing results. The results showed that EREG had positive relationship of high-density-lipoprotein c (HDL-c) and apolipoprotein A1 (ApoA1), ENTPD6 had positive relationship of APO A1 (Figure 3). On the other hand, significant negative relationships of plasma E2, HDL-c, and ApoA1 levels with NONHSAT101926.2 were noted. In contrast to the case in Controls, the plasma LH level was positively correlated with NONHSAT230904.1 and NONHSAT227177.1; such a correlation was also noted between plasma basic FSH and NONHSAT227177.1. The LH/FSH ratio was positively correlated with NONHSAT010538.2 expression. Additionally, the endometrial thickness on the day of trigger, plasma E2 level on the ovulation trigger day, number of dominant follicles and oocytes retrieved were all positively correlated with ENST00000607307. Plasma progesterone level on the ovulation day and number of oocytes retrieved were positively correlated with NONHSAT191377.1 (Figure 3). The ROC curve of all 7 lncRNAs demonstrated a better accuracy in distinguishing patients with PCOS from Controls (Figure 4; Table 7).
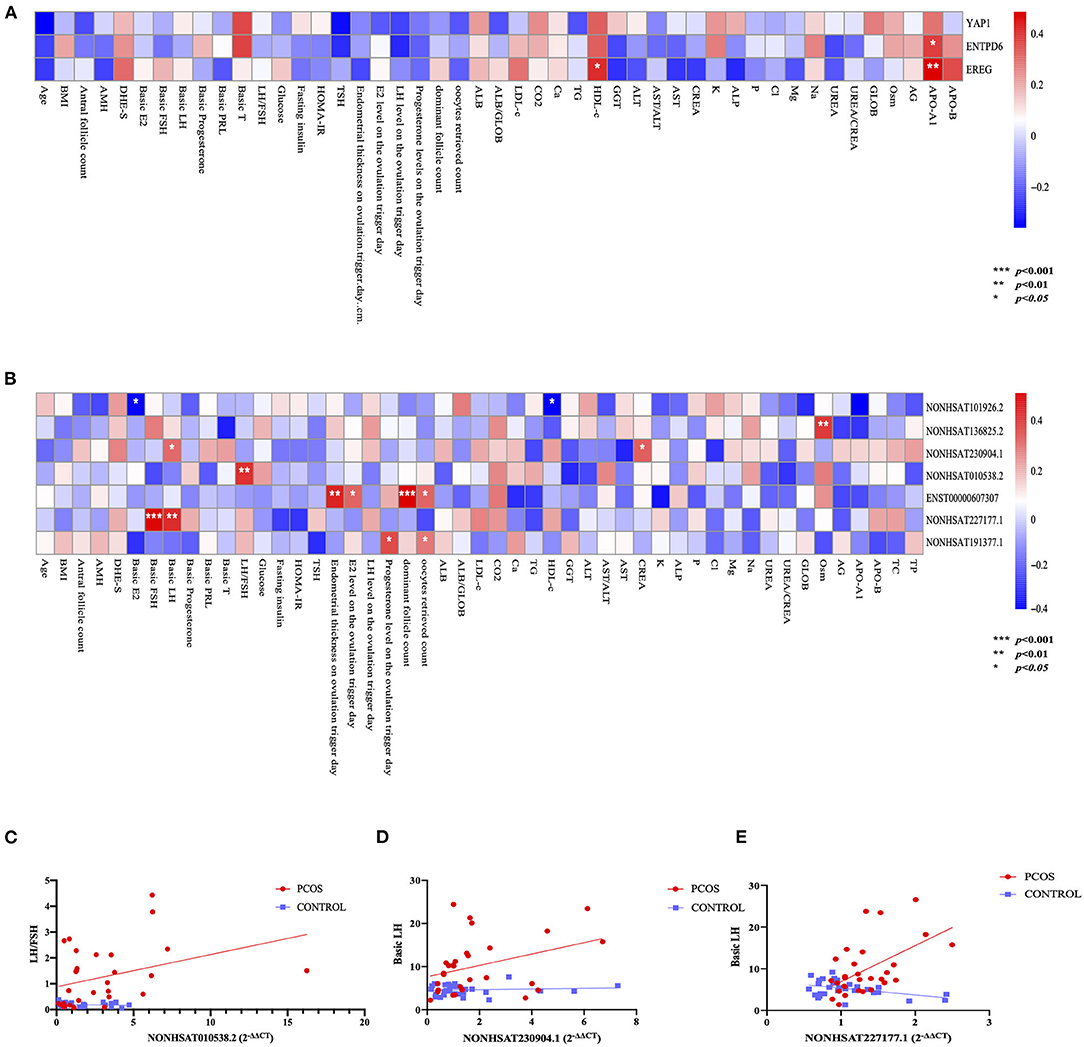
Figure 3. Correlation between polycystic ovary syndrome (PCOS) phenotypes and long non-coding RNAs (lncRNAs). (A) Binary correlation analysis between 3 mRNAs and PCOS phenotypes. (B) Binary correlation analysis between 7 lncRNAs and PCOS phenotypes. (C) Scatter diagram and linear correlation analysis of luteinizing hormone (LH)/follicle-stimulating hormone (FSH) ratio and NONHSAT010538.2 (r = 0.4905, p = 0.0059). (D) Scatter diagram and linear correlation analysis of basic luteinizing hormone (LH) level and NONHSAT230904.1 (r = 0.3813, p = 0.0412). (E) Scatter diagram and linear correlation analysis of basic luteinizing hormone (LH) level and NONHSAT227177.1 (r = 0.5046, p = 0.0045).
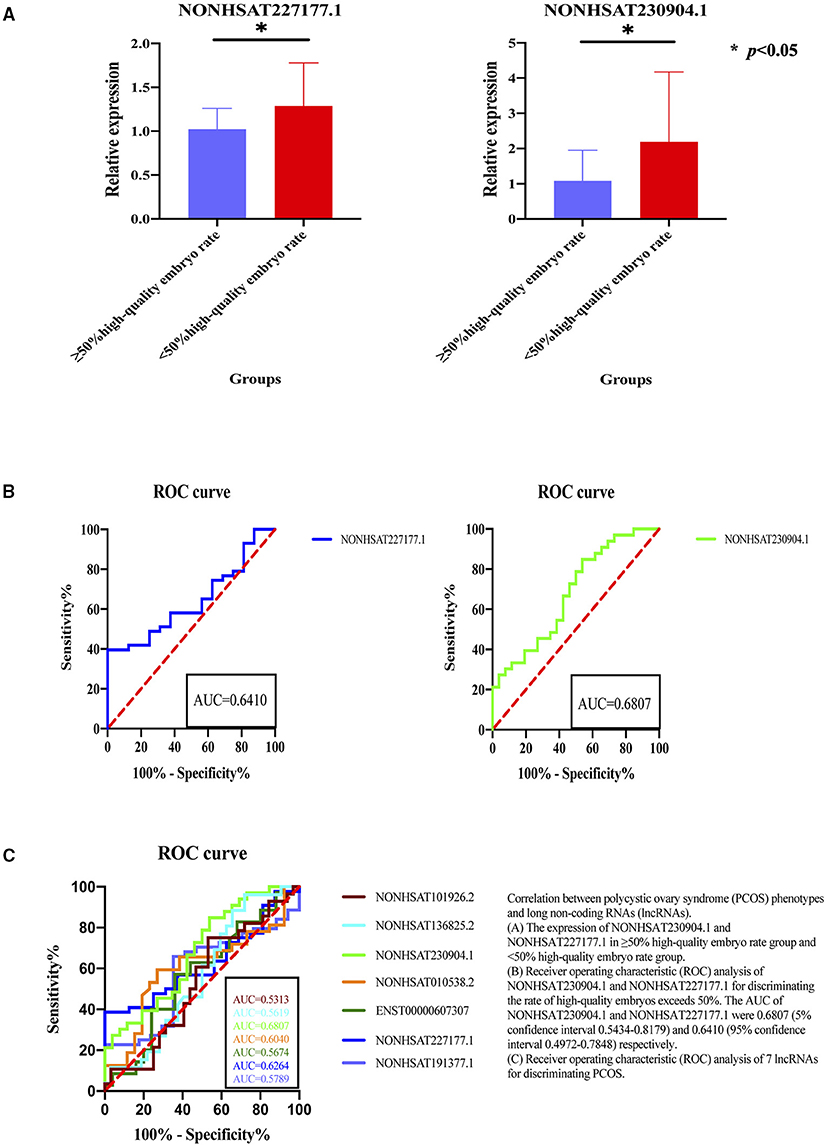
Figure 4. Correlation between polycystic ovary syndrome (PCOS) phenotypes and long non-coding RNAs (lncRNAs). (A) The expression of NONHSAT230904.1 and NONHSAT227177.1 in ≥50% high-quality embryo rate group and <50% high-quality embryo rate group. (B) Receiver operating characteristic (ROC) analysis of NONHSAT230904.1 and NONHSAT227177.1 for discriminating the rate of high-quality embryos exceeds 50%. The AUC of NONHSAT230904.1 and NONHSAT227177.1 were 0.6807 (5% confidence interval 0.5434-0.8179) and 0.6410 (95% confidence interval 0.4972–0.7848) respectively. (C) Receiver operating characteristic (ROC) analysis of 7 lncRNAs for discriminating PCOS. *p < 0.05.
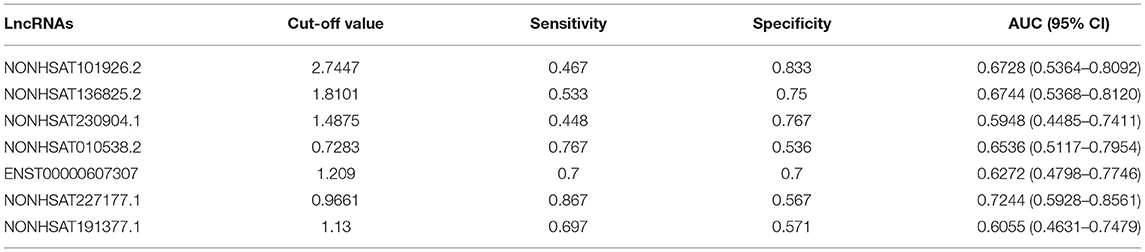
Table 7. Receiver operating characteristic (ROC) analysis of 7 lncRNAs to determine effectiveness in diagnosing PCOS.
According the quality of embryos standard: low-quality embryos have 2–7 blastomeres (fragmentation >20%) and high-quality embryos have 8 or more blastomeres (fragmentation ≤ 20%) (31), we divided the patients into two groups: the group with high-quality embryos exceeding 50% of the total number of embryos (≥50% high-quality embryo rate) and the group with high-quality embryos below 50% (<50% high-quality embryo rate). We analyzed the expression of lncRNAs between different embryo quality (rate of high-quality embryos exceeds 50% or not) and the results showed that the NONHSAT230904.1 and NONHSAT227177.1 expressed significantly different (Figure 4). Further, these two lncRNAs had a fine discrimination in embryo quality, with the AUC of were 0.6807 (95% CI, 0.5434–0.8179) and 0.6410 (95% CI 0.4972–0.7848), for NONHSAT230904.1 and NONHSAT227177.1, respectively (Figure 4).
Discussion
High-throughput sequencing technology could provide a wealth of information about coding and non-coding RNAs that may be involved in PCOS. In this study, seven unfamiliar lncRNAs showed significant increases in PCOS patients. Functional enrichment of the lncRNAs and mRNAs differentially expressed in PCOS suggested their associations with androgen, steroid hormone metabolism, ovarian steroid production, lipid metabolism, immune signaling pathways, and the PI3K–Akt signaling pathway.
The mRNAs that were selected at random for screening, including EREG, YAP1, ENTPD6, were all upregulated in PCOS patients. So far, the research on PCOS for these three mRNAs has been covered, but there are limitations.
EREG can regulate the proliferation and apoptosis of GCs, and is positively correlated with the proliferation of ovarian GCs (32). Some studies have suggested that it is also related to insulin secretion (33). YAP1 participates in many signaling pathways that regulate organic morphology, including ovarian enlargement, which is a major manifestation of PCOS. YAP1 is highly expressed in the GCs of PCOS patients (34). Testosterone (T) and estradiol (E2) induce the hyperactivation of YAP1 to stimulate GC proliferation, which may participate in hyperandrogenism-induced oligo-ovulation (35). In addition, the overexpression of YAP can promote the proliferation of human pancreatic β cells and maintain their insulin secretion (36). Regarding ENTPD6, in research on obesity using genetic, functional, and computational follow-up analyses, Turcot et al. found that it may play a role in controlling energy intake and consumption, but further research on this is still needed (37). In addition, Rich et al. performed a genome-wide association study on the acute insulin response to glucose in Hispanic Americans, finding that ENTPD6 may be related to pancreatic β-cell response, the acute insulin response to glucose (AIRg) (38). According to the above results, EREG, YAP1 and ENTPD6 may be involved in the pathogenesis of PCOS, but the detailed pathogenic mechanisms remain unclear. Further experiments would be performed to demonstrate and explain the mechanism of action.
In our profiles, GO analysis indicated that the differentially expressed mRNAs are mainly related to androgen and steroid hormone metabolism. Meanwhile, the KEGG pathway analysis results indicated that these aberrantly expressed mRNAs were associated with ovarian steroid production, lipid metabolism, immune signaling pathways, and the PI3K–Akt signaling pathway, among others. In recent years, many studies have been performed on the involvement of lncRNAs in the pathogenesis of PCOS. Some sequencing results were also obtained showing that there were more significant differences in coding and non-coding RNAs between PCOS patients and Controls. Jiao et al. conducted a study on the characterization of lncRNA and mRNA profiles in follicular fluid from mature and immature ovarian follicles of women and PCOS patients. Their sequencing profiles showed that the lncRNAs with significant differences in expression were mainly linked to metabolism, nervous system, and olfactory transduction pathways (6). Moreover, deep sequencing of mRNA and lncRNA profiles in a letrozole-induced PCOS rat model showed that the differentially expressed mRNAs were associated with biological adhesion, reproduction, and metabolic processes, and they were also enriched in several specific signaling pathways, including insulin resistance, steroid hormone biosynthesis, PPAR signaling pathway, cell adhesion molecules, autoimmune thyroid disease, and AMPK signaling pathway (39). Furthermore, a pilot study found significant or borderline significant increases in several parameters reflecting intestinal barrier dysfunction and inflammation in PCOS patients, suggesting that PCOS may be related to metabolic disorders (40). This summary from previous works shows that our sequencing profiles exhibit some differences from others' results. The reason for these discrepancies may be that we selected different sample tissues.
In recent years, many studies focused on the relationship between lncRNAs and PCOS. Li et al. found that the lncRNA SRLR participated in PCOS by upregulating IL-6 and promoting apoptosis of human granulosa-like tumor cells (KGN) (41). Geng et al. showed that the lnc-MAP3K13-7:1 inhibited the ovarian GCs proliferation in PCOS via DNMT1 inhibiting CDKN1A/p21 expression (42). Some PCOS animal model studies also provided evidences that lncRNAs might be used as therapeutic targets for PCOS. Jiang et al. observed a down-regulated of lncRNA HOTAIR could alleviates PCOS (43). And Yang et al. showed that silencing the lncRNA UCA1 could inhibit the development of PCOS via regulating PI3K-AKT signaling pathway (44). Moreover, downregulating lncRNA NEAT1 could also inhibit apoptosis and improve cell proliferation of ovarian GCs via microRNA-381/IGF1 axis, which associated with improved pathological process of PCOS (45). And our results exhibited that the expression of lncRNA was also significantly correlated with abnormal hormone, plasma metabolites level and embryo quality: NONHSAT101926.2 was mainly related to lipid metabolism, NONHSAT227177.1 and NONHSAT230904.1 were mainly related to plasma steroid hormones, NONHSAT010538.2 was positively associated with the LH/FSH ratio, while ENST00000607307 and NONHSAT191377.1 were related to plasma steroid hormones and follicle development. Moreover, we also observed high expression of NONHSAT230904.1 and NONHSAT227177.1 were associated with fewer high-quality embryos, which provided evidence that they could be related to embryo quality. Therefore, we speculate that these 7 lncRNAs and 3 mRNAs mentioned above were likely to be invovled in the pathogenesis of PCOS and might become a new molecular therapeutic targets for PCOS.
However, there are several limitations in this study. We only introduced our high-throughput sequencing profiles and they need to be confirmed on a larger cohort. Likewise, we have analyzed the relationships between lncRNAs, mRNAs and PCOS clinical parameters, but further in-depth research is needed to elucidate the exact mechanisms linking these RNAs to PCOS.
Conclusion
Our findings expanded the knowledge that abnormal expression of lncRNAs and mRNAs mentioned in present study in ovarian granulosa cells were associated with PCOS, which also could be reflected in abnormal hormone, plasma biomedical indicators and embryo quality. And these preliminary findings might enlight some new directions for understanding PCOS.
Data Availability Statement
The original contributions presented in the study are publicly available. This data can be found here: https://www.ncbi.nlm.nih.gov/geo/query/acc.cgi?acc=GSE173160.
Ethics Statement
The studies involving human participants were reviewed and approved by the Ethics Committee of the Reproductive Hospital Affiliated to Shandong University. The patients/participants provided their written informed consent to participate in this study.
Author Contributions
YZ and YS conceived and designed this study. RW, WC, and XY collected clinical data. YZ, YB, PL, LF, YW, and SZ contributed to statistical analysis. YZ drafted the manuscript. YZ, YB, and YS participated in the discussion and critically revised the article. All authors contributed to the article and approved the submitted version.
Funding
This study was supported by National Key R&D Program of China (2018YFC1003202 and 2017YFC1001004) and Taishan scholar project special funds (No. ts201712103).
Conflict of Interest
The authors declare that the research was conducted in the absence of any commercial or financial relationships that could be construed as a potential conflict of interest.
Publisher's Note
All claims expressed in this article are solely those of the authors and do not necessarily represent those of their affiliated organizations, or those of the publisher, the editors and the reviewers. Any product that may be evaluated in this article, or claim that may be made by its manufacturer, is not guaranteed or endorsed by the publisher.
Acknowledgments
The authors thank all participants and their families.
Supplementary Material
The Supplementary Material for this article can be found online at: https://www.frontiersin.org/articles/10.3389/fmed.2021.741803/full#supplementary-material
References
1. Teede H, Misso M, Tassone EC, Dewailly D, Ng EH, Azziz R, et al. Anti-mullerian hormone in PCOS: a review informing international guidelines. Trends Endocrinol Metabolism. (2019) 30:467–78. doi: 10.1016/j.tem.2019.04.006
2. Goodarzi MO, Dumesic DA, Chazenbalk G, Azziz R. Polycystic ovary syndrome: etiology, pathogenesis and diagnosis. Nat Rev Endocrinol. (2011) 7:219–31. doi: 10.1038/nrendo.2010.217
3. Patel S. Polycystic ovary syndrome (PCOS), an inflammatory, systemic, lifestyle endocrinopathy. J Steroid Biochem Mol Biol. (2018) 182:27–36. doi: 10.1016/j.jsbmb.2018.04.008
4. Witchel SF, Teede HJ, Peña AS. Curtailing PCOS. Pediatr Res. (2020) 87:353–61. doi: 10.1038/s41390-019-0615-1
5. Ajmal N, Khan SZ, Shaikh R. Polycystic ovary syndrome (PCOS) and genetic predisposition: A review article. Eur J Obstet Gynecol Reprod Biol X. (2019) 3:100060. doi: 10.1016/j.eurox.2019.100060
6. Jiao J, Shi B, Wang T, Fang Y, Cao T, Zhou Y, et al. Characterization of long non-coding RNA and messenger RNA profiles in follicular fluid from mature and immature ovarian follicles of healthy women and women with polycystic ovary syndrome. Human Reprod. (2018) 33:1735–48. doi: 10.1093/humrep/dey255
7. Ponjavic J, Ponting CP, Lunter G. Functionality or transcriptional noise? Evidence for selection within long noncoding RNAs. Genome Res. (2007) 17:556–65. doi: 10.1101/gr.6036807
8. Ma L, Bajic VB, Zhang Z. On the classification of long non-coding RNAs. RNA Biol. (2013) 10:925–33. doi: 10.4161/rna.24604
9. Devaux Y, Zangrando J, Schroen B, Creemers EE, Pedrazzini T, Chang CP, et al. Long noncoding RNAs in cardiac development and ageing. Nat Rev Cardiol. (2015) 12:415–25. doi: 10.1038/nrcardio.2015.55
10. Ponting CP, Oliver PL, Reik W. Evolution and functions of long noncoding RNAs. Cell. (2009) 136:629–41. doi: 10.1016/j.cell.2009.02.006
11. Geisler S, Coller J. RNA in unexpected places: long non-coding RNA functions in diverse cellular contexts. Nat Rev Mol Cell Biol. (2013) 14:699–712. doi: 10.1038/nrm3679
12. Paraskevopoulou MD, Hatzigeorgiou AG. Analyzing MiRNA-LncRNA Interactions. Meth Mol Biol. (2016) 1402:271–86. doi: 10.1007/978-1-4939-3378-5_21
13. He T, Sun Y, Zhang Y, Zhao S, Zheng Y, Hao G, et al. MicroRNA-200b and microRNA-200c are up-regulated in PCOS granulosa cell and inhibit KGN cell proliferation via targeting PTEN. Reprod Biol Endocrinol. (2019) 17:68. doi: 10.1186/s12958-019-0505-8
14. Valdmanis PN, Kim HK, Chu K, Zhang F, Xu J, Munding EM, et al. miR-122 removal in the liver activates imprinted microRNAs and enables more effective microRNA-mediated gene repression. Nat Commun. (2018) 9:5321. doi: 10.1038/s41467-018-07786-7
15. Mohr AM, Mott JL. Overview of microRNA biology. Semin Liver Dis. (2015) 35:3–11. doi: 10.1055/s-0034-1397344
16. Huang X, Hao C, Bao H, Wang M, Dai H. Aberrant expression of long noncoding RNAs in cumulus cells isolated from PCOS patients. J Assist Reprod Genetics. (2016) 33:111–21. doi: 10.1007/s10815-015-0630-z
17. Liu YD, Li Y, Feng SX, Ye DS, Chen X, Zhou XY, et al. Long noncoding RNAs: potential regulators involved in the pathogenesis of polycystic ovary syndrome. Endocrinology. (2017) 158:3890–9. doi: 10.1210/en.2017-00605
18. Jin L, Yang Q, Zhou C, Liu L, Wang H, Hou M, et al. Profiles for long non-coding RNAs in ovarian granulosa cells from women with PCOS with or without hyperandrogenism. Reprod Biomed Online. (2018) 37:613–23. doi: 10.1016/j.rbmo.2018.08.005
19. Liu G, Liu S, Xing G, Wang F. lncRNA PVT1/MicroRNA-17-5p/PTEN axis regulates secretion of E2 and P4, proliferation, and apoptosis of ovarian granulosa cells in PCOS. Mol Ther Nucleic Acids. (2020) 20:205–16. doi: 10.1016/j.omtn.2020.02.007
20. Huang C, Zhu B, Leng D, Ge W, Zhang XD. Long noncoding RNAs implicated in embryonic development in Ybx1 knockout zebrafish. FEBS Open Bio. (2020) 11:1259–76. doi: 10.1002/2211-5463.13057
21. Feng M, Darabi M, Tubeuf E, Canicio A, Lhomme M, Frisdal E, et al. Free cholesterol transfer to high-density lipoprotein (HDL) upon triglyceride lipolysis underlies the U-shape relationship between HDL-cholesterol and cardiovascular disease. Eur J Prev Cardiol. (2020) 27:1606–16. doi: 10.1177/2047487319894114
22. Yu XH, Deng WY, Chen JJ, Xu XD, Liu XX, Chen L, et al. LncRNA kcnq1ot1 promotes lipid accumulation and accelerates atherosclerosis via functioning as a ceRNA through the miR-452-3p/HDAC3/ABCA1 axis. Cell Death Dis. (2020) 11:1043. doi: 10.1038/s41419-020-03263-6
23. Wang X, Guo S, Hu Y, Guo H, Zhang X, Yan Y, et al. Microarray analysis of long non-coding RNA expression profiles in low high-density lipoprotein cholesterol disease. Lipids Health Dis. (2020) 19:175. doi: 10.1186/s12944-020-01348-x
24. Mahpour A, Mullen AC. Our emerging understanding of the roles of long non-coding RNAs in normal liver function, disease, and malignancy. JHEP Rep. (2021) 3:100177. doi: 10.1016/j.jhepr.2020.100177
25. Shi Y, Wei D, Liang X, Sun Y, Liu J, Cao Y, et al. Live birth after fresh embryo transfer vs elective embryo cryopreservation/frozen embryo transfer in women with polycystic ovary syndrome undergoing IVF (FreFro-PCOS): study protocol for a multicenter, prospective, randomized controlled clinical trial. Trials. (2014) 15:154. doi: 10.1186/1745-6215-15-154
26. Liu F, Jiang Q, Sun X, Huang Y, Zhang Z, Han T, et al. Lipid metabolic disorders and ovarian hyperstimulation syndrome: a retrospective analysis. Front Physiol. (2020) 11:491892. doi: 10.3389/fphys.2020.491892
27. Lizneva D, Suturina L, Walker W, Brakta S, Gavrilova-Jordan L, Azziz R. Criteria, prevalence, and phenotypes of polycystic ovary syndrome. Fertility Sterility. (2016) 106:6–15. doi: 10.1016/j.fertnstert.2016.05.003
28. Rotterdam ESHRE/ASRM-Sponsored PCOS Consensus Workshop Group. Revised 2003 consensus on diagnostic criteria and long-term health risks related to polycystic ovary syndrome. Fertility Sterility. (2004) 81:19–25. doi: 10.1016/j.fertnstert.2003.10.004
29. Brower MA, Hai Y, Jones MR, Guo X, Chen YI, Rotter JI, et al. Bidirectional mendelian randomization to explore the causal relationships between body mass index and polycystic ovary syndrome. Human Reprod. (2019) 34:127–36. doi: 10.1093/humrep/dey343
30. Matsubara H, Ikuta K, Ozaki Y, Suzuki Y, Suzuki N, Sato T, et al. Gonadotropins and cytokines affect luteal function through control of apoptosis in human luteinized granulosa cells. J Clin Endocrinol Metab. (2000) 85:1620–6. doi: 10.1210/jcem.85.4.6509
31. Alpha Scientists in Reproductive Medicine and ESHRE Special Interest Group of Embryology. The Istanbul consensus workshop on embryo assessment: proceedings of an expert meeting. Human Reprod. (2011) 26:1270–83. doi: 10.1093/humrep/der037
32. Zheng Q, Zhou F, Cui X, Liu M, Li Y, Liu S, et al. Novel serum biomarkers detected by protein array in polycystic ovary syndrome with low progesterone level. Cell Physiol Biochem. (2018) 46:2297–310. doi: 10.1159/000489619
33. Kuntz E, Broca C, Komurasaki T, Kaltenbacher MC, Gross R, Pinget M, et al. Effect of epiregulin on pancreatic beta cell growth and insulin secretion. Growth Factors. (2005) 23:285–93. doi: 10.1080/08977190500233367
34. Jiang LL, Xie JK, Cui JQ, Wei D, Yin BL, Zhang YN, et al. Promoter methylation of yes-associated protein (YAP1) gene in polycystic ovary syndrome. Medicine. (2017) 96:e5768. doi: 10.1097/MD.0000000000005768
35. Ji SY, Liu XM, Li BT, Zhang YL, Liu HB, Zhang YC, et al. The polycystic ovary syndrome-associated gene Yap1 is regulated by gonadotropins and sex steroid hormones in hyperandrogenism-induced oligo-ovulation in mouse. Mol Human Reprod. (2017) 23:698–707. doi: 10.1093/molehr/gax046
36. Yuan T, Rafizadeh S, Azizi Z, Lupse B, Gorrepati KDD, Awal S, et al. Proproliferative and antiapoptotic action of exogenously introduced YAP in pancreatic beta cells. JCI Insight. (2016) 1:e86326. doi: 10.1172/jci.insight.86326
37. Turcot V, Lu Y, Highland HM, Schurmann C, Justice AE, Fine RS, et al. Protein-altering variants associated with body mass index implicate pathways that control energy intake and expenditure in obesity. Nat Genetics. (2018) 50:26–41. doi: 10.1038/s41588-018-0050-y
38. Rich SS, Goodarzi MO, Palmer ND, Langefeld CD, Ziegler J, Haffner SM, et al. A genome-wide association scan for acute insulin response to glucose in hispanic-americans: the insulin resistance atherosclerosis family study (IRAS FS). Diabetologia. (2009) 52:1326–33. doi: 10.1007/s00125-009-1373-0
39. Fu LL, Xu Y, Li DD, Dai XW, Xu X, Zhang JS, et al. Expression profiles of mRNA and long noncoding RNA in the ovaries of letrozole-induced polycystic ovary syndrome rat model through deep sequencing. Gene. (2018) 657:19–29. doi: 10.1016/j.gene.2018.03.002
40. Lindheim L, Bashir M, Münzker J, Trummer C, Zachhuber V, Leber B, et al. Alterations in gut microbiome composition and barrier function are associated with reproductive and metabolic defects in women with polycystic ovary syndrome (PCOS): a pilot study. PLoS ONE. (2017) 12:e0168390. doi: 10.1371/journal.pone.0168390
41. Li L, Zhu J, Ye F, Duan Z, Zhou J, Huang Z, et al. Upregulation of the lncRNA SRLR in polycystic ovary syndrome regulates cell apoptosis and IL-6 expression. Cell Biochem Function. (2020) 38:880–5. doi: 10.1002/cbf.3507
42. Geng X, Zhao J, Huang J, Li S, Chu W, Wang WS, et al. lnc-MAP3K13-7:1 inhibits ovarian GC proliferation in PCOS via DNMT1 downregulation-mediated CDKN1A promoter hypomethylation. Mol Ther. (2021) 29:1279–93. doi: 10.1016/j.ymthe.2020.11.018
43. Jiang B, Xue M, Xu D, Song J, Zhu S. Down-regulated lncRNA HOTAIR alleviates polycystic ovaries syndrome in rats by reducing expression of insulin-like growth factor 1 via microRNA-130a. J Cell Mol Med. (2020) 24:451–64. doi: 10.1111/jcmm.14753
44. Yang D, Wang Y, Zheng Y, Dai F, Liu S, Yuan M, et al. Silencing of lncRNA UCA1 inhibited the pathological progression in PCOS mice through the regulation of PI3K/AKT signaling pathway. J Ovarian Res. (2021) 14:48. doi: 10.1186/s13048-021-00792-2
Keywords: ovarian granulosa cells, lncRNA, mRNA, pcos, high-throughput sequencing
Citation: Zheng Y, Bian Y, Wu R, Chen W, Fu L, Li P, Wang Y, Yang X, Zhao S and Shi Y (2021) High-Throughput Sequencing Profiles About lncRNAs and mRNAs of Ovarian Granulosa Cells in Polycystic Ovary Syndrome. Front. Med. 8:741803. doi: 10.3389/fmed.2021.741803
Received: 15 July 2021; Accepted: 26 October 2021;
Published: 22 November 2021.
Edited by:
Simone Garzon, Verona University Hospital, ItalyReviewed by:
Yaqiong He, Shanghai Jiao Tong University, ChinaAlice Bongrani, INRA Center Val de Loire, France
Copyright © 2021 Zheng, Bian, Wu, Chen, Fu, Li, Wang, Yang, Zhao and Shi. This is an open-access article distributed under the terms of the Creative Commons Attribution License (CC BY). The use, distribution or reproduction in other forums is permitted, provided the original author(s) and the copyright owner(s) are credited and that the original publication in this journal is cited, in accordance with accepted academic practice. No use, distribution or reproduction is permitted which does not comply with these terms.
*Correspondence: Yuhua Shi, c2hpeXVodWEyMDAzJiN4MDAwNDA7MTI2LmNvbQ==