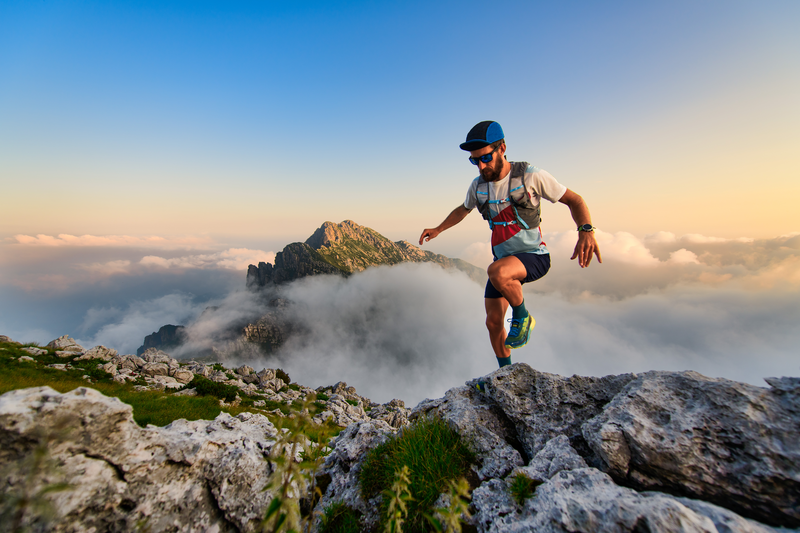
94% of researchers rate our articles as excellent or good
Learn more about the work of our research integrity team to safeguard the quality of each article we publish.
Find out more
REVIEW article
Front. Med. , 25 January 2022
Sec. Precision Medicine
Volume 8 - 2021 | https://doi.org/10.3389/fmed.2021.734643
This article is part of the Research Topic Insights in Precision Medicine: 2021 View all 10 articles
Long non-coding RNAs (lncRNAs) exceed 200 nucleotides in length are considered to be involved in both developmental processes and various diseases. Here, we focus on lncRNA MALAT1 (metastasis-associated lung adenocarcinoma transcript 1), which was one of the most important lncRNAs in proliferation, apoptosis, and migration. MALAT1 plays a regulatory role in liver diseases, including hepatic fibrosis, liver regeneration, liver cancer, and fatty liver diseases. In the current review, we summarize the latest literature about the function roles of MALAT1 in liver disorders. Probing the regulatory mechanism and cross talk of MALAT1 with other signaling pathways of pathological processes would improve the prognosis, diagnosis of liver diseases, and offer a promising candidate target for therapeutic interventions.
Seventy-five percent of the human genome generates transcripts, yet only about two percent of the genome encodes proteins. The majority of transcripts, including microRNAs (miRNAs) and long non-coding RNAs (lncRNAs), do not encode proteins (1). Small non-coding RNAs of ~22 nucleotides regulate the translation and stability of mRNA at the post-transcriptional level. Several biological processes are regulated by lncRNAs (more than 200 nucleotides), including carcinogenesis, development, and differentiation (2, 3). lncRNAs modulate various cellular processes, including nuclear organization and transcriptional and post-transcriptional modulation of gene expression (4, 5). lncRNAs serve as competitive endogenous RNAs (ceRNAs) in a regulatory network by “sponging” target miRNAs to regulate mRNA expression. This regulatory network is implicated in cancer development, apoptosis, and drug resistance (6–8).
To date, over 50,000 human lncRNAs have been identified (9). One of the most extensively studied of lncRNAs is Metastasis-associated lung adenocarcinoma transcript 1 (MALAT1, ENSG00000251562), located in human chromosome 11q13.1 (mouse chromosome 19qA); MALAT1 is also known as nuclear-enriched abundant transcript first described to be associated with non-small cell lung cancer (10–12). Elevated MALAT1 expression is implicated in hyperproliferation, cellular and molecular functions, metastasis, and poor prognosis (13, 14). MALAT1 is overexpressed in a variety of human diseases in the form of a ceRNA network, which is important for gene expression, proliferation, and metastasis (15). The pathological processes would be activated once the level of MALAT1 expression changes to abnormal condition due to various endogenous or exogenous inducers. Here, we summarize the roles of MALAT1 in liver diseases including hepatic fibrosis, hepatic carcinoma, liver regeneration, and fatty liver diseases.
MALAT1 is preferentially associated with transcriptionally active genes in the nucleus (16). MALAT1 plays a very critical cellular function for normal physiology. Given the nuclear localization of nuclear speckles, MALAT1 could be implicated in the regulation of alternative splicing in terms of binding splicing factors (17). The interaction between MALAT1 and the splicing factors of serine/arginine-rich (SR) proteins regulates selective splicing by modulating phosphorylation and distribution in the nuclear macular region. MALAT1 also regulates SR proteins by affecting the localization and activities of shear factor kinases such as serine/arginine protein kinases 1 (SRPK1) (18, 19). As a result of MALAT1 transcripts located in the nucleus, MALAT1 combined with release of pre-mRNA splicing factors via antisense oligonucleotides triggers could induce overexpression of the related factors. Therefore, MALAT1 might serve as an “anchor point” for localization of certain genes near nuclear speckles and induce transcriptional activation to affect the processing of their RNAs, implicating MALAT1 in transcriptional regulation (20). The major mechanisms of post-transcriptional regulation of MALAT1 include alternative splicing, protein activities, and competitive ceRNAs (15). MALAT1 is also expressed in vascular endothelial cells and plays important roles in regulating vascular growth. The cell cycle inhibitory proteins were also significantly increased accompanied by the depletion of MALAT1 (21).
MicroRNAs bind to the 3'UTR of their target genes and negatively regulate gene expression through depressing translation or promoting mRNA attenuation. This strategy was proposed initially by Poliseno to explain how mRNAs communicate with lncRNAs through miRNA response elements (MREs) as “language” (22). In the process of RNA-miRNA regulation, MREs act as binding sites for ceRNAs, which positively adjust miRNAs availability to bind their target mRNAs (23). MALAT1 also followed the ceRNA regulatory system through the negatively regulation between MALAT1 and micRNAs (Figure 1).
Figure 1. Molecular Function of MALAT1. (A) The transcriptional regulation of MALAT1 by interaction between MALAT1 and SR splicing factors. (B) The post-transcriptional regulation of MALAT1 via competitive ceRNAs.
MALAT1 binds to chromatin of activated transcribing genes, transcription factors and transcriptional co-activators and regulates their expression at the transcriptional level to promote cell proliferation and inhibit cell apoptosis. MALAT1 was also triggered to upregulate transcriptional activators of proteosome genes (24).
Hepatic fibrosis is characterized by chronic abnormal hyperplasia and accumulation of large amounts of extracellular matrix (ECM) [including smooth muscle actin (SMA) and type I collagen], and the release of proinflammatory and profibrotic factors. Fibrosis can result from a variety of chronic liver diseases such as viral hepatitis, alcoholism, drug abuse, metabolic syndrome, genetic metabolic diseases, and autoimmune hepatitis (25). The regulatory mechanism of liver fibrosis is not entirely clear. The activation of hepatic stellate cells (HSCs), the resident perisinusoidal cell type, is critical in the development of liver fibrosis (26). Activated HSCs are considered proliferative cells that secrete profibrogenic mediators and express ECM; they may also be involved in fibrosis progression (27). Yu et al. (28) found that MALAT1 was significantly upregulated in activated HSCs and negatively correlated with the expression of miR-101b in in the mouse liver fibrosis model induced by carbon tetrachloride (CCl4). MALAT1 and ras-related C3 botulinum toxin substrate 1 (Rac1) are targets of miR-101b, and the former acts as a ceRNA to enhance the expression of Rac1, thus promoting HSC proliferation and activation. Dai et al. (29) found that MALAT1 extracted from arsenite-treated human hepatocytes promoted the activation of LX-2 HSCs (an immortalized HSC cell lines) by binding miRNA-26b. Wu et al. (30) reported that MALAT1 influenced the progression of hepatic fibrosis by repressing the expression and function of silent information regulator 1 (SIRT1), a nicotinamide adenine nicotinamide (NAD+)-dependent III class of histone deacetylases in the Sirtuin family (16). SIRT1 suppressed the ability of the promoters of fibrogenic genes, such as collagen type I, to bind Smad3, a downstream mediator of the TGF-β signaling pathway (31, 32). In short, MALAT1 promotes HSC activation by blocking SIRT1-mediated inhibition of the TGF-β signaling pathway in hepatic fibrosis.
The normal liver has a strong regenerative ability and can maintain the original liver volume by inducing mitosis of its own cells, or tissue repair through rapid division and proliferation from endogenous liver stem cells (such as liver oval cells) to mature liver cells (33). Because of the limited regenerative ability of endogenous liver stem cells, exogenous measures are needed to promote liver regeneration and maintain the liver function of patients with severe liver injury or liver failure (34). Liver cells begin proliferate and divide in the initial period. The proliferation of liver is not only completed by parenchyma cells, but by a variety of cells which also participate in the coordination and completion at the stage of proliferation (35). The timely and automatic termination of hepatocyte proliferation is distinct from the unlimited proliferation of liver tumors in the termination stage, which are are important for the liver regulation of growth, development, and differentiation (36). The interaction and regulatory factors were activated rested during the biological processes of liver development, liver regeneration and hepatic carcinoma. Tripathi et al. (37) showed that MALAT1 promotes the proliferation of normal cells. MALAT1 also promotes the expression of cell cycle genes (such as the transcription factor B-myb) and progression from G1 to S phase, thus inducing mitosis. Knockout of MALAT1 activated p53 and its reporter gene, a downstream gene of MALAT1. Maxy et al. (38, 39) studied the expression of MALAT1 in various tissues and organs of mice and found that MALAT1 expression in mice was highest in the liver followed by the kidney, lowest in skeletal muscle. MALAT1 expression was elevated, and its inhibition or knockout may promote the proliferation, migration, and tubulation, and suppress apoptosis, of endothelial cells by activating the PI3K/Akt signaling pathway (40).
Liver regeneration is initiated immediately after liver resection, and upregulation of hepatocyte growth factor (HGF) induces the expression of MALAT1, damaging the stability of the p-catenin degradation complex (41). The resulting increased total p-catenin activates the Wnt/β-catenin signaling pathway and upregulates cyclinD1, promoting progression from G1 to S phase and shortening the cell cycle, thereby accelerating liver cell proliferation and liver regeneration (42). Deficiency of MALAT1 inhibits VEGFR2 expression, reduces angiogenesis, perfusion, and functional recovery of ischemic hind limbs in mice, and inhibits blood flow recovery and capillary density in gastrocnemeal muscle tissue after ischemia, suggesting that MALAT1 affects angiogenesis via multiple mechanisms (21). MALAT1 regulates angiogenesis and immune responses (43). MiR-3064-5p inhibits the FOXA1/CD24/Src pathway to exert an antiangiogenic effect, whereas MALAT1 adsorbs miR-3064-5p, thereby alleviating the inhibition of FOXA1 and promoting hepatic hemangiogenesis (44). Hou et al. (45, 46) confirmed that MALAT1 is an important upstream target of miR-140, which inhibits VEGF-A expression and M2 macrophage polarization, thereby inhibiting angiogenesis and immunosuppression.
The expression of MALAT1 is significantly increased in HCC tissues and cell lines, which promotes HCC proliferation and metastasis and inhibits HCC cell apoptosis by acting as an oncogene (47–49). Silencing of MALAT1 reduces the proliferation, invasion, and migration of cancer cells, and induces their apoptosis (50). The upstream of MALAT1 contains 5 specific protein 1/3(SP1/3) binding sites, and the combined regulation of SP1 and SP3 in cancer cells promote the expression of MALAT1. Yes-related protein (YAP) upregulates the expression of MALAT1 at the transcriptional and post-transcriptional levels, whereas serine/arginine splicing factor 1 (SRSF1) rich in serine/arginine had the opposite effect. Overexpression of YAP reduces SRSF1 nuclear retention (51).
MALAT1 plays a role in HCC cell proliferation and apoptosis by multiple pathways. Upregulation of MALAT1 promotes cancer cell proliferation, and its downregulation promotes cancer cell death and autophagy. Malakar et al. (52) found that high expression of MALAT1 in HCC cells upregulates oncogenic shear factor SRSF1 and activates the mTOR pathway, thereby promoting the proliferation and survival of HCC cells. Peng et al. (50) found that MALAT1 expression regulates the proliferation, apoptosis, and autophagy of HCC cells by adsorption of miR-146a, whereas downregulation of miR-146a upregulates PI3K, modulating the phosphorylation of downstream Akt and mTOR. Therefore, apoptosis and autophagy of HCC cells can be inhibited by targeting the PI3K/Akt/mTOR signaling axis. Liu et al. (53) showed that MALAT1 acted as a molecular sponge to absorb miR-195, which inhibited its downstream target EGFR, inducing the activation of PI3K/Akt and JAK/STAT pathways by overexpression of EGFR, thus promote the growth activity of HCC cells. Chen et al. (54) reported that MALAT1 regulates the expression of zinc finger E-box binding homeobox (ZEB1) by sponging miR-143-3p. In conclusion, the up- or downregulation of MALAT1 is related to the proliferation and apoptosis of HCC cells. MALAT1 acts as a molecular sponge to regulate miRNA signaling pathways affecting downstream factors and promotes HCC cell proliferation and inhibits their apoptosis.
HCC invasion and metastasis are aggravated via signaling pathways and MALAT1. YAP1 in vascular endothelial cells reduces vascular proliferation (55). Exosomes containing MALAT1 are released into the tumor microenvironment, inhibiting and depleting YAP1, activating ERK1/2 signal transduction, and enhancing the expression of MMP2 and MMP9, thereby promoting tumor invasion and metastasis. The tumor transcription factor FOXM1 is the target of miR-125a-3p, targeting of which by MALAT1 upregulates FOXM1 expression and promotes HCC invasion and migration (51, 56). SNAI1, a key transcription factor in the epithelial–mesenchymal transition, is also a direct target of miR-22, which could be absorbed by MALAT1, promoting the enrichment of enhancer of zeste homolog 2 (EZH2) to inhibit miR-22 transcription, thereby upregulating SNAI1 expression and facilitating HCC invasion and distant metastasis. Li et al. (57) reported that MALAT1 acted as a molecular sponge for miR-146b-5p, inhibiting HCC growth and metastasis by targeting Akt phosphorylation mediated by TNF receptor-related factor 6. Hou et al. (58) found that MALAT1 reduced the inhibition of SIRT1 by miR-204 by competitively binding miR-204. Chen et al. (54) showed that MALAT1 regulates ZEB1 expression by sponging miR-143-3p, promoting HCC invasion and metastasis.
MALAT1 also promotes glycolysis in HCC. Aerobic glycolysis (Warburg effect) is a marker of tumor cells' ability to escape apoptosis to promote proliferation and migration. It could provide material basis for tumor cells and create an acidic microenvironment (59, 60). Malakar et al. (61) found that MALAT1 enhances the translation of metabolic transcription factor TCF7L2 by upregulating shear factor SRSF1 and activating the mTORC1-4EBP1 axis to upregulate glycolysis genes and inhibit gluconeogenesis, promoting the development of HCC (62). Additionally, it has been shown that MALAT1 enhances glycolysis in liver, and inhibits gluconeogenesis, via elevated translation of the transcription factor TCF7L2 and as such also plays a crucial role in metabolic stress (63). MALAT1 promotes the progression of inflammation-associated HCC. Huang et al. (64) reported that MALAT1 induces the secretion of inflammatory cytokines, promoting the progression of inflammation-related HCC by mobilizing chromatin and remodeling subunit brahma-related gene 1 (BRG1) to the promoter region of the inflammatory cytokines IL-6 and C-X-C motif chemokine ligand 8 (CXCL8).
Cancer stem cells (CSCs) are characterized by self-renewal and differentiation and are considered the seeds of tumor genesis, development, and metastasis. MALAT1 promotes HCC stem cell properties. The higher the proportion of CSCs, the more aggressive the tumor (65). He et al. (4) reported that HBx protein induced CSC production in HCC via the PI3K/Akt signaling pathway (66). MALAT1 emerged as the function of competing endogenous RNA, preventing miR-124-mediated inhibition of PI3K/Akt signaling. This induced CSC characteristics and ultimately promoted HBV-associated HCC. Chen et al. (67) reported that circ-MALAT1 generated by reverse shear of lncRNA MALAT1 acts as a “brake” in the ribosome, and forms a complex with the ribosome and mRNA, preventing the transcription factor PAX5 mRNA translation and promoting CSC self-renewal. However, circ-MALAT1 also acts as a sponge for miR-6887-3p to enhance the phosphorylation of JAK2, activating the JAK2/STAT3 signaling pathway and promoting CSC self-renewal (68). Thus, MALAT1 represents both a promising cancer bio-marker as well as a potential therapeutic target for limiting metastatic growth.
MALAT1 significantly inhibits palmitic acid-induced lipid accumulation and increases expression of SREBP-1c, an important regulator of cholesterol and fatty acid synthesis in abnormal lipid metabolism and fatty liver disease (69, 70). MALAT1 abundance in liver tissue is closely related to the pathological changes in non-alcoholic fatty liver disease (NAFLD), a clinicopathologic syndrome characterized by diffuse bullae steatosis (abnormal accumulation of lipids in liver tissues) not caused by alcohol or other hepatotoxic factors. MALAT1 may mediate chemokines by regulating C-X-C motif chemokine ligand 5 (CXCL5) in hepatic stellate cells in the occurrence of non-alcoholic steatohepatitis (NASH) and fibrosis in patients with NAFLD (71, 72). MALAT1 abundance increases significantly in NASH with hepatocyte ballooning degeneration and lobular inflammation, and in hepatocyte dysfunction with elevated alanine aminotransferase, aspartate aminotransferase, and alkaline phosphatase. The schematic representation of MALAT1 in liver diseases was Figure 2. A form was also presented to explain different roles of MALAT1 under different liver diseases and how MALAT1 influences pathophysiology in Table 1.
LncRNA is a hot research topic in the field of liver disease in recent years. As a member of the lncRNA family, MALAT1 is a multi-functional lncRNA and an important regulator in hepatic fibrosis, liver regeneration, cancer, and fatty liver diseases. The molecular mechanisms mediated by one lncRNA would be complicated. Compared to most lncRNAs, MALAT1 is expressed at relatively high level in almost all human tissues in a variety of regulating pathways, thus makes it intricate to be targeted by simply silencing or overexpressing in pathological conditions. Further challenge to therapeutically measures would include small molecules specifically designed to intervene the gene-protein interaction for MALAT1 functions (73). A deeper understanding of the functions of MALAT1 and its interaction network will lay the foundation for the development of lncRNAs as therapeutic targets and as diagnostic or prognostic biomarkers for liver diseases.
JLu and KX structured the text and content. JLu and JG wrote and edited the manuscript. JLi generated the figures. XM reviewed the literature. All authors contributed to the article and approved the submitted version.
This research was funded by the Independent Project Fund of the State Key Laboratory for Diagnosis and Treatment of Infectious Diseases, the National Key Research and Development Program of China (No. 2016YFC1101404/3), and Zhejiang Basic Public Welfare Research Program of China (No. LQ20H030012).
The authors declare that the research was conducted in the absence of any commercial or financial relationships that could be construed as a potential conflict of interest.
All claims expressed in this article are solely those of the authors and do not necessarily represent those of their affiliated organizations, or those of the publisher, the editors and the reviewers. Any product that may be evaluated in this article, or claim that may be made by its manufacturer, is not guaranteed or endorsed by the publisher.
1. Clamp M, Fry B, Kamal M, Xie X, Cuff J, Lin MF, et al. Distinguishing protein-coding, and noncoding genes in the human genome. Proc Natl Acad Sci USA. (2007) 104:19428–33. doi: 10.1073/pnas.0709013104
2. Esteller M. Non-coding RNAs in human disease. Nat Rev Genet. (2011) 12:861–74. doi: 10.1038/nrg3074
3. Quinn JJ, Chang HY. Unique features of long non-coding RNA biogenesis and function. Nat Rev Genet. (2016) 17:47–62. doi: 10.1038/nrg.2015.10
4. Mercer TR, Dinger ME, Mattick JS. Long non-coding RNAs: insights into functions. Nat Rev Genet. (2009) 10:155–9. doi: 10.1038/nrg2521
5. Malissovas N, Ninou E, Michail A, Politis PK. Targeting long non-coding RNAs in nervous system cancers: new insights in prognosis, diagnosis and therapy. Curr Med Chem. (2019) 26:5649–63. doi: 10.2174/0929867325666180831170227
6. Lin Z, Li X, Zhan X, Sun L, Gao J, Cao Y, et al. Construction of competitive endogenous RNA network reveals regulatory role of long non-coding RNAs in type 2 diabetes mellitus. J Cell Mol Med. (2017) 21:3204–13. doi: 10.1111/jcmm.13224
7. Shi X, Sun M, Liu H, Yao Y, Song Y. Long non-coding RNAs: a new frontier in the study of human diseases. Cancer Lett. (2013) 339:159–66. doi: 10.1016/j.canlet.2013.06.013
8. Kim TK, Hemberg M, Gray JM, Costa AM, Bear DM, Wu J, et al. Widespread transcription at neuronal activity-regulated enhancers. Nature. (2010) 465:182–7. doi: 10.1038/nature09033
9. Iyer MK, Niknafs YS, Malik R, Singhal U, Sahu A, Hosono Y, et al. The landscape of long noncoding RNAs in the human transcriptome. Nat Genet. (2015) 47:199–208. doi: 10.1038/ng.3192
10. Ji P, Diederichs S, Wang W, Boing S, Metzger R, Schneider PM, et al. MALAT-1, a novel noncoding RNA, and thymosin beta4 predict metastasis and survival in early-stage non-small cell lung cancer. Oncogene. (2003) 22:8031–41. doi: 10.1038/sj.onc.1206928
11. Tani H, Nakamura Y, Ijiri K, Akimitsu N. Stability of MALAT-1, a nuclear long non-coding RNA in mammalian cells, varies in various cancer cells. Drug Discov Ther. (2010) 4:235–9.
12. Ren D, Li H, Li R, Sun J, Guo P, Han H, et al. Novel insight into MALAT-1 in cancer: therapeutic targets and clinical applications. Oncol Lett. (2016) 11:1621–30. doi: 10.3892/ol.2016.4138
13. Liang T, Xu F, Wan P, Zhang L, Huang S, Yang N, et al. Malat-1 expression in bladder carcinoma tissues and its clinical significance. Am J Transl Res. (2021) 13:3555–60.
14. Fu S, Wang Y, Li H, Chen L, Liu Q. Regulatory networks of LncRNA MALAT-1 in cancer. Cancer Manag Res. (2020) 12:10181–98. doi: 10.2147/CMAR.S276022
15. Kong X, Wang J, Cao Y, Zhang H, Lu X, Wang Y, et al. The long noncoding RNA MALAT-1 functions as a competing endogenous RNA to regulate MSL2 expression by sponging miR-338-3p in myasthenia gravis. J Cell Biochem. (2019) 120:5542–50. doi: 10.1002/jcb.27838
16. West JA, Davis CP, Sunwoo H, Simon MD, Sadreyev RI, Wang PI, et al. The long noncoding RNAs NEAT1 and MALAT1 bind active chromatin sites. Mol Cell. (2014) 55:791–802. doi: 10.1016/j.molcel.2014.07.012
17. Sridhar B, Rivas-Astroza M, Nguyen TC, Chen W, Yan Z, Cao X, et al. Systematic mapping of RNA-chromatin interactions In Vivo. Curr Biol. (2017) 27:610–2. doi: 10.1016/j.cub.2017.01.068
18. Romero-Barrios N, Legascue MF, Benhamed M, Ariel F, Crespi M. Splicing regulation by long noncoding RNAs. Nucleic Acids Res. (2018) 46:2169–84. doi: 10.1093/nar/gky095
19. Luco RF. Retrotransposons jump into alternative-splicing regulation via a long noncoding RNA. Nat Struct Mol Biol. (2016) 23:952–4. doi: 10.1038/nsmb.3318
20. Gutschner T, Hammerle M, Eissmann M, Hsu J, Kim Y, Hung G, et al. The noncoding RNA MALAT1 is a critical regulator of the metastasis phenotype of lung cancer cells. Cancer Res. (2013) 73:1180–9. doi: 10.1158/0008-5472.CAN-12-2850
21. Michalik KM, You X, Manavski Y, Doddaballapur A, Zörnig M, Braun T, et al. Long noncoding RNA MALAT1 regulates endothelial cell function and vessel growth. Circ Res. (2014) 114:1389–97. doi: 10.1161/CIRCRESAHA.114.303265
22. Salmena L, Poliseno L, Tay Y, Kats L, Pandolfi PP. A ceRNA hypothesis: the rosetta stone of a hidden RNA language? Cell. (2011) 146:353–8. doi: 10.1016/j.cell.2011.07.014
23. Poliseno L, Salmena L, Zhang J, Carver B, Haveman WJ, Pandolfi PP. A coding-independent function of gene and pseudogene mRNAs regulates tumour biology. Nature. (2010) 465:1033–8. doi: 10.1038/nature09144
24. Amodio N, Stamato MA, Juli G, Morelli E, Fulciniti M, Manzoni M, et al. Drugging the lncRNA MALAT1 via LNA gapmeR ASO inhibits gene expression of proteasome subunits and triggers anti-multiple myeloma activity. Leukemia. (2018) 32:1948–57. doi: 10.1038/s41375-018-0067-3
25. Teng KY, Ghoshal K. Role of noncoding RNAs as biomarker and therapeutic targets for liver fibrosis. Gene Expr. (2015) 16:155–62. doi: 10.3727/105221615X14399878166078
26. Zhang H, Sun D, Wang G, Cui S, Field RA, Li J, et al. Alogliptin alleviates liver fibrosis via suppression of activated hepatic stellate cell. Biochem Biophys Res Commun. (2019) 511:387–93. doi: 10.1016/j.bbrc.2019.02.065
27. Schon HT, Bartneck M, Borkham-Kamphorst E, Nattermann J, Lammers T, Tacke F, et al. Pharmacological intervention in hepatic stellate cell activation and hepatic fibrosis. Front Pharmacol. (2016) 7:33. doi: 10.3389/fphar.2016.00033
28. Yu F, Lu Z, Cai J, Huang K, Chen B, Li G, et al. MALAT1 functions as a competing endogenous RNA to mediate Rac1 expression by sequestering miR-101b in liver fibrosis. Cell Cycle. (2015) 14:3885–96. doi: 10.1080/15384101.2015.1120917
29. Dai X, Chen C, Xue J, Xiao T, Mostofa G, Wang D, et al. Exosomal MALAT1 derived from hepatic cells is involved in the activation of hepatic stellate cells via miRNA-26b in fibrosis induced by arsenite. Toxicol Lett. (2019) 316:73–84. doi: 10.1016/j.toxlet.2019.09.008
30. Wu Y, Liu X, Zhou Q, Huang C, Meng X, Xu F, et al. Silent information regulator 1 (SIRT1) ameliorates liver fibrosis via promoting activated stellate cell apoptosis and reversion. Toxicol Appl Pharmacol. (2015) 289:163–76. doi: 10.1016/j.taap.2015.09.028
31. Sun L, Fan Z, Chen J, Tian W, Li M, Xu H, et al. Corrigendum: transcriptional repression of SIRT1 by protein inhibitor of activated STAT 4 (PIAS4) in hepatic stellate cells contributes to liver fibrosis. Sci Rep. (2016) 6:30513. doi: 10.1038/srep30513
32. Wei J, Ghosh AK, Chu H, Fang F, Hinchcliff ME, Wang J, et al. The histone deacetylase sirtuin 1 is reduced in systemic sclerosis and abrogates fibrotic responses by targeting transforming growth factor beta signaling. Arthritis Rheumatol. (2015) 67:1323–34. doi: 10.1002/art.39061
33. Bangru S, Kalsotra A. Cellular and molecular basis of liver regeneration. Semin Cell Dev Biol. (2020) 100:74–87. doi: 10.1016/j.semcdb.2019.12.004
34. Ray K. Therapy: targeting liver tissue repair and regeneration. Nat Rev Gastroenterol Hepatol. (2016) 13:559. doi: 10.1038/nrgastro.2016.146
35. Michalopoulos GK, Bhushan B. Liver regeneration: biological and pathological mechanisms and implications. Nat Rev Gastroenterol Hepatol. (2021) 18:40–55. doi: 10.1038/s41575-020-0342-4
36. Xu D, Yang F, Yuan JH, Zhang L, Bi HS, Zhou CC, et al. Long noncoding RNAs associated with liver regeneration 1 accelerates hepatocyte proliferation during liver regeneration by activating Wnt/beta-catenin signaling. Hepatology. (2013) 58:739–51. doi: 10.1002/hep.26361
37. Tripathi V, Ellis JD, Shen Z, Song DY, Pan Q, Watt AT, et al. The nuclear-retained noncoding RNA MALAT1 regulates alternative splicing by modulating SR splicing factor phosphorylation. Mol Cell. (2010) 3:925–38. doi: 10.1016/j.molcel.2010.08.011
38. Jeffers LK, Duan K, Ellies LG, Seaman WT, Burger-Calderon RA, Diatchenko LB, et al. Correlation of transcription of MALAT-1, a novel noncoding RNA, with deregulated expression of tumor suppressor p53 in small DNA tumor virus models. J Cancer Ther. (2013) 4:3. doi: 10.4236/jct.2013.43094
39. Pruszko M, Milano E, Forcato M, Donzelli S, Ganci F, Di Agostino S, et al. The mutant p53-ID4 complex controls VEGFA isoforms by recruiting lncRNA MALAT1. EMBO Rep. (2017) 18:1331–51. doi: 10.15252/embr.201643370
40. Zhang SH, Zhang SG, Zhou P, Wei X, Mao XD, Lin SG, et al. LncRNA MALAT1 affects high glucose-induced endothelial cell proliferation, apoptosis, migration and angiogenesis by regulating the PI3K/Akt signaling pathway. Eur Rev Med Pharmacol Sci. (2019) 23:8551–9. doi: 10.26355/eurrev_201910_19170
41. Tripathi V, Shen Z, Chakraborty A, Giri S, Freier SM, Wu X, et al. Long noncoding RNA MALAT1 controls cell cycle progression by regulating the expression of oncogenic transcription factor B-MYB. PLoS Genet. (2013) 9:e1003368. doi: 10.1371/journal.pgen.1003368
42. Yang F, Yi F, Han X, Du Q, Liang Z. MALAT-1 interacts with hnRNP C in cell cycle regulation. FEBS Lett. (2013) 587:3175–81. doi: 10.1016/j.febslet.2013.07.048
43. Ma Z, Zhang J, Xu X, Qu Y, Dong H, Dang J, et al. LncRNA expression profile during autophagy and Malat1 function in macrophages. PLoS One. (2019) 14:e0221104. doi: 10.1371/journal.pone.0221104
44. Zhang P, Ha M, Li L, Huang X, Liu C. MicroRNA-3064-5p sponged by MALAT1 suppresses angiogenesis in human hepatocellular carcinoma by targeting the FOXA1/CD24/Src pathway. FASEB J. (2020) 34:66–81. doi: 10.1096/fj.201901834R
45. Bao M, Liu G, Song J, Gao Y. Long non-coding RNA MALAT1 promotes odontogenic differentiation of human dental pulp stem cells by impairing microRNA-140-5p-dependent downregulation of GIT2. Cell Tissue Res. (2020) 38:487–98. doi: 10.1007/s00441-020-03246-1
46. Hou ZH, Xu XW, Fu XY, Zhou LD, Liu SP, Tan DM. Long non-coding RNA MALAT1 promotes angiogenesis and immunosuppressive properties of HCC cells by sponging miR-140. Am J Physiol Cell Physiol. (2020) 318:C649–63. doi: 10.1152/ajpcell.00510.2018
47. Toraih EA, Ellawindy A, Fala SY, Al Ageeli E, Gouda NS, Fawzy MS, et al. Oncogenic long noncoding RNA MALAT1 and HCV-related hepatocellular carcinoma. Biomed Pharmacother. (2018) 102:653–69. doi: 10.1016/j.biopha.2018.03.105
48. Goyal B, Yadav SRM, Awasthee N, Gupta S, Kunnumakkara AB, Gupta SC. Diagnostic, prognostic, and therapeutic significance of long non-coding RNA MALAT1 in cancer. Biochim Biophys Acta Rev Cancer. (2021) 1875:188502. doi: 10.1016/j.bbcan.2021.188502
49. Wang C, Zhang Q, Hu Y, Zhu J, Yang J. Emerging role of long non-coding RNA MALAT1 in predicting clinical outcomes of patients with digestive system malignancies: a meta-analysis. Oncol Lett. (2019) 17:2159–70. doi: 10.3892/ol.2018.9875
50. Peng N, He J, Li J, Huang H, Huang W, Liao Y, et al. Long noncoding RNA MALAT1 inhibits the apoptosis and autophagy of hepatocellular carcinoma cell by targeting the microRNA-146a/PI3K/Akt/mTOR axis. Cancer Cell Int. (2020) 20:165. doi: 10.1186/s12935-020-01231-w
51. Wang J, Wang H, Zhang Y, Zhen N, Zhang L, Qiao Y, et al. Mutual inhibition between YAP and SRSF1 maintains long non-coding RNA, Malat1-induced tumourigenesis in liver cancer. Cell Signal. (2014) 26:1048–59. doi: 10.1016/j.cellsig.2014.01.022
52. Malakar P, Shilo A, Mogilevsky A, Stein I, Pikarsky E, Nevo Y, et al. Long noncoding RNA MALAT1 promotes hepatocellular carcinoma development by SRSF1 upregulation and mTOR activation. Cancer Res. (2017) 77:1155–67. doi: 10.1158/0008-5472.CAN-16-1508
53. Liu D, Zhu Y, Pang J, Weng X, Feng X, Guo Y. Knockdown of long non-coding RNA MALAT1 inhibits growth and motility of human hepatoma cells via modulation of miR-195. J Cell Biochem. (2018) 119:1368–80. doi: 10.1002/jcb.26297
54. Chen L, Yao H, Wang K, Liu X. Long non-coding RNA MALAT1 regulates ZEB1 expression by sponging miR-143-3p and promotes hepatocellular carcinoma progression. J Cell Biochem. (2017) 118:4836–43. doi: 10.1002/jcb.26158
55. Li Y, Zhang X, Zheng Q, Zhang Y, Ma Y, Zhu C, et al. YAP1 inhibition in HUVECs Is associated with released exosomes and increased hepatocarcinoma invasion and metastasis. Mol Ther Nucleic Acids. (2020) 21:86–97. doi: 10.1016/j.omtn.2020.05.021
56. Zhao L, Lou G, Li A, Liu Y. lncRNA MALAT1 modulates cancer stem cell properties of liver cancer cells by regulating YAP1 expression via miR375 sponging. Mol Med Rep. (2020) 22:1449–57. doi: 10.3892/mmr.2020.11196
57. Li C, Miao R, Liu S, Wan Y, Zhang S, Deng Y, et al. Down-regulation of miR-146b-5p by long noncoding RNA MALAT1 in hepatocellular carcinoma promotes cancer growth and metastasis. Oncotarget. (2017) 8:28683–95. doi: 10.18632/oncotarget.15640
58. Hou Z, Xu X, Zhou L, Fu X, Tao S, Zhou J, et al. The long non-coding RNA MALAT1 promotes the migration and invasion of hepatocellular carcinoma by sponging miR-204 and releasing SIRT1. Tumour Biol. (2017) 39:1010428317718135. doi: 10.1177/1010428317718135
59. Das L, Vinayak M. Long term effect of curcumin in regulation of glycolytic pathway and angiogenesis via modulation of stress activated genes in prevention of cancer. PLoS ONE. (2014) 9:e99583. doi: 10.1371/journal.pone.0099583
60. Ma ZJ, Yan H, Wang YJ, Yang Y, Li XB, Shi AC, et al. Proteomics analysis demonstrating rosmarinic acid suppresses cell growth by blocking the glycolytic pathway in human HepG2 cells. Biomed Pharmacother. (2018) 105:334–49. doi: 10.1016/j.biopha.2018.05.129
61. Malakar P, Stein I, Saragovi A, Winkler R, Stern-Ginossar N, Berger M, et al. Long noncoding RNA MALAT1 regulates cancer glucose metabolism by enhancing mTOR-mediated translation of TCF7L2. Cancer Res. (2019) 79:2480–93. doi: 10.1158/0008-5472.CAN-18-1432
62. Chen W. Cancer statistics: updated cancer burden in China. Chin J Cancer Res. (2015) 27:1. doi: 10.3978/j.issn.1000-9604.2015.02.07
63. Sridhar B, Rivas-Astroza M, Nguyen TC, Chen W, Yan Z, Cao X, et al. Systematic mapping of RNA-chromatin interactions In Vivo. Curr Biol. (2017) 27-602–609. doi: 10.1016/j.cub.2017.01.011
64. Huang M, Wang H, Hu X, Cao X. lncRNA MALAT1 binds chromatin remodeling subunit BRG1 to epigenetically promote inflammation-related hepatocellular carcinoma progression. Oncoimmunology. (2019) 8:e1518628. doi: 10.1080/2162402X.2018.1518628
65. Han Y, Zhou L, Wu T, Huang Y, Cheng Z, Li X, et al. Downregulation of lncRNA-MALAT1 affects proliferation and the expression of stemness markers in glioma stem cell line SHG139S. Cell Mol Neurobiol. (2016) 36:1097–107. doi: 10.1007/s10571-015-0303-6
66. He B, Peng F, Li W, Jiang Y. Interaction of lncRNA-MALAT1 and miR-124 regulates HBx-induced cancer stem cell properties in HepG2 through PI3K/Akt signaling. J Cell Biochem. (2019) 120:2908–18. doi: 10.1002/jcb.26823
67. Chen L, Kong R, Wu C, Wang S, Liu Z, Liu S, et al. Circ-MALAT1 functions as both an mrna translation brake and a microRNA sponge to promote self-renewal of hepatocellular cancer stem cells. Adv Sci. (2020) 7:1900949. doi: 10.1002/advs.201900949
68. Chang HL, Bamodu OA, Ong JR, Lee WH, Yeh CT, Tsai JT. Targeting the epigenetic non-coding RNA MALAT1/Wnt signaling axis as a therapeutic approach to suppress stemness and metastasis in hepatocellular carcinoma. Cells. (2020) 9:1020. doi: 10.3390/cells9041020
69. Oliva J, Bardag-Gorce F, French BA, Li J, French SW. The regulation of non-coding RNA expression in the liver of mice fed DDC. Exp Mol Pathol. (2009) 87:12–9. doi: 10.1016/j.yexmp.2009.03.006
70. Yan C, Chen J, Chen N. Long noncoding RNA MALAT1 promotes hepatic steatosis and insulin resistance by increasing nuclear SREBP-1c protein stability. Sci Rep. (2016) 6:22640. doi: 10.1038/srep22640
71. Leti F, Legendre C, Still CD, Chu X, Petrick A, Gerhard GS, et al. Altered expression of MALAT1 lncRNA in nonalcoholic steatohepatitis fibrosis regulates CXCL5 in hepatic stellate cells. Transl Res. (2017) 190:25–39.e21. doi: 10.1016/j.trsl.2017.09.001
72. Li JZ, Ye LH, Wang DH, Zhang HC, Li TY, Liu ZQ, et al. The identify role and molecular mechanism of the MALAT1/hsa-mir-20b-5p/TXNIP axis in liver inflammation caused by CHB in patients with chronic HBV infection complicated with NAFLD. Virus Res. (2021) 298:198405. doi: 10.1016/j.virusres.2021.198405
73. Zhang X, Tang X, Liu K, Hamblin MH, Yin KJ. Long non-coding RNA malat1 regulates cerebrovascular pathologies in ischemic stroke. J Neurosci. (2017) 37:1797–806. doi: 10.1523/JNEUROSCI.3389-16.2017
The following abbreviations are used in this manuscript:
Keywords: lncRNAs, MALAT1, mechanism, signaling pathways, liver diseases
Citation: Lu J, Guo J, Liu J, Mao X and Xu K (2022) Long Non-coding RNA MALAT1: A Key Player in Liver Diseases. Front. Med. 8:734643. doi: 10.3389/fmed.2021.734643
Received: 01 July 2021; Accepted: 13 December 2021;
Published: 25 January 2022.
Edited by:
Alice Chen, National Cancer Institute (NCI), United StatesReviewed by:
En-Qiang Chen, Sichuan University, ChinaCopyright © 2022 Lu, Guo, Liu, Mao and Xu. This is an open-access article distributed under the terms of the Creative Commons Attribution License (CC BY). The use, distribution or reproduction in other forums is permitted, provided the original author(s) and the copyright owner(s) are credited and that the original publication in this journal is cited, in accordance with accepted academic practice. No use, distribution or reproduction is permitted which does not comply with these terms.
*Correspondence: Kaijin Xu, emR5eHl4a2pAemp1LmVkdS5jbg==
†These authors share first authorship
Disclaimer: All claims expressed in this article are solely those of the authors and do not necessarily represent those of their affiliated organizations, or those of the publisher, the editors and the reviewers. Any product that may be evaluated in this article or claim that may be made by its manufacturer is not guaranteed or endorsed by the publisher.
Research integrity at Frontiers
Learn more about the work of our research integrity team to safeguard the quality of each article we publish.