- 1Translational Lung Research Center (TLRC), German Center for Lung Research (DZL), University of Heidelberg, Heidelberg, Germany
- 2Department of Diagnostic and Interventional Radiology, University of Heidelberg, Heidelberg, Germany
- 3Department of Diagnostic and Interventional Radiology With Nuclear Medicine, Thoraxklinik at University of Heidelberg, Heidelberg, Germany
A Commentary on
Imaging Bronchopulmonary Dysplasia—A Multimodality Update
by Semple, T., Akhtar M. R., and Owens, C. M. (2017). Front. Med. 4:00088. doi: 10.3389/fmed.2017.00088
Chronic lung disease in infancy and early childhood poses a diagnostic challenge to medicine. (1) Entities are rare, with bronchopulmonary dysplasia (BPD) being the most prevalent in infancy and with increasing prevalence in grown-ups due to improved survival of prematurity. (2) Functional impairment is hard to measure because spirometry is practically impossible to perform at an early age. (3) Structural imaging may be a key component to diagnosis and monitoring, with computed tomography delivering high spatially resolved morphological information, but cumulative radiation dose and periprocedural efforts may be of high concern in radiation-susceptible young individuals and restrictive for imaging procedures (1). (4) Further, apart from definite diagnosis, therapeutic relevance should guide the decision to perform imaging tests and must be weighed against potential risks. Obviously, this somewhat trivial dogma holds true for all diagnostic tests in pediatric and adult clinical medicine, but it is specifically important in the research context where the generation of advances in knowledge and the testing of hypotheses may require a more liberal—yet well-considered—acquisition of study data, even in studies of human subjects. In the case of pediatric lung disease, imaging with structural and functional techniques may be the most comprehensive approach to characterize a pathophysiological process of the lungs and to study the natural evolution of findings and the changes induced by therapeutic intervention. In the case of new medication made available, imaging may provide a sensitive endpoint for measuring successful treatment (2).
Cystic fibrosis lung disease has been the most prominent example, for which sustained efforts in avoidance of radiation and improving imaging diagnostics have lead to the stepwise introduction of proton magnetic resonance imaging (MRI) for structural and functional lung disease, with a widely available MRI protocol, a scoring system for semi-quantification (3), cross-validation against CT (4), X-ray (5, 6), multiple breath wash-out (7) and spirometry (8), initial studies using MRI to monitor mid-term natural evolution (8), and as an endpoint for therapeutic intervention (6, 7, 9, 10). Of note, contrast-enhanced perfusion MRI is the first among many functional techniques to become widely available for the assessment of functional abnormalities in the CF lung (Figure 1) (11, 12). Besides, a multitude of novel functional techniques based on inhalational contrast agents such as hyperpolarized gas MRI using alternative nuclei, or non-contrast-dependent techniques such as Fourier Decomposition MRI and T1 mapping have driven deeper insight into structure-function relationships in cystic fibrosis lung disease (13–18). Logically, evidence from CF research as a model has been transferred to rarer pediatric lung diseases and vice versa, such as primary ciliary dyskinesia or BPD (19, 20). Although MRI has the advantage of relatively easy repeatability and “no dose,” its application in neonates and infants, especially in prematurity, may be cumbersome due to sedation, acquisition time, MRI-compatible equipment, lines, and drug delivery, and clinicians might demand a quick examination with highest structural resolution. Specific MRI scanners for neonates will probably remain a rare research tool in the very near future (21). In this case, “low dose” may be acceptable, and CT can play to its recent advantages in speed and clarity of details (22). Most recent works focus on the use of “ultra low dose” CT with a radiation dose close to a conventional X-ray, and will very quickly redefine the aforementioned case-by-case balance between the benefits and detriments of an imaging study.
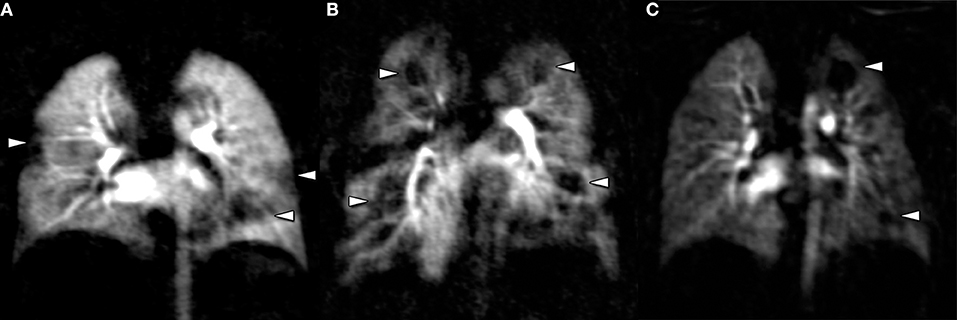
Figure 1. Perfusion MRI is a ready-to-use technique to study functional impairment in pediatric lung disease, based on gadolinium enhancement. Perfusion abnormalities can be found in conjunction with structural lung disease in cystic fibrosis (A), Langerhans cell histiocytosis (B), or bronchopulmonary dysplasia (C). Of note, perfusion abnormalities are non-specific and must be reviewed together with morphological imaging studies.
At this point, the authors Semple et al. (23) step in and elucidate on the recent advancements in radiation dose reduction of CT techniques, and developments in MRI and lung ultrasound to study BPD in infants and young children, and bring in their personal long-standing experience in CF lung imaging research. They provide a short introduction into the growing clinical relevance of BPD in pediatric medicine, and why imaging will play an important role for diagnosis and follow-up, similar to what has been discussed in CF. With regard to CT in BPD, the most relevant developments are summarized concisely, offering the technical specifications applicable in routine medicine. The frontiers of research on quantitative imaging and implications for standardization of CT imaging parameters similar to other diagnostic tests are only briefly touched upon.
MRI is presented as a research tool for BPD, and most studies cited and discussed in the present manuscript refer to studies performed in CF patients, whereas actual evidence for MRI in BPD is relatively low, mostly due to the limitations mentioned above in very young children and high technical effort to achieve structural detail in their very small lungs. BPD as a disease is even detrimental to the conditions for lung MRI, because of regional tissue and thus proton loss (“minus pathology”) (24). Apart from the aforementioned neonate-specific prototype MRI (25), Förster et al. have added further BPD-specific evidence for the use of clinical state-of-the-art MRI for studying the pathophysiology of BPD in neonates. The authors found a decrease in T1 and an increase in T2 relaxation times were associated with an increased risk for BPD, which can partially be explained by tissue loss and perfusion changes in the diseased lung (26), and which was found also in COPD and CF (17, 27). Such findings may support the use of quantitative MRI for risk stratification and monitoring in BPD.
One should not forget about the potential of ultra-sound for pediatric lung imaging in the hands of experienced and ambitious examiners, which is also its main drawback—high levels of training required, and limited inter-observer agreement together with very limited overview render it an ancillary technique, and Semple et al. emphasize that in the context of BPD and intensive care treatment, X-ray remains the mainstay of everyday routine imaging to monitor lines and tubes, and also parenchymal lung changes.
Author Contributions
The author confirms being the sole contributor of this work and has approved it for publication.
Conflict of Interest
The author declares that the research was conducted in the absence of any commercial or financial relationships that could be construed as a potential conflict of interest.
Publisher's Note
All claims expressed in this article are solely those of the authors and do not necessarily represent those of their affiliated organizations, or those of the publisher, the editors and the reviewers. Any product that may be evaluated in this article, or claim that may be made by its manufacturer, is not guaranteed or endorsed by the publisher.
References
1. Donadieu J, Roudier C, Saguintaah M, Maccia C, Chiron R. Estimation of the radiation dose from thoracic CT scans in a cystic fibrosis population. Chest. (2007) 132:1233–8. doi: 10.1378/chest.07-0221
2. Bell SC, Mall MA, Gutierrez H, Macek M, Madge S, Davies JC, et al. The future of cystic fibrosis care: a global perspective. Lancet Respir Med. (2020) 8:65–124. doi: 10.1016/S2213-2600(19)30337-6
3. Eichinger M, Optazaite D-E, Kopp-Schneider A, Hintze C, Biederer J, Niemann A, et al. Morphologic and functional scoring of cystic fibrosis lung disease using MRI. Eur J Radiol. (2012) 81:1321–9. doi: 10.1016/j.ejrad.2011.02.045
4. Puderbach M, Eichinger M, Gahr J, Ley S, Tuengerthal S, Schmahl A, et al. Proton MRI appearance of cystic fibrosis: comparison to CT. Eur Radiol. (2007) 17:716–24. doi: 10.1007/s00330-006-0373-4
5. Puderbach M, Eichinger M, Haeselbarth J, Ley S, Kopp-Schneider A, Tuengerthal S, et al. Assessment of morphological MRI for pulmonary changes in cystic fibrosis (CF) patients: comparison to thin-section CT and chest X-ray. Invest Radiol. (2007) 42:715–24. doi: 10.1097/RLI.0b013e318074fd81
6. Wielpütz MO, Puderbach M, Kopp-Schneider A, Stahl M, Fritzsching E, Sommerburg O, et al. Magnetic resonance imaging detects changes in structure and perfusion, and response to therapy in early cystic fibrosis lung disease. Am J Respir Crit Care Med. (2014) 189:956–65. doi: 10.1164/rccm.201309-1659OC
7. Stahl M, Wielpütz MO, Graeber SY, Joachim C, Sommerburg O, Kauczor HU, et al. Comparison of lung clearance index and magnetic resonance imaging for assessment of lung disease in children with cystic fibrosis. Am J Respir Crit Care Med. (2017) 195:349–59. doi: 10.1164/rccm.201604-0893OC
8. Wielpütz MO, Eichinger M, Wege S, Eberhardt R, Mall MA, Kauczor HU, et al. Mid-term reproducibility of chest MRI in adults with clinically stable cystic fibrosis and chronic obstructive pulmonary disease. Am J Respir Crit Care Med. (2019) 200:103–7. doi: 10.1164/rccm.201812-2356LE
9. Stahl M, Wielpütz MO, Ricklefs I, Dopfer C, Barth S, Schlegtendal A, et al. Preventive inhalation of hypertonic saline in infants with cystic fibrosis (PRESIS). A randomized, double-blind, controlled study. Am J Respir Crit Care Med. (2019) 199:1238–48. doi: 10.1164/rccm.201807-1203OC
10. Graeber SY, Boutin S, Wielpütz MO, Joachim C, Frey DL, Wege S, et al. Effects of lumacaftor-ivacaftor on lung clearance index, magnetic resonance imaging and airway microbiome in Phe508del homozygous patients with cystic fibrosis. Ann Am Thorac Soc. (2021) 18:971–80. doi: 10.1513/AnnalsATS.202008-1054OC
11. Leutz-Schmidt P, Eichinger M, Sommerburg O, Stahl M, Triphan SMF, Gehlen S, et al. [Magnetic resonance imaging of the lungs in cystic fibrosis]. Radiologe. (2020) 60:813–22. doi: 10.1007/s00117-020-00723-0
12. Woods JC, Wild JM, Wielpütz MO, Clancy JP, Hatabu H, Kauczor HU, et al. Current state of the art MRI for the longitudinal assessment of cystic fibrosis. J Magn Reson Imaging. (2020) 52:130–20. doi: 10.1002/jmri.27030
13. Altes TA, Johnson M, Fidler M, Botfield M, Tustison NJ, Leiva-Salinas C, et al. Use of hyperpolarized helium-3 MRI to assess response to ivacaftor treatment in patients with cystic fibrosis. J Cyst Fibros. (2017) 16:267–74. doi: 10.1016/j.jcf.2016.12.004
14. Smith L, Marshall H, Aldag I, Horn F, Collier G, Hughes D, et al. Longitudinal assessment of children with mild cystic fibrosis using hyperpolarized gas lung magnetic resonance imaging and lung clearance index. Am J Respir Crit Care Med. (2018) 197:397–400. doi: 10.1164/rccm.201705-0894LE
15. Nyilas S, Bauman G, Pusterla O, Ramsey K, Singer F, Stranzinger E, et al. Ventilation and perfusion assessed by functional MRI in children with CF: reproducibility in comparison to lung function. J Cyst Fibros. (2018) 18:543–50. doi: 10.1016/j.jcf.2018.10.003
16. Rayment JH, Couch MJ, McDonald N, Kanhere N, Manson D, Santyr G, et al. Hyperpolarised(129) Xe MRI to monitor treatment response in children with cystic fibrosis. Eur Respir J. (2019) 53:1802188. doi: 10.1183/13993003.02188-2018
17. Triphan SMF, Stahl M, Jobst BJ, Sommerburg O, Kauczor HU, Schenk JP, et al. Echo time-dependence of observed lung T(1) in patients with cystic fibrosis and correlation with clinical metrics. J Magn Reson Imaging. (2020) 52:1645–54. doi: 10.1002/jmri.27271
18. Wielpütz MO. Making contrast material obsolete: functional lung imaging with MRI. Radiology. (2020) 296:200–1. doi: 10.1148/radiol.2020200964
19. Wielpütz MO, Mall MA. MRI accelerating progress in functional assessment of cystic fibrosis lung disease. J Cyst Fibros. (2017) 16:165–7. doi: 10.1016/j.jcf.2016.12.010
20. Hatabu H, Ohno Y, Gefter WB, Parraga G, Madore B, Lee KS, et al. Expanding applications of pulmonary MRI in the clinical evaluation of lung disorders: fleischner society position paper. Radiology. (2020) 297:286–301. doi: 10.1148/radiol.2020201138
21. Hahn AD, Higano NS, Walkup LL, Thomen RP, Cao X, Merhar SL, et al. Pulmonary MRI of neonates in the intensive care unit using 3D ultrashort echo time and a small footprint MRI system. J Magn Reson Imaging. (2017) 45:463–71. doi: 10.1002/jmri.25394
22. Kuo W. Kemner-van de Corput MP, Perez-Rovira A, de Bruijne M, Fajac I, Tiddens HA, et al. Multicentre chest computed tomography standardisation in children and adolescents with cystic fibrosis: the way forward. Eur Respir J. (2016) 47:1706–17. doi: 10.1183/13993003.01601-2015
23. Semple T, Akhtar MR, Owens CM. Imaging bronchopulmonary dysplasia-a multimodality update. Front Med (Lausanne). (2017) 4:88. doi: 10.3389/fmed.2017.00088
24. Wielpütz M, Kauczor H-U. MRI of the lung: state of the art. Diagn Interv Radiol. (2012) 18:344–53. doi: 10.4261/1305-3825.DIR.5365-11.0
25. Walkup LL, Tkach JA, Higano NS, Thomen RP, Fain SB, Merhar SL, et al. Quantitative magnetic resonance imaging of bronchopulmonary dysplasia in the neonatal intensive care unit environment. Am J Respir Crit Care Med. (2015) 192:1215–22. doi: 10.1164/rccm.201503-0552OC
26. Förster K, Ertl-Wagner B, Ehrhardt H, Busen H, Sass S, Pomschar A, et al. Altered relaxation times in MRI indicate bronchopulmonary dysplasia. Thorax. (2020) 75:184–7. doi: 10.1136/thoraxjnl-2018-212384
Keywords: magnetic resonance imaging, lung imaging, dysplasia, computed tomography, cystic fibrosis—CF, langerhans cell histiocytosis (LCH)
Citation: Wielpütz MO (2021) Commentary: Expert Opinion to “Imaging Bronchopulmonary Dysplasia—A Multimodality Update”. Front. Med. 8:737724. doi: 10.3389/fmed.2021.737724
Received: 07 July 2021; Accepted: 23 September 2021;
Published: 21 October 2021.
Edited by:
Anne Hilgendorff, Ludwig Maximilian University of Munich, GermanyReviewed by:
Jim Wild, The University of Sheffield, United KingdomCopyright © 2021 Wielpütz. This is an open-access article distributed under the terms of the Creative Commons Attribution License (CC BY). The use, distribution or reproduction in other forums is permitted, provided the original author(s) and the copyright owner(s) are credited and that the original publication in this journal is cited, in accordance with accepted academic practice. No use, distribution or reproduction is permitted which does not comply with these terms.
*Correspondence: Mark O. Wielpütz, bWFyay53aWVscHVldHpAbWVkLnVuaS1oZWlkZWxiZXJnLmRl